ABSTRACT
Biologics (vaccines, monoclonal antibodies (mAb), and genetically modified enzymes) offer a promising class of therapeutics to treat substance use disorders (SUD) involving abuse of opioids and stimulants such as nicotine, cocaine, and methamphetamine. In contrast to small molecule medications targeting brain receptors, biologics for SUD are larger molecules that do not cross the blood-brain barrier (BBB), but target the drug itself, preventing its distribution to the brain and blunting its effects on the central nervous system (CNS). Active and passive immunization approaches rely on antibodies (Ab) that bind drugs of abuse in serum and block their distribution to the brain, preventing the rewarding effects of drugs and addiction-related behaviors. Alternatives to vaccines and anti-drug mAb are genetically engineered human or bacterial enzymes that metabolize drugs of abuse, lowering the concentration of free active drug. Pre-clinical and clinical data support development of effective biologics for SUD.
The need for development of safe and effective therapies for SUD
Substance use disorders are a public health and economic threat worldwide.Citation1 In the U.S., there are approximately 70 million tobacco users and 21.5 million people affected from other SUD.Citation2,3 Abuse of tobacco products accounts for about 500,000 deaths annually causing up to 90% deaths due to lung cancer, cardiovascular, and lower tract respiratory diseases.Citation3 In 2014, an estimated 2.5 million people were dependent on heroin and prescription opioid analgesics, leading to a total of 29,500 deaths by overdose.Citation2 The yearly public cost of SUD exceeds $700 billion in criminal activities, lost work productivity, and health care expenditures.Citation4 Despite the societal impact of SUD, limited therapeutic options exist,Citation5-7 necessitating the development of new strategies for treating SUD.Citation8 This review broadly describes the development of biological therapies for SUD, and mainly focuses on vaccines for SUD. Detailed reviews on the various biological approaches targeting specific drugs of abuse are found elsewhere.Citation9-19
Biologics for SUD: Overview
Biologics for SUD encompass a wide range of therapeutic strategies such as active immunization with vaccines that stimulate generation of polyclonal anti-drug Ab, passive immunization with anti-drug mAb, genetically modified enzymes that degrade drugs of abuse, viral-mediated transfer of genes encoding for anti-drug mAb or enzymes, and catalytic Ab that facilitate drug degradation. Approved medications for SUD are small molecules that provide pharmacodynamic-based therapy targeting CNS receptors involved in drug addiction (e.g., the opioid receptor agonist methadone, or the nicotinic receptor partial agonist varenicline). In contrast, biologics for SUD are larger molecules that do not cross the BBB, but target the drug itself. Biologics for SUD bind or degrade the free drug, preventing its distribution to the brain and blunting its central effects. Due to their pharmacokinetic-based mechanism of action, biologics for SUD may be used in combination with existing pharmacodynamic-based medications. SUD are complex and heterogeneous diseases that will benefit from combination therapies to improve overall treatment outcomes and/or from precision medicine strategies to target specific patient subsets.
Vaccines for SUD
Vaccines for SUD are immunogens consisting of synthetic drug-derived haptens chemically conjugated to immunogenic carriers and packaged in adjuvants to increase immunogenicity and provide an injectable formulation. Vaccination with these conjugate immunogens leads to T cell-dependent B cell activation to generate polyclonal anti-drug Ab that bind free drug in serum. Ab-bound drugs do not cross the BBB, thus reducing drug distribution to the brain and blocking addiction-related behaviors.Citation10,20 Although this approach has shown promising pre-clinical efficacy against nicotine, cocaine, methamphetamine, heroin and prescription opioids, no vaccine for SUD has yet been approved for clinical use. Vaccines for SUD could offer long-lasting, safe, and cost-effective interventions that avoid side effects associated with current addiction medications.
Clinical studies
Clinical evaluation of first-generation vaccines against nicotine and cocaine involved intramuscular injections of immunogens adsorbed on aluminum-based adjuvants.Citation21-23 Immunization schedules included 3–5 monthly injections followed by boosts every 2–6 months to maintain serum anti-drug Ab levels.Citation21-23 summarizes clinical studies of vaccines, and other biologics for SUD. First-generation vaccines for nicotine and cocaine showed proof of efficacy (e.g., smoking cessation, or cocaine-free urine) only in the subset of immunized subjects (∼30%) that achieved the highest anti-drug Ab concentrations (≥40 μg/ml).Citation21-24 Newer vaccine formulations are currently under pre-clinical and early-stage clinical development. Selecta Biosciences developed a polymer-based nanoparticle nicotine vaccine (SEL-068), which recently completed a phase I trial, but data are not yet available (NCT01478893). Pfizer has recently conducted clinical studies to test the safety, tolerability, immunogenicity, and efficacy of 2 lead nicotine immunogens, but results have not been disclosed (NIC7-001, and NIC7-003, NCT01672645). A phase I trial of a cocaine vaccine containing a disrupted adenovirus as carrier is currently recruiting patients (NCT02455479).
Table 1. Clinical trials and human laboratory studies of biologics for SUD.Footnote*
Clinical trials of first-generation SUD vaccines highlight the need to understand why potentially clinically effective Ab responses were achieved only in a fraction of immunized subjects, and how to improve the magnitude, quality and duration of the post-immunization serum Ab response to generate more effective vaccines.
Pre-clinical development
This section first discusses immunological mechanisms underlying generation of polyclonal anti-drug Ab responses, which may help to explain, and predict, post-immunization individual variability in vaccine efficacy against SUD. Then new components, designs, materials, and immunization strategies currently explored in pre-clinical development of next-generation vaccines for SUD are reviewed.
Immunological mechanisms underlying polyclonal Ab generation
After immunization, vaccines are processed by antigen-presenting cells (APC) displaying major histocompatibility complex II (MHC II) receptors. After presentation to B and T cell lymphocytes, generation of Ab relies on CD4+ T helper (Th) cell-dependent B cell activation in germinal centers (GC), within the lymph nodes and spleen.Citation25-30 In the GC, antigen-specific B cells go through isotype switching, affinity maturation and clonal selection.Citation29,30 B cell maturation and differentiation in the GC are supported by T follicular helper (Tfh) cells and GC-Tfh, which are Th subsets uniquely specialized for B cell help.Citation31,32 Germinal center formation is essential for generating long-lived high-affinity plasma cells and switched immunoglobulin memory B cells.Citation25-30 This series of cellular and molecular events is critical for generating long-lasting high-affinity antigen-specific Ab.
a) B and T cell lymphocyte responses to SUD vaccines. Vaccines for SUD consist of drug-derived haptens (B cell epitope) conjugated to larger foreign immunogenic carriers (e.g., proteins or peptides) that provide signaling for activation of T cells (T cell epitope). Characterization of hapten-specific B cells and carrier-specific T cells can help elucidate the cellular and molecular mechanisms underlying generation of effective anti-drug Ab responses in immunized subjects.
To address this question, fluorescent antigen-based magnetic enrichment paired with flow cytometry allows analyses of polyclonal antigen-specific B and T cell populations.Citation33,34 The strength of this approach is that very rare antigen-specific B and T cells are detected prior to, or shortly after immunization.Citation35-39 To date, this approach has been used to test the effect of hapten structure,Citation40,41 adjuvant,Citation42 or host geneticsCitation42 on the B cell response to vaccines for SUD. This strategy has also been used to study the relationship between antigen-specific B and T cells and individual variability in post-immunization Ab titers, or efficacy against drug distribution and drug-induced behavior in mice.Citation41,43 These studies found that naïve and early-activated B cells can discriminate between structurally-related haptens, and that the size of the polyclonal hapten-specific B cell population determines vaccine immunogenicity and efficacy against drugs of abuse.Citation40,41 When comparing individuals, differences in the population size of hapten-specific B cells and carrier-specific T cells are found before and/or after immunization in individual mice, and correlate to vaccine immunogenicity and efficacy.Citation41,43 Hence, analysis of naïve and early-activated B and T cells could be used to examine vaccine formulations and individual variability across subjects.
Development of SUD vaccines has made use of serum Ab subclasses analysis to test whether specific vaccine formulations induced post-immunization anti-drug Ab more characteristic of a Th1- (IgG2a and IgG3 subtypes) or a Th2- (IgG1) response.Citation44-47 Yet, it is not fully understood whether immunization against drugs of abuse benefits from Th2-polarized or more balanced Th1/Th2 responses.Citation44-47 Immunogen dose and adjuvant choice are known to affect CD4+ T cell clonal expansion, differentiation, and polarization.Citation48-50 It is possible that specific polarization patterns in the CD4+ T cell repertoire (Th1 v. Th2, or Th1 v. TfhCitation48,39,51) are associated with increased efficacy of vaccines for SUD. Vaccine formulations could be screened for their ability to induce desired antigen-specific CD4+ T cell subsets (e.g., Tfh and GC-TfhCitation31,32) known to help GC B cell activation, and generation of high-affinity Ab.
b) Frequency of naïve and early-activated vaccine-specific B and T cell subsets correlates to individual vaccine efficacy. Pre-clinical and clinical studies of SUD vaccines showed that post-immunization anti-drug Ab levels and affinity vary greatly across subjects, but the cause of such variability is not clear.Citation10 Before and after immunization, the number of antigen-specific B and T cells in the total lymphocyte repertoire varies in individual miceCitation33,36-39,52-58 and in human subjects.Citation59,60 Consistently, individual variability of similar magnitude has been found in the polyclonal hapten-specific B cell population in blood, peripheral lymph nodes and spleen of various mouse strains, before and after immunization with vaccines for oxycodone and nicotine.Citation40-43 Multiple mechanisms may underlie the pre- and post-immunization variability in the antigen-specific B and T cell subsets and contribute to individual vaccine efficacy. Apoptosis and antigen affinity affect the heterogeneity of the primary immune response by limiting differentiation of a single naïve B cell shortly after immunization.Citation38 Clonal selection and affinity for antigen affect the antigen-specific naïve T cell population size, heterogeneity, and its differentiation into specialized subsets after immunization or infection.Citation36,39,48,51,54-56 These data suggest that optimal activation of both antigen-specific naïve B and T cell populations is a key process for achieving clinically effective Ab responses, and support the hypothesis that variations in the population size of vaccine-specific B or T cells underlie individual efficacy of SUD vaccines.
B cells. The frequency of naïve and/or early-activated hapten-specific B cells correlated post-immunization Ab responses and vaccine efficacy against oxycodoneCitation40,43 and nicotine.Citation41 The size of the polyclonal hapten-specific IgMhigh naïve and memory B cell and GC B cell subsets best correlated with post-vaccination serum anti-drug IgG Ab titers and vaccine efficacy against drug distribution or drug-induced behavior in mice.Citation41,43 A greater number of pre-immunization hapten-specific IgMhigh naïve B cells correlated to increased post-immunization serum anti-oxycodone Ab titers, and efficacy in blocking oxycodone distribution to the brain.Citation43 These data suggest that variations in the pre-immunization polyclonal hapten-specific B cell repertoire underlie individual responses to vaccines for SUD. Immunization with an oxycodone vaccine increased GC activation over time, and the extent of GC activation was highly correlated with individual vaccine efficacy in mice.Citation43 These data suggest that variations in the magnitude and quality of GC formation underlie individual vaccine efficacy. New immunization strategies that enhance GC activation shall be adopted to increase efficacy of vaccines and the fraction of subjects achieving clinically effective anti-drug Ab.
T cells. The carrier is a key component of conjugate immunogens for its role in stimulating specific subsets of cognate CD4+ T cells to help hapten-specific B cell activation.Citation32 Selective depletion of CD4+ T cells blunted clonal expansion of hapten-specific B cells and vaccine efficacy against oxycodone, suggesting that the magnitude of CD4+ T cell activation is key for adequate post-vaccination B cell and Ab responses.Citation43 Using a magnetic enrichment strategy involving use of T cell epitope peptides covalently bound to soluble MHC II receptors labeled with fluorescent proteins, it was shown that greater frequency of carrier-specific MHC II-restricted CD4+ T cells prior to immunization correlated to increased post-immunization efficacy in retaining serum oxycodone.Citation43 These data highlight the importance of carrier-specific CD4+ T cells in contributing to the vaccine efficacy against SUD. Consistently, nanoparticle nicotine vaccines containing MHC II peptide sequences that stimulate CD4+ T cells elicited effective anti-nicotine Ab in mice and non-human primates (NHP), and stimulated recall T cell memory in human peripheral blood mononuclear cells (PBMC) ex vivo.Citation61
c) Vaccine-specific B and T cells are biomarkers predictive of vaccine efficacy. A greater frequency of vaccine-specific B or T cells correlates with more effective immunization against SUD.Citation40,41,43 Early appearance of anti-oxycodone Ab predicted vaccine efficacy in blocking oxycodone distribution to the brain in mice (, reproduced from ref. 43). However, the frequency of hapten-specific B cells in blood prior to, and after immunization was also predictive of vaccine efficacy (, reproduced from ref. 43). Consistently, the frequency of early hapten-specific B cell responses predicted the immunogenicity and efficacy of nicotine vaccines containing structurally different haptens.Citation41 These data suggest that analysis of vaccine-specific B or T cells prior to, or shortly after immunization could be used to anticipate clinically significant individual responses to vaccination against SUD, which will greatly facilitate adaptive trial design, patient stratification, and personalized medicine. Analysis of hapten-specific B cells prior to immunization could be paired to analyses of genetic markers predictive of individual responses to vaccination.Citation62-64 For instance, early screening of vaccine-specific B and T cells in PBMC could be combined with other behavioral, biochemical or genetic tests to predict whether individual, or subsets of, SUD patients will be more likely to benefit from vaccination, pharmacotherapy, or a combination of vaccination and medication.
