ABSTRACT
Influenza viral infections are significant global public health concerns due to the morbidity and mortality associated with acute respiratory disease, secondary complications, and pandemic threats; thus, continuous efforts have been made to develop potent influenza vaccines. In this study, 3 different mucosal adjuvants—alkyl polyglycoside (APG), gellan gum, and chitosan (CS)—were evaluated for their efficacy in intranasal A/H1N1 or B split influenza vaccines administered to BALB/c mice. Protective immunity was monitored by serum analysis for IgG, hemagglutination inhibition (HI), and neutralizing antibody levels, as well as mucosal IgA levels in nasal and pulmonary lavage fluids. Survival, body weight, lung viral titer, and pulmonary immunopathology were also examined following lethal influenza challenge. Notably, all adjuvants amplified the IgG and IgA immune responses (not detected in immunization of influenza B) and increased survival rate compared with controls administered adjuvant-free intranasal vaccines. Alternatively, intramuscular immunization stimulated IgG production, but had no effect on IgA levels. Our collective analysis identified that APG was the most effective intranasal adjuvant, as all mice survived influenza challenge with limited body weight loss, viral titer, and pulmonary pathology, similar to those observed with intramuscular vaccination. This evidence supports that APG can elicit both systemic and mucosal immunity, and may be an effective adjuvant in intranasal split influenza A/H1N1 and B vaccines.
Introduction
Influenza virus infection can cause mild to severe illness in humans, sometimes leading to death. Influenza epidemics occur annually; however, new influenza A virus subtypes can also cause occasional and unpredictable pandemics in naïve populations.Citation1 Currently, vaccination is considered the optimal approach to control widespread infection.
Split vaccines are commonly used by intramuscular (i.m.) administration for influenza because of their adequate immunogenicity and relative ease of production. Mucosal vaccination is an attractive alternative because of its safety and ability to induce mucosal-associated lymphoid tissue (MALT)-mediated immune responses. Moreover, intranasal (i.n.) vaccines can be administered by non-medically trained personnel, which may be advantageous during large-scale immunization campaigns likely to occur during pandemic outbreaks or in remote communities.
For i.n. immunization, split influenza vaccines are safer than live attenuated counterparts—particularly in young children under 2 years; however, they elicit little to no protective immunity.Citation2 Thus, mucosal adjuvants and delivery systems that efficiently target antigens to immune cells may improve the immune responses invoked by these vaccines.
Alkyl glycoside (APG) is an environmentally friendly, non-ionic surfactant that is synthesized from natural fatty alcohol and glucose. It has high surface activity and biodegradability, and is widely used in detergents, cosmetics, and food processing industry. Because of its capability to increase macromolecule absorption from nasal mucous membrane, we tried it and found that there were substantial serum hemagglutination inhibitory (HI) antibodies in mice several weeks after i.n. immunization with a split influenza vaccine containing APG.
Gellan gum is an acidic compound that neutralizes and undergoes cation-mediated condensation upon exposure to physiologic cations (Na+, K+, Ca2+), resulting in a solution-to-gel phase transition that increases the vaccine's viscosity. This promotes the adherence of vaccine components to mucosal surfaces in the upper respiratory tract and prolongs retention in the nasal cavity. Chitosan (CS) is a well-known polymer used to boost the mucosal immune response after i.n. immunization by several mechanisms to facilitate antigen uptake.Citation3 To select a most powerful adjuvant, we test these 3 candidate adjuvants together.
The present study examined the efficacy of 3 prospective mucosal adjuvants—APG, gellan gum, and CS—in BALB/c mice immunized with i.n. split influenza vaccine. Notably, we found that APG was the most effective in generating the mucosal and systemic immune responses necessary to inhibit influenza virus replication in the lung and increase the survival rate, thereby supporting its use as an adjuvant in split influenza vaccines.
Results
Systemic immune responses to split influenza vaccine
The aim of this study was to evaluate the immunogenicity of split influenza vaccines delivered i.n. with APG, gellan gum, or CS as mucosal adjuvants. Serum HI, neutralizing, and IgG antibodies against influenza A or B virus were then compared between groups. Because hemagglutinin (HA) virus (influenza) could agglutinate red blood cells of human or animal, HI reflect the production of specific antibodies to inhibit viral particles or HA. Neutralizing antibody is produced by B lymphocytes, which can bind to the antigen on the surface of pathogenic microorganisms, thereby preventing the pathogen from adhering to the target cell receptor and preventing the invasion of cells. IgG antibodies, a key index of humoral immunity, is also influenza virus specific. All these assay methods refer to Materials and Methods part.
APG and CS induced similarly high systemic hemagglutination inhibition responses
The protective potential of currently available influenza vaccines correlates with serum HI antibody levels. HI assays revealed that i.m. vaccination of influenza A induced high HI antibody titer 7 d after the second immunization (day 21), which decreased around day 42. In contrast, i.n. vaccination with APG or CS adjuvant induced about 50% of the HI antibody titer observed in i.m. vaccination counterparts on day 21, but this difference was not statistically significant (). For influenza B, i.m. vaccination induced a high HI antibody titer 14 d after second immunization (day 28) that was maintained for 2 weeks until day 42 (). In contrast, i.n. vaccination with APG and CS only induced ∼33% of the HI antibody of i.m. vaccinated mice on day 28. The adjuvant-free and gellan gum i.n. vaccinated mice showed only a modest immune response compared with those receiving APG or CS adjuvant following influenza A or B immunization.
