ABSTRACT
Chronic periodontitis is caused by interactions between the oral polymicrobial community and host factors. Periodontal diseases are associated with dysbiotic shift in oral microbiota. Vaccination against periodontopathic bacteria could be a fundamental therapeutic to modulate polymicrobial biofilms. Because oral cavity is the site of periodontopathic bacterial colonization, mucosal vaccines should provide better protection than vaccines administered systemically. We previously reported that bacterial flagellin is an excellent mucosal adjuvant. In this study, we investigated whether mucosal immunization with a flagellin-adjuvanted polypeptide vaccine induces protective immune responses using a Porphyromonas gingivalis infection model. We used the Hgp44 domain polypeptide of Arg-gingipain A (RgpA) as a mucosal antigen. Intranasal (IN) immunization induced a significantly higher Hgp44-specific IgG titer in the serum of mice than sublingual (SL) administration. The co-administration of flagellin potentiated serum IgG responses for both the IN and SL vaccinations. On the other hand, the anti-Hgp44-specific IgA titer in the saliva was comparable between IN and SL vaccinations, suggesting SL administration as more compliant vaccination route for periodontal vaccines. The co-administration of flagellin significantly potentiated the secretory IgA response in saliva also. Furthermore, mice administered a mixture of Hgp44 and flagellin via the IN and SL routes exhibited significant reductions in alveolar bone loss induced by live P. gingivalis infections. An intranasally administered Hgp44-flagellin fusion protein induced a comparable level of Hgp44-specific antibody responses to the mixture of Hgp44 and flagellin. Overall, a flagellin-adjuvanted Hgp44 antigen would serve an important component for a multivalent mucosal vaccine against polymicrobial periodontitis.
Introduction
Periodontitis is a highly prevalent disease that ranges from simple inflammation to serious disease resulting in the destruction of alveolar bone and tooth loss.Citation1-4 It is well documented that this disease is associated with the polymicrobial biofilm residing in oral tissues.Citation5-9 The composition of the microbial biofilm is different in healthy and periodontal tissues, suggesting that periodontal disease is related to a shift of microbiota. Several bacterial species are predominantly observed in oral tissues from periodontitis patients than in healthy control.Citation10 Porphyromonas gingivalis, a gram-negative anaerobic bacterium, is one of the prevalent bacteria in the dysbiotic microbiome of periodontal pathology.Citation7,9-12 It has been suggested that interactions between P. gingivalis and other bacteria in the oral cavity are essential for disease progression.Citation9 Recently, prophylactic approaches to prevent periodontitis through vaccination against major etiologic pathogens, as an attempt to modify the disequilibrated polymicrobial biofilm into a healthy dental plaque, have been received much attentions.Citation11,13-15 Because P. gingivalis is an oral pathogen, salivary antibodies are expected to play a dominant role in optimal protective immune responses. However, systemic immunization via the subcutaneous (SC) and/or intramuscular (IM) route does not induce effective IgA production in mucosal secretions.Citation16,17 It has been reported that systemic vaccination with oral pathogens did not prevent murine experimental periodontitis.Citation18 Given that mucosal immunizations can induce both systemic and mucosal immune responses,Citation16,17,19 mucosal vaccines for periodontal disease are hypothesized to be a better option to induce protective immune responses. However, in general, mucosal vaccines require appropriate adjuvants for effective collaboration between the innate and adaptive immunity to induce the optimal immune response.Citation19
In our previous studies, we reported that a bacterial flagellin (Vibrio vulnificus FlaB), a cognate agonist of Toll-like receptor (TLR) 5, serves as an efficacious adjuvant for various mucosal vaccines against infectious diseases.Citation20-24 Recently, our group reported that TLR5 expression, unlike that of other TLRs, is maintained in immune cells isolated from 24-month-old mice, indicating that flagellin may be used as a promising immune activator during immunosenescence.Citation24 Periodontal disease is a representative aging-related chronic inflammatory disease.Citation1,2 Hence, mucosal periodontitis vaccines formulated with a flagellin adjuvant are hypothesized to provide substantial prevention or attenuation of periodontal diseases in the elderly population. To develop a successful vaccine against periodontal diseases, many research groups have struggled to identify anti-P. gingivalis protective antigens.Citation11,25 Gingipain, a cysteine protease produced by P. gingivalis, has been considered a major virulence factor,Citation26-28 and there have been many efforts investigating gingipain as a vaccine candidate.Citation25,29-32 It has been reported that a DNA vaccine expressing the Hgp44 domain of Arg-gingipain A (RgpA) induced a protective response against P. gingivalis-induced bone loss.Citation29 In this study, we hypothesized that FlaB would further potentiate the Hgp44-mediated immune response, especially in the oral mucosal environment. To test whether the vaccination with flagellin-adjuvanted Hgp44 would be efficacious for preventing periodontopathy, we evaluated Hgp44-specific antibody responses in both systemic and oral compartments and assessed the preventive efficacy against bone loss caused by live P. gingivalis infections. We also tested the efficacy of Hgp44-FlaB fusion protein since antigen-flagellin fusion combination vaccine was more potent than mixture in stimulating host antigen-specific immune responses in our previous study.Citation21
Results
Recombinant Hgp44 polypeptide antigen
We constructed the pCMM 9103 plasmid using the pTYB12 system and purified the Hgp44 protein as described previously.Citation23 The purity of the recombinant protein was confirmed by SDS-PAGE (). To test whether the P. gingivalis Hgp44 polypeptide could be recognized by the mouse immune system, we raised anti-P. gingivalis (anti-Pg) serum using formalin-inactivated bacteria and anti-Hgp44 serum using the purified recombinant antigen and performed immunoblot analysis. As shown in , the anti-Pg serum detected the Hgp44 antigen as indicated by SDS-PAGE, while pre-immune serum did not. Concurrently, the anti-Hgp44 sera could detect the Hgp44 polypeptide in the P. gingivalis lysate. The F. nucleatum cell lysate was used as a negative control (). This result suggests that the Hgp44 polypeptide could be displayed by P. gingivalis to be recognized by the host immune system. Next, to test whether the anti-Hgp44 antibody plays an inhibitory role in the host-bacteria interaction in vivo, we performed a hemagglutination inhibition assay (HIA) as described previously.Citation33-35 The anti-Hgp44 and anti-Pg sera showed an inhibitory effect on P. gingivalis-mediated hemagglutination, which is a surrogate virulence property of the pathogen, while the pre-immune sera did not inhibit hemagglutination (). Taken together, these data suggest that the Hgp44 polypeptide could be used as an appropriate subunit vaccine for further mucosal vaccination against P. gingivalis infection.
