ABSTRACT
Immune checkpoint inhibitors (ICI) based cancer immunotherapy has recently attracted considerable interest in the field of cancer therapy. The relevant immunotherapeutic agents do not directly attack the tumor, but boost the body's immune system to recognize and kill cancer cells. In this commentary, recent efforts utilizing immunoengineering for local delivery of these immune checkpoint antibodies are introduced. Future opportunities and challenges in this research theme are also commented.
As a paradigm shift in cancer treatment, cancer immunotherapy has received tremendous attentions. More than half of the current cancer clinical trials include formats of immunotherapy. Instead of directly attacking the tumor, immunotherapeutic agents boost the body's immune system to kill the cancer cells.Citation1,2 Particularly, the immune checkpoint blockade has elicited durable antitumor responses and long-term remissions in a subset of patients.Citation1-3 Immunologic effector T cells may be blocked by inhibitory regulatory molecules, which serve as checkpoints to control or “turn off” the immune response.Citation4 Cancer cells utilize these mechanisms to evade elimination by immune system.Citation5 The immune regulatory checkpoint inhibitors agents, including anti-CTLA-4 and anti-PD-1/PD-L1, are antibodies targeting cytotoxic T-lymphocyte antigen 4 (CTLA4), programmed cell death 1 (PD-1), and programmed cell death ligand 1 (PD-L1), respectively. They can overcome this main defenses strategy of cancer cells by blocking the normal protein ligands on cancer cells or on T cells that respond against the immune system attack.Citation6 So far, 4 checkpoint inhibitors have received rapid approval from the US Food and Drug Administration (FDA) for cancer, including ipilimumab (Yervoy®), pembrolizumab (Keytruda®), nivolumab (Opdivo®), and atezolizumab (Tecentriq®).Citation7-11
Despite remarkable progress, current methods of checkpoint blockade therapy limit therapeutic benefit in many patients.Citation2 The largest limitation observed in clinical trials maybe the severity of the adverse effects, grade 3/4 adverse events have often occurred.Citation12-15 Since the blockade of these regulatory pathways is not antigen specific, the off-target effect from these drugs can be significant. In this context, it could be desirable for specifically targeting the tumor site, rather than systemic activation of the immune system.Citation3,16 Meanwhile, a large fraction of patients failed to respond to these agents.Citation2,7-9,17 How to enhance immune checkpoints inhibitors based cancer therapy with limited side effects has become one of the central themes in the field of cancer immunology and immunotherapy.Citation6
In light of this, we have recently leveraged design of biomaterials to deliver checkpoint inhibitor antibodies to the tumor microenvironment (TME) for the improvement of treatment efficacy. For example, a biodegradable microneedle was developed for controlled delivery of anti-PD-1 (aPD1) toward melanoma ().Citation18 Microneedle (MN) patches have been widely used in transdermal drug and vaccine delivery in preclinical studies,Citation19,20 such as insulin,Citation21,22 influenza,Citation23 human papillomavirus (HPV)Citation24 during the last decade. In this study, the microneedle patch was composed of biocompatible hyaluronic acid (HA), which has been widely applied in numerous FDA approved therapeutic formulations or medical devices due to its excellent biocompatibility and biodegradability. HA was integrated with pH-sensitive dextran nanoparticles that encapsulate aPD1. The self-dissociated nanoparticles distributed inside the MN tips resulted in the sustained release of aPD1 over a 3-day period of time in vitro. In the animal study, we demonstrated that one microneedle patch enhanced the retention of aPD1 in the tumor microenvironment and induced robust immune responses against B16F10 mouse melanoma in comparison of aPD1 in the free form. These effects are associated with increased effector T cell infiltration in the TME. Additionally, the MN patch can be served as a platform for combination delivery of other immune checkpoint inhibitors, such as anti-CTLA4 (aCTLA4). A remarkable synergistic effect was achieved by combination therapeutics of aCTLA4 and aPD1 co-delivered via MNs. 70% long-term disease-free survival of mice was achieved after being treated with a combinational MN patch of aCTLA4 and aPD1 in 60 d. Collectively, our strategy based on the biodegradable microneedle for local treatment of melanoma improves the therapeutic efficacy of immune checkpoint inhibitors and can reduce the systemic toxicity simultaneously. This “immune patch” could offer an alternative option for patients with an early stage of melanoma. The patch can prevent primary tumor growth and metastasis before the surgery, if not destroying the primary tumor.