Figure 1. Serum HI antibody titers at different times after immunization with influenza vaccines. (A) Immunization with H1N1 influenza vaccine. (B) Immunization with B influenza vaccine. Values are expressed as mean ± SD (n = 5). *Indicates p< 0.05, **Indicates p< 0.01, ***Indicates p< 0.001, compared with the group immunized i.m. All mice received one primer on day 0 and one booster vaccination on day 14 i.m.( positive control) or i.n. in different formulations with the same amount of vaccine(1.5μg of HA) and PBS without influenza antigen was administered i.n. as negative control.
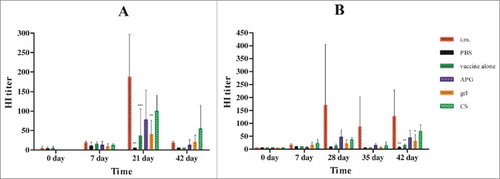
APG induced highest systemic neutralizing antibodies responses of 3 candidate adjuvants
Analysis of neutralizing antibody production is the best method to determine whether an individual can neutralize the infectivity of a given virus strain.Citation4 For influenza A, neutralizing antibodies was detected after the boost only in i.m. and APG groups (), with no significant difference between the 2 groups. For influenza B, the APG adjuvant induced highest neutralizing antibody titer of the 4 i.n. vaccination groups, while adjuvant-free i.n. vaccination induced the lowest (). Overall, i.n. vaccination groups elicited substantially lower induction of neutralizing antibodies when compared with that observed in i.m. vaccinated mice.
Figure 2. Serum neutralizing antibodies at different times after immunization with influenza vaccines. (A) Immunization with H1N1 influenza vaccine. (B) Immunization with B influenza vaccine. Bars and error bars represent means and standard deviations for each group (n = 5). Mice were immunized as described in .
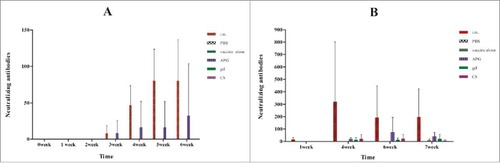
Systemic IgG responses were induced in all 3 candidate adjuvants
Total serum IgG responses against influenza antigens were induced in all adjuvanted i.n. vaccination groups, but were only ∼66% of the response observed in the i.m. group (). For influenza A, a significant difference was found between adjuvanted and non-adjuvanted groups, while the IgG levels in the different adjuvanted groups were similar. For influenza B, i.n. vaccination with APG induced highest IgG response among the 4 i.n. vaccination groups, but was only 50% of that found in i.m. mice. Therefore, it can be concluded that APG is an effective adjuvant for i.n. split inactivated influenza vaccines, although the resulting immunity is weaker than that produced by i.m immunization.
Figure 3. Serum IgG level at various times after immunization with influenza vaccines. (A) Immunization with H1N1 influenza vaccine. (B) Immunization with B influenza vaccine. IgG level was determined by ELISA method and results are expressed as mean ± SD (n = 5).*Indicates p< 0.05, **Indicates p< 0.01, ***Indicates p< 0.001, compared with the group immunized with vaccine alone. Immunization schedule was as in .
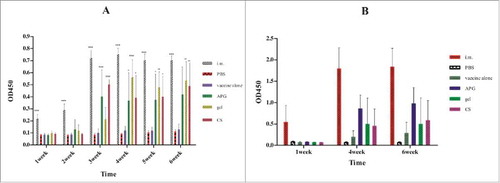
Local respiratory antibody responses- gellan gum and APG induced highest IgA titer in nasopharyngeal and pulmonary lavage fluids respectively
Since mucosal immunization can induce a local immune response, IgA levels were monitored by Enzyme-linked immunosorbent assay(ELISA) in nasopharyngeal and pulmonary lavage fluids collected from mice 4 weeks after the second vaccination. Notably, mice immunized with i.n. vaccines containing adjuvant showed a detectable increase in IgA antibodies in nasopharyngeal and pulmonary lavage fluid following influenza A challenge (), whereas those immunized with influenza B had no effect (data not shown). Among the 3 adjuvanted groups, mice administered with APG or gellan gum displayed the highest IgA titers in nasopharyngeal and pulmonary lavage fluids, respectively.
Figure 4. IgA level after immunization with H1N1 influenza vaccine (A, Nasal lavage fluid; B, lung lavage fluid)(n = 5). IgA level was determined by ELISA method. Each dot represents one individual and bars show the median.*Indicates p< 0.01, compared with the group immunized with vaccine alone. All mice received one primer on day 0 and one booster vaccination on day 14 i.m.( positive control) or i.n. in different formulations and PBS without influenza antigen was administered i.n. as negative control.