Figure 1. Development of the recombinant Hgp44 antigen. (A) The purity of Hgpp44 recombinant antigen was confirmed by SDS-PAGE. (B) Western blot analysis of the purified antigen using pre-immune and anti-P. gingivalis serum. (C) Evaluation of the Hgp44 polypeptide expression in P. gingivalis (ATCC33277) lysate was performed by western blotting using anti-Hgp44 while Fusobacterium nucleatum (ATCC 10953) lysate was used as control. (D) The hemagglutination inhibition assay (HIA). P. gingivalis was pre-incubated with the diluted Hgp44 antisera and then mixed with an equal volume of mouse RBC solution. The anti-Pg sera were used as a hemagglutination inhibitor control.
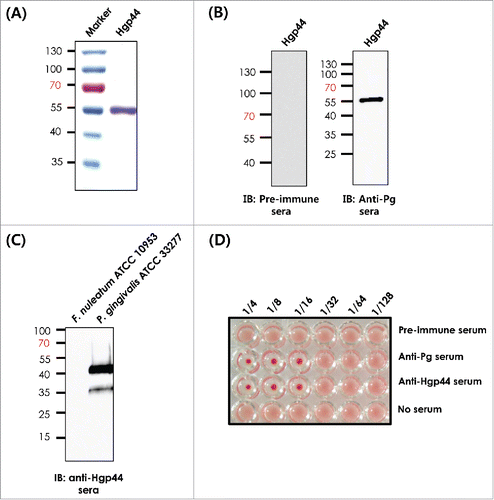
Hgp44-specific antibody responses induced by immunization of flagellin-adjuvanted Hgp44
To investigate whether flagellin-adjuvanted Hgp44 could be applied as a mucosal vaccine for periodontal disease, we immunized BALB/c mice with phosphate-buffered saline (PBS), Hgp44 alone (H) or a mixture of Hgp44 with flagellin (FlaB) (H+F) through the IN or sublingual (SL) routes 3 times in 2-week intervals. Two weeks after the final vaccination, the Hgp44-specific antibody responses were determined by ELISA. As shown in , the IN administration of Hgp44 induced significantly higher levels of antibody responses than the PBS control group, and the IN co-administration of flagellin potentiated the Hgp44-specific IgG in the serum. Although SL-immunized Hgp44 alone did not induce a significant serum IgG response, the co-administration of flagellin significantly enhanced (P < 0.001) the Hgp44-specific IgG response. Overall, the IN vaccination is more efficacious at inducing a systemic Hgp44-specific IgG response than the SL administration, and flagellin enhances the Hgp44-specific serum humoral immune response caused by both the IN and SL immunizations. The SL co-administration of flagellin induced a significant systemic humoral immune response against Hgp44. These results suggest that flagellin could help to overcome the low immunogenicity of SL subunit vaccines. Next, Hgp44-specific IgA titers were determined in the saliva () to evaluate the Hgp44-specific mucosal immune responses. In contrast to the Hgp44-specific IgG response in the serum, comparable levels of Hgp44-specific IgA were observed in the saliva of both IN- and SL-vaccinated mice. The co-administration of flagellin significantly potentiated the levels of Hgp44-specific salivary IgA in both the IN- (P < 0.05) and SL- vaccinated (P < 0.001) groups (). These results suggest that the SL vaccination exerted a predilection toward local IgA responses in the oral secretions.
