ABSTRACT
Background: The objective of this study was to explore the effects of viral co-detection in individuals recently vaccinated with the live-attenuated intranasal influenza virus vaccine (LAIV) on the detection of influenza RNA.
Methods: Before the 2013–2014 influenza season, nasal swabs were obtained from 59 pediatric participants with cystic fibrosis (CF) and 17 of their healthy siblings immediately before vaccination and 4 times during the week of follow-up. Real-time RT-PCR assays were used to detect influenza RNA. Co-detection of a non-influenza respiratory virus (NIRV) at the time of vaccination was determined by a multiplex RT-PCR assay. Differences in the proportions and rates of influenza detection and their 95% credible intervals (CrI) were estimated.
Results: Influenza RNA was detected in 16% fewer participants (95% CrI: −7, 39%) throughout follow-up in the NIRV-positive group compared with the NIRV-negative group (59% vs. 75%). This was also observed in participants with CF alone (66% vs. 74%; RD = 8% 95% CrI: −16, 33%) as well as in healthy participants only (75% vs. 30%; RD = 45%, 95% CrI: −2, 81%). Influenza was detected in NIRV-negative subjects for 0.49 d more compared with NIRV-positive subjects (95% CrI: −0.37, 1.26).
Conclusion: The observed proportion of subjects in whom influenza RNA was detected and the duration of detection differed slightly between NIRV- positive and −negative subjects. However, wide credible intervals for the difference preclude definitive conclusions. If true, this observed association may be related to a recent viral respiratory infection, a phenomenon known as viral interference.
Introduction
Influenza is the most common respiratory viral infection for which there is a vaccine. Seasonal vaccination is of special importance to individuals at high risk of morbidity resulting from influenza infection. Many acute pulmonary exacerbations in children with cystic fibrosis (CF) are associated with respiratory virus infections.Citation1 Flumist®, a live-attenuated influenza virus vaccine (LAIV) administered by intranasal spray, was approved for seasonal use in Canadians 2–59 y of age in June 2010. LAIV was subsequently introduced into publicly funded Canadian vaccination programs in the 2012–2013 influenza season. In Canada, LAIV is currently recommended for use in children and adolescents 2–17 y old, including non-immunosuppressed individuals with chronic conditions (including CF) with no contraindications to LAIV.Citation2 Recently, the U.S. Advisory Committee on Immunization voted against the use of LAIV for the 2016–17 influenza season, given its poor or relatively lower effectiveness when compared with the injectable vaccine from 2013 to 2016.Citation3
Viral interference is a phenomenon whereby infection with one virus limits infection and replication of a second virus.Citation4 Previous studies introduced the concept of viral interference between live vaccinesCitation5-12 and form the basis for the current vaccine recommendations, which advise to either administer live vaccines in the same bodily compartment concurrently or at an interval of 4 weeks.Citation13 The U.S. Advisory Committee on Immunization Practices recommends administering LAIV in the same way as other live vaccines are administered,Citation14 while the Canadian National Advisory Committee on Immunizations does not impose any specific interval between LAIV and other live-attenuated vaccines, as long as these are administered in different body compartments. According to the interference concept, LAIV replication in recent vaccinees could be impaired by interferon produced from a previously administered live vaccine or from naturally acquired non-influenza respiratory virus (NIRVs) infections. As replication of LAIV vaccine strains in the nasal mucosa is the first step required to induce protection, this interference may potentially impact LAIV effectiveness. This study represents the first step in evaluating this potential association.
CF is characterized by, among other things, abnormal inflammatory responses to viral respiratory infections,Citation15 Citation16 and chronic inflammation of the lungs and airways.Citation17 Both of these characteristics can impact the incidence and course of viral infections, making this patient population of particular interest when evaluating viral interference. The objective of this study was thus to explore the effects of viral co-detection (i.e. a pre-existing NIRV infection) on the detection of influenza RNA after vaccination with LAIV in children and adolescents with CF and their healthy siblings.