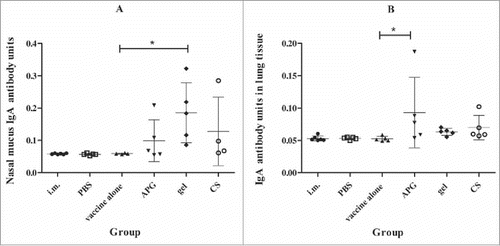
APG displayed highest protective immunity against lethal influenza virus challenge of 3 candidate adjuvants, close to that of i.m. immunization
Survival analysis showed that all mice immunized i.n. with adjuvant or i.m. survived lethal influenza A virus challenge, whereas only 40% of those given adjuvant-free i.n. immunization survived. However, all PBS control mice succumbed to infection, suggesting that addition of an adjuvant greatly improved the protective immunity conveyed by i.n. vaccination (). Moreover, weight loss rates in mice immunized i.n. with adjuvant-containing vaccines were substantially lower than those in mice administered adjuvant-free i.n. vaccines or PBS only (). Furthermore, almost no weight loss was observed in mice of vaccinated i.m. or i.n. with APG and gellan gum adjuvants. Alternatively, those receiving CS adjuvant or PBS groups started to regain weight at 7 and 10 d post-challenge, respectively.
Figure 5. Protection against challenge after immunization with H1N1 influenza vaccine (n = 5). The figure shows the survival rate (A), percentage of bodyweight compared with day 0 (B), virus load in lungs (C), pathology score (D) and H & E staining of lungs (E) over the 14 d following live H1N1 virus challenge of immunized mice. Mice were weighed daily from the date of the challenge to 14 d after the challenge. The rest mice were sacrificed and lungs were collected 5 d after challenge (lungs of mice were collected to calculate virus titers), and lung virus titers were determined as described in Materials and methods. Values are expressed as mean ± SD in A. Each dot represents one individual and bars show the median in C and D.*Indicates p< 0.05, **Indicates p<0.01, ***Indicates p< 0.001, compared with the group immunized i.m.
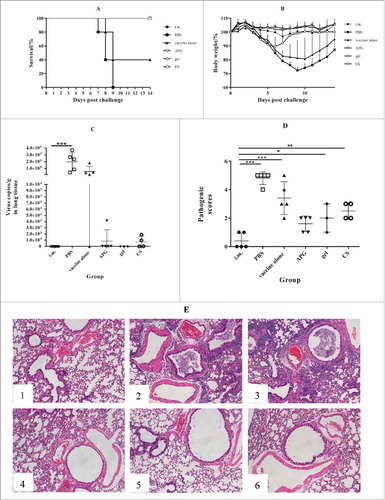
Viral clearance in the lung is a sign of effective immunity; thus, we compared lung virus titer 5 d after challenge. Interestingly, mice immunized i.n. with adjuvants, as well as the i.m. group, showed markedly lower virus titers compared with PBS controls. In particular, i.n. immunization with gellan gum showed a 5-log reduction of that in PBS control mice, while titers were only 25% lower in mice administered adjuvant-free i.n. vaccine. Thus, these results suggest that adjuvant APG, CS, or gellan gum effectively improved the protective effects of split influenza A vaccine.
To examine pulmonary immunopathology, lung lobes were harvested 5 d after challenge, stained with hematoxylin and eosin, and scored as summarized in . As expected, pathology scores correlated with the results of lung virus titers. Specifically, lower scores were observed in the i.m. immunization group and all adjuvanted i.n. immunization groups following influenza A challenge when compared with PBS controls. Further, the pathology observed in mice immunized with adjuvant-free i.n. vaccine was roughly similar to that of the control group.
For influenza B challenge, all mice immunized i.m. or i.n. with APG or CS survived, whereas only 67% or 60% of those receiving gellan gum or no adjuvant survived (). Thus, only APG or CS substantially enhanced protective immunity against influenza B. Similarly, mice administered APG or CS adjuvant showed only limited weight loss compared with mice immunized i.n. with vaccine alone or PBS controls (). However, surviving mice in the gellan gum or PBS groups started to regain weight at 9 or 10 d after challenge, respectively. Moreover, a 4- or 2-log decrease in lung virus titer was observed in i.m. or APG i.n. mice compared with PBS controls (). The CS, gellan gum, or vaccine alone groups exhibited no significant differences versus PBS control. Further, notable pulmonary pathology was observed in almost all (except APG) i.n. vaccinated groups comparable to PBS controls, whereas only limited damage was found in the i.m. immunization group and APG i.n. group (). Collectively, these data indicate that only the APG adjuvant was sufficient to enhance protective immunity in response to influenza B infection.
Figure 6. Protection against challenge after immunization with B influenza vaccine (n = 5). The figure shows the survival rate (A), percentage of bodyweight compared with day 0 (B), virus load in lungs (C), pathology score (D) and H & E staining of lungs (E) over the 14 d following live H1N1 virus challenge of immunized mice. Mice were weighed daily from the date of the challenge to 14 d after the challenge. The rest mice were sacrificed and lungs were collected 5 d after challenge (lungs of mice were collected to calculate virus titers), and lung virus titers were determined as described in Materials and methods. Values are expressed as mean ± SD in A. Each dot represents one individual and bars show the median in C and D.*Indicates p< 0.05, **Indicates p<0.01, ***Indicates p< 0.001, compared with the group immunized i.m.