Effect of flagellin-adjuvanted Hgp44 on alveolar bone loss induced by a P. gingivalis infection
To test the efficacy of the vaccines for preventing the pathological consequences of P. ginigivalis infection, vaccinated mice were challenged with live P. gingivalis by an oral gavage as described previously,Citation36 and P. gingivalis-induced bone loss was determined using micro-CT analysis ( and Fig. S1). Mice intranasally immunized with H+F showed significantly higher bone volume density (BV/TV; bone volume/tissue volume) values compared with the PBS and H groups (P < 0.05 and P < 0.01, respectively; left panel of ). SL immunization with H+F resulted in significant increases in the bone volume fraction relative to the PBS group (right panel of ). We also estimated the distance from the cemento-enamel junction (CEJ) to the alveolar bone crest (ABC), which increase if alveolar bone loss occurs, using the Mimics software (version 14.0). As shown in , H+F immunization reduced alveolar bone loss following both the IN (left panel of ) and SL (right panel of ) vaccinations (Fig. S1). These results suggest that IN and SL vaccinations with Hgp44 and flagellin induce a protective immune response to prevent alveolar bone loss caused by P. gingivalis infection. Together, the IN and SL vaccination with Hgp44 and flagellin serve as an efficacious prophylactic measure against P. gingivalis-induced periodontal diseases.
Figure 3. Protective immune response induced by flagellin-adjuvanted Hgp44 mucosal vaccination in a mouse P. gingivalis infection model. The mice were intranasally or sublingually immunized as described above. One week after the final immunization, the immunized mice were orally challenged with P. gingivalis (ATCC 33277) for 3 successive days as described in the Materials and Methods section. Forty days after the final challenge, the alveolar bone loss was determined by micro-CT. (A) Analysis of the micro-CT volumetric parameter (bone volume/tissue volume; BV/TV) (n = 8). The ROI was defined as a cuboidal bone body encompassing the roots of the M1 and M2 isolated from intranasally vaccinated or sublingually vaccinated mice. n = 4–6. (B) Determination of the distance from the CEJ to the ABC in the maxillae. Forty days after the final challenge, the alveolar bone loss was determined using micro-CT by measuring the distance from the CEJ to the ABC with M2. The data are presented as the mean ± SEM in each group. Naïve n = 4; PBS (IN) n = 14; H (IN) n = 7; H + F (IN) n = 8; PBS (SL) n = 16, H (SL) n = 8; H + F (SL) n = 7) *P < 0.05, **P < 0.01.
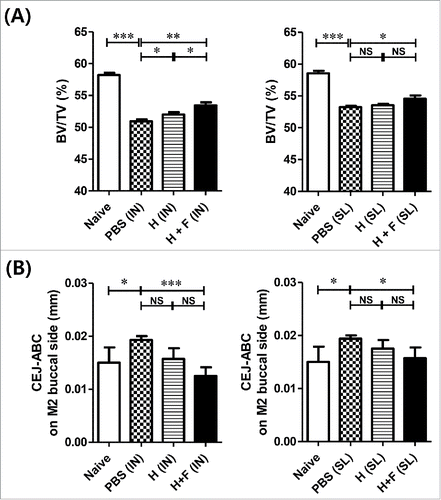
TLR5-stimulating activity of fusion proteins containing Hgp44 and flagellin (FlaB)
To test whether recombinant fusion proteins composed of Hgp44 and FlaB can be used as vaccine formulation, we constructed plasmids encoding the FlaB-Hgp44 (N-terminal FlaB and C-terminal Hgp44) and Hgp44-FlaB (N-terminal Hgp44 and C-terminal FlaB) fusion proteins. The recombinant proteins were purified using chitin affinity chromatography. The purity of recombinant proteins was confirmed by SDS-PAGE (). To test whether the fusion proteins induce TLR5-dependent signal transduction in the host cells, we performed an NF-κB luciferase reporter assay as described previously.Citation20,23 The NF-κB-stimulating activity of the HF fusion protein was significantly higher than that of FlaB alone (P = 0.0007) at equimolar concentrations (100 ng/ml FlaB vs. 209.5 ng/ml HF). Unexpectedly, the FH fusion protein did not induce TLR5-dependent NF-κB-stimulation (). To ascertain whether the FH fusion protein was inappropriate for TLR5 interaction, we analyzed the putative 3-dimensional structures of the FH and HF fusion proteins using the PyMol Molecular Graphics System Software. As shown in Figure S2, the HF fusion protein forms a stable 3-dimensional structure where 2 proteins are well separated and the TLR5 binding domain of FlaB appears to be freely exposed. In contrast, the putative TLR5 binding motif is hidden in the FH fusion protein. This analysis may provide an answer to why the FH fusion protein did not appropriately stimulate TLR5 signaling.
Figure 4. Development and evaluation of the recombinant fusion antigens. (A) SDS-PAGE of the recombinant fusion proteins. (B) Determination of the TLR5-dependent NF-κB stimulating activity by fusion proteins. Fusion-protein-mediated TLR5 signaling was determined using an NF-κB-luciferase reporter assay. The 293 cells were co-transfected with hTLR5 and pNF-κB-Luc and further treated with FlaB, FlaB-Hgp44 (FH) or Hgp44-FlaB (HF) for 24 h. The relative luciferase activities in the cell extracts were analyzed by a dual-luciferase reporter assay system and normalized using the pCMV-β-galactosidase plasmid as a control. The data are presented as the mean ± SEM in each group. ** P < 0.01, *** P < 0.001.