Figure 1. Schematic of the MN patch-assisted delivery of aPD1 for the skin cancer treatment. (a) Schematic of the aPD1 delivered by an MN patch loaded with physiologically self-dissociated NPs. With GOx/CAT enzymatic system immobilized inside the NPs by double-emulsion method, the enzyme-mediated conversion of blood glucose to gluconic acid promotes the sustained dissociation of NPs, subsequently leading to the release of aPD1. (b) The blockade of PD-1 by aPD1 to activate the immune system to destroy skin cancer cells. © 2016 American Chemical Society.Citation18 Reproduced by permission of American Chemical Society. Permission to reuse must be obtained from the rightsholder.
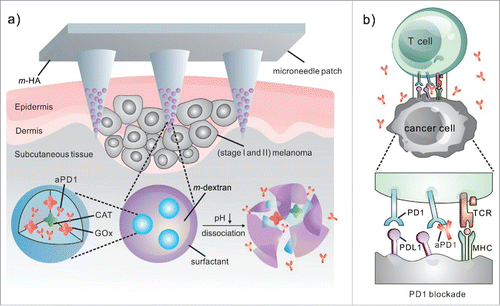
Checkpoint inhibition combined with other immunomodulators has demonstrated a synergistic increase of antitumor activity.Citation25 In another work, we described a new delivery carrier for the controlled release of loaded anti-PD1 antibody and CpG oligodeoxynucleotides (CpG ODNs) in response to inflammation conditions for post-surgery treatment ().Citation26 The delivery carrier was “weaved” through the rolling circle amplificationCitation27-29 using a CpG ODN-encoded template. DNCs (DNA nano-cocoons) were loaded with aPD1 and inflammation-responsive triglycerol monostearate (TGMS) nanoparticles caging a restriction enzyme inside, which can specifically cleave DNCs into short pieces of CpG ODNs. After injection into the tumor resection sites, TGMS nanoparticles were disassembled upon the digestion of inflammatory-associated proteases to release the caged enzyme, allowing fragmentation of DNCs into CpG ODN and releasing aPD-1. These DNCs provided sustained release of immunomodulators that can evoke T cell activity, break tumor tolerance and elicit durable, tumor-specific immunity after removal of the solid tumor. 40% of mice showed complete responses with nondetectable recurrent tumor to our combination therapy. The CpG DNA-based carrier not only serves as a therapeutic loading matrix for aPD1, but also can enhance therapy efficacy by enzymatic fragmentation into another drug-CpG pieces to active DCs.
Figure 2. Schematic illustration of delivery of CpG and anti-PD1 antibody (aPD1) by DNA nano-cocoon under an inflammation condition. (a) CpG-sequence containing DNA nano-cocoon (DNC) loaded with anti-PD1 antibody and caged restriction enzyme for inflammation-trigged fragmentation of DNC for releasing both CpG DNA and aPD1. (b) A schematic representation of in vivo tumor immunotherapy after primary tumor resection, local injection and treatment of DNC-based delivery system. (c) Released CpG DNA activates dendritic cells (DCs) to drive T cell response with aPD1 for PD 1 blockade. © 2016 Wiley-VCH. Citation26 Reproduced by permission of Wiley-VCH. Permission to reuse must be obtained from the rightsholder.
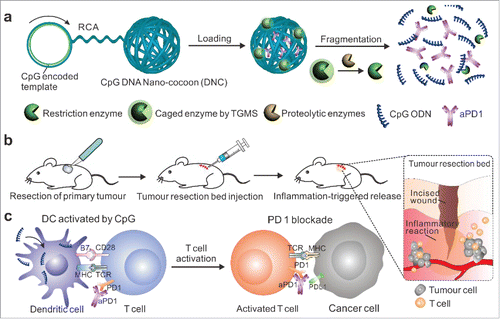
Outlook
Despite the promise of using local delivery of checkpoints to treat cancer, there are still several challenges. First of all, MN can only deliver checkpoint antibodies to superficial tumors microenviroment such as melanoma. How to develop delivery technologies for targeting tumor microenvironment inside the body and avoiding overstimulating tumor-irrelevant T cells thus limit autoimmunity-related side effects are vast opportunities for biomedical engineers. Second, a large fraction of patients failed to respond to checkpoints agents. For atezolizumab, the entire population had an objective response rate (ORR) of only 14.8%. Even for the PDL1 positive subgroup, ORR was increased to 26%.Citation11 Tumor lymphocytic infiltration is a prerequisite for response. Creating “T cell-inflamed” TME, generating a “PD-L1-positive tumor,” and boosting T cells activity as well as other immune cells when delivering the checkpoint antibodies would further enhance the anti-PD1/PDL1 immunotherapy and promote the objective response rate. Moreover, checkpoint blockade therapies combined with other anticancer therapeutics and/or treatment modalities will be a promising strategy to achieve synergistic anticancer effects.Citation30,31-33
Disclosure of potential conflicts of interest
No potential conflicts of interest were disclosed.