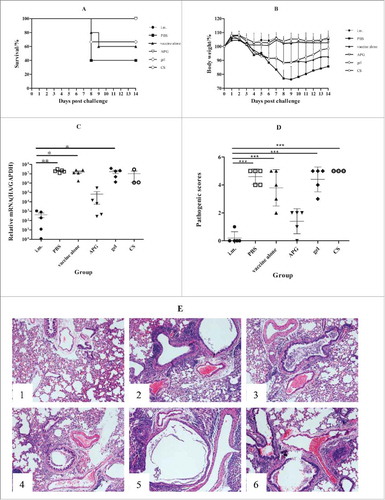
Discussion
The common mucosal immune system consists of several secondary lymphoid tissues, such as the Peyer's patches, nasopharyngeal-associated lymphoid tissue (NALT), and effector tissues of the intestinal and respiratory tracts, which prevent the invasion of infectious agents through mucosal surfaces.Citation5 Many i.n. vaccination strategies have been attempted against influenza virus infection,Citation6-9 including the i.n. live-attenuated vaccine Flumist®, which came into market in 2003Citation10. Live-attenuated vaccines mimic the natural influenza virus infection route to elicit strong, localized mucosal and cell-mediated immune responses that are more effective in preventing influenza infection when compared with inactivated counterparts. However, because of safety concerns, live vaccines can only be administered to healthy children and adults between 2 and 49 y old.Citation11 Thus, an i.n. inactivated influenza vaccine would be optimal with respect protective immunity and safety.
The limited immunogenicity of i.n. inactivated influenza virus vaccines have hindered their clinical application.Citation1 The nasal epithelium has a large, highly vascularized surface area that offers great opportunities for drug delivery; however, the thick mucus layer, enzymatic activity, and mucociliary clearance within the nasal cavity prevents adequate vaccine absorption. As such, various approaches have been investigated to improve immunogenicity of i.n. influenza vaccines in recent years, including the use of adjuvants such as CTA1-DD (Cholera toxin subunit A),Citation12 IL-12, IL-23Citation13, poly I:C (TLR3 agonist),Citation14-16 and CS,Citation17 as well as genetically modified whole viruses.Citation18 Several delivery systems have been developed to address this issue, including liposomes,Citation19-22 nanoparticles,Citation23 and microspheresCitation24; however, all of these attempts are still at pre-clinical stage maybe due to unsatisfactory immunogenicity, safety concern or complexity in production process. Thus, here we sought to compare the effects of 3 different mucosal adjuvants in i.n. split influenza vaccine that would yield high immunogenicity with increased safety and a feasible production process.
In the present study, mice immunized with i.n. inactivated influenza vaccine containing one of 3 potential adjuvants were monitored for protective immunity following lethal influenza and humoral immunity responses, compared with control mice that received adjuvant-free i.m. immunization. As expected, control mice displayed functional immunity after influenza vaccine A/B immunization, including very low viral titers in the lung and high serum IgG, HI, and neutralizing antibody levels. Similarly, mice that received i.n. vaccination with APG adjuvant survived without body weight loss and low lung viral titers, but relatively low serum IgG, HI, and neutralizing antibodies compared with those observed in i.m. controls. Further analyses indicated that complete protection may partly attributed to local immune responses induced by APG, as increased IgA levels were observed in nasopharyngeal and pulmonary lavage fluid. Nevertheless, we could not exclude the possibility of cellular immunity since cell responses were not examined. Basically, seasonal influenza vaccines with some adjuvants increase vaccine potency through enhanced antibody responses. For examples, addition of the MF-59 to subunit influenza vaccine leads to higher HI and neutralizing antibody titers.Citation25 However, Vaxfectin, a cationic lipid-based adjuvant, combined with seasonal influenza vaccine can significantly increase both specific antibody and T cell responses. The potential role of APG-induced T cell responses in the efficacy of influenza vaccine will be investigated further. As APG is a non-ionic surfactant, the enhanced immunity likely resulted from increased membrane permeability and antigen absorption. The CS adjuvant group showed 100% survival rate with <10% body weight loss in response to influenza virus A/B challenge; however, high lung viral titers were observed in influenza virus B challenged animals, which may be partly attributed to lower IgG and neutralizing antibody production. These data suggest that CS is only marginally effective as an adjuvant for i.n. trivalent split influenza vaccines. While CS is often used as an adjuvant in i.n. vaccines, its mechanism of action is not fully understood,Citation26 but likely function is to reduce the clearance of administered formulations from the nasal cavityCitation27 and/or facilitate antigen uptake into NALT through its transient effect on epithelial tight junctions. CS is also reported to exhibit immunomodulatory properties, such as natural killer (NK) cell and macrophage stimulation.Citation28 Further, its viscosity creates an environment favorable for antigen protection critical for its use as an experimental adjuvant in subcutaneous vaccinesCitation28; thus, it is possible that CS protects i.n. antigens before NALT uptake.