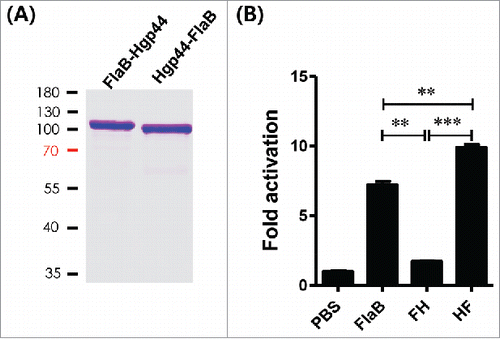
The Hgp44-specific antibody responses induced by the Hgp44-FlaB fusion protein
Since the H+F immunization induced enhanced immune responses in both the systemic and local compartments, we tested whether the HF fusion protein may further improve the protective immune responses. Mice were intranasally immunized with PBS, 4 μg of Hgp44 alone (H), 4 μg of FlaB with 4 μg of Hgp44 (F+H), or 8 μg Hgp44-FlaB (HF) 3 times at a 2-week interval as was mentioned in Materials and methods. Two weeks after the final immunization, the level of the Hgp44-specific serum IgG was measured by ELISA. As shown in , the F+H (P < 0.0001) and HF (P < 0.0001) groups had a significantly higher Hgp44-specific IgG titer than that of the H-alone group. Against our expectation, the HF and H+F groups had a similar level of Hgp44-specific serum IgG responses (). The F+H and HF groups induced a significantly higher Hgp44-specific IgA titer in the saliva than that of the H-alone group (P < 0.005 in both groups, ). The Hgp44-specific IgA titer in the HF group showed a similar level to the H+F group (P = 0.0650). The HF fusion formulation showed no superior efficacy in inducing Hgp44-specific antibody responses to the co-administration of Hgp44 and FlaB (). This result suggests that efficacy of epitope-flagellin fusion may be changed depending upon fusion partner antigens. In our previous study, streptococcal PspA specific immune response was significantly enhanced by the physical fusion with FlaB compared with the mixture formulation.Citation21 Additionally, we also measured the flagellin-specific antibody responses induced by the flagellin-adjuvated Hgp44 mucosal vaccine for both the mixture and fusion protein formulations to test whether repeated vaccination could induce unwanted flagellin-specific immune responses. After 3 IN vaccinations with PBS, 4 μg of FlaB (F), 4 μg of Hgp44 (H), 4 μg of FlaB with 4 μg of Hgp44 (H+F) or 8 μg of Hgp44-FlaB FlaB (HF), the FlaB-specific serum IgG titers were determined. Surprisingly, as shown Figure S3A, the H+F and HF groups showed significantly lower levels of FlaB-specific serum IgG titers than that of the F-alone group (P < 0.0001 in both groups). To confirm this result, we also performed western blotting analysis using the same sera. The antisera elicited by 3 administration of the vaccination of FlaB or Hgp44 recognized the cognate immunogen (Figure S3B). However, anti-H+F or anti-HF sera could not detect FlaB bands in the same immunoblot. Additionally, the band density detected by the anti-H+F or anti-HF was stronger than that of the anti-H sera. This result indicates that FlaB, in both the mixture and fusion protein formulations, preferentially drives the Hgp44-specific antibody responses while masking the immune responses toward adjuvant itself in vivo. The fusion protein may have an economical edge for further development since it would less costly for preclinical tests in comparison with the mixed formulation, which requires tests for each component and mixed formulations. Taken together, since periodontitis is a polymicrobial and multilayered infectious disease, the polyvalent vaccine formulation covering multiple periodontal pathogens is expected to be the final destination for periodontal vaccine development. The flagellin-adjuvanted Hgp44 vaccine, preferably as a fusion protein, could be used as a component of a polyvalent periodontal vaccine as either an antigen and flagellin mixture or a fusion protein formulation.
Figure 5. The Hgp44-specific antibody titers induces by IN vaccination. The mice were intranasally immunized with PBS, 4 μg of Hgp44 alone (H) or 4 μg of FlaB along with 4 μg of Hgp44 (H+F), and 8 \mu{g} Hgp44-FlaB fusion protein (HF); 3 times at 2-week intervals. Two weeks after the final vaccination, an ELISA was performed to determine the Hgp44-specific antibody. Determination of the anti-Hgp44-specific serum IgG (A) and saliva IgA (B) titers. The data are presented as the mean ± SEM in each group. n = 5. ** P < 0.01, *** P < 0.001, (NS) non-significant.