Figure 1. The frequency of hapten-specific B cells in blood predicts vaccine efficacy. Pre- and post-vaccination 6OXY-specific B cells were analyzed in 0.2 ml of blood collected before and after immunization with either 6OXY-KLH or KLH. Balb/c mice were immunized on days 0, 14 and 28, and challenged with 2.25 mg/kg oxycodone a week after the third immunization to measure the effect of immunization on oxycodone distribution. A) Immunization reduced distribution of oxycodone to the brain. Data are mean ± SEM. B) Early serum IgG antibody titers correlated to subsequent blockage of oxycodone distribution to the brain. C) The frequency of 6OXY-specific IgMhigh B cells prior to immunization correlated with vaccine efficacy in the 6OXY-KLH group. D) Increased frequency of 6OXY-specific IgMhigh B cells 14 days after the first immunization correlated to greater vaccine efficacy on oxycodone distribution to the brain. Frequencies are the % of total lymphocytes in the bound fraction after positive enrichment of blood. Data include 3 independent experiments with a total of n = 12 mice each group. *** p < 0.001 compared to KLH control. Copyright 2015. The American Association of Immunologists, Inc.
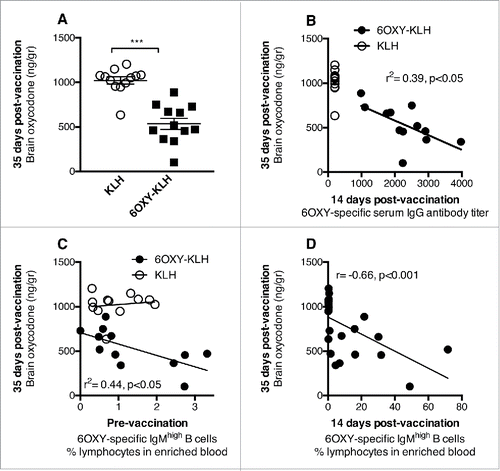
Vaccine design
a) Improving individual vaccine components: hapten, carrier, and adjuvant. Pre-clinical studies have examined the role of hapten and linker design, bioconjugation chemistry, carrier, and adjuvant to improve the anti-drug Ab response and vaccine efficacy.Citation10,20,65-68
Hapten, linker, and bioconjugation chemistry. Drug-based haptens are key components for generating drug-specific Ab. Hapten development is a complex process of screening hapten series containing various chemical modifications and linkers placed at different positions on the target drug molecule, and optimization of lead hapten synthesis and bioconjugation chemistry for coupling to carriers. For instance, increased hapten stability correlated to higher post-immunization anti-cocaine Ab and vaccine efficacy.Citation69 Hapten enantiopurity has been shown to improve quantity and quality of post-vaccination Ab specific for (−)nicotine, suggesting that enantiopure haptens should be favored over racemic mixtures to generate immunogens for clinical evaluation.Citation70 Additionally, hapten fluorination,Citation71 hapten clustering,Citation72 and conformationally-constrained hapten structuresCitation73 have been explored as potential strategies to design more effective haptens. These data suggest that continuous efforts on hapten synthesis will generate more chemically defined and effective haptens.
A candidate vaccine for prescription opioids oxycodone and hydrocodone consists of an oxycodone-based hapten containing a tetraglycine linker at the C6 position (6OXY(Gly)4OH) and conjugated to keyhole limpet hemocyanin (KLH) by carbodiimide chemistry.Citation74-76 The 6OXY(Gly)4-KLH conjugate was more effective in blocking oxycodone distribution to the brain and oxycodone-induced antinociception than vaccines consisting of C6-oxycodone haptens conjugated to KLH through an hemisuccinate linkerCitation74 or thiol-maleimide bondage.Citation75 The 6OXY(Gly)4-KLH was also more effective in blocking hydrocodone distribution to the brain and hydrocodone antinociception than immunogens containing C6- and C8-derivatized hydrocodone haptens.Citation75 Other positions on oxycodone and hydrocodone still remain to be explored. In contrast, development of heroin vaccines explored a wider range of hapten structures, generated from derivatization of the morphinan structure at the C3 and C6 position,Citation77-82 and at the bridgehead nitrogenCitation83,84 (reviewed in detail elsewhereCitation11,19). Although these series of opioid-based immunogens provided a comprehensive and informative structure-function dataset, data interpretation needs to account for differences in conjugation chemistry, haptenization ratio, and hapten or carrier structure.
Optimization of lead immunogens benefits from screening structurally-similar haptens paired with different bioconjugation chemistry (e.g., carbodiimide v. maleimide coupling) to improve hapten density, and vaccine immunogenicity or efficacy.Citation75,85-87 Increased efficacy of a heroin vaccine correlated with higher haptenization ratios in conjugates containing a tetanus toxoid (TT) carrier but not the diphtheria toxin cross-reactive material (CRM197) carrier.Citation88 Yet, haptenization ratio did not correlate with anti-heroin Ab titer nor affinity.Citation88 These data suggest that each hapten-conjugate combination needs to be tailored to specific formulations or immunization regimens, and evaluated for multiple parameters of immunogenicity and efficacy.
A large series of nicotine-based haptens containing linkers of different length, polarity, and flexibility placed at various positions on the nicotine structure was tested for immunogenicity and function.Citation87 This impressive effort yielded a lead immunogen consisting of the 5-aminoethoxy-nicotine (Hapten 7) conjugated to CRM197.Citation87 The optimized NIC7–CRM197 adsorbed on alum plus the toll-like receptor (TLR) 9 agonist CpG adjuvant showed promising pre-clinical efficacy in both mice and NHP,Citation46,87,89 and these candidates were recently evaluated in a clinical trial (). Analysis of several lots of NIC7–CRM197 showed that higher vaccine immunogenicity and efficacy correlated to lower degree of conjugate aggregation, and minimal presence of adducts.Citation89 Also, NIC7–CRM197 conjugates with haptenization ratios between 11 and 18 showed best nicotine-specific Ab levels, affinity for nicotine, and function.Citation89
In summary, hapten chemistry, linker composition and position, bioconjugation chemistry, hapten load, and conjugate purity are important parameters that affect immunogenicity and efficacy of vaccines for SUD.
Carrier. Vaccines for SUD have employed TT, KLH, Cholera Toxin B, recombinant exotoxin A from Pseudomonas aeruginosa (rEPA), CRM197, virus-like particles (VLP), or peptide-based carriers.Citation90-92
To identify the most promising carrier suitable for translation of a vaccine against oxycodone and hydrocodone, BSA, decamer and dimer KLH, TT, and a TT-derived peptide were tested.Citation40,42,43,74-76,93 To develop vaccines for treatment of methamphetamine abuse, for which no therapies exist, immunogens containing either native or dimer KLH, or TT, showed promising pre-clinical efficacy in blocking distribution of methamphetamine to the brain and blocking a variety of methamphetamine-induced behaviors, including intravenous self-administration.Citation94-98
Other carrier proteins have adjuvant-like properties, such as the well-characterized CRM197.Citation99 A cocaine hapten conjugated to the TLR5 agonist flagellin elicited greater cocaine-specific Ab concentrations than the same hapten conjugated to KLH.Citation100 A novel nicotine vaccine employed a peptide-based trimeric coiled-coil (TCC) carrier containing CD4+ T cell epitopes.Citation101 The TCC-based vaccine was delivered with alum plus an additional adjuvant formulation containing the TLR4 agonist glucopyranosyl lipid and a stable oil-in-water emulsion.Citation101 The TCC carrier displayed a higher density of lysines compared to KLH, rEPA, TT or CRM197, permitting higher hapten load. The TCC-based vaccine containing 12 nicotine haptens per trimer performed better than a KLH-conjugate containing 22 nicotine haptens per monomer, but performed similarly to a KLH-conjugate displaying higher hapten density.Citation101
Conjugate nicotine and cocaine vaccines have made use of disrupted adenoviruses as carriers, and showed pre-clinical efficacy in various animal models including NHP.Citation102-105 In this approach, the recombinant E1− E2− replication-deficient serotype 5 adenovirus (Ad5) is disrupted by a combination of chemicals and heat, and its capsid proteins conjugate to haptens.Citation102,103 A cocaine vaccine containing the Ad5-based carrier is now advanced toward clinical evaluation ().
In sum, use of better characterized and more immunogenic carrier proteins with adjuvant-like properties will provide more effective vaccines for SUD. Development of more structurally defined carriers (see next section for particle-based scaffolds) will also improve control of hapten-to-carrier ratio and provide immunogens suitable for pharmaceutical manufacturing and scale-up.
Adjuvant. The most commonly used adjuvants for pre-clinical development of vaccines for SUD have been Freund's complete and incomplete adjuvant, aluminum, the TLR4 agonist monophosphoryl lipid A (MPLA), and the TLR9 agonists class B CpG oligodeoxynucleotides, containing either a phosphodiester or a modified nuclease-resistant phosphorothioate backbone.Citation67 Additional adjuvant options for SUD vaccines include: AS01, a combination of liposomes containing MPLA and QS21 saponin; AS03, an oil-in-water emulsion containing α-tocopherol, squalene, and polysorbate 80; and AS04, a combination of aluminum hydroxide and MPLA.Citation67 Development of SUD vaccines could also benefit from testing other adjuvants of interest, which are at different stages of development or regulatory approval.Citation106 For instance, the Food and Drug Administration (FDA) recently approved MF59, a squalene-based oil-in-water emulsion used in influenza vaccines, which promotes GC B cell and Tfh cell responses,Citation52,107 key features for generation of high-affinity Ab.
Adjuvants, or adjuvant combinations, have been tailored to specific immunogens to determine the best formulation. For instance, an oxycodone vaccine was effective in Freund's and alum adjuvants,Citation40,42,43,74-76,93 but not MPLA.Citation42 The combination of alum and CpG with a candidate vaccine against nicotine improved Ab titer, affinity, nicotine binding capacity, and Ab efficacy in blocking nicotine distribution to the brain, compared to immunization with the same vaccines adsorbed on alum only, in mice and NHP.Citation46,108 Similarly, addition of phosphorothioated CpG to a heroin vaccine adsorbed on alum enhanced Ab titer, affinity, blockage of opioid-induced antinociception.Citation45 Combination of CpG and alum also showed pre-clinical efficacy for vaccines directed against the prescription opioid fentanyl.Citation109 These data suggest that addition of CpG to alum adjuvant improves the quantity, quality, and function of the post-vaccination Ab response, and that this approach will likely improve other vaccines for SUD.
Combinations of vaccines and adjuvants should also be evaluated for immunogenicity and efficacy by different routes of administration.Citation42,45 Intradermal immunization with a nicotine vaccine combined with skin illumination by a laser source, which increases the motility of dendritic cells,Citation110 showed immunogenicity and efficacy comparable to intramuscular immunization with the same vaccine adsorbed on alum, MPLA, and MPLA/CpG.Citation111 Although translation of new adjuvants is often limited by toxicity, side effects, proprietary restrictions, or regulatory approval, it will be important to test available pre-clinical stage, licensed, and/or non-traditional adjuvants to constantly improve the efficacy of SUD vaccines.
b) Particle-based vaccines for SUD: new materials. Particles provide flexible platforms for delivery of vaccines and adjuvants, and offer the unique advantage of building defined vaccine scaffolds. Micro- and nano-particles are built from polymers, liposomes, VLP, self-assembly peptides, and complex combinations of other materials.Citation112-114 In contrast to soluble formulations, particle-based delivery is expected to enhance vaccine immunogenicity and efficacy by providing enhanced APC stimulation, precise stoichiometry or geometry of vaccine components, controlled hapten load, focused delivery at injection site of adjuvants or other immunomodulators, and creation of a depot effect for sustained release.