Finally, the gellan gum adjuvant group displayed 100% and 67% survival in influenza virus A and B challenges, with high lung viral titers in influenza B vaccination. This is consistent with previous data showing higher serum IgG levels in mice immunized with influenza vaccine A when compared with influenza B. As such, gellan gum is likely an unsatisfactory adjuvant for influenza B vaccinations despite its known function as a gelatinizing agent. Its adjuvanted mechanism seems to be increase formulation viscosity, then reduce the clearance of administered formulations.
In human, vaccines administered i.n. are mainly deposited in the anterior nasal cavity where mucociliary clearance can clear liquid formulations within 15–20 min, before effective antigen uptake by immune effectors.Citation1 Here, mice were immunized with i.n. influenza vaccines containing different adjuvants. Among them, gellan gum increased formulation viscosity from 0.018Pa.s to 1.016 Pa.s, CS changed the negative charge(−6.49 mV) to a positive charge(+59.2mV). These 2 adjuvants both helped to enhance influenza vaccine's i.n. retention. Otherwise, while all 3 adjuvants enhanced protective immunity above that observed in vaccine alone i.n. vaccinated mice, APG proved to be the most effective. Moreover, we also found that influenza B was more difficult to eliminate than influenza A, consistent with previous reports. Collectively, these data indicate that APG may be useful as an adjuvant in mucosal vaccines; however, further studies on tolerability, safety, efficacy, and mechanism of action are necessary before its transition to clinical application.
Materials and methods
Materials
The 2015–2016 season monovalent split inactivated vaccines for influenza A/NIB-74XP (H1N1) and B/Phuket/3073/2013 (henceforth referred to as influenza A and B, respectively) were kindly provided by Changchun Institute of Biological Products Co., Ltd. Influenza virus strains were purchased from National Institute for Biological Standards and Control (NIBSC). CS was purchased from Zhejiang Golden Shell Pharmaceutical Co., Ltd. (MW ≈1000,000 Da; degree of deacetylation = 90.68%, food grade). APG(APG1214, a mixture of dodecyl and tetradecyl) and gellan gum (low acyl)were purchased from Shanghai Fakai Chemical Co., Ltd. and Shanghai Zhong Wei Biochemical Co., Ltd, respectively. All other chemicals were of analytical grade.
Vaccine formulations
Intramuscular (i.m.) vaccine preparation
Virus was propagated in embryonated chicken eggs, purified, inactivated, and split. The final split inactivated influenza A and B solutions contained 717 μg and 460 μg of HA per milliliter, respectively. Inactivated virions were dissolved in phosphate buffered saline (PBS; 0.01 M, pH 7.2) at 15 μg/mL, 0.1 mL of which was administered into the quadriceps muscle of each mouse.
Intranasal (i.n.) vaccine preparation
For i.n. vaccines, split influenza virus (75 μg/mL) was dissolved with APG(10 mg/mL), gellan gum (5 mg/mL), or CS(10 mg/mL) adjuvants in PBS, water, or acetic buffer saline (0.1 M, pH 4.5), respectively. Split virus in PBS served as an adjuvant-free vaccine control.
Mouse immunization and challenge
All animal protocols were approved by the Institutional Animal Care and Use Committee of the Institut Pasteur of Shanghai, Chinese Academy of Science (Permit No: A2015026). Viral infection was performed in a biosafety level 2 facility with protocols approved by the institute's Biosafety Committee.
Mouse immunization
Split inactivated vaccines for influenza A and B were administered intranasally (i.n.) or intramuscularly (i.m.) to mice. Specific pathogen-free female BALB/c mice (purchased from Shanghai Lingchang BioTech Co.Ltd), aged 7–8 weeks, were maintained under sterile conditions throughout the study. Mice were transiently anesthetized with ether and immunized on days 0 and 14. For each influenza strain, 30 BALB/c mice were randomly divided into 6 groups (n = 5). Four groups were immunized i.n. with split influenza (10 μL/nostril; 1.5 μg of HA/mouse) with or without adjuvant. Control mice were given i.n. PBS or immunized in the quadriceps muscle with non-adjuvant split influenza (1.5 μg of HA/mouse).
Serum, nasopharyngeal, and pulmonary fluid collection
Blood was collected from the posterior orbital venous plexus before and after the first immunization at 2-week intervals and allowed to clot at room temperature. Sera was harvested from centrifuged samples and stored at −20 °C until analysis.
Nasopharyngeal and pulmonary fluid was collected 6 weeks after the first immunization. For this, mice were sacrificed and a smooth plastic cannula was inserted into an incision in the trachea. Two PBS (0.5 mL) washes were slowly injected and approximately 300 μL was collected from each mouse as pulmonary lavage fluid. Nasopharyngeal lavage fluid was harvested by injecting 0.5 mL of PBS into the tracheal cannula inserted in the opposite direction and collecting the fluid from the nostrils. All lavage samples were centrifuged and frozen at −20°C until analysis.