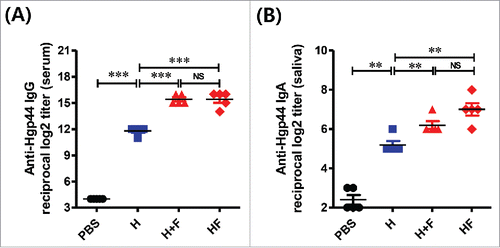
Discussion
In the present study, we have shown that flagellin serves an efficacious adjuvant to Hgp44 polypeptide-based mucosal vaccine to induce Hgp44-specific serum IgG and saliva IgA, thus preventing P. gingivalis-mediated alveolar bone loss. The Hgp44-FlaB fusion protein formulation showed efficacy equivalent to that of the Hgp44 and FlaB mixture in inducing Hgp44-specific antibody responses. Since flagellin is a proteinaceous TLR agonist, recombinant fusion proteins composed of antigenic epitope and flagellin would provide a useful approach for the development of polyvalent periodontal vaccines. It is well known that adjuvanted vaccines elicit an early, high and long-lasting antigen-specific immune responses. Concurrently, the adjuvant-mediated reactogenicity should be a serious concern. We show here that flagellin preferentially drives the Hgp44-specific antibody responses while masking the immune responses toward adjuvant itself in vivo. This is the first report that immune responses were differentially induced against co-administered antigen and flagellin adjuvant. This may not be a big surprise since immune responses are preferentially directed toward more immunogenic epitopes in the same antigen as was noted after influenza vaccination. When hemagglutinin antigen of influenza virus antigen was immunized to animals, antibody responses are apt to be directed more immunogenic globular head epitopes while those against less immunogenic stalk epitopes are less dominant.Citation37 Same phenomenon may be observed with separate antigens vaccinated in a same formulation. In the present study, we used P. gingivalis infection model system as a murine periodontal disease. Since periodontitis is a polymicrobial and multilayered infectious disease caused by interaction of host and polymicrobial biofilm, the P. gingivalis infection model cannot fully represent human periodontal disease. For humans, the polyvalent vaccine approach should be more physiological option. Our results suggest that flagellin may serve as an optimal adjuvant, as a separate adjuvant or a fusion partner of protein epitope, for polyvalent recombinant subunit vaccines for periodontal diseases.
The primary sites of P. gingivalis infection are the oral mucosae, therefore secretory IgA in the oral cavity is expected to play a dominant role in counteracting P. gingivalis-mediated pathogenesis. As shown in , SL and IN delivery of flagellin-adjuvanted Hgp44 vaccines induced equivalent levels of IgA production in the saliva. With the IN vaccination, nasopharynx-associated lymphoid tissue (NALT) acts as a mucosal immune inductive site.Citation38 Although SL-administered antigens can cross SL epithelial cells to ductal antigen presenting cells,Citation39 there is no known organized lymphoid organ in the oral mucosae, which probably accounts for the lower serum IgG levels in the SL-vaccinated mice. Our data show that the SL vaccination induced levels of salivary IgA that were comparable to those produced by the IN route (). In terms of administration compliance and safety concerns, the SL route is a superior choice to the IN route. Generally, SL-administered vaccines are easily diluted or degraded by saliva and various enzymes. In our previous study,Citation22 we have shown that (in contrast to intranasal immunization) flagellin does not have significant adjuvant activity when administered through the subcutaneous route. We speculated that flagellin (Vv-FlaB; 43kDa) has no pharmacokinetic advantages in systemic vaccination as compared with mucosal administration. Furthermore, systemic vaccination did not induce significant Ag-specific secretory IgA responses, which should be the target immune response of periodontal vaccines. However, this will be pointed out in the later part of Discussion as requested. Therefore, the combination of the IN and SL vaccinations in a prime-boost schedule may provide greater efficacy, and appropriate delivery systems with pharmacokinetic advantages are expected to enhance the beneficial effects of SL vaccinations.Citation40
The anti-Hgp44 serum detected 44- and 37-kDa bands in P. gingivalis lysate (). Considering that the 44 kDa Hgp44A domain of RgpA is generally processed into the 37-kDa Hgp44B,Citation28 our immunoblot results confirm that Hgp44 can induce specific antibodies that interact directly with P. gingivalis gingipain in vivo. As shown in , the anti-Hgp44 sera inhibited the hemagglutination induced by P. gingivalis, suggesting that anti-Hgp44 Ab could inhibit the host-bacteria interaction during infection. Because fibrinogen forms bridges between Hgp44 on the P. gingivalis surface and the GPIIb/IIa integrins of platelets,Citation28 it is likely that the serum anti-Hgp44 antibodies can inhibit P. gingivalis-mediated platelet aggregation and additional atherosclerotic inflammation. The prevention of bone loss highly correlated with the Hgp44-specific antibody responses. The protective effect of the H+F vaccination on alveolar bone loss was also shown in the acute gum pocket injection model, suggesting that the vaccine would protect from periodontal diseases from earlier stages of infection (data not shown).Citation14 Hgp44 should not be the only target for the development of periodontitis vaccines. Based upon our previous experience with recombinant protein and polyvalent vaccine formulation technologies,Citation22 we hypothesize that a flagellin-adjuvanted polyvalent mucosal periodontitis vaccine using additional protective antigen from co-infecting pathogen such as Fusobacterium will provide multilayered protection against pathologic biofilm formation and subsequent inflammatory tissue destruction. Since flagellin is a proteinaceous TLR agonist, the engineering of a recombinant flagellin protein with vaccine epitopes is expected to generate multiple platforms for the modification of vaccine components.Citation23,41-43
Materials and methods
Bacterial strains and plasmids
The bacterial strains and plasmids used in this study are listed in . P. gingivalis (ATCC 33277) was grown in tryptic soy broth (BD, Cat. 211825) supplemented with 0.5% yeast extract (BD, REF. 212750), 0.05% cysteine (Sigma, Cat. 168149), 10 μg/ml of hemin (Sigma, Cat. 51280), 5 μg/ml of menadione (Sigma, Cat. M5750), 0.1 mM of dithiothreitol (Amresco, Cat. 0281), and 50 μg/ml of gentamicin (Gibco, Cat. 15750–060) at 37°C under an anaerobic condition (85% N2, 10% H2, and 5% CO2).Citation44
Table 1. Bacterial strains and plasmids used in this study.