Results
A total of 76 subjects (59 with CF) attending the 2 participating CF clinics were enrolled and vaccinated with LAIV between October 8-November 28, 2013 (). The mean age of the cohort was 10.2 y (SD = 4.98, range: 2–19 years), about half the participants were female (39/76) and 95% (72/76) of the cohort was vaccinated against influenza in the year before study participation: 29 of 72 previously vaccinated (40%) had received LAIV and the remainder had received the trivalent-inactivated vaccine. One participant was lost to follow-up (after D0) and the D0-sample was missing for another participant. A total of 372 nasal swabs were received. Compliance with the protocol for obtaining nasal swabs from participants was high: of the self-procured samples, only 0.7% (2/304) did not have any detectable levels of influenza RNA and RNP (1 sample each on D1 and D7) and were considered missing. A very low amount of human DNA (CtRNP >37) was found in only 1 of 372 samples. Overall, 69% (52/75) of the study cohort had PCR-detected influenza RNA (either type A and/or B) during follow-up (D1-D7). Specifically, 24 subjects had RT-PCR detected influenza B virus RNA only, 7 had influenza A virus RNA detected only and 21 subjects had both virus types detected at least once throughout follow up. Detailed results of the detection of influenza viruses are published elsewhere.Citation18 Results were not stratified by influenza type given the small number of subjects.
Table 1. Demographic characteristics of study participants with CF and healthy siblings.
Genetic material from at least one NIRV was identified in 22 subjects on D0, and none of the viruses detected on D0 were influenza types A or B. At baseline, 3 healthy participants were infected with enterovirus-rhinovirus and one with human coronavirus 1. No healthy participants were found to be infected with 2 with NIRVs (referred to as co-infection) at baseline. Among participant with CF, 14 were found to be infected with enterovirus-rhinovirus, one with parainfluenza virus 1, one with parainfluenza virus 2, one co-infected with human coronavirus OC43 and enterovirus-rhinovirus and one co-infected with parainfluenza 4 and enterovirus-rhinovirus. The prevalence of detected NIRV on D0 was greater in subjects with CF compared with healthy subjects, although wide credible intervals preclude definitive conclusions (18/58 vs. 4/17, respectively; prevalence ratio: 1.52, 95% CrI: 0.58, 3.81).
Overall, fewer participants had detectable influenza RNA during follow-up in the group that had a NIRV infection detected at baseline compared with those that did not have an NIRV at baseline, however this difference was not statistically significant (59% vs. 75%; RD = 16% 95% CrI: −7, 39%). This was also observed in participants with CF alone (66% vs. 74%; RD = 8% 95% CrI: −16, 33%) and in healthy participants only (75% vs. 30%; RD = 45%, 95% CrI: −2, 81%). The effect estimate for the entire cohort was likely unbiased by diagnostic measurement error from the 2 viral detection assays used (). Rather, the estimated RD shifted slightly away from the null and the variability in the 95% CrI increased in both sensitivity analyses.
Figure 1. Results of the sensitivity analyses estimating the effect of measurement error in the diagnostic assays used to ascertain exposure (D0-NIRV) and outcome (detection of influenza RNA). The point and line edges represent the mean, 2.5th and 97.5th percentiles of the posterior distribution, respectively.
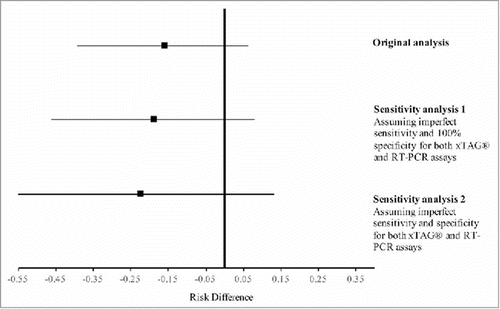
An episode of virus detection is defined as a stretch of consecutive samples positive for influenza virus genetic material. Among LAIV vaccinees in whom influenza RNA (either type A and/or type B) was detected throughout follow-up, 14 episodes of influenza virus detection occurred in the 13 D0-NIRV-positive subjects (total of 52 d of follow-up) compared with 44 episodes in the 39 D0-NIRV-negative subjects (total of 153 d of follow-up). This corresponds to 0.27 detection episodes/day in the NIRV-positive group (95% CI: 0.15, 0.43) versus 0.29 detection episodes/day in the NIRV-negative group (95% CI: 0.21, 0.38). There was almost an identical number of detection episodes in the 2 groups with and without viral co-detection at the time of vaccination: the D0-NIRV(−) experienced 0.02 more incident detection episodes per day compared with their D0-NIRV(+) counterparts (95% CrI −0.16, 0.17).