Immune analysis of vaccinated mice following influenza virus challenge
Twelve groups of 10 mice were immunized as described above (6 groups for influenza A and B, respectively). Four weeks following the last immunization, all mice were anesthetized and 5 × LD50 of influenza virus in 30 μL was administered i.n. Five mice from each group were weighed every day for 2 weeks to monitor weight loss and survival. Body weight loss of > 25% was considered as death. The remaining 5 mice in each group were killed 5 d post-challenge to obtain pulmonary tissue samples and immunopathology was scored by histological analysis as described previously.Citation29
For viral titers assays, lung tissue sections were mechanically disrupted with a Precellys homogenizer (Bertin Technologies, France) and RNA was isolated with TRIzol reagent (Invitrogen, Carlsbad, CA, USA).
For influenza A titration, the M genes were isolated from PR/8 viruses and cloned into the pMD18-T vector, which was used to generate a standard curve by 10-fold serial dilution. Viral RNA levels were assessed by qRT-PCR using SYBR Premix Ex TaqTM kit (TaKaRa, Dalian, China) according to the manufacturer's instructions with primers specific to the influenza A matrix protein gene (MP, Fwd: 5′-AAG ACC AAT CCT GTC ACC TCT GA-3′, Rev: 5′-CAA AGC GTC TAC GCT GCA GTC C-3′). Reactions were run on a 7900HT Real-Time PCR System (Applied Biosystems, USA) with the following protocol: an initial denaturation step at 95 °C for 30 seconds, followed by 40 cycles of 95 °C for 5 seconds and 60 °C for 30 seconds. All samples were analyzed in triplicate. Viral copy numbers were normalized with the original tissue sample masses, and calculated based on the standard curve described above.
For the influenza B virus titer, the HiScript II Q Select RT SuperMix qPCR and AceQ qPCR SYBR Green Master Mix kits (Vazyme) were used for RNA reverse transcription and qRT-PCR, respectively. GAPDH expression was used as an internal reference. Primers for this analysis were as follows: GAPDH, Fwd: 5′-CAT GTT TGT GAT GGG TGT GAA CCA-3′; Rev: 5′-AGT GAT GGC ATG GAC TGT GGT CTA-3′ B influenza HA, Fwd: 5′-AGA ACA AAA ATC AGG CAA CTA CCC-3′; Rev: 5′-ACA GTA ATT TGG TCT TCC CCT TCT-3′.
ELISA and micro-neutralization assays
Total serum IgG and IgA in nasopharyngeal and pulmonary lavage fluids were measured by ELISA as described previously.Citation29 Micro-neutralization assays were performed according to a previously published method.Citation29 Of note, the A/Puerto Rico/8/1934 H1N1 strain (Institute Pasteur of Shanghai, Chinese Academy of Sciences) was used in place of the A/NIB-74XP H1N1 strain because of the latter's low infectivity in Madin-Darby canine kidney (MDCK) cells.
HI assay
Specific anti-HA antibody levels in serum were determined with HI assays. Briefly, 15 μL of sera was incubated with 60 μL of receptor destroying enzyme (RDE, Denka Seiken Co., Ltd.) overnight at 37 °C and then heated for 50 min at 56 °C to clear non-specific inhibitors. Red blood cell adsorption was performed to remove nonspecific agglutinins and prevent false negatives.
Two-fold serial dilutions of sera (from 1:2 to 1:4096 in PBS) were prepared in 96-well plates and 25 μL of PBS containing 4 HA units of influenza virus was added to each well and incubated for 1 h at room temperature, followed by the addition of 25 μL of chicken RBCs (1% w/v in PBS) and another room temperature incubation for 30 min. Agglutination was determined by visual examination and HI antibody titer was expressed as the reciprocal of the highest serum dilution inducing hemagglutination inhibition after normalizing to a reference sample on each plate.
Statistical analysis
One-way ANOVA with post-hoc Tukey's testing was performed in GraphPad Prism 6.0 software to analyze the differences between groups. P < 0.05 was considered to be statistically significant.
Abbreviations
APG | = | Alkyl polyglycoside |
CS | = | Chitosan |
ELISA | = | Enzyme-linked immunosorbent assay |
HI | = | Hemagglutination inhibition |
Ig | = | Immunoglobulin |
IL | = | Interleukin |
i.m. | = | Intramuscular |
i.n. | = | Intranasal |
LD50 | = | Lethal dose; 50% |
MALT | = | Mucosal-associated lymphoid tissue |
MDCK | = | Madin-Darby canine kidney |
NALT | = | Nasopharyngeal-associated lymphoid tissue |
NK | = | Natural killer |
PBS | = | Phosphate buffered saline |
RBC | = | Red blood cell |
RDE | = | Receptor deactivating enzyme |
RT-PCR | = | Real time- polymerase chain reaction |
TLR | = | Toll-like receptor |
Disclosure of potential conflicts of interest
No potential conflicts of interest were disclosed.
Acknowledgments
We would like to thank Prof. Xuemei Zhang for providing the split inactivated influenza A/NIB-74 XP (H1N1) and B/Phuket/3073/2013 vaccines.