References
- Klevorn LE, Teague RM. Adapting Cancer Immunotherapy Models for the Real World. Trends Immunol 2016; 37:354-63; PMID:27105824
- Sharma P, Allison JP. The future of immune checkpoint therapy. Science 2015; 348:56-61
- Chen L, Han X. Anti-PD-1/PD-L1 therapy of human cancer: past, present, and future. J Clin. Invest 2015; 125:3384-91; PMID:26325035; http://dx.doi.org/10.1172/JCI80011
- Mahoney KM, Rennert PD, Freeman GJ. Combination cancer immunotherapy and new immunomodulatory targets. Nat Rev Drug Discov 2015; 14:561-84; PMID:26228759; http://dx.doi.org/10.1038/nrd4591
- Verdegaal EM, de Miranda NF, Visser M, Harryvan T, van Buuren MM, Andersen RS, Hadrup SR, van der Minne CE, Schotte R, Spits H. Neoantigen landscape dynamics during human melanoma–T cell interactions. Nature 2016; 536:91-5; PMID:27350335
- Zou W, Wolchok JD, Chen L. PD-L1 (B7-H1) and PD-1 pathway blockade for cancer therapy: Mechanisms, response biomarkers, and combinations. Sci Transl Med 2016; 8:328rv4; PMID:26936508; http://dx.doi.org/10.1126/scitranslmed.aad7118
- Robert C, Schachter J, Long GV, Arance A, Grob JJ, Mortier L, Daud A, Carlino MS, McNeil C, Lotem M, et al. KEYNOTE-006 investigators. Pembrolizumab versus Ipilimumab in Advanced Melanoma. N Engl J Med 2015; 372:2521-32; PMID:25891173; http://dx.doi.org/10.1056/NEJMoa1503093
- Robert C, Long GV, Brady B, Dutriaux C, Maio M, Mortier L, Hassel JC, Rutkowski P, McNeil C, Kalinka-Warzocha E, et al. Nivolumab in Previously Untreated Melanoma without BRAF Mutation. N Engl J Med 2015; 372:320-30; PMID:25399552; http://dx.doi.org/10.1056/NEJMoa1412082
- Postow MA, Chesney J, Pavlick AC, Robert C, Grossmann K, McDermott D, Linette G. P, Meyer N, Giguere JK, Agarwala SS, et al. Nivolumab and ipilimumab versus ipilimumab in untreated melanoma. N Engl J Med 2015; 372:2006-17; PMID:25891304; http://dx.doi.org/10.1056/NEJMoa1414428
- Larkin J, Chiarion-Sileni V, Gonzalez R, Grob JJ, Cowey CL, Lao CD, Schadendorf D, Dummer R, Smylie M, Rutkowski P, et al. Combined Nivolumab and Ipilimumab or Monotherapy in Untreated Melanoma. N Engl J Med 2015; 373:23-34; PMID:26027431; http://dx.doi.org/10.1056/NEJMoa1504030
- Rosenberg JE, Hoffman-Censits J, Powles T, van der Heijden MS, Balar AV, Necchi A, Dawson N, O'Donnell PH, Balmanoukian A, Loriot Y. Atezolizumab in patients with locally advanced and metastatic urothelial carcinoma who have progressed following treatment with platinum-based chemotherapy: a single-arm, multicentre, phase 2 trial. Lancet 2016; 387:1909-0
- Mellati M, Eaton KD, Brooks-Worrell BM, Hagopian WA, Martins R, Palmer JP, Hirsch IB. Anti-PD-1 and Anti-PDL-1 Monoclonal Antibodies Causing Type 1 Diabetes. Diabetes Care 2015; 38:e137-8; PMID:26116720; http://dx.doi.org/10.2337/dc15-0889
- Boutros C, Tarhini A, Routier E, Lambotte O, Ladurie FL, Carbonnel F, Izzeddine H, Marabelle A, Champiat S, Berdelou A, et al. Safety profiles of anti-CTLA-4 and anti-PD-1 antibodies alone and in combination. Nat Rev Clin Oncol 2016; 13:473-86; PMID:27141885
- Naidoo J, Page DB, Li BT, Connell LC, Schindler K, Lacouture ME, Postow MA, Wolchok JD. Toxicities of the anti-PD-1 and anti-PD-L1 immune checkpoint antibodies. Ann. Oncol. 2015; 26:2375-91 mdv383; PMID:26371282
- Buchbinder EI, Hodi FS. Melanoma in 2015: Immune-checkpoint blockade - durable cancer control. Nat Rev Clin Oncol 2016; 13:77-8; http://dx.doi.org/10.1038/nrclinonc.2015.237
- Jeanbart L, Swartz MA. Engineering opportunities in cancer immunotherapy. Proc Natl Acad Sci USA 2015; 112:14467-72; http://dx.doi.org/10.1073/pnas.1508516112