VLP. Virus-like particles are known to promote memory B cell differentiation and induced CD4+ T cell help.Citation115,116 A nicotine vaccine using a VLP scaffold derived from the bacteriophage Qβ advanced to clinical evaluation (Nic-Qβ).Citation21 Similarly to other first-generation nicotine vaccines, Nic-Qβ showed efficacy only in the fraction of subsets that achieved the highest anti-nicotine Ab levels.Citation21 A malaria vaccine containing Plasmodium falciparum epitopes displayed on the surface of VLP from hepatitis B and administered in AS01 adjuvant showed clinical efficacy in phase III trials,Citation117,118 received regulatory approval from the European Medicines Agency (EMA), and is now the world's first licensed malaria vaccine (Mosquirix®). These data indicate that VLP are safe and effective scaffolds capable of inducing long-lasting immune responses, suggesting that more VLP-based formulations should be evaluated in SUD vaccines.
Liposomes. Use of liposomes, or their combination with other materials, is a general and versatile approach that could generate novel scaffolds for SUD vaccines. Strategies for development of SUD vaccines have included: heroin immunogens combined with, or conjugated to, liposomes containing MPLACitation90; a methamphetamine vaccine combined with liposomes containing a lipid analog of the immunomodulator tucaserolCitation119; and nicotine immunogens conjugated to an adjuvanted lipid vesicle platform.Citation120 A novel nicotine vaccine was built from nicotine-BSA conjugated to a liposome-based particle, and described as a lipoplex complex.Citation121 A second-generation lipoplex contained a carbon-based nanoparticle backbone, called a nanohorn, that increased the stability of the liposome structure,Citation47 and showed greater nicotine-specific Ab titers than the nicotine-BSA conjugated to lipoplexes without the nanohorn scaffold.Citation47 It will be of interest to test whether other adjuvants, immunomodulators, or carriers could be incorporated into liposomes or liposome-based nanoparticles.
Polymers. Selecta Bioscience's nanoparticle nicotine vaccine (SEL-068) consists of polymers conjugated to nicotine haptens and TLR ligands, and contains immunogenic peptides (). The SEL-068 has shown pre-clinical efficacy in mice and NHP,Citation61,122 selectively blocking nicotine discrimination and decreasing behavioral effects of nicotine in squirrel monkeys.Citation92 The effect of immunization was greater in naive than nicotine-experienced squirrel monkeys, suggesting that immunization approaches may be more effective in treating light smokers, subjects at early stages of tobacco addiction, or those at risk of relapse.Citation123 The Selecta Bioscience group also showed that polymer encapsulation increased adjuvant properties of a TLR ligand and immunogenicity of a model antigen, and reduced the likelihood of adjuvant systemic effects.Citation124 These data support further studies to explore whether polymer-based vaccine designs or delivery platforms will generate more effective vaccines for SUD.
Other strategies. Approaches for improving antigen presentation include DNA-protein structures,Citation125 self-assembled peptide-based nanofibers,Citation126,127 and coat glycoproteins of the T. brucei parasite.Citation128 These approaches incorporate cutting-edge materials and delivery systems that are being investigated in vaccines for other indications (e.g., carbohydrate vaccinesCitation129), and that can be harnessed to design micro- and nano-particle vaccines for SUD. Vaccine physical-chemical characteristics, including size, molecular patterns, and geometry play a role in vaccine delivery and efficacy.Citation130 Hence, biomaterials can be used to rationally design vaccines that exploit pathogen's features including spatially organized antigens to stimulate B cell receptor (BCR) cross-linking, and display of appropriate signals to stimulate APC uptake, and antigen processing.Citation131 Alternatively, synthetic vaccines can be functionalized for targeted delivery to lymph nodes and GC activation.Citation132,133 Although particles and new materials allow for development of more structurally defined vaccines, it is of interest to test whether their efficacy will be superior to more traditional hapten-protein conjugate immunogens.
Immunization strategies
To increase a vaccine's efficacy, it is possible to co-administer different vaccines in multivalent immunization strategies. A pediatric trivalent vaccine targets tetanus, diphtheria, and acellular pertussis (TDaP®), while a 23-valent pneumococcal polysaccharide vaccine (Pneumovax®) is effective against 23 serotypes of S. pneumonia. Multivalent immunization strategies have also shown pre-clinical efficacy against nicotine and opioids.Citation93,134 Nicotine vaccines containing structurally-related haptens elicit non-overlapping anti-nicotine Ab responses, possibly through activation of distinct hapten-specific B cells populations.Citation86 To target heroin and prescription opioids, co-administration of an oxycodone vaccine with an analogous morphine vaccine blocked both oxycodone and the active heroin metabolite 6-monoacetyl-morphine (6-MAM) in rats.Citation93 Additionally, an unexpected synergistic effect of bivalent immunization elicited greater anti-opioid Ab titers, and efficacy to block oxycodone and 6-MAM distribution compared to individual immunogens.Citation93 These data suggest that combining different vaccines may achieve greater efficacy against the target drug(s), and that multivalent immunization strategies increase vaccine efficacy, maintain selective blockade of specific compounds, and provide protection against multiple compounds. This approach may generalize to other SUD.
General considerations for development and translation of vaccines for SUD
Selection criteria for next-generation vaccines
Although clinical evaluation of first-generation vaccines set benchmarks for the minimum requirements of clinically effective anti-drug Ab responses, there are no absolute criteria for successful immunization against SUD. Measurement of anti-drug Ab titers is a well-established parameter of immunogenicity, but methodological differences between laboratories or vaccine formulations complicate comparisons. Pre-clinical development plans should include stringent and standard go/no-go criteria of vaccine immunogenicity and efficacy. Screening of new vaccine candidates should include analyses of anti-drug serum Ab titer or concentration, binding/ avidity/ affinity/ selectivity for the target drug, or other measures of Ab function. Characterization of the anti-drug Ab subclasses and analysis of post-immunization B and T cell population subsets will provide additional insights into the mechanisms underlying the efficacy of specific vaccine formulations. Vaccine candidates displaying promising immunogenicity profiles should be tested for efficacy in adequate pre-clinical models of SUD. Screenings should include testing the effect of immunization on drug distribution to the serum and to the brain, drug-induced behaviors, or drug-induced alteration of brain neurochemistry. To increase the likelihood of translation, lead vaccines should also display pre-clinical efficacy across strains or species. A detailed review of animal models for screening of candidate vaccines for SUD can be found elsewhere.Citation135
Realistic expectations for vaccines for SUD
Although vaccines represent one of the most effective interventions introduced in medical practice,Citation136 no vaccine is 100% effective. Based on the track record of vaccines for infectious diseases, it is not realistic to expect that vaccines for SUD, or other non-communicable chronic diseases, will be effective in all immunized subjects. To increase the likelihood of successful translation of SUD vaccines, a few considerations need to be made: (1) Most vaccines for infectious diseases are prophylactic interventions administered in neonates, infants and small children to prevent infection or block toxin activity when exposed. Non-standard immunization regimens are also administered to at-risk subjects prior to exposure. Vaccines for tropical diseases (e.g., yellow fever, or Japanese encephalitis) and biological warfare agents (e.g., Biothrax against Bacillus antracis toxin) are administered prior to travel, or deployment of military personnel in endemic areas. In contrast, therapeutic vaccines for SUD are tested in current drug users, when the concentration of drug in serum and brain greatly exceeds the number of drug-specific binding sites on Ab (i.e., stoichiometric ratio of drug>biologic). It would be more practical to test their clinical efficacy in preventing early escalation of drug use after an initial exposure, or relapse after a period of abstinence. Clinical use of SUD vaccines could also benefit selected at-risk populations, younger addicts in the early stages of drug abuse, or people who have quit, and are at-risk of relapse. (2) Most vaccines for infectious diseases rely on herd immunity, which significantly reduces the overall possibility of transmission. In contrast, vaccines for non-communicable diseases do not benefit from herd immunity. Therefore, their efficacy depends exclusively on individual responses. (3) In clinical trials of vaccines for SUD, analyses of B and T cells in blood could be performed before and after immunization to guide patient stratification and predict individual vaccine responses. Biomarkers predictive of vaccine efficacy could improve therapeutic outcomes by identifying patients most likely to benefit from vaccination. (4) Clinical-stage vaccines for SUD have been tested in adults using 3–5 monthly injections to quickly stimulate high levels of anti-drug Ab. In contrast, most vaccines for infectious diseases are administered to children and adults using longer immunization schedules (see http://www.cdc.gov/vaccines) to stimulate development of long-term high-affinity memory and plasma B cells over time. Vaccines for SUD should be tested through various immunization regimens, including schedules similar to vaccines for infectious diseases to increase the likelihood of generating high-affinity long-lived plasma and memory B cells.
Other biologics for SUD
Clinical studies
mAb
The challenges of achieving post-vaccination clinically effective serum polyclonal Ab levels sufficient to effectively curb SUD may be overcome by delivering high doses of high-affinity anti-drug mAb. Administration of anti-drug mAb is a strategy for treating drug dependence or overdose that has shown pre-clinical proof of efficacy against nicotine,Citation137,138 cocaine,Citation139-141 phencyclidine,Citation142 methamphetamine,Citation143 and heroin.Citation144,145 The first human study of the anti-methamphetamine chimeric mAb7F9, 0.2–20 mg/kg i.v., showed safety (NCT01603147, ), and reported mAb's half-life of 17–19 days.Citation146 The authors also detected anti-mAb auto-Ab, a sign of immunogenicity, in 4 out of 32 subjects, but concluded that more studies were needed to understand their biological relevance or their impact on the function of anti-methamphetamine mAb.Citation146 To facilitate FDA approval, it will be useful to characterize recombinant humanized anti-drug mAb for presence of post-translational modifications, resulting in protein heterogeneity, that may potentially affect in vivo efficacy.Citation147 Although clinical data show promise for mAb-based therapy for SUD, long-term treatment of SUD will require multiple mAb doses throughout the entire course of therapy and may still be limited by cost. Monoclonal Ab are currently the most expensive class of biologics, but their costs will likely decrease as more effective manufacturing technologies are developed and more mAb are granted regulatory approval.
Enzymes
Another class of biologics for SUD consists of delivering enzymes that quickly metabolize or degrade the target active drug, thus preventing its neuropharmacological and behavioral effects.Citation9,18 Cocaine-degrading enzymes derived from human plasma butyrylcholinesterases (BChE) and bacterial cocaine esterases (CocE) have been tested for treatment of cocaine dependence and overdose (NCT01887366, and NCT01846681, ).Citation148-150 These clinical studies showed no evidence of side effects, and provide proof of concept for enzyme-based SUD treatments. A detailed review of clinical studies of cocaine esterases can be found elsewhere.Citation17 Similar to mAb, enzyme-based therapies are not as limited by medication dose as vaccines. Enzymes may be more cost-effective than mAb, but no projected costs per dose have been currently disclosed.
Pre-clinical development
Passive immunization
Although the first-generation of anti-drug mAb made use of classic hybridoma technology, newer strategies can generate cost effective and longer lasting mAb, and more efficient delivery systems. To improve mAb half-life, a nanotechnology-based approach generated anti-methamphetamine mAb conjugated to dendrimers, known as dendribodies, which showed proof of pre-clinical efficacy.Citation151 Antigen-specific mAb can also be directly isolated from B cells of vaccinated subjects. Using various strategies, antigen-specific B cells are isolated from PBMC by cell sorting and their BCR is sequenced, cloned, and expressed in mammalian cell systems to produce antigen-specific mAb.Citation152-154 This approach has been used to produce mAb against infectious diseases,Citation153 but also to isolate high-affinity anti-nicotine mAb.Citation155 Isolation of anti-drug mAb from human B cells is a general approach, and therefore applies to other SUD.
Another approach to improve efficacy of passive immunization is to combine anti-drug mAb with medications or other biologics. An anti-nicotine mAb combined with the nicotine receptor antagonist mecamylamine showed a synergistic effect for blocking nicotine drug discrimination, a behavioral task that measures the subjective effects of drugs of abuse.Citation156 Combination of anti-drug mAb and antagonists could lower the costs associated with mAb, or reduce medications' side effects by reducing the dose of mAb or drug required to achieve clinical efficacy. Anti-drug mAb can also be combined with vaccines to enhance overall efficacy of both approaches.Citation157,158 These data suggest that biologics are well-suited for combination therapy.
Enzymes
Clinical studies of cocaine-degrading BChE and CocE () provided proof of concept for using enzymes as therapeutics for treating SUD. To speed translation and improve clinical efficacy, it will be important to develop methods for production of longer lasting, cost effective, and high activity cocaine-degrading enzymes. Plants may provide a solution to scale-up production of cocaine-hydrolyzing variants of BChE.Citation159 Rational design of amino acid mutations and in silico approaches also guided design of BChE mutants with longer half-life or greater catalytic efficiency against cocaine.Citation160,161 Similarly, polymer-based modifications increased the half-life of a bacterial CocE.Citation162 These studies offer a snapshot of the numerous strategies employed to improve the efficacy of BChE and CocE.