Funding
This research was supported by the Project of Pharmaceutical Engineering Technical Service Platform Construction from the Shanghai Municipal Science and Technology Commission [grant number 15DZ2290600].
References
- Coucke D, Schotsaert M, Libert C, Pringels E, Vervaet C, Foreman P, Saelens X, Remon JP. Spray-dried powders of starch and crosslinked poly(acrylic acid) as carriers for nasal delivery of inactivated influenza vaccine. Vaccine 2009; 27:1279-86; PMID:19114075; https://doi.org/10.1016/j.vaccine.2008.12.013
- Saito S, Ainai A, Suzuki T, Harada N, Ami Y, Yuki Y, Takeyama H, Kiyono H, Tsukada H, Hasegawa H. The effect of mucoadhesive excipient on the nasal retention time of and the antibody responses induced by an intranasal influenza vaccine. Vaccine 2016; 34:3258-62; https://doi.org/10.1016/j.vaccine.2016.01.020
- Tlaxca JL, Ellis S, Remmele RL Jr. Live attenuated and inactivated viral vaccine formulation and nasal delivery: Potential and challenges. Adv Drug Deliv Rev 2014; 93:56-78; PMID:25312673; https://doi.org/10.1016/j.addr.2014.10.002
- World Health Organization. Manual for the laboratory diagnosis and virological surveillance of influenza. Geneva: WHO Press; 2011.
- Yuki Y, Kiyono H. New generation of mucosal adjuvants for the induction of protective immunity. Rev Med Virol 2003; 13:293-310; PMID:12931340; https://doi.org/10.1002/rmv.398
- Sui Z, Chen Q, Fang F, Zheng M, Chen Z. Cross-protection against influenza virus infection by intranasal administration of M1-based vaccine with chitosan as an adjuvant. Vaccine 2010; 28:7690-8; PMID:20870054; https://doi.org/10.1016/j.vaccine.2010.09.019
- Wang D, Christopher ME, Nagata LP, Zabielski MA, Li H, Wong JP, Samuel J. Intranasal immunization with liposome-encapsulated plasmid DNA encoding influenza virus hemagglutinin elicits mucosal, cellular and humoral immune responses. J Clin Virol 2004; 31(Suppl 1):S99-106; PMID:15567101; https://doi.org/10.1016/j.jcv.2004.09.013
- Ichihashi T, Yoshida R, Sugimoto C, Takada A, Kajino K. Cross-protective peptide vaccine against influenza A viruses developed in HLA-A*2402 human immunity model. PLoS One 2011; 6:e24626; PMID:21949735; https://doi.org/10.1371/journal.pone.0024626
- Ninomiya A, Ogasawara K, Kajino K, Takada A, Kida H. Intranasal administration of a synthetic peptide vaccine encapsulated in liposome together with an anti-CD40 antibody induces protective immunity against influenza A virus in mice. Vaccine 2002; 20:3123-9; PMID:12163263; https://doi.org/10.1016/S0264-410X(02)00261-X
- Soema PC, Kompier R, Amorij JP, Kersten GF. Current and next generation influenza vaccines: formulation and production strategies. Eur J Pharm Biopharm 2015; 94:251-63; PMID:26047796; https://doi.org/10.1016/j.ejpb.2015.05.023
- Rose MA, Zielen S, Baumann U. Mucosal immunity and nasal influenza vaccination. Expert Rev Vaccines 2012; 11:595-607; PMID:22827245; https://doi.org/10.1586/erv.12.31
- Eliasson DG, El Bakkouri K, Schön K, Ramne A, Festjens E, Löwenadler B, Fiers W, Saelens X, Lycke N. CTA1-M2e-DD: a novel mucosal adjuvant targeted influenza vaccine. Vaccine 2008; 26:1243-52; PMID:18243429; https://doi.org/10.1016/j.vaccine.2007.12.027
- Khan T, Heffron CL, High KP, Roberts PC. Membrane-bound IL-12 and IL-23 serve as potent mucosal adjuvants when co-presented on whole inactivated influenza vaccines. Virol J 2014; 11:78; PMID:24884849; https://doi.org/10.1186/1743-422X-11-78
- Ichinohe T, Ainai A, Ami Y, Nagata N, Iwata N, Kawaguchi A, Suzaki Y, Odagiri T, Tashiro M, Takahashi H, et al. Intranasal administration of adjuvant-combined vaccine protects monkeys from challenge with the highly pathogenic influenza A H5N1 virus. J Med Virol 2010; 82:1754-61; PMID:20827774; https://doi.org/10.1002/jmv.21824
- Ichinohe T, Watanabe I, Ito S, Fujii H, Moriyama M, Tamura SI, Takahashi H, Sawa H, Chiba J, Kurata T, et al. Synthetic double-stranded RNA Poly(I:C) combined with mucosal vaccine protects against influenza virus infection. J Virol 2005; 79:2910-9; PMID:15709010; https://doi.org/10.1128/JVI.79.5.2910-2919.2005