Plasmid construction for recombinant proteins
A DNA fragment encoding the Hgp44 domain of Arg-gingipain A (RgpA), which spans amino acid residues 716 to 1,135, was PCR-amplified from the genomic DNA of P. gingivalis ATCC 33277 using the following PCR primers: forward 5′-CATATGAGCGGTCAGGCCGAAATTGTG-3′ and reverse 5′-GTCGACTCTTTTGCCGTTAGCTTTGATCTCC-3′. Underlined sequences are endonuclease restrictions sites for cloning into vectors. The amplified DNA fragments were initially cloned into the pCR2.1 TOPO vector (Invitrogen, 45–0641), yielding the plasmid pCMM9101. The 1.3 kb hgp44 fragment was then cloned into the pTYB12 expression vector, yielding the pCMM9103 (). To produce the Hgp44-FlaB fusion recombinant protein, the Hgp44-specific DNA fragment was excised from the pCMM9101 with the appropriate restriction enzymes (NdeI-SalI) and subcloned at the N-terminus of the FlaB-specific DNA sequence which containing SalI-EcoRI overhangs in the pTYB12 vector, yielding the pCMM22005 (). Similarly, the pCMM22004 (FlaB-Hgp44) was constructed by the PCR-amplification of Hgp44-specific DNA sequences using the following PCR primers: forward 5′-GTCGACAGCGGTCAGGCCGAAATTGTG-3′ and reverse 5′-GAATTCTCTTTTGCCGTTAGCTTTGATCTCC-3′ with SalI-EcoRI overhangs. The amplified DNA fragment was cloned into the pCR2.1 TOPO vector. Then the Hgp44 DNA fragment was excited and inserted into the C-terminus of FlaB with NdeI-SalI in the pTYB12. For the optimal purification of the fusion proteins, the appropriate linker sequences were used. The DNA sequences of the resulting expression vectors were confirmed by the dideoxy-chain termination sequencing method via the Macrogen Online Sequencing Order System (http://dna.macrogen.com/kor/). The structure prediction of fusion proteins was performed using the PyMol Molecular Graphics System Software (http://pymol-molecular-graphics-system.soft112.com/)
Recombinant protein purification and immunoblot analysis
The recombinant proteins were prepared and analyzed as described previously.Citation21,23 The LPS level in the protein preparations was maintained below the FDA guideline (less than 0.15 EU/30 g for mouse).
Anti-P. gingivalis antibody production
Six-week-old female BALB/c mice were immunized with 2 × 109 inactivated P. gingivalis 3 times with one-week intervals. P. gingivalis (ATCC 33277) was inactivated with 0.3% (v/v) formalin (T&I, BPP-9004) overnight and thoroughly washed with PBS containing 137 mM of NaCl (AMRESCO, 2041), 2.7 mM of KCl (JUNSEI, 18190–0350), 10 mM of Na2HPO4 (JUNSEI, 84130–1250), and 1.8 mM of KH2PO4 (JUNSEI, 84185–0350). The inactivated bacteria were mixed with same volume of complete Freund's adjuvant (CFA) (Sigma, CAS9007–81–2), and the mixtures were subcutaneously injected for immunization.
Vaccination schedule
Mice (BALB/c, female, 8 weeks old) were intranasally (IN) (20 μl) or sublingually (SL) (10 μl) immunized with PBS, Hgp44 (4 μg), or Hgp44 (4 μg) with FlaB (4 μg) 3 times at 2-week intervals while under anesthesia from an intraperitoneal injection of 100 μl of PBS containing a mixture of 2 mg of ketamine (Virbac Laboratories, Zoletil®50) and 0.2 mg of xylazine (Bayer Korea, Rompun™). All animal experimental procedures were conducted in accordance with the guidelines of the Animal Care and Use Committee of Chonnam National University. In a separate study, mice were intranasally inoculated with final concentration of 4 μg of FlaB, 4 μg of Hgp44, or 8 μg of Hgp44-FlaB in 20 μl/mouse under the same schedule as mentioned above. After 2 weeks of immunization, the final sera and saliva were collected and stored at -80°C till use.
Hemagglutination inhibition assay
The hemagglutination inhibition assay was performed as described previously.Citation28 Briefly, anti-P. gingivalis and anti-Hgp44 sera were serially diluted in PBS in a 96-well round bottom plate (COSTAR, 3799). The pre-immunization serum was used as a negative control. Ten microliter of P. gingivalis suspension in PBS containing 108 cell was added to make a final volume 50 μl per well and incubated 1 hour at 37°C. To induce hemagglutination reaction, 50 μl of 2% mouse RBS suspension was added to the plate and then the plate was incubated at 37°C for 3 hours to determine hemagglutination inhibition (HAI) titers.