Furthermore, peak detection of influenza RNA in subjects that tested positive for a NIRV on D0 occurred later (peak at D4) than non-NIRV-infected subjects (peak at D1), regardless of CF status (). Detection appeared to decrease by D7 in all except healthy participants without an NIRV. This opposite trend observed likely relates to the increased variation due to the small number of D0-NIRV negative participants without CF. The mean duration of detection of RT-PCR-detected influenza RNA in the entire cohort was 2.09 d (95% CrI: 1.73, 2.48). The D0-NIRV-positive subjects had influenza RNA detected for 0.49 d more compared with NIRV-negative subjects, but wide credible intervals preclude definitive conclusions (95% CrI: −0.37, 1.26) ().
Figure 2. Proportion of subjects with any influenza virus RNA detection (either type (A)and/or B), by study day, cystic fibrosis (CF) status and non-influenza respiratory virus (D0-NIRV) detection status on day 0 (nCF, D0-NIRV+ = 18, nCF, D0-NIRV- = 40, nnon-CF, D0-NIRV+ = 4, nnon-CF, D0-NIRV- = 13).
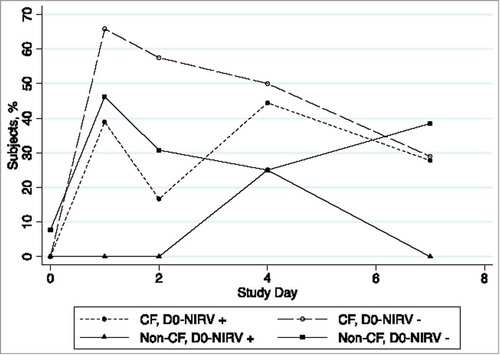
Table 2. Summary of influenza detection after vaccination with LAIV in study cohort (days 1–7 of follow-up).
The study had too small a sample size to accurately estimate the effects of both NIRV co-detection and CF status on the detection of influenza virus RNA post-LAIV vaccination (see Details & Results of Univariable and Multivariable Logistic Regression Models, see Appendix B Section 1, Supplemental Material).
Discussion
Overall, the prevalence of detected NIRV on D0 was greater (albeit statistically non-significantly) in subjects with CF compared with their healthy sibling participant counterparts. Some published evidence does not support the theory that pediatric patients with CF experience an increased incidence of laboratory-confirmed respiratory viral infections compared with healthy subjects,Citation19-21 while one recent study supports this theory.Citation22 Rather, it has been postulated that the increased prevalence of viral respiratory infections may be attributed to differences in the duration of infections (and the consequent duration of viral nucleic acid detection) due to a diminished specific antiviral defense Citation22-24 in patients with CF.Citation25 Furthermore, our 2 sensitivity analyses indicated that the RD estimate was robust to potential information bias from the diagnostic assays used to detect the presence of respiratory viruses. The increased variability in the sensitivity analyses did, however, highlight the need for adequate sample size to account for this potential measurement error when making inferences using these diagnostic assays.
We also determined that a slightly larger proportion of D0-NIRV negative participants had influenza RNA detected following vaccination compared with those who were positive for NIRV. This moderate trend was also observed when the duration of the detection episodes was compared between both groups. No D0-NIRV positive subjects were symptomatic (chest congestion, coughing, difficulty breathing, wheezing and/or increased sputum) at baseline. Since detection of NIRV genetic material on D0 may represent a carryover from a recent previous infection, the differences in the detection of influenza virus post-LAIV administration in those with and without a NIRV infection could be related to the primary respiratory virus infection. This phenomenon, described as viral interference, occurs when infection with one virus limits infection and replication of a second virus.Citation4 Mechanisms postulated to contribute to viral interference include competition for cellular receptors, competition for molecular substrates required for replication and induction of inhibitory host proteins, such as interferon, for the initiation of an anti-viral response.Citation26,27 In this way, individuals currently or recently infected with a respiratory virus on D0 could have less replication of the LAIV strains and thus less influenza RNA detected during follow-up. These findings support the results from a 2012 study which determined that viral detection was significantly shorter in patients who were co-infected by pH1N1 and NIRV or by 2 different NIRVs compared with those who were infected with only one virus (regardless of what virus was detected).Citation28 Furthermore, a large degree of bias in the overall RD estimate was not observed when potential misclassification of D0-NIRV status and influenza detection status during follow-up was taken into account This is not unexpected for 2 reasons. First, a large error rate was assumed from the literature for only the sensitivity of the diagnostic assay used to ascertain exposure (xTAG®). Second, the error rates for D0-NIRV infection and influenza RNA detection were assumed to be non-differential (independent).