- Ichinohe T, Ainai A, Tashiro M, Sata T, Hasegawa H. Poly I: polyC12U adjuvant-combined intranasal vaccine protects mice against highly pathogenic H5N1 influenza virus variants. Vaccine 2009; 27:6276-9; PMID:19840660; https://doi.org/10.1016/j.vaccine.2009.04.074
- Mann AJ, Noulin N, Catchpole A, Stittelaar KJ, de Waal L, Veldhuis Kroeze EJ, Hinchcliffe M, Smith A, Montomoli E, Piccirella S, et al. Intranasal H5N1 vaccines, adjuvanted with chitosan derivatives, protect ferrets against highly pathogenic influenza intranasal and intratracheal challenge. PLoS One 2014; 9:e93761; PMID:24850536; https://doi.org/10.1371/journal.pone.0093761
- Treanor JJ, Kotloff K, Betts RF, Belshe R, Newman F, Iacuzio D, Wittes J, Bryant M. Evaluation of trivalent, live, cold-adapted (CAIV-T) and inactivated (TIV) influenza vaccines in prevention of virus infection and illness following challenge of adults with wild-type influenza A (H1N1), A (H3N2) and B viruses. Vaccine 1999; 18:899-906; PMID:10580204; https://doi.org/10.1016/S0264-410X(99)00334-5
- Christensen D, Foged C, Rosenkrands I, Lundberg CV, Andersen P, Agger EM, Nielsen HM. CAF01 liposomes as a mucosal vaccine adjuvant: In vitro, and in vivo, investigations. Int J Pharm 2010; 390:19-24; PMID:19879346; https://doi.org/10.1016/j.ijpharm.2009.10.043
- Matsui M, Kohyama S, Suda T, Yokoyama S, Mori M, Kobayashi A, Taneichi M, Uchida T. A CTL-based liposomal vaccine capable of inducing protection against heterosubtypic influenza viruses in HLA-A*0201 transgenic mice. Biochem Biophys Res Commun 2010; 391:1494-9;PMID:20060099;https://doi.org/10.1016/j.bbrc.2009.12.100
- Thueng-in K, Maneewatch S, Srimanote P, Songserm T, Tapchaisri P, Sookrung N, Tongtawe P, Channarong S, Chaicumpa W. Heterosubtypic immunity to influenza mediated by liposome adjuvanted H5N1 recombinant protein vaccines. Vaccine 2010; 28:6765-77; PMID:20688037; https://doi.org/10.1016/j.vaccine.2010.07.065
- Even-Or O, Joseph A, Itskovitz-Cooper N, Samira S, Rochlin E, Eliyahu H, Goldwaser I, Balasingam S, Mann AJ, Lambkin-Williams R, et al. A new intranasal influenza vaccine based on a novel polycationic lipid-ceramide carbamoyl-spermine (CCS). II. Studies in mice and ferrets and mechanism of adjuvanticity. Vaccine 2011; 29:2474-86.
- Vila A1, Sánchez A, Janes K, Behrens I, Kissel T, Vila Jato JL, Alonso MJ. Low molecular weight chitosan nanoparticles as new carriers for nasal vaccine delivery in mice. Eur J Pharm Biopharm 2004; 57:123-31; PMID:14729088; https://doi.org/10.1016/j.ejpb.2003.09.006
- Singh M, Briones M, O'Hagan DT. A novel bioadhesive intranasal delivery system for inactivated influenza vaccines. J Control Release 2001; 70:267-76; PMID:11182197; https://doi.org/10.1016/S0168-3659(00)00330-8
- Smith LR, Wodal W, Crowe BA, Kerschbaum A, Bruehl P, Schwendinger MG, Savidis-Dacho H, Sullivan SM, Shlapobersky M, Hartikka J, et al. Preclinical evaluation of Vaxfectin-adjuvanted Vero cell-derived seasonal split and pandemic whole virus influenza vaccines[J]. Hum Vaccin Immunother 2013; 9(6):1333-45; https://doi.org/10.4161/hv.24209
- Smith A, Perelman M, Hinchcliffe M. Chitosan: a promising safe and immune-enhancing adjuvant for intranasal vaccines. Hum Vaccin Immunother 2014; 10:797-807; PMID:24346613; https://doi.org/10.4161/hv.27449
- Illum L, Jabbal-Gill I, Hinchcliffe M, Fisher AN, Davis SS. Chitosan as a novel nasal delivery system for vaccines. Adv Drug Deliv Rev 2001; 51:81-96; PMID:11516781; https://doi.org/10.1016/S0169-409X(01)00171-5
- Zaharoff DA, Rogers CJ, Hance KW, Schlom J, Greiner JW. Chitosan solution enhances both humoral and cell-mediated immune responses to subcutaneous vaccination. Vaccine 2007; 25:2085-94; PMID:17258843; https://doi.org/10.1016/j.vaccine.2006.11.034
- Song Y, Wang X, Zhang H, Tang X, Li M, Yao J, Jin X, Ertl HC, Zhou D. Repeated low-dose influenza virus infection causes severe disease in mice: A model for vaccine evaluation. J Virol 2015; 89:7841-51; PMID:25995265; https://doi.org/10.1128/JVI.00976-15