Challenge with live P. gingivalis
To assess the protection against live P. gingivalis challenge, vaccinated mice were infected with P. gingivalis ATCC33277.Citation29,31 Briefly, the vaccinated mice were orally administered 2 mg/ml of sulfamethoxazole and 0.4 mg/ml of trimethoprim (Samil Pharm, Septrin Syrup), for 3 d and then subjected to an antibiotic-free period of 3 more days. Live P. gingivalis (1 × 109 CFU, ATCC 33277) with 2% carboxymethylcellulose (Sigma, IGEPAL®CA-630) was administered into the oral cavity 3 times with a 2-day interval between treatments using feeding needle under anesthesia.
ELISA for Hgp44- or FlaB-specific antibody response
Two weeks after the final immunization, the serum and saliva were collected from immunized mice as described previously.Citation21 The saliva was collected from vaccinated mice following the intraperitoneal administration of 100 μl of 500 μg/ml of Isoto Carpine® (Novartis, 070414).Citation45 To measure the Hgp44-specific antibody responses, 96-well ELISA plates (Corning Laboratories, 3690) were coated with 1 μg/ml of Hgp44 in PBS for 24 hours at 4°C. For the FlaB-specific serum IgG and IgA measurement, FlaB was used as the coating antigen at a final concentration of 1 μg/ml in PBS. The plates were washed and blocked with blocking buffer containing PBS with 0.5% BSA (Sigma, cat. A2153–50G), 1 mM of EDTA (BIONEER, C-9007), and 0.05% Tween-20 (AMRESCO, 0777) for 1 hour at room temperature. After 5 washes, the serially diluted samples were incubated in the plates at RT for 2 h. Then horseradish peroxidase (HRP)-conjugated anti-mouse IgG or IgA Abs (Southern Biotech, 1030–05 or 1040–05, respectively) was added to the plates at RT for 1 h. The color was developed with 30 μl of TMB substrate (3,3′5,5′-tetramethylbenzidine) (BD OptEIA, 555214). The reaction was stopped by the addition of 30 μl of 1 N H2SO4. The absorbance was measured with a microplate reader (Molecular Devices Corp., Menlo Park, CA) at 450 nm. The titers were expressed as the reciprocal log2 value of the dilution that yielded an optical density at 450 nm.
Micro-computed tomography (micro-CT) analysis
Forty days after the last challenge of P. gingivalis, the right sides of the maxillae were collected, cleaned of soft tissue, and fixed in 10% formalin. Micro-CT images were captured with the high-resolution Skyscan 1172 system (Skyscan, Aartselaar, Belgium) with an X-ray source at 50 kV and 201 mA with a 0.5-mm aluminum filter. Images with a pixel size of 15 mm were captured every 0.7° over an angular range of 180°. The raw images were reconstructed into serial cross-sectional images with identical thresholds for all samples (0 to 8,000 in Hounsfield units) using an image reconstruction software (version 1.6.2.0, Skyscan). The bone volume density (BV/TV) in the region of interest (ROI) was determined using the CT Analyzer program (version 1.10.0.5, Skyscan) as described previously.Citation46-48 Three-dimensional surface rendering images were obtained with the Mimics imaging software (version 14.0, Materialise). The ROI length extended from the most distal aspect of the M2 root to the most mesial aspect of the M1 root. The width extended from the most buccal aspect to the most palatal (lingual) part of the M1 or M2 root, including the alveolar bone, and the height extended from the most apical aspect of the M1 or M2 crown to the most apical aspect of the M1 or M2 root. The linear distance of the CEJ to the ABC in maxillae M1 and M2 was determined using the Mimics software 14.0 (Materialise).
NF-κB luciferase reporter assay
To confirm whether the fusion protein could stimulate TLR5 signaling similarly as FlaB only, we performed a TLR5-stimuated NF-κB activity assay as described previously.Citation20 Briefly, overnight 2 × 105 of HEK293T cells/well were transfected with p3xFlag-hTLR5 (100 ng/well), the reporter pNFκB luc plasmid (100 ng/well), and 50 ng/well of pCMV-β-Gal (BD Biosciences lontech, Palo Alto, CA, USA) using 5 ul/well of Effectene (Qiagen, 301427). Twenty-four hours after transfection, the cells were treated with respective purified recombinant proteins (FlaB, FlaB-Hgp44, and Hgp44-FlaB) overnight based upon the molar ratio using 100 ng FlaB, and 209.5 ng FlaB-Hgp44 or Hgp44-FlaB per well. Transfected cells treated with PBS were used as a negative control. The cells were treated overnight and then lysed using lysis buffer (Promega, E153A), and the luciferase activity was measured by a luminometer (MicroLumat-Plus LB 96V, Berthold, Wilbad, Germany). The TLR5-stimulated luciferase signals from the respective samples were developed and measured as the fold-change activity relative to the negative control.