- Pitt JM, Vétizou M, Daillère R, Roberti MP, Yamazaki T, Routy B, Lepage P, Boneca I. G, Chamaillard M, Kroemer G. Resistance Mechanisms to Immune-Checkpoint Blockade in Cancer: Tumor-Intrinsic and-Extrinsic Factors. Immunity 2016; 44:1255-69
- Wang C, Ye Y, Hochu GM, Sadeghifar H, Gu Z. Enhanced Cancer Immunotherapy by Microneedle Patch-Assisted Delivery of Anti-PD1 Antibody. Nano Lett 2016; 16:2334-40; PMID:26999507; http://dx.doi.org/10.1021/acs.nanolett.5b05030
- Quinn HL, Kearney MC, Courtenay AJ, McCrudden MTC, Donnelly RF. The role of microneedles for drug and vaccine delivery. Expert Opin Drug Deliv 2014; 11:1769-80; PMID:25020088; http://dx.doi.org/10.1517/17425247.2014.938635
- Kim YC, Park JH, Prausnitz MR. Microneedles for drug and vaccine delivery. Adv Drug Deliv Rev 2012; 64:1547-68; PMID:22575858; http://dx.doi.org/10.1016/j.addr.2012.04.005
- Ye Y, Yu J, Wang C, Nguyen NY, Walker GM, Buse JB, Gu Z. Microneedles integrated with pancreatic cells and synthetic glucose-signal amplifiers for smart insulin delivery. Adv Mater 2016; 28:3115-21; PMID:26928976; http://dx.doi.org/10.1002/adma.201506025
- Yu J, Zhang Y, Ye Y, DiSanto R, Sun W, Ranson D, Ligler FS, Buse JB, Gu Z. Microneedle-array patches loaded with hypoxia-sensitive vesicles provide fast glucose-responsive insulin delivery. Proc Natl Acad Sci USA 2015; 112:8260-5; PMID:26100900; http://dx.doi.org/10.1073/pnas.1505405112
- Sullivan SP, Koutsonanos DG, Del Pilar Martin M, Lee JW, Zarnitsyn V, Choi SO, Murthy N, Compans RW, Skountzou I, et al. Dissolving polymer microneedle patches for influenza vaccination. Nat Med 2010; 16:915-20; PMID:20639891; http://dx.doi.org/10.1038/nm.2182
- Kines RC, Zarnitsyn V, Johnson TR, Pang YYS, Corbett KS, Nicewonger JD, Gangopadhyay A, Chen M, Liu J, Prausnitz MR, et al. Vaccination with human papillomavirus pseudovirus-encapsidated plasmids targeted to skin using microneedles. Plos One 2015; 10:e0120797; PMID:25785935
- Minn AJ, Wherry EJ. Combination cancer therapies with immune checkpoint blockade: convergence on interferon signaling. Cell 2016; 165:272-5
- Wang C, Sun W, Wright G, Wang A, Gu Z. Inflammation-triggered cancer immunotherapy by programmed delivery of CpG and Anti-PD1 antibody. Adv Mater 2016
- Sun WJ, Gu Z. Engineering DNA scaffolds for delivery of anticancer therapeutics. Biomaterials Sci 2015; 3:1018-24; http://dx.doi.org/10.1039/C4BM00459K
- Sun W, Ji W, Hall JM, Hu Q, Wang C, Beisel CL, Gu Z. Self-assembled DNA nanoclews for the efficient delivery of CRISPR-Cas9 for genome editing. Angew Chem Int Ed 2015; 54:12029-33; http://dx.doi.org/10.1002/anie.201506030
- Sun W, Jiang T, Lu Y, Reiff M, Mo R, Gu Z. Cocoon-like self-degradable DNA nanoclew for anticancer drug delivery. J Am Chem Soc 2014; 136:14722-5; PMID:25336272; http://dx.doi.org/10.1021/ja5088024
- Demaria S, Coleman CN, Formenti SC. Radiotherapy: changing the game in immunotherapy. Trends Cancer 2016; 2:286-94
- Hu QY, Sun WJ, Wang C, Gu Z. Recent advances of cocktail chemotherapy by combination drug delivery systems. Adv Drug Deliv Rev 2016; 98:19-34; PMID:26546751; http://dx.doi.org/10.1016/j.addr.2015.10.022
- Goldberg MS. Immunoengineering: how nanotechnology can enhance cancer immunotherapy. Cell 2015; 161:201-4
- Gu L, Mooney DJ. Biomaterials and emerging anticancer therapeutics: engineering the microenvironment. Nat Rev Cancer 2016; 16:56-66; PMID:26694936; http://dx.doi.org/10.1038/nrc.2015.3