The results of this study should be interpreted in light of several limitations. First, the RT-PCR primers used to identify influenza RNA were not specific to cold-adapted (LAIV) influenza viruses. It is therefore possible that the influenza viruses detected were naturally circulating, and not vaccine-strain. However, specimen collection occurred before the beginning of the seasonal epidemic in 2013, minimizing the possibility that the specimens collected were not LAIV. Similarly, as the virus detected on the nasal swabs was not cultured, the identity of the RT-PCR signal as live viruses cannot be definitively be confirmed. Second, we also found a larger number of influenza detection episodes than subjects, indicating the detection of influenza RNA on non-consecutive days during follow-up for 6 subjects. These biologically implausible results are likely due to sub-optimal swabbing during specimen collection or to the thawing of samples during transport. This could also mean that the viral concentration may have been below the RT-PCR assay's limit of detection. Third, some studies that evaluated the duration of viral detection of pandemic influenzaCitation29-31 required at least 2 consecutive negative specimens to infer the end of detection and avoid under-estimating the detection duration. We decided to not censor detection in this manner given that the study samples were not procured on consecutive days following vaccination, the one-week follow-up was of relatively short duration and non-consecutive detection was found for a small subset of the study population (8%). Fourth, the small sample size of our study should be emphasized, as the results obtained in this analysis may be due to chance alone. As highlighted by our sensitivity analyses, further research is required to explore and potentially confirm our findings. Fifth, we did not have information linking siblings in this data set and were thus unable to explore the effect of co-habitation of CF and non-CF siblings on D0-NIRV status. Finally, we were unable to evaluate whether influenza virus RNA detection differs between clinically apparent and asymptomatic D0-NIRV-positive subjects given that only one subject (D0-NIRV negative) was mildly symptomatic on the day of vaccination (coughing) and the estimation of D0-NIRV viral load was not possible. Similarly, changes in longer-term detection of influenza could not be evaluated given that follow-up was only for one week following vaccination. However, we believe this duration of follow-up is adequate since previous pediatric studies showed that influenza virus shedding post-LAIV was highest within this time period.Citation32-34
To our knowledge this is the first study conducted evaluating viral interference, a controversial and understudied topic in the realm of live-attenuated vaccines, in a pediatric cohort with CF. Given the potential signal for viral interference observed in this study cohort, results of this study also raise the question of whether LAIV's efficacy and effectiveness could be affected by a contemporary viral infection at the time of vaccination with LAIV.Citation35 As this study does not evaluate any of these outcomes, future research should first further assess the signal for viral interference with CF and, contingent on those results, then evaluate potential changes in vaccine efficacy associated with the presence of respiratory virus infection at the time of LAIV administration. While previous studies have evaluated the effect of viral interference between LAIV-strain viruses,Citation27 our research is also the first to evaluate the effect of a natural respiratory virus infection, at the time of LAIV-vaccination, on the detection of influenza in recent pediatric LAIV vaccinees. Given that viral replication is required to elicit protection by LAIVCitation27 and that viral interference may prevent LAIV-replication, further studies are required to determine the clinical significance of these findings.
Methods
Study population
Individuals 2 to 19 y of age with CF that had no contraindication to LAIVCitation18 were recruited for a prospective cohort study. Subjects were followed in either one of 2 participating CF clinics in Canada (British Columbia Children's Hospital, Vancouver and The Montreal Children's Hospital, Montreal). Participants' siblings were also recruited if they had no chronic health conditions, respiratory or other (hereby referred to as ‘healthy’). The informed consent for this study did not include a clause allowing for vaccination of contra-indicated children.
Vaccination & follow-up
After obtaining informed consent, participants were vaccinated as part of their regular process of care between October 8 and November 28, 2013, before the influenza season began. LAIV was administered to participants as a 0.1 mL aliquot spray in each nostril. Each dose contained approximately 106.5–7.5 fluorescent focus units of each of the 3 recombinant strains for the 2013–14 influenza season, as recommended by the World Health Organization: A/California/7/2009 (H1N1)pdm09-like virus, A/Texas/50/2012-like virus and B/ Massachusetts/2/2012-like virus.Citation36 Participants were followed for 8 d (day of vaccination plus 7 days) since previous pediatric studies showed that influenza virus shedding post-LAIV was highest within this time period.Citation32-34 Trained research nurses took flocked nasal swabs (Copan Diagnostics, Murrieta, CA, USA) from participants immediately before vaccination on day 0 (D0). Self-procured swabs (by participants and/or their parents, trained by research nurses) were obtained on days 1, 2, 4 and 7 (D1-D7, respectively) following vaccination. Swabs were placed in 2.0 mL of universal transport medium (Copan) after specimen procurement and then kept in home freezers and delivered frozen to the hospital sites. At the study sites, the specimens were stored at −80˚C. At the end of the study, all diagnostic testing for respiratory viruses was performed at the Laboratoire de Santé Publique du Québec.