Co-administration of cocaine hydrolases with cocaine vaccines showed additive, and possibly synergistic, effects in pre-clinical models.Citation163 These data support the validity of combining biological approaches acting through different mechanisms, and to further explore this approach against other SUD.
A nicotine-degrading bacterial enzyme, the flavin-dependent NicA2, has also been identified and isolated from strains of Pseudomonas putida found in tobacco-cultivated soil.Citation164 Biochemical characterization of NicA2 revealed promising stability at physiological conditions, and showed nicotine-degradation ex vivo.Citation164 Further in-depth studies involving enzyme engineering to improve catalytic activity against nicotine, and in vivo testing in pre-clinical models of nicotine addiction, are warranted. In sum, pre-clinical and clinical studies showed that use of genetically engineered enzymes is a promising approach for treatment of SUD, which could also be combined with other biological or pharmacological approaches to increase overall treatment outcome.
Catalytic Ab, and other biologics
Antibodies directed against transition-state analogs of cocaine catalyze the non-enzymatic hydrolysis of the cocaine benzoyl ester group, and are dubbed catalytic Ab.Citation165 From this first report,Citation165 a variety of strategies to induce catalytic polyclonal Ab through active immunization, or deliver catalytic mAb was examined.Citation18 Use of catalytic Ab has also been explored for methamphetamineCitation166 and nicotine.Citation167 In contrast to enzymes such as BChE or CocE, it has been challenging to further improve activity of catalytic Ab because their mechanism of action is to stabilize the transition state in non-enzymatic cocaine hydrolysis.Citation9,18 As a cautionary warning for readers, this section highlights the importance of exploring catalytic Ab as a potential SUD treatment, but it does not provide a detailed review.
A recent study reported the development of a novel biological agent against cocaine that incorporates both the long half-life of an Ab and the high catalytic activity of a cocaine hydrolase (CocH).Citation168 The Ab-enzyme hybrid was constructed by replacing each variable (Fab) region of a human IgG1 with a CocH, and by retaining the Ab constant region (Fc). The dimeric CocH-Fc fusion enzyme displayed high efficiency for cocaine hydrolysis, and Fc portion-dependent prolonged half-life.Citation168 This study shows how the field of biologics for SUD is constantly evolving.
Gene therapy
To improve passive immunization efficacy, and circumvent some of the limitations of mAb-based therapy, gene therapy has been explored as a new strategy to deliver anti-drug mAb.Citation169,170 This approach made use of adeno-associated viral-mediated transfer of genes encoding full anti-nicotine and anti-cocaine mAb, and showed efficacy in various pre-clinical models.Citation169,170
Gene transfer strategies have also been examined to prolong, or enhance, the effect of cocaine hydrolases.Citation12 Viral vector-mediated gene transfer of a BChE-based mutant engineered for high catalytic activity, showed pre-clinical efficacy alone, or in combination with a cocaine vaccine, by blocking cocaine-induced motor activity in mice and rats challenged with doses up to 120 mg/kg.Citation171 Additionally, helper-dependent adenoviral vector-mediated delivery of cocaine hydrolases showed long-term efficacy in blocking cocaine self-administration in rats.Citation172
To facilitate translation and regulatory approval, gene therapy approaches for treatment of SUD will need to be carefully evaluated for host immune responses to the viral vector components and other potential adverse effects in chronic toxicology studies. For instance, viral-mediated transfer of BChE did not show signs of motor, cognitive, or cardiovascular toxicity in mice and rats.Citation172-175 Despite promising pre-clinical proof of efficacy and safety, it is not yet clear whether gene transfer approaches will be acceptable by the FDA, EMA, or other regulatory agencies. To date, the FDA's Center for Biologics Evaluation and Research (CBER) has not yet approved any human gene therapy product for market.
Biologics for SUD: Economic incentives for translation
Medication development is a costly and laborious process. The potential revenue from a biologic for SUD could be roughly estimated from sales data of current medications for SUD.Citation8 Varenicline (Chantix®, Pfizer) made $647 million in worldwide sales last year, of which $377 million was made in the U.S. Depot naltrexone (Vivitrol®, Alkermes) made a total net sale of $94.2 millions in 2014 in the U.S. (www.alkermes.com). Sublingual buprenorphine/naloxone (Suboxone®, Reckitt Benckiser Pharmaceuticals) reached total net sales of $7 million in 2015, ranking 17th among the 100 best-selling, most prescribed branded drugs in the U.S. between April 2014-March 2015 (www.medscape.com). These data support development of biologics for SUD and steps toward technology commercialization through academic entrepreneurial activities, academia-private sector collaborations, NIH-funded small-business innovation research (SBIR) awards, attracting private investors, or licensing to pharmaceutical companies.
Conclusions
Vaccines and other biologics offer promising strategies to treat SUD. Pre-clinical and clinical studies will benefit from a better understanding of the cellular and molecular mechanisms underlying activation of specific B and T cell lymphocyte populations involved in generation of effective polyclonal anti-drug Ab responses. These data will provide a blueprint for rational development of more effective next-generation vaccines for SUD. B or T cell-based blood biomarkers predictive of vaccine efficacy will likely support patient stratification in clinical trials and identify patient subsets that will benefit from vaccination, or other therapeutic options. Development of newer hapten synthesis strategies, use of more immunogenic and well characterized carriers, better adjuvants, modular or particle scaffolds, and cutting-edge biomaterials will generate more effective next-generation vaccines for SUD. In addition to vaccines, other biologics such as anti-drug mAb and drug-degrading enzymes have shown promising pre-clinical and clinical outcomes. Once approved, biologics will expand the arsenal of therapeutic options to treat SUD. In the clinic, vaccines, anti-drug mAb and enzymes, and/or medications may be administered as combination therapy to circumvent the shortcomings of each individual line of treatment or as individualized therapy to target specific patient populations.
Disclosure of potential conflicts of interest
No potential conflicts of interest were disclosed.
Funding
NIH DA038876 and Minneapolis Medical Research Foundation Translational Research Program Award.
References
- United Nations Office on Drugs and Crime (UNODC). World Drug Report 2015. Available at: http://www.unodc.org/wdr2015/
- National Survey on Drug Use and Health (NSDUH), 2014. United States Department of Health and Human Services. Substance Abuse and Mental Health Services Administration. Center for Behavioral Health Statistics and Quality. http://doi.org/10.3886/ICPSR36361.v1
- CDC. Wide-ranging OnLine Data for Epidemiologic Research (WONDER); 2014. Available at: http://wonder.cdc.gov/
- U.S. Department of Justice, Drug Enforcement Administration. National Drug Threat Assessment Summary, 2015. Available at: https://www.dea.gov/docs/2015%20NDTA%20Report.pdf
- Dodrill CL, Helmer DA, Kosten TR. Prescription pain medication dependence. Am J Psychiatry 2011; 168(5):466-71; PMID:21536702; http://dx.doi.org/10.1176/appi.ajp.2010.10020260
- Hartmann-Boyce J, Stead LF, Cahill K, Lancaster T. Efficacy of interventions to combat tobacco addiction: Cochrane update of 2013 reviews. Addiction 2014; 109(9):1414-25; PMID:24995905; http://dx.doi.org/10.1111/add.12633
- Stotts AL, Dodrill CL, Kosten TR. Opioid dependence treatment: options in pharmacotherapy. Expert Opin Pharmacother 2009; 10(11):1727-40; PMID:19538000; http://dx.doi.org/10.1517/14656560903037168
- Skolnick P, Volkow ND. Addiction therapeutics: obstacles and opportunities. Biol Psychiatry 2012; 72(11):890-1; PMID:23121867; http://dx.doi.org/10.1016/j.biopsych.2012.08.004
- Narasimhan D, Woods JH, Sunahara RK. Bacterial cocaine esterase: a protein-based therapy for cocaine overdose and addiction. Future Med Chem 2012; 4(2):137-50; PMID:22300094; http://dx.doi.org/10.4155/fmc.11.194
- Pentel PR, LeSage MG. New directions in nicotine vaccine design and use. Adv Pharmacol 2014; 69:553-80; PMID:24484987; http://dx.doi.org/10.1016/B978-0-12-420118-7.00014-7
- Stowe GN, Schlosburg JE, Vendruscolo LF, Edwards S, Misra KK, Schulteis G, Zakhari JS, Koob GF, Janda KD. Developing a vaccine against multiple psychoactive targets: a case study of heroin. CNS Neurol Disord Drug Targets 2011; 10(8):865-75; PMID:22229311; http://dx.doi.org/10.2174/187152711799219316
- Brimijoin S. Interception of cocaine by enzyme or antibody delivered with viral gene transfer: a novel strategy for preventing relapse in recovering drug users. CNS Neurol Disord Drug Targets 2011; 10(8):880-91; PMID:22229308; http://dx.doi.org/10.2174/187152711799219398
- Brimijoin S, Gao Y. Cocaine hydrolase gene therapy for cocaine abuse. Future Med Chem 2012; 4(2):151-62; PMID:22300095; http://dx.doi.org/10.4155/fmc.11.183
- Brimijoin S, Shen X, Orson F, Kosten T. Prospects, promise and problems on the road to effective vaccines and related therapies for substance abuse. Expert Rev Vaccines 2013; 12(3):323-32; PMID:23496671; http://dx.doi.org/10.1586/erv.13.1
- Orson FM, Wang R, Brimijoin S, Kinsey BM, Singh RA, Ramakrishnan M, Wang HY, Kosten TR. The future potential for cocaine vaccines. Expert Opin Biol Ther 2014; 14(9):1271-83; PMID:24835496; http://dx.doi.org/10.1517/14712598.2014.920319
- Peterson EC, Gentry WB, Owens SM. Customizing monoclonal antibodies for the treatment of methamphetamine abuse: current and future applications. Adv Pharmacol 2014; 69:107-27; PMID:24484976; http://dx.doi.org/10.1016/B978-0-12-420118-7.00003-2
- Skolnick P. Biologic approaches to treat substance-use disorders. Trends Pharmacol Sci 2015; 36(10):628-35; PMID:26435208; http://dx.doi.org/10.1016/j.tips.2015.07.002
- Zheng F, Zhan CG. Structure-and-mechanism-based design and discovery of therapeutics for cocaine overdose and addiction. Org Biomol Chem 2008; 6(5):836-43; PMID:18292872; http://dx.doi.org/10.1039/B716268E