Laboratory methods
Nucleic acids from D0 samples were extracted using the NucliSENS easyMag platform (bioMérieux, St. Laurent, Canada) according to the manufacturer's instructions and tested for the presence of NIRV genetic material with the xTAG® Respiratory Virus Panel FAST V2 (Luminex Corporation, Toronto, Canada) multiplex RT-PCR assay (Luminex Molecular Diagnostics Inc., Toronto, ON, Canada).Citation37 Specifically, the study samples were tested for the presence of: respiratory syncytial virus (RSV), influenza A virus (H1, H3 and 2009 H1N1 subtypes), influenza B virus, parainfluenza virus (types 1, 2, 3 and 4), human metapneumovirus (hMPV), adenovirus, enterovirus/rhinovirus, coronavirus (NL63, HKU1, 229E and OC43 species) and bocavirus. Reverse-transcription polymerase chain reaction (RT-PCR) assays were also used to detect influenza A and B RNA in participants' nasal swab samples (for more detailed information see RT-PCR Assay Methodology, Appendix A, Supplemental Material). A threshold cycle (Ct) value below 37 was considered positive for the RT-PCR assay. Specimens with Ct values higher than 37 in the first RT-PCR run were confirmed in a second RT-PCR run. Specimens that tested positive in both RT-PCR runs were considered positive even if the Ct values obtained were >37 (upper bound of 40). The human enzyme ribonuclease P (RNP) gene was used as an internal positive control for the RT-PCR assays to determine the presence of human nucleic acids and to confirm successful nucleic acid extraction.
Statistical analyses
Descriptive statistics and graphics were created using Stata 13.1. In addition, Bayesian methods were used to derive inferences for between-group comparisons. The proportions of interest as well as their 95% credible intervals (CrI) and that of the risk difference (RD) were estimated using the Gibbs sampler as implemented by WinBUGS (Version 1.4.3, MRC Biostatistics Unit, Cambridge, UK). Differences among the proportions of NIRV-positive and –negative groups were summarized from the posterior distribution, estimated using the binomial likelihood and Jeffreys prior (β (0.5,0.5)). Similarly, differences in influenza detection rates between NIRV-positive and –negative groups were summarized from the posterior distribution, estimated using a Poisson likelihood and a noninformative prior distribution (gamma(0.001,0.001)) on the Poisson parameter. Univariable and multivariable logistic regression models (with non-informative normal prior distributions on the intercepts and slopes) were used to further estimate the effect of NIRV-status at baseline on the detection of influenza RNA (yes/no) throughout follow-up. The missing outcome variable for one participant and the missing NIRV-status for another participant was multiply imputed (see Details & Results of Univariable and Multivariable Logistic Regression Models, Appendix B Section 1, Supplemental Material).
Sensitivity analysis: Measurement error
Both the xTAG® assay used for the identification of a D0-NIRV (exposure) and RT-PCR assays used for the detection of influenza RNA (outcome) are subject to a degree of inaccuracy. Consequently, misclassification of D0-NIRV infections and of influenza RNA detection during follow-up may bias the estimated RD. However, most analyses do not account for this potential bias and erroneously assume 100% sensitivity and 100% specificity for these diagnostic tests. We conducted 2 sensitivity analyses whereby we accounted for errors in the diagnostic tests by assigning informative prior distributions to the sensitivities and specificities of both assays (see Sensitivity Analysis Methodology, Appendix B Section 2, Supplemental Material). The first sensitivity analysis assumed perfect specificity (no false positive results) for both assays while in the second sensitivity analysis this assumption was relaxed.
Abbreviations
CF | = | Cystic Fibrosis |
Ct | = | threshold cycle |
LAIV | = | Live attenuated influenza virus vaccine |
NIRV | = | non-influenza respiratory virus |
RD | = | risk difference |
RNP | = | RNase P |
RT-PCR | = | Reverse transcription polymerase chain reaction |
Disclosure of potential conflicts of interest
The authors of this manuscript do not have a commercial or other association that might pose a conflict of interest (e.g., pharmaceutical stock ownership, consultancy, advisory board membership, relevant patents, or research funding).