- Montoya ID, editor. Biologics to treat substance use disorders: vaccines, monoclonal antibodies, and enzymes. Cham, Switzerland: Springer; 2016
- Shen XY, Orson FM, Kosten TR. Vaccines against drug abuse. Clin Pharmacol Ther 2012; 91(1):60-70; PMID:22130115; http://dx.doi.org/10.1038/clpt.2011.281
- Cornuz J, Zwahlen S, Jungi WF, Osterwalder J, Klingler K, van Melle G, Bangala Y, Guessous I, Muller P, Willers J, et al. A vaccine against nicotine for smoking cessation: a randomized controlled trial. PLoS One 2008; 3(6):e2547; PMID:18575629
- Hatsukami DK, Jorenby DE, Gonzales D, Rigotti NA, Glover ED, Oncken CA, Tashkin DP, Reus VI, Akhavain RC, Fahim RE, et al. Immunogenicity and smoking-cessation outcomes for a novel nicotine immunotherapeutic. Clin Pharmacol Ther 2011; 89(3):392-9; PMID:21270788
- Martell BA, Orson FM, Poling J, Mitchell E, Rossen RD, Gardner T, Kosten TR. Cocaine vaccine for the treatment of cocaine dependence in methadone-maintained patients: a randomized, double-blind, placebo-controlled efficacy trial. Arch Gen Psychiatry 2009; 66(10):1116-23; PMID:19805702
- Fahim RE, Kessler PD, Kalnik MW. Therapeutic vaccines against tobacco addiction. Expert Rev Vaccines 2013; 12(3):333-42; PMID:23496672
- McHeyzer-Williams LJ, McHeyzer-Williams MG. Antigen-specific memory B cell development. Annu Rev Immunol 2005; 23:487-513; PMID:15771579
- McHeyzer-Williams M, Okitsu S, Wang N, McHeyzer-Williams L. Molecular programming of B cell memory. Nat Rev Immunol 2012; 12(1):24-34
- Shulman Z, Gitlin AD, Targ S, Jankovic M, Pasqual G, Nussenzweig MC, Victora GD. T follicular helper cell dynamics in germinal centers. Science 2013; 341(6146):673-7; PMID:23887872
- Tarlinton DM. Evolution in miniature: selection, survival and distribution of antigen reactive cells in the germinal centre. Immunol Cell Biol 2008; 86(2):133-8; PMID:18180800
- Victora GD, Mesin L. Clonal and cellular dynamics in germinal centers. Curr Opin Immunol 2014; 28:90-6; PMID:24681449
- Victora GD, Nussenzweig MC. Germinal centers. Annu Rev Immunol 2012; 30:429-57; PMID:22224772
- Crotty S. Follicular helper CD4 T cells (TFH). Annu Rev Immunol 2011; 29:621-63; PMID:21314428
- Crotty S. A brief history of T cell help to B cells. Nat Rev Immunol 2015; 15(3):185-9; PMID:25677493
- Pape KA, Taylor JJ, Maul RW, Gearhart PJ, Jenkins MK. Different B cell populations mediate early and late memory during an endogenous immune response. Science 2011; 331(6021):1203-7; PMID:21310965
- Moon JJ, Chu HH, Hataye J, Pagan AJ, Pepper M, McLachlan JB, Zell T, Jenkins MK. Tracking epitope-specific T cells. Nat Protoc 2009; 4(4):565-81; PMID:19373228
- Jenkins MK, Chu HH, McLachlan JB, Moon JJ. On the composition of the preimmune repertoire of T cells specific for Peptide-major histocompatibility complex ligands. Annu Rev Immunol 2010; 28:275-94; PMID:20307209
- Jenkins MK, Moon JJ. The role of naive T cell precursor frequency and recruitment in dictating immune response magnitude. J Immunol 2012; 188(9):4135-40; PMID:22517866; http://dx.doi.org/10.4049/jimmunol.1102661
- Taylor JJ, Martinez RJ, Titcombe PJ, Barsness LO, Thomas SR, Zhang N, Katzman SD, Jenkins MK, Mueller DL. Deletion and anergy of polyclonal B cells specific for ubiquitous membrane-bound self-antigen. J Exp Med 2012; 209(11):2065-77; PMID:23071255; http://dx.doi.org/10.1084/jem.20112272
- Taylor JJ, Pape KA, Steach HR, Jenkins MK. Humoral immunity. Apoptosis and antigen affinity limit effector cell differentiation of a single naive B cell. Science 2015; 347(6223):784-7; PMID:25636798; http://dx.doi.org/10.1126/science.aaa1342
- Tubo NJ, Pagan AJ, Taylor JJ, Nelson RW, Linehan JL, Ertelt JM, Huseby ES, Way SS, Jenkins MK. Single naive CD4(+) T Cells from a diverse repertoire produce different effector cell types during infection. Cell 2013; 153(4):785-96; PMID:23663778; http://dx.doi.org/10.1016/j.cell.2013.04.007
- Taylor JJ, Laudenbach M, Tucker AM, Jenkins MK, Pravetoni M. Hapten-specific naive B cells are biomarkers of vaccine efficacy against drugs of abuse. J Immunol Methods 2014; 405:74-86; PMID:24462800; http://dx.doi.org/10.1016/j.jim.2014.01.010
- Laudenbach M, Tucker AM, Runyon SP, Carroll FI, Pravetoni M. The frequency of early-activated hapten-specific B cell subsets predicts the efficacy of vaccines for nicotine dependence. Vaccine 2015; 33(46):6332-9; PMID:26409811; http://dx.doi.org/10.1016/j.vaccine.2015.09.015
- Pravetoni M, Vervacke JS, Distefano MD, Tucker AM, Laudenbach M, Pentel PR. Effect of currently approved carriers and adjuvants on the pre-clinical efficacy of a conjugate vaccine against oxycodone in mice and rats. PLoS One 2014; 9(5):e96547; PMID:24797666; http://dx.doi.org/10.1371/journal.pone.0096547
- Laudenbach M, Baruffaldi F, Vervacke JS, Distefano MD, Titcombe PJ, Mueller DL, Tubo NJ, Griffith TS, Pravetoni M. The frequency of naive and early-activated hapten-specific B cell subsets dictates the efficacy of a therapeutic vaccine against prescription opioid abuse. J Immunol 2015; 194(12):5926-36; PMID:25972483; http://dx.doi.org/10.4049/jimmunol.1500385
- Bremer PT, Janda KD. Investigating the effects of a hydrolytically stable hapten and a Th1 adjuvant on heroin vaccine performance. J Med Chem 2012; 55(23):10776-80; PMID:23134263; http://dx.doi.org/10.1021/jm301262z
- Bremer PT, Schlosburg JE, Lively JM, Janda KD. Injection route and TLR9 agonist addition significantly impact heroin vaccine efficacy. Mol Pharm 2014; 11(3):1075-80; PMID:24517171; http://dx.doi.org/10.1021/mp400631w
- McCluskie MJ, Pryde DC, Gervais DP, Stead DR, Zhang N, Benoit M, Robertson K, Kim IJ, Tharmanathan T, Merson JR, et al. Enhancing immunogenicity of a 3'aminomethylnicotine-DT-conjugate anti-nicotine vaccine with CpG adjuvant in mice and non-human primates. Int Immunopharmacol 2013; 16(1):50-6; PMID:23562759; http://dx.doi.org/10.1016/j.intimp.2013.03.021
- Zheng H, Hu Y, Huang W, de Villiers S, Pentel P, Zhang J, Dorn H, Ehrich M, Zhang C. Negatively charged carbon nanohorn supported cationic liposome nanoparticles: A novel delivery vehicle for anti-nicotine vaccine. J Biomed Nanotechnol 2015; 11(12):2197-210; PMID:26510313; http://dx.doi.org/10.1166/jbn.2015.2156
- Tubo NJ, Jenkins MK. TCR signal quantity and quality in CD4 T cell differentiation. Trends Immunol 2014; 35(12):591-6; PMID:25457838; http://dx.doi.org/10.1016/j.it.2014.09.008
- Malherbe L, Mark L, Fazilleau N, McHeyzer-Williams LJ, McHeyzer-Williams MG. Vaccine adjuvants alter TCR-based selection thresholds. Immunity 2008; 28(5):698-709; PMID:18450485; http://dx.doi.org/10.1016/j.immuni.2008.03.014
- van Panhuys N, Klauschen F, Germain RN. T-cell-receptor-dependent signal intensity dominantly controls CD4(+) T cell polarization in vivo. Immunity 2014; 41(1):63-74; PMID:24981853; http://dx.doi.org/10.1016/j.immuni.2014.06.003
- Tubo NJ, Fife BT, Pagan AJ, Kotov DI, Goldberg MF, Jenkins MK. Most microbe-specific naive CD4(+) T cells produce memory cells during infection. Science 2016; 351(6272):511-4; PMID:26823430; http://dx.doi.org/10.1126/science.aad0483
- Lofano G, Mancini F, Salvatore G, Cantisani R, Monaci E, Carrisi C, Tavarini S, Sammicheli C, Rossi Paccani S, Soldaini E, et al. Oil-in-water emulsion MF59 increases Germinal Center B cell differentiation and persistence in response to vaccination. J Immunol 2015; 195(4):1617-27; PMID:26170383; http://dx.doi.org/10.4049/jimmunol.1402604
- Baumjohann D, Preite S, Reboldi A, Ronchi F, Ansel KM, Lanzavecchia A, Sallusto F. Persistent antigen and germinal center B cells sustain T follicular helper cell responses and phenotype. Immunity 2013; 38(3):596-605; PMID:23499493; http://dx.doi.org/10.1016/j.immuni.2012.11.020
- Chu HH, Moon JJ, Kruse AC, Pepper M, Jenkins MK. Negative selection and peptide chemistry determine the size of naive foreign peptide-MHC class II-specific CD4+ T cell populations. J Immunol 2010; 185(8):4705-13; PMID:20861357; http://dx.doi.org/10.4049/jimmunol.1002276
- Chu HH, Moon JJ, Takada K, Pepper M, Molitor JA, Schacker TW, Hogquist KA, Jameson SC, Jenkins MK. Positive selection optimizes the number and function of MHCII-restricted CD4+ T cell clones in the naive polyclonal repertoire. Proc Natl Acad Sci U S A 2009; 106(27):11241-5; PMID:19541603; http://dx.doi.org/10.1073/pnas.0902015106
- Moon JJ, Chu HH, Pepper M, McSorley SJ, Jameson SC, Kedl RM, Jenkins MK. Naive CD4(+) T cell frequency varies for different epitopes and predicts repertoire diversity and response magnitude. Immunity 2007; 27(2):203-13; PMID:17707129; http://dx.doi.org/10.1016/j.immuni.2007.07.007
- Taylor JJ, Jenkins MK, Pape KA. Heterogeneity in the differentiation and function of memory B cells. Trends Immunol 2012; 33(12):590-7; PMID:22920843; http://dx.doi.org/10.1016/j.it.2012.07.005
- Taylor JJ, Pape KA, Jenkins MK. A germinal center-independent pathway generates unswitched memory B cells early in the primary response. J Exp Med 2012; 209(3):597-06; PMID:22370719; http://dx.doi.org/10.1084/jem.20111696
- Becattini S, Latorre D, Mele F, Foglierini M, De Gregorio C, Cassotta A, Fernandez B, Kelderman S, Schumacher TN, Corti D, et al. T cell immunity. Functional heterogeneity of human memory CD4(+) T cell clones primed by pathogens or vaccines. Science 2015; 347(6220):400-6; PMID:25477212; http://dx.doi.org/10.1126/science.1260668
- Geiger R, Duhen T, Lanzavecchia A, Sallusto F. Human naive and memory CD4+ T cell repertoires specific for naturally processed antigens analyzed using libraries of amplified T cells. J Exp Med 2009; 206(7):1525-34; PMID:19564353; http://dx.doi.org/10.1084/jem.20090504
- Fraser CC, Altreuter DH, Ilyinskii P, Pittet L, LaMothe RA, Keegan M, Johnston L, Kishimoto TK. Generation of a universal CD4 memory T cell recall peptide effective in humans, mice and non-human primates. Vaccine 2014; 32(24):2896-903; PMID:24583006; http://dx.doi.org/10.1016/j.vaccine.2014.02.024
- Koff WC, Burton DR, Johnson PR, Walker BD, King CR, Nabel GJ, Ahmed R, Bhan MK, Plotkin SA. Accelerating next-generation vaccine development for global disease prevention. Science 2013; 340(6136):1232910; PMID:23723240; http://dx.doi.org/10.1126/science.1232910
- Georgiou G, Ippolito GC, Beausang J, Busse CE, Wardemann H, Quake SR. The promise and challenge of high-throughput sequencing of the antibody repertoire. Nat Biotechnol 2014; 32(2):158-68; PMID:24441474; http://dx.doi.org/10.1038/nbt.2782
- Tsang JS, Schwartzberg PL, Kotliarov Y, Biancotto A, Xie Z, Germain RN, Wang E, Olnes MJ, Narayanan M, Golding H, et al. Global analyses of human immune variation reveal baseline predictors of postvaccination responses. Cell 2014; 157(2):499-513; PMID:24725414; http://dx.doi.org/10.1016/j.cell.2014.03.031
- Janda KD, Treweek JB. Vaccines targeting drugs of abuse: is the glass half-empty or half-full? Nat Rev Immunol 2011; 12(1):67-72; PMID:22173478
- Kinsey B. Vaccines against drugs of abuse: where are we now? Ther Adv Vaccines 2014; 2(4):106-17; PMID:24982760; http://dx.doi.org/10.1177/2051013614537818
- Alving CR, Matyas GR, Torres O, Jalah R, Beck Z. Adjuvants for vaccines to drugs of abuse and addiction. Vaccine 2014; 32(42):5382-9; PMID:25111169; http://dx.doi.org/10.1016/j.vaccine.2014.07.085
- Kosten T, Domingo C, Orson F, Kinsey B. Vaccines against stimulants: cocaine and MA. Br J Clin Pharmacol 2014; 77(2):368-74; PMID:23509915; http://dx.doi.org/10.1111/bcp.12115
- Cai X, Whitfield T, Moreno AY, Grant Y, Hixon MS, Koob GF, Janda KD. Probing the effects of hapten stability on cocaine vaccine immunogenicity. Mol Pharm 2013; 10(11):4176-84; PMID:23927436; http://dx.doi.org/10.1021/mp400214w
- Lockner JW, Lively JM, Collins KC, Vendruscolo JC, Azar MR, Janda KD. A conjugate vaccine using enantiopure hapten imparts superior nicotine-binding capacity. J Med Chem 2015; 58(2):1005-11; PMID:25493909; http://dx.doi.org/10.1021/jm501625j
- Cai X, Tsuchikama K, Janda KD. Modulating cocaine vaccine potency through hapten fluorination. J Am Chem Soc 2013; 135(8):2971-4; PMID:23398531; http://dx.doi.org/10.1021/ja400356g
- Collins KC, Janda KD. Investigating hapten clustering as a strategy to enhance vaccines against drugs of abuse. Bioconjug Chem 2014; 25(3):593-600; PMID:24521489; http://dx.doi.org/10.1021/bc500016k
- Moreno AY, Mayorov AV, Janda KD. Impact of distinct chemical structures for the development of a methamphetamine vaccine. J Am Chem Soc 2011; 133(17):6587-95; PMID:21473576; http://dx.doi.org/10.1021/ja108807j
- Pravetoni M, Le Naour M, Harmon T, Tucker A, Portoghese PS, Pentel PR. An oxycodone conjugate vaccine elicits oxycodone-specific antibodies that reduce oxycodone distribution to brain and hot-plate analgesia. J Pharmacol Exp Ther 2012; 341(1):225-32; PMID:22262924; http://dx.doi.org/10.1124/jpet.111.189506
- Pravetoni M, Le Naour M, Tucker AM, Harmon TM, Hawley TM, Portoghese PS, Pentel PR. Reduced antinociception of opioids in rats and mice by vaccination with immunogens containing oxycodone and hydrocodone haptens. J Med Chem 2013; 56(3):915-23; PMID:23249238; http://dx.doi.org/10.1021/jm3013745
- Pravetoni M, Pentel PR, Potter DN, Chartoff EH, Tally L, LeSage MG. Effects of an oxycodone conjugate vaccine on oxycodone self-administration and oxycodone-induced brain gene expression in rats. PLoS One 2014; 9(7):e101807; PMID:25025380; http://dx.doi.org/10.1371/journal.pone.0101807
- Raleigh MD, Pentel PR, LeSage MG. Pharmacokinetic correlates of the effects of a heroin vaccine on heroin self-administration in rats. PLoS One 2014; 9(12):e115696; PMID:25536404; http://dx.doi.org/10.1371/journal.pone.0115696
- Raleigh MD, Pravetoni M, Harris AC, Birnbaum AK, Pentel PR. Selective effects of a morphine conjugate vaccine on heroin and metabolite distribution and heroin-induced behaviors in rats. J Pharmacol Exp Ther 2013; 344(2):397-406; PMID:23220743; http://dx.doi.org/10.1124/jpet.112.201194
- Anton B, Leff P. A novel bivalent morphine/heroin vaccine that prevents relapse to heroin addiction in rodents. Vaccine 2006; 24(16):3232-40; PMID:16494974; http://dx.doi.org/10.1016/j.vaccine.2006.01.047
- Li QQ, Luo YX, Sun CY, Xue YX, Zhu WL, Shi HS, Zhai HF, Shi J, Lu L. A morphine/heroin vaccine with new hapten design attenuates behavioral effects in rats. J Neurochem 2011; 119(6):1271-81; PMID:21951213; http://dx.doi.org/10.1111/j.1471-4159.2011.07502.x
- Kosten TA, Shen XY, O'Malley PW, Kinsey BM, Lykissa ED, Orson FM, Kosten TR. A morphine conjugate vaccine attenuates the behavioral effects of morphine in rats. Prog Neuropsychopharmacol Biol Psychiatry 2013; 45:223-9; PMID:23739535; http://dx.doi.org/10.1016/j.pnpbp.2013.05.012
- Li F, Cheng K, Antoline JF, Iyer MR, Matyas GR, Torres OB, Jalah R, Beck Z, Alving CR, Parrish DA, et al. Synthesis and immunological effects of heroin vaccines. Org Biomol Chem 2014; 12(37):7211-32; PMID:24995943; http://dx.doi.org/10.1039/C4OB01053A
- Schlosburg JE, Vendruscolo LF, Bremer PT, Lockner JW, Wade CL, Nunes AA, Stowe GN, Edwards S, Janda KD, Koob GF. Dynamic vaccine blocks relapse to compulsive intake of heroin. Proc Natl Acad Sci U S A 2013; 110(22):9036-41; PMID:23650354; http://dx.doi.org/10.1073/pnas.1219159110
- Stowe GN, Vendruscolo LF, Edwards S, Schlosburg JE, Misra KK, Schulteis G, Mayorov AV, Zakhari JS, Koob GF, Janda KD. A vaccine strategy that induces protective immunity against heroin. J Med Chem 2011; 54(14):5195-204; PMID:21692508; http://dx.doi.org/10.1021/jm200461m
- Carroll FI, Blough BE, Pidaparthi RR, Abraham P, Gong PK, Deng L, Huang X, Gunnell M, Lay JO, Jr, Peterson EC, et al. Synthesis of mercapto-(+)-methamphetamine haptens and their use for obtaining improved epitope density on (+)-methamphetamine conjugate vaccines. J Med Chem 2011; 54(14):5221-8; PMID:21682289; http://dx.doi.org/10.1021/jm2004943
- Pravetoni M, Keyler DE, Pidaparthi RR, Carroll FI, Runyon SP, Murtaugh MP, Earley CA, Pentel PR. Structurally distinct nicotine immunogens elicit antibodies with non-overlapping specificities. Biochem Pharmacol 2012; 83(4):543-50; PMID:22100986; http://dx.doi.org/10.1016/j.bcp.2011.11.004
- Pryde DC, Jones LH, Gervais DP, Stead DR, Blakemore DC, Selby MD, Brown AD, Coe JW, Badland M, Beal DM, et al. Selection of a novel anti-nicotine vaccine: influence of antigen design on antibody function in mice. PLoS One 2013; 8(10):e76557; PMID:24098532; http://dx.doi.org/10.1371/journal.pone.0076557
- Jalah R, Torres OB, Mayorov AV, Li F, Antoline JF, Jacobson AE, Rice KC, Deschamps JR, Beck Z, Alving CR, et al. Efficacy, but not antibody titer or affinity, of a heroin hapten conjugate vaccine correlates with increasing hapten densities on tetanus toxoid, but not on CRM197 carriers. Bioconjug Chem 2015; 26(6):1041-53; PMID:25970207; http://dx.doi.org/10.1021/acs.bioconjchem.5b00085
- McCluskie MJ, Thorn J, Mehelic PR, Kolhe P, Bhattacharya K, Finneman JI, Stead DR, Piatchek MB, Zhang N, Chikh G, et al. Molecular attributes of conjugate antigen influence function of antibodies induced by anti-nicotine vaccine in mice and non-human primates. Int Immunopharmacol 2015; 25(2):518-27; PMID:25737198; http://dx.doi.org/10.1016/j.intimp.2015.02.030
- Matyas GR, Mayorov AV, Rice KC, Jacobson AE, Cheng K, Iyer MR, Li F, Beck Z, Janda KD, Alving CR. Liposomes containing monophosphoryl lipid A: A potent adjuvant system for inducing antibodies to heroin hapten analogs. Vaccine 2013; 31(26):2804-10; PMID:23624097; http://dx.doi.org/10.1016/j.vaccine.2013.04.027
- Sanderson SD, Cheruku SR, Padmanilayam MP, Vennerstrom JL, Thiele GM, Palmatier MI, Bevins RA. Immunization to nicotine with a peptide-based vaccine composed of a conformationally biased agonist of C5a as a molecular adjuvant. Int Immunopharmacol 2003; 3(1):137-46; PMID:12538044; http://dx.doi.org/10.1016/S1567-5769(02)00260-6
- Duryee MJ, Bevins RA, Reichel CM, Murray JE, Dong Y, Thiele GM, Sanderson SD. Immune responses to methamphetamine by active immunization with peptide-based, molecular adjuvant-containing vaccines. Vaccine 2009; 27(22):2981-8; PMID:19428909; http://dx.doi.org/10.1016/j.vaccine.2009.02.105
- Pravetoni M, Raleigh MD, Le Naour M, Tucker AM, Harmon TM, Jones JM, Birnbaum AK, Portoghese PS, Pentel PR. Co-administration of morphine and oxycodone vaccines reduces the distribution of 6-monoacetylmorphine and oxycodone to brain in rats. Vaccine 2012; 30(31):4617-24; PMID:22583811; http://dx.doi.org/10.1016/j.vaccine.2012.04.101
- Haile CN, Kosten TA, Shen XY, O'Malley PW, Winoske KJ, Kinsey BM, Wu Y, Huang Z, Lykissa ED, Naidu N, et al. Altered methamphetamine place conditioning in mice vaccinated with a succinyl-methamphetamine-tetanus-toxoid vaccine. Am J Addict 2015; 24(8):748-55; PMID:26584468; http://dx.doi.org/10.1111/ajad.12307
- Miller ML, Aarde SM, Moreno AY, Creehan KM, Janda KD, Taffe MA. Effects of active anti-methamphetamine vaccination on intravenous self-administration in rats. Drug Alcohol Depend 2015; 153:29-36; PMID:26118833; http://dx.doi.org/10.1016/j.drugalcdep.2015.06.014
- Miller ML, Moreno AY, Aarde SM, Creehan KM, Vandewater SA, Vaillancourt BD, Wright MJ, Jr, Janda KD, Taffe MA. A methamphetamine vaccine attenuates methamphetamine-induced disruptions in thermoregulation and activity in rats. Biol Psychiatry 2013; 73(8):721-8; PMID:23098894; http://dx.doi.org/10.1016/j.biopsych.2012.09.010
- Ruedi-Bettschen D, Wood SL, Gunnell MG, West CM, Pidaparthi RR, Carroll FI, Blough BE, Owens SM. Vaccination protects rats from methamphetamine-induced impairment of behavioral responding for food. Vaccine 2013; 31(41):4596-602; PMID:23906885; http://dx.doi.org/10.1016/j.vaccine.2013.07.038
- Shen XY, Kosten TA, Lopez AY, Kinsey BM, Kosten TR, Orson FM. A vaccine against methamphetamine attenuates its behavioral effects in mice. Drug Alcohol Depend 2013; 129(1-2):41-8; PMID:23022610; http://dx.doi.org/10.1016/j.drugalcdep.2012.09.007
- Broker M, Costantino P, DeTora L, McIntosh ED, Rappuoli R. Biochemical and biological characteristics of cross-reacting material 197 CRM197, a non-toxic mutant of diphtheria toxin: use as a conjugation protein in vaccines and other potential clinical applications. Biologicals 2011; 39(4):195-204; PMID:21715186; http://dx.doi.org/10.1016/j.biologicals.2011.05.004
- Lockner JW, Eubanks LM, Choi JL, Lively JM, Schlosburg JE, Collins KC, Globisch D, Rosenfeld-Gunn RJ, Wilson IA, Janda KD. Flagellin as carrier and adjuvant in cocaine vaccine development. Mol Pharm 2015; 12(2):653-62; PMID:25531528; http://dx.doi.org/10.1021/mp500520r
- Miller KD, Roque R, Clegg CH. Novel anti-nicotine vaccine using a trimeric coiled-coil hapten carrier. PLoS One 2014; 9(12):e114366; PMID:25494044; http://dx.doi.org/10.1371/journal.pone.0114366
- Maoz A, Hicks MJ, Vallabhjosula S, Synan M, Kothari PJ, Dyke JP, Ballon DJ, Kaminsky SM, De BP, Rosenberg JB, et al. Adenovirus capsid-based anti-cocaine vaccine prevents cocaine from binding to the nonhuman primate CNS dopamine transporter. Neuropsychopharmacol 2013; 38(11):2170-8; http://dx.doi.org/10.1038/npp.2013.114
- Rosenberg J, De B, Hicks M, Janda K, Kaminsky S, Worgall S, Crystal R. Suppression of nicotine-induced pathophysiology by an adenovirus hexon-based anti-nicotine vaccine. Hum Gene Ther 2013; 24(6):595-603; PMID:23611296; http://dx.doi.org/10.1089/hum.2012.245