• | Caroline Quach has received funding from GlaxoSmithKline, Pfizer, Sage and AbbVie (as investigator-initiated research grants). | ||||
• | Gaston De Serres has received funding from GSK and Pfizer as investigator-initiated research grants, was reimbursed travel expenses to attend a GSK ad hoc advisory committee and received honorarium from the Ontario Nurse Association for expert testimony on influenza vaccination | ||||
• | Jesse Papenburg has received funding from Becton, Dickenson and Company (as investigator-initiated research grants), has participated in an AbbVie ad hoc advisory committee and is on the advisory board of RPS Diagnostics. |
KHVI_A_1287641_SUPPLMENTARYFILES.zip
Download Zip (436.4 KB)Acknowledgements
MUHC Vaccine Study Center team, for recruitment of patients in Montréal and data management and the Vaccine Evaluation Centre (Vancouver) team for recruitment of patients in Vancouver.
Funding
Funding for this study was provided by Cystic Fibrosis (CF) Canada (Grant #2620) and the Quebec Ministère de la Santé et des services sociaux (MSSS). The Québec MSSS had no input in the study design, data analysis or the manuscript.
References
- Wat D, Gelder C, Hibbitts S, Cafferty F, Bowler I, Pierrepoint M, Evans R, Doull I. The role of respiratory viruses in cystic fibrosis. J Cyst Fibros 2008; 7:320-8; PMID:18255355; https://doi.org/10.1016/j.jcf.2007.12.002
- An Advisory Committee Statement (ACS) National Advisory Committee on Immunization (NACI) Statement on Seasonal Influenza Vaccine for 2014–15. July 2014. Available at: http://www.phac-aspc.gc.ca/naci-ccni/assets/pdf/flu-grippe-eng.pdf
- ACIP votes down use of LAIV for 2016–2017 flu season. In: SERVICES USDOHAH, ed.: CDC Newsroom, 2016.
- Laurie KL, Guarnaccia TA, Carolan LA, Yan AW, Aban M, Petrie S, Cao P, Heffernan JM, McVernon J, Mosse J, et al. Interval Between Infections and Viral Hierarchy Are Determinants of Viral Interference Following Influenza Virus Infection in a Ferret Model. J Infect Dis 2015; PMID:25943206
- Berger R, Just M, Gluck R. Interference between strains in live virus vaccines, I: Combined vaccination with measles, mumps and rubella vaccine. J Biol Stand 1988; 16:269-73; PMID:3198657; https://doi.org/10.1016/0092-1157(88)90014-5
- Stefano I, Sato HK, Pannuti CS, Omoto TM, Mann G, Freire MS, Yamamura AM, Vasconcelos PF, Oselka GW, Weckx LW, et al. Recent immunization against measles does not interfere with the sero-response to yellow fever vaccine. Vaccine 1999; 17:1042-6; PMID:10195613; https://doi.org/10.1016/S0264-410X(98)00320-X
- Simultaneous administration of varicella vaccine and other recommended childhood vaccines–United States, 1995–1999. MMWR Morb Mortal Wkly Rep 2001; 50:1058-61; PMID:11808928
- Petralli JK, Merigan TC, Wilbur JR. Action of Endogenous Interferon Against Vaccinia Infection in Children. Lancet (London, England) 1965; 2:401-5; PMID:14346758; https://doi.org/10.1016/S0140-6736(65)90755-5
- Petralli JK, Merigan TC, Wilbur JR. Circulating Interferon After Measles Vaccination. N Engl J Med 1965; 273:198-201; PMID:14306336; https://doi.org/10.1056/NEJM196507222730405