- Hicks MJ, Kaminsky SM, De BP, Rosenberg JB, Evans SM, Foltin RW, Andrenyak DM, Moody DE, Koob GF, Janda KD, et al. Fate of systemically administered cocaine in nonhuman primates treated with the dAd5GNE anticocaine vaccine. Hum Gene Ther Clin Dev 2014; 25(1):40-9; PMID:24649839; http://dx.doi.org/10.1089/humc.2013.231
- Koob G, Hicks MJ, Wee S, Rosenberg JB, De BP, Kaminsky SM, Moreno A, Janda KD, Crystal RG. Anti-cocaine vaccine based on coupling a cocaine analog to a disrupted adenovirus. CNS Neurol Disord Drug Targets 2011; 10(8):899-904; PMID:22229312; http://dx.doi.org/10.2174/187152711799219334
- O'Hagan DT, Fox CB. New generation adjuvants–from empiricism to rational design. Vaccine 2015; 33(Suppl 2):B14-20; http://dx.doi.org/10.1016/j.vaccine.2015.01.088
- Mastelic Gavillet B, Eberhardt CS, Auderset F, Castellino F, Seubert A, Tregoning JS, Lambert PH, de Gregorio E, Del Giudice G, Siegrist CA. MF59 mediates its B cell adjuvanticity by promoting T Follicular helper cells and thus germinal center responses in adult and early life. J Immunol 2015; 194(10):4836-45; PMID:25870238; http://dx.doi.org/10.4049/jimmunol.1402071
- McCluskie MJ, Thorn J, Gervais DP, Stead DR, Zhang N, Benoit M, Cartier J, Kim IJ, Bhattacharya K, Finneman JI, et al. Anti-nicotine vaccines: Comparison of adjuvanted CRM197 and Qb-VLP conjugate formulations for immunogenicity and function in non-human primates. Int Immunopharmacol 2015; 29(2):663-71; PMID:26404190; http://dx.doi.org/10.1016/j.intimp.2015.09.012
- Bremer PT, Kimishima A, Schlosburg JE, Zhou B, Collins KC, Janda KD. Combatting synthetic designer opioids: A conjugate vaccine ablates lethal doses of fentanyl class drugs. Angew Chem Int Ed Engl 2016; 55(11):3772-5; PMID:26879590; http://dx.doi.org/10.1002/anie.201511654
- Chen X, Kim P, Farinelli B, Doukas A, Yun SH, Gelfand JA, Anderson RR, Wu MX. A novel laser vaccine adjuvant increases the motility of antigen presenting cells. PLoS One 2010; 5(10):e13776; PMID:21048884; http://dx.doi.org/10.1371/journal.pone.0013776
- Chen X, Pravetoni M, Bhayana B, Pentel PR, Wu MX. High immunogenicity of nicotine vaccines obtained by intradermal delivery with safe adjuvants. Vaccine 2012; 31(1):159-64; PMID:23123021; http://dx.doi.org/10.1016/j.vaccine.2012.10.069
- Irvine DJ, Hanson MC, Rakhra K, Tokatlian T. Synthetic nanoparticles for vaccines and immunotherapy. Chem Rev 2015; 115(19):11109-46; PMID:26154342; http://dx.doi.org/10.1021/acs.chemrev.5b00109
- Moon JJ, Huang B, Irvine DJ. Engineering nano- and microparticles to tune immunity. Adv Mater 2012; 24(28):3724-46; PMID:22641380; http://dx.doi.org/10.1002/adma.201200446
- Jain S, O'Hagan DT, Singh M. The long-term potential of biodegradable poly(lactide-co-glycolide) microparticles as the next-generation vaccine adjuvant. Expert Rev Vaccines 2011; 10(12):1731-42; PMID:22085176; http://dx.doi.org/10.1586/erv.11.126
- Braun M, Jandus C, Maurer P, Hammann-Haenni A, Schwarz K, Bachmann MF, Speiser DE, Romero P. Virus-like particles induce robust human T-helper cell responses. Eur J Immunol 2012; 42(2):330-40; PMID:22057679; http://dx.doi.org/10.1002/eji.201142064
- Zabel F, Mohanan D, Bessa J, Link A, Fettelschoss A, Saudan P, Kundig TM, Bachmann MF. Viral particles drive rapid differentiation of memory B cells into secondary plasma cells producing increased levels of antibodies. J Immunol 2014; 192(12):5499-508; PMID:24821969; http://dx.doi.org/10.4049/jimmunol.1400065
- Agnandji ST, Lell B, Soulanoudjingar SS, Fernandes JF, Abossolo BP, Conzelmann C, Methogo BG, Doucka Y, Flamen A, Mordmuller B, et al. First results of phase 3 trial of RTS,S/AS01 malaria vaccine in African children. N Engl J Med 2011; 365(20):1863-75; PMID:22007715; http://dx.doi.org/10.1056/NEJMoa1102287
- RTS,S Clinical Trials Partnership, Agnandji ST, Lell B, Fernandes JF, Abossolo BP, Methogo BG, Kabwende AL, Adegnika AA, Mordmuller B, Issifou S, et al. A phase 3 trial of RTS,S/AS01 malaria vaccine in African infants. N Engl J Med 2012; 367(24):2284-95; PMID:23136909; http://dx.doi.org/10.1056/NEJMoa1208394
- Collins KC, Schlosburg JE, Lockner JW, Bremer PT, Ellis BA, Janda KD. Lipid tucaresol as an adjuvant for methamphetamine vaccine development. Chem Commun (Camb) 2014; 50(31):4079-81; PMID:24615284; http://dx.doi.org/10.1039/c4cc00682h
- Lockner JW, Ho SO, McCague KC, Chiang SM, Do TQ, Fujii G, Janda KD. Enhancing nicotine vaccine immunogenicity with liposomes. Bioorg Med Chem Lett 2013; 23(4):975-8; PMID:23313243; http://dx.doi.org/10.1016/j.bmcl.2012.12.048
- Hu Y, Zheng H, Huang W, Zhang C. A novel and efficient nicotine vaccine using nano-lipoplex as a delivery vehicle. Hum Vaccin Immunother 2014; 10(1):64-72; http://dx.doi.org/10.4161/hv.26635
- Desai RI, Bergman J. Effects of the nanoparticle-based vaccine, SEL-068, on nicotine discrimination in squirrel monkeys. Neuropsychopharmacol 2015; 40(9):2207-16; http://dx.doi.org/10.1038/npp.2015.64
- Desai RI, Bergman J. Nicotine-targeting nano-vaccines for smoking cessation. Neuropsychopharmacol 2016; 41(1):377-8; http://dx.doi.org/10.1038/npp.2015.234
- Ilyinskii PO, Roy CJ, O'Neil CP, Browning EA, Pittet LA, Altreuter DH, Alexis F, Tonti E, Shi J, Basto PA, et al. Adjuvant-carrying synthetic vaccine particles augment the immune response to encapsulated antigen and exhibit strong local immune activation without inducing systemic cytokine release. Vaccine 2014; 32(24):2882-95; PMID:24593999; http://dx.doi.org/10.1016/j.vaccine.2014.02.027
- Liu X, Xu Y, Yu T, Clifford C, Liu Y, Yan H, Chang Y. A DNA nanostructure platform for directed assembly of synthetic vaccines. Nano Lett 2012; 12(8):4254-9; PMID:22746330; http://dx.doi.org/10.1021/nl301877k
- Rudra JS, Mishra S, Chong AS, Mitchell RA, Nardin EH, Nussenzweig V, Collier JH. Self-assembled peptide nanofibers raising durable antibody responses against a malaria epitope. Biomaterials 2012; 33(27):6476-84; PMID:22695068; http://dx.doi.org/10.1016/j.biomaterials.2012.05.041
- Rudra JS, Ding Y, Neelakantan H, Ding C, Appavu R, Stutz S, Snook JD, Chen H, Cunningham KA, Zhou J. Suppression of Cocaine-Evoked Hyperactivity by Self-Adjuvanting and Multivalent Peptide Nanofiber Vaccines. ACS Chem Neurosci 2016; 7(5):546-52; PMID:26926328; http://dx.doi.org/10.1021/acschemneuro.5b00345
- Stavropoulos P, Papavasiliou FN. Using T. brucei as a biological epitope-display platform to elicit specific antibody responses. J Immunol Methods 2010; 362(1-2):190-4; PMID:20800596; http://dx.doi.org/10.1016/j.jim.2010.08.009
- Astronomo RD, Burton DR. Carbohydrate vaccines: developing sweet solutions to sticky situations? Nat Rev Drug Discov 2010; 9(4):308-24; PMID:20357803; http://dx.doi.org/10.1038/nrd3012
- Bachmann MF, Jennings GT. Vaccine delivery: a matter of size, geometry, kinetics and molecular patterns. Nat Rev Immunol 2010; 10(11):787-96; PMID:20948547; http://dx.doi.org/10.1038/nri2868
- Irvine DJ, Swartz MA, Szeto GL. Engineering synthetic vaccines using cues from natural immunity. Nat Mater 2013; 12(11):978-90; PMID:24150416; http://dx.doi.org/10.1038/nmat3775
- Hanson MC, Crespo MP, Abraham W, Moynihan KD, Szeto GL, Chen SH, Melo MB, Mueller S, Irvine DJ. Nanoparticulate STING agonists are potent lymph node-targeted vaccine adjuvants. J Clin Invest 2015; 125(6):2532-46; PMID:25938786; http://dx.doi.org/10.1172/JCI79915
- Liu H, Moynihan KD, Zheng Y, Szeto GL, Li AV, Huang B, Van Egeren DS, Park C, Irvine DJ. Structure-based programming of lymph-node targeting in molecular vaccines. Nature 2014; 507(7493):519-22; PMID:24531764; http://dx.doi.org/10.1038/nature12978
- de Villiers SH, Cornish KE, Troska AJ, Pravetoni M, Pentel PR. Increased efficacy of a trivalent nicotine vaccine compared to a dose-matched monovalent vaccine when formulated with alum. Vaccine 2013; 31(52):6185-93; PMID:24176492; http://dx.doi.org/10.1016/j.vaccine.2013.10.051
- Ohia-Nwoko O, Kosten TA, Haile CN. Animal models and the development of vaccines to treat substance use disorders. Int Rev Neurobiol 2016; 126:263-91; PMID:27055616; http://dx.doi.org/10.1016/bs.irn.2016.02.009
- Rappuoli R, Pizza M, Del Giudice G, De Gregorio E. Vaccines, new opportunities for a new society. Proc Natl Acad Sci U S A 2014; 111(34):12288-93; PMID:25136130; http://dx.doi.org/10.1073/pnas.1402981111
- Carrera MR, Ashley JA, Hoffman TZ, Isomura S, Wirsching P, Koob GF, Janda KD. Investigations using immunization to attenuate the psychoactive effects of nicotine. Bioorg Med Chem 2004; 12(3):563-70; PMID:14738965; http://dx.doi.org/10.1016/j.bmc.2003.11.029
- Keyler DE, Roiko SA, Benlhabib E, LeSage MG, St Peter JV, Stewart S, Fuller S, Le CT, Pentel PR. Monoclonal nicotine-specific antibodies reduce nicotine distribution to brain in rats: dose- and affinity-response relationships. Drug Metab Dispos 2005; 33(7):1056-61; PMID:15843487; http://dx.doi.org/10.1124/dmd.105.004234
- Fox BS, Kantak KM, Edwards MA, Black KM, Bollinger BK, Botka AJ, French TL, Thompson TL, Schad VC, Greenstein JL, et al. Efficacy of a therapeutic cocaine vaccine in rodent models. Nat Med 1996; 2(10):1129-32; PMID:8837612; http://dx.doi.org/10.1038/nm1096-1129
- Carrera MR, Ashley JA, Zhou B, Wirsching P, Koob GF, Janda KD. Cocaine vaccines: antibody protection against relapse in a rat model. Proc Natl Acad Sci U S A 2000; 97(11):6202-6; PMID:10823960; http://dx.doi.org/10.1073/pnas.97.11.6202
- Norman AB, Norman MK, Buesing WR, Tabet MR, Tsibulsky VL, Ball WJ. The effect of a chimeric human/murine anti-cocaine monoclonal antibody on cocaine self-administration in rats. J Pharmacol Exp Ther 2009; 328(3):873-81; PMID:19088302; http://dx.doi.org/10.1124/jpet.108.146407
- Hardin JS, Wessinger WD, Wenger GR, Proksch JW, Laurenzana EM, Owens SM. A single dose of monoclonal anti-phencyclidine IgG offers long-term reductions in phencyclidine behavioral effects in rats. J Pharmacol Exp Ther 2002; 302(1):119-26; PMID:12065708; http://dx.doi.org/10.1124/jpet.302.1.119
- Gentry WB, Laurenzana EM, Williams DK, West JR, Berg RJ, Terlea T, Owens SM. Safety and efficiency of an anti-(+)-methamphetamine monoclonal antibody in the protection against cardiovascular and central nervous system effects of (+)-methamphetamine in rats. Int Immunopharmacol 2006; 6(6):968-77; PMID:16644483; http://dx.doi.org/10.1016/j.intimp.2006.01.008
- Bogen IL, Boix F, Nerem E, Morland J, Andersen JM. A monoclonal antibody specific for 6-monoacetylmorphine reduces acute heroin effects in mice. J Pharmacol Exp Ther 2014; 349(3):568-76; PMID:24700886; http://dx.doi.org/10.1124/jpet.113.212035
- Kvello AM, Andersen JM, Oiestad EL, Morland J, Bogen IL. The pharmacological effects of a monoclonal antibody against 6-monoacetylmorphine upon heroin induced locomotor activity and pharmacokinetics in mice. J Pharmacol Exp Ther 2016; 358(2):181-9; PMID:27217591; http://dx.doi.org/10.1124/jpet.116.233510
- Stevens MW, Henry RL, Owens SM, Schutz R, Gentry WB. First human study of a chimeric anti-methamphetamine monoclonal antibody in healthy volunteers. MAbs 2014; 6(6):1649-56; PMID:25484042; http://dx.doi.org/10.4161/19420862.2014.976431
- Kirley TL, Norman AB. Characterization of a recombinant humanized anti-cocaine monoclonal antibody and its fab fragment. Hum Vaccin Immunother 2015; 11(2):458-67; PMID:25692880; http://dx.doi.org/10.4161/21645515.2014.990856
- Cohen-Barak O, Wildeman J, van de Wetering J, Hettinga J, Schuilenga-Hut P, Gross A, Clark S, Bassan M, Gilgun-Sherki Y, Mendzelevski B, et al. Safety, pharmacokinetics, and pharmacodynamics of TV-1380, a novel mutated butyrylcholinesterase treatment for cocaine addiction, after single and multiple intramuscular injections in healthy subjects. J Clin Pharmacol 2015; 55(5):573-83; PMID:25524052; http://dx.doi.org/10.1002/jcph.450
- Nasser AF, Fudala PJ, Zheng B, Liu Y, Heidbreder C. A randomized, double-blind, placebo-controlled trial of RBP-8000 in cocaine abusers: pharmacokinetic profile of rbp-8000 and cocaine and effects of RBP-8000 on cocaine-induced physiological effects. J Addict Dis 2014; 33(4):289-302; PMID:25299069; http://dx.doi.org/10.1080/10550887.2014.969603
- Shram MJ, Cohen-Barak O, Chakraborty B, Bassan M, Schoedel KA, Hallak H, Eyal E, Weiss S, Gilgun Y, Sellers EM, et al. Assessment of Pharmacokinetic and Pharmacodynamic Interactions between Albumin-Fused Mutated Butyrylcholinesterase and Intravenously Administered Cocaine in Recreational Cocaine Users. J Clin Psychopharmacol 2015; 35(4):396-405; PMID:26082975
- Nanaware-Kharade N, Thakkar S, Gonzalez GA, 3rd, Peterson EC. A Nanotechnology-Based platform for extending the pharmacokinetic and binding properties of anti-methamphetamine antibody fragments. Sci Rep 2015; 5:12060; PMID:26159352; http://dx.doi.org/10.1038/srep12060
- Wilson PC, Andrews SF. Tools to therapeutically harness the human antibody response. Nat Rev Immunol 2012; 12(10):709-19; PMID:23007571; http://dx.doi.org/10.1038/nri3285
- Wrammert J, Smith K, Miller J, Langley WA, Kokko K, Larsen C, Zheng NY, Mays I, Garman L, Helms C, et al. Rapid cloning of high-affinity human monoclonal antibodies against influenza virus. Nature 2008; 453(7195):667-71; PMID:18449194; http://dx.doi.org/10.1038/nature06890
- Corti D, Lanzavecchia A. Efficient methods to isolate human monoclonal antibodies from memory B cells and plasma cells. Microbiol Spectr 2014; 2(5); PMID:26104354; http://dx.doi.org/10.1128/microbiolspec.AID-0018-2014
- Beerli RR, Bauer M, Buser RB, Gwerder M, Muntwiler S, Maurer P, Saudan P, Bachmann MF. Isolation of human monoclonal antibodies by mammalian cell display. Proc Natl Acad Sci U S A 2008; 105(38):14336-41; PMID:18812621; http://dx.doi.org/10.1073/pnas.0805942105
- LeSage MG, Shelley D, Pravetoni M, Pentel PR. Enhanced attenuation of nicotine discrimination in rats by combining nicotine-specific antibodies with a nicotinic receptor antagonist. Pharmacol Biochem Behav 2012; 102(1):157-62; PMID:22503967; http://dx.doi.org/10.1016/j.pbb.2012.03.026
- Cornish KE, Harris AC, LeSage MG, Keyler DE, Burroughs D, Earley C, Pentel PR. Combined active and passive immunization against nicotine: minimizing monoclonal antibody requirements using a target antibody concentration strategy. Int Immunopharmacol 2011; 11(11):1809-15; PMID:21802529; http://dx.doi.org/10.1016/j.intimp.2011.07.009
- Hambuchen MD, Carroll FI, Ruedi-Bettschen D, Hendrickson HP, Hennings LJ, Blough BE, Brieaddy LE, Pidaparthi RR, Owens SM. Combining active immunization with monoclonal antibody therapy to facilitate early initiation of a long-acting anti-methamphetamine antibody response. J Med Chem 2015; 58(11):4665-77; PMID:25973614; http://dx.doi.org/10.1021/acs.jmedchem.5b00220
- Larrimore KE, Barcus M, Kannan L, Gao Y, Zhan CG, Brimijoin S, Mor T. Plants as a source of butyrylcholinesterase variants designed for enhanced cocaine hydrolase activity. Chem Biol Interact 2013; 203(1):217-20; PMID:23000451; http://dx.doi.org/10.1016/j.cbi.2012.09.004
- Zheng F, Xue L, Hou S, Liu J, Zhan M, Yang W, Zhan CG. A highly efficient cocaine-detoxifying enzyme obtained by computational design. Nat Commun. 2014; 5:3457; PMID:24643289
- Fang L, Hou S, Xue L, Zheng F, Zhan CG. Amino-acid mutations to extend the biological half-life of a therapeutically valuable mutant of human butyrylcholinesterase. Chem Biol Interact 2014; 214:18-25; PMID:24582612; http://dx.doi.org/10.1016/j.cbi.2014.02.007
- Collins GT, Narasimhan D, Cunningham AR, Zaks ME, Nichols J, Ko MC, Sunahara RK, Woods JH. Long-lasting effects of a PEGylated mutant cocaine esterase (CocE) on the reinforcing and discriminative stimulus effects of cocaine in rats. Neuropsychopharmacol 2012; 37(5):1092-103; http://dx.doi.org/10.1038/npp.2011.226
- Brimijoin S, Orson F, Kosten TR, Kinsey B, Shen XY, White SJ, Gao Y. Anti-cocaine antibody and butyrylcholinesterase-derived cocaine hydrolase exert cooperative effects on cocaine pharmacokinetics and cocaine-induced locomotor activity in mice. Chem Biol Interact 2013; 203(1):212-6; PMID:22960160; http://dx.doi.org/10.1016/j.cbi.2012.08.015
- Xue S, Schlosburg JE, Janda KD. A new strategy for smoking cessation: characterization of a bacterial enzyme for the degradation of nicotine. J Am Chem Soc 2015; 137(32):10136-9; PMID:26237398; http://dx.doi.org/10.1021/jacs.5b06605
- Landry DW, Zhao K, Yang GX, Glickman M, Georgiadis TM. Antibody-catalyzed degradation of cocaine. Science 1993; 259(5103):1899-901; PMID:8456315; http://dx.doi.org/10.1126/science.8456315
- Xu Y, Hixon MS, Yamamoto N, McAllister LA, Wentworth AD, Wentworth P, Jr, Janda KD. Antibody-catalyzed anaerobic destruction of methamphetamine. Proc Natl Acad Sci U S A 2007; 104(10):3681-6; PMID:17360412; http://dx.doi.org/10.1073/pnas.0611094104
- Dickerson TJ, Yamamoto N, Janda KD. Antibody-catalyzed oxidative degradation of nicotine using riboflavin. Bioorg Med Chem 2004; 12(18):4981-7; PMID:15336277; http://dx.doi.org/10.1016/j.bmc.2004.07.006
- Chen X, Xue L, Hou S, Jin Z, Zhang T, Zheng F, Zhan CG. Long-acting cocaine hydrolase for addiction therapy. Proc Natl Acad Sci U S A 2016; 113(2):422-7; PMID:26712009; http://dx.doi.org/10.1073/pnas.1517713113
- Hicks MJ, Rosenberg JB, De BP, Pagovich OE, Young CN, Qiu JP, Kaminsky SM, Hackett NR, Worgall S, Janda KD, et al. AAV-directed persistent expression of a gene encoding anti-nicotine antibody for smoking cessation. Sci Transl Med 2012; 4(140):140ra187; http://dx.doi.org/10.1126/scitranslmed.3003611
- Rosenberg JB, Hicks MJ, De BP, Pagovich O, Frenk E, Janda KD, Wee S, Koob GF, Hackett NR, Kaminsky SM, et al. AAVrh.10-mediated expression of an anti-cocaine antibody mediates persistent passive immunization that suppresses cocaine-induced behavior. Hum Gene Ther 2012; 23(5):451-9; PMID:22486244; http://dx.doi.org/10.1089/hum.2011.178
- Carroll ME, Zlebnik NE, Anker JJ, Kosten TR, Orson FM, Shen X, Kinsey B, Parks RJ, Gao Y, Brimijoin S. Combined cocaine hydrolase gene transfer and anti-cocaine vaccine synergistically block cocaine-induced locomotion. PLoS One 2012; 7(8):e43536; PMID:22912888; http://dx.doi.org/10.1371/journal.pone.0043536
- Smethells JR, Swalve N, Brimijoin S, Gao Y, Parks RJ, Greer A, Carroll ME. Long-Term blockade of cocaine self-administration and locomotor activation in rats by an adenoviral vector-delivered cocaine hydrolase. J Pharmacol Exp Ther 2016; 357(2):375-81; PMID:26968195; http://dx.doi.org/10.1124/jpet.116.232504
- Murthy V, Gao Y, Geng L, LeBrasseur NK, White TA, Parks RJ, Brimijoin S. Physiologic and metabolic safety of butyrylcholinesterase gene therapy in mice. Vaccine 2014; 32(33):4155-62; PMID:24892251; http://dx.doi.org/10.1016/j.vaccine.2014.05.067
- Murthy V, Gao Y, Geng L, LeBrasseur N, White T, Brimijoin S. Preclinical studies on neurobehavioral and neuromuscular effects of cocaine hydrolase gene therapy in mice. J Mol Neurosci 2014; 53(3):409-16; PMID:24085526; http://dx.doi.org/10.1007/s12031-013-0130-5
- Murthy V, Reyes S, Geng L, Gao Y, Brimijoin S. Cocaine Hydrolase Gene Transfer Demonstrates Cardiac Safety and Efficacy against Cocaine-Induced QT Prolongation in Mice. J Pharmacol Exp Ther 2016; 356(3):720-5; PMID:26669428; http://dx.doi.org/10.1124/jpet.115.228825
- Hatsukami DK, Rennard S, Jorenby D, Fiore M, Koopmeiners J, de Vos A, Horwith G, Pentel PR. Safety and immunogenicity of a nicotine conjugate vaccine in current smokers. Clin Pharmacol Ther 2005; 78(5):456-67; PMID:16321612; http://dx.doi.org/10.1016/j.clpt.2005.08.007
- Hoogsteder PH, Kotz D, van Spiegel PI, Viechtbauer W, van Schayck OC. Efficacy of the nicotine vaccine 3'-AmNic-rEPA (NicVAX) co-administered with varenicline and counselling for smoking cessation: a randomized placebo-controlled trial. Addiction 2014; 109(8):1252-9; PMID:24894625; http://dx.doi.org/10.1111/add.12573
- Hoogsteder PH, Kotz D, van Spiegel PI, Viechtbauer W, Brauer R, Kessler PD, Kalnik MW, Fahim RE, van Schayck OC. The efficacy and safety of a nicotine conjugate vaccine (NicVAX(R)) or placebo co-administered with varenicline (Champix(R)) for smoking cessation: study protocol of a phase IIb, double blind, randomized, placebo controlled trial. BMC Public Health 2012; 12:1052; PMID:23216646; http://dx.doi.org/10.1186/1471-2458-12-1052
- Esterlis I, Hannestad JO, Perkins E, Bois F, D'Souza DC, Tyndale RF, Seibyl JP, Hatsukami DM, Cosgrove KP, O'Malley SS. Effect of a nicotine vaccine on nicotine binding to beta2*-nicotinic acetylcholine receptors in vivo in human tobacco smokers. Am J Psychiatry 2013; 170(4):399-407; PMID:23429725; http://dx.doi.org/10.1176/appi.ajp.2012.12060793
- Tonstad S, Heggen E, Giljam H, Lagerback PA, Tonnesen P, Wikingsson LD, Lindblom N, de Villiers S, Svensson TH, Fagerstrom KO. Niccine(R), a nicotine vaccine, for relapse prevention: A Phase II, randomized, placebo-controlled, multicenter clinical trial. Nicotine Tob Res 2013; 15(9):1492-501; PMID:23471101; http://dx.doi.org/10.1093/ntr/ntt003
- Kosten TR, Rosen M, Bond J, Settles M, Roberts JS, Shields J, Jack L, Fox B. Human therapeutic cocaine vaccine: safety and immunogenicity. Vaccine 2002; 20(7-8):1196-204; PMID:11803082; http://dx.doi.org/10.1016/S0264-410X(01)00425-X
- Haney M, Gunderson EW, Jiang H, Collins ED, Foltin RW. Cocaine-specific antibodies blunt the subjective effects of smoked cocaine in humans. Biol Psychiatry 2010; 67(1):59-65; PMID:19846066; http://dx.doi.org/10.1016/j.biopsych.2009.08.031