- Verstraeten T, Jumaan AO, Mullooly JP, Seward JF, Izurieta HS, DeStefano F, Black SB, Chen RT; Vaccine Safety Datalink Research Group. A retrospective cohort study of the association of varicella vaccine failure with asthma, steroid use, age at vaccination, and measles-mumps-rubella vaccination. Pediatrics 2003; 112:e98-103; PMID:12897314; https://doi.org/10.1542/peds.112.2.e98
- Giammanco G, De Grandi V, Lupo L, Mistretta A, Pignato S, Teuween D, Bogaerts H, Andre FE. Interference of oral poliovirus vaccine on RIT 4237 oral rotavirus vaccine. Eur J Epidemiol 1988; 4:121-3; PMID:2833407; https://doi.org/10.1007/BF00152704
- Vodopija I, Baklaic Z, Vlatkovic R, Bogaerts H, Delem A, Andre FE. Combined vaccination with live oral polio vaccine and the bovine rotavirus RIT 4237 strain. Vaccine 1986; 4:233-6; PMID:3026106; https://doi.org/10.1016/0264-410X(86)90135-0
- Canadian Immunization Guide. Part 4 - Active Vaccines 2015. Available at: https://www.canada.ca/en/public-health/services/publications/healthy-living/canadian-immunization-guide-part-4-active-vaccines.html
- Grohskopf LA, Sokolow LZ, Olsen SJ, Bresee JS, Broder KR, Karron RA. Prevention and Control of Influenza with Vaccines: Recommendations of the Advisory Committee on Immunization Practices, United States, 2015–16 Influenza Season. MMWR Morb Mortal Wkly Rep 2015; 64:818-25; PMID:26247435; https://doi.org/10.15585/mmwr.mm6430a3
- Sutanto EN, Kicic A, Foo CJ, Stevens PT, Mullane D, Knight DA, Stick SM; Australian Respiratory Early Surveillance Team for Cystic Fibrosis. Innate inflammatory responses of pediatric cystic fibrosis airway epithelial cells: effects of nonviral and viral stimulation. Am J Respir Cell Mol Biol 2011; 44:761-7; PMID:21317379; https://doi.org/10.1165/rcmb.2010-0368OC
- Xu W, Zheng S, Goggans TM, Kiser P, Quinones-Mateu ME, Janocha AJ, Comhair SA, Slee R, Williams BR, Erzurum SC. Cystic fibrosis and normal human airway epithelial cell response to influenza a viral infection. J Interferon Cytokine Res 2006; 26:609-27; https://doi.org/10.1089/jir.2006.26.609
- Nichols D, Chmiel J, Berger M. Chronic inflammation in the cystic fibrosis lung: alterations in inter- and intracellular signaling. Clin Rev Allergy Immunol 2008; 34:146-62; https://doi.org/10.1007/s12016-007-8039-9
- Boikos C, De Serres G, Lands LC, Boucher FD, Tapiero B, Daigneault P, Quach C. Safety of live-attenuated influenza vaccination in cystic fibrosis. Pediatrics 2014; 134:e983-91; PMID:25225145; https://doi.org/10.1542/peds.2014-0887
- Hiatt PW, Grace SC, Kozinetz CA, Raboudi SH, Treece DG, Taber LH, Piedra PA. Effects of viral lower respiratory tract infection on lung function in infants with cystic fibrosis. Pediatrics 1999; 103:619-26; PMID:10049966; https://doi.org/10.1542/peds.103.3.619
- Wang EE, Prober CG, Manson B, Corey M, Levison H. Association of respiratory viral infections with pulmonary deterioration in patients with cystic fibrosis. N Engl J Med 1984; 311:1653-8; PMID:6504106; https://doi.org/10.1056/NEJM198412273112602
- Ramsey BW, Gore EJ, Smith AL, Cooney MK, Redding GJ, Foy H. The effect of respiratory viral infections on patients with cystic fibrosis. American journal of diseases of children (1960) 1989; 143:662-8.
- Dijkema JS, van Ewijk BE, Wilbrink B, Wolfs TF, Kimpen JL, van der Ent CK. Frequency and Duration of Rhinovirus Infections in Children With Cystic Fibrosis and Healthy Controls: A Longitudinal Cohort Study. Pediatr Infect Dis J 2016; 35:379-83; PMID:26658528; https://doi.org/10.1097/INF.0000000000001014
- Zheng S, Xu W, Bose S, Banerjee AK, Haque SJ, Erzurum SC. Impaired nitric oxide synthase-2 signaling pathway in cystic fibrosis airway epithelium. Am J Physiol Lung Cell Mol Physiol 2004; 287:L374-81; PMID:15107292; https://doi.org/10.1152/ajplung.00039.2004
- Colasurdo GN, Fullmer JJ, Elidemir O, Atkins C, Khan AM, Stark JM. Respiratory syncytial virus infection in a murine model of cystic fibrosis. Journal of medical virology 2006; 78:651-8; PMID:16555274; https://doi.org/10.1002/jmv.20589
- van Ewijk BE, van der Zalm MM, Wolfs TF, Fleer A, Kimpen JL, Wilbrink B, van der Ent CK. Prevalence and impact of respiratory viral infections in young children with cystic fibrosis: prospective cohort study. Pediatrics 2008; 122:1171-6; PMID:19047230; https://doi.org/10.1542/peds.2007-3139
- DaPalma T, Doonan BP, Trager NM, Kasman LM. A systematic approach to virus-virus interactions. Virus Res 2010; 149:1-9; PMID:20093154; https://doi.org/10.1016/j.virusres.2010.01.002
- Bandell A, Woo J, Coelingh K. Protective efficacy of live-attenuated influenza vaccine (multivalent, Ann Arbor strain): a literature review addressing interference. Expert Rev Vaccines 2011; 10:1131-41; PMID:21854309; https://doi.org/10.1586/erv.11.73
- Pascalis H, Temmam S, Turpin M, Rollot O, Flahault A, Carrat F, de Lamballerie X, Gérardin P, Dellagi K. Intense Co-Circulation of Non-Influenza Respiratory Viruses during the First Wave of Pandemic Influenza pH1N1/2009: A Cohort Study in Reunion Island. PLoS ONE 2012; 7:e44755; PMID:22984554; https://doi.org/10.1371/journal.pone.0044755
- Bhattarai A, Villanueva J, Palekar RS, Fagan R, Sessions W, Winter J, Berman L, Lute J, Leap R, Marchbanks T, et al. Viral shedding duration of pandemic influenza A H1N1 virus during an elementary school outbreak–Pennsylvania, May-June 2009. Clin Infect Dis 2011; 52 Suppl 1:S102-8; PMID:21342880; https://doi.org/10.1093/cid/ciq026
- Suryaprasad A, Morgan OW, Peebles P, Warner A, Kerin TK, Esona MD, Bowen MD, Sessions W, Xu X, Cromeans T, et al. Virus detection and duration of illness among patients with 2009 pandemic influenza A (H1N1) virus infection in Texas. Clin Infect Dis 2011; 52 Suppl 1:S109-15; PMID:21342881; https://doi.org/10.1093/cid/ciq014
- Hien TT, Boni MF, Bryant JE, Ngan TT, Wolbers M, Nguyen TD, Truong NT, Dung NT, Ha do Q, Hien VM, et al. Early pandemic influenza (2009 H1N1) in Ho Chi Minh City, Vietnam: a clinical virological and epidemiological analysis. PLoS medicine 2010; 7:e1000277; PMID:20502525; https://doi.org/10.1371/journal.pmed.1000277
- Mallory RM, Yi T, Ambrose CS. Shedding of Ann Arbor strain live attenuated influenza vaccine virus in children 6–59 months of age. Vaccine 2011; 29:4322-7; PMID:21513761; https://doi.org/10.1016/j.vaccine.2011.04.022
- Vesikari T, Karvonen A, Korhonen T, Edelman K, Vainionpaa R, Salmi A, Saville MK, Cho I, Razmpour A, Rappaport R, et al. A randomized, double-blind study of the safety, transmissibility and phenotypic and genotypic stability of cold-adapted influenza virus vaccine. Pediatr Infect Dis J 2006; 25:590-5; PMID:16804427; https://doi.org/10.1097/01.inf.0000220229.51531.47
- Block SL, Yogev R, Hayden FG, Ambrose CS, Zeng W, Walker RE. Shedding and immunogenicity of live attenuated influenza vaccine virus in subjects 5–49 years of age. Vaccine 2008; 26:4940-6; PMID:18662737; https://doi.org/10.1016/j.vaccine.2008.07.013
- Moe N, Pedersen B, Nordbo SA, Skanke LH, Krokstad S, Smyrnaios A, Døllner H. Respiratory Virus Detection and Clinical Diagnosis in Children Attending Day Care. PLoS One 2016; 11:e0159196; PMID:27433803; https://doi.org/10.1371/journal.pone.0159196
- National Advisory Committee on Immunization (NACI) Statement on Seasonal Influenza Vaccine for 2013–2014. Canada Communicable Disease Report 2013; 39:ACS-4. Available at: http://www.phac-aspc.gc.ca/publicat/ccdr-rmtc/13vol39/acs-dcc-4/index-eng.php
- Xu W, Zheng S, Dweik RA, Erzurum SC. Role of epithelial nitric oxide in airway viral infection. Free Radic Biol Med 2006; 41:19-28; https://doi.org/10.1016/j.freeradbiomed.2006.01.037