Western blotting analysis
Mice were immunized and sampled as mentioned above. Two weeks after the final immunization, 50 μl of antiserum from 5 individual animals per group were transferred to 1.5 ml of Eppendorf tubes and stored at -80°C for subsequent western blotting analysis. The same dilution (1/400 in PBS with 0.05% Tween 20) of antisera (primary antibody) from the respective samples and the same dilution (1/2000 in PBS with 0.05% Tween-20) of HRP-conjugated secondary antibody (Dako, P0260) were used to detect FlaB, and Hgp44 antigens separated on an 8% SDS-PAGE acrylamide gel. After the antigens were completely transferred onto the respective membranes, Ponceau S staining solution (Sigma, P7170) was used for that membranes subjected to SDS-PAGE western blotting to ensure the quality of the transferred antigens.
Statistical analysis
The results are expressed as the mean ± SEM unless otherwise noted. Student's t-test was used to compare 2 groups. All experiments were repeated more than 3 times, and the results from representative experiments are shown.
Disclosure of potential conflicts of interest
No potential conflicts of interest were disclosed.
Supplementary Figures
Download Zip (1.3 MB)Acknowledgment
The authors thank Hee Sam Na (Pusan National University, Korea) for critical help with the establishment of P. gingivalis infection model.
Funding
This study was supported by the Well Aging Research Center, Samsung Advanced Institute of Technology, by an NRF grant from the MSIP [NRF-2016R1A2B4009611], and by a COMPA grant from the MSIP [2016K000103] of the Republic of Korea.
References
- Darveau RP. Periodontitis: a polymicrobial disruption of host homeostasis. Nat Rev Microbiol. 2010;8:481–90. doi:10.1038/nrmicro2337. PMID:20514045
- Gil-Montoya JA, de Mello AL, Barrios R, Gonzalez-Moles MA, Bravo M. Oral health in the elderly patient and its impact on general well-being: a nonsystematic review. Clin Interv Aging. 2015;10:461–7. doi:10.2147/CIA.S54630. PMID:25709420
- Eke PI, Dye BA, Wei L, Slade GD, Thornton-Evans GO, Borgnakke WS, Taylor GW, Page RC, Beck JD, Genco RJ. Update on Prevalence of Periodontitis in Adults in the United States: NHANES 2009 to 2012. J Periodontol. 2015;86:611–22. doi:10.1902/jop.2015.140520. PMID:25688694
- Page RC, Offenbacher S, Schroeder HE, Seymour GJ, Kornman KS. Advances in the pathogenesis of periodontitis: summary of developments, clinical implications and future directions. Periodontol 2000. 1997;14:216–48. doi:10.1111/j.1600-0757.1997.tb00199.x. PMID:9567973
- Ng HM, Kin LX, Dashper SG, Slakeski N, Butler CA, Reynolds EC. Bacterial interactions in pathogenic subgingival plaque. Microb Pathog. 2016;94:60–9. doi:10.1016/j.micpath.2015.10.022. PMID:26541672
- Hajishengallis G, Lamont RJ. Beyond the red complex and into more complexity: the polymicrobial synergy and dysbiosis (PSD) model of periodontal disease etiology. Mol Oral Microbiol. 2012;27:409–19. doi:10.1111/j.2041-1014.2012.00663.x. PMID:23134607
- Hajishengallis G. Periodontitis: from microbial immune subversion to systemic inflammation. Nat Rev Immunol. 2015;15:30–44. doi:10.1038/nri3785. PMID:25534621
- Meyle J, Chapple I. Molecular aspects of the pathogenesis of periodontitis. Periodontol 2000. 2015;69:7–17. doi:10.1111/prd.12104. PMID:26252398
- Ng HM, Kin LX, Dashper SG, Slakeski N, Butler CA, Reynolds EC. Bacterial interactions in pathogenic subgingival plaque. Microb Pathog. 2015; PMID:26541672
- Hasan A, Palmer RM. A clinical guide to periodontology: pathology of periodontal disease. Br Dent J. 2014;216:457–61. doi:10.1038/sj.bdj.2014.299. PMID:24762896
- Jong RA, van der Reijden WA. Feasibility and therapeutic strategies of vaccines against Porphyromonas gingivalis. Expert Rev Vaccines. 2010;9:193–208. doi:10.1586/erv.09.156. PMID:20109029
- How KY, Song KP, Chan KG. Porphyromonas gingivalis: An Overview of Periodontopathic Pathogen below the Gum Line. Front Microbiol. 2016;7:53. doi:10.3389/fmicb.2016.00053. PMID:26903954
- Grover V, Kapoor A, Malhotra R, Kaur G. Porphyromonas gingivalis antigenic determinants–potential targets for the vaccine development against periodontitis. Infect Disord Drug Targets. 2014;14:1–13. doi:10.2174/1871526514666140827100930. PMID:25159307
- Liu PF, Haake SK, Gallo RL, Huang CM. A novel vaccine targeting Fusobacterium nucleatum against abscesses and halitosis. Vaccine. 2009;27:1589–95. doi:10.1016/j.vaccine.2008.12.058. PMID:19162109
- Reynolds EC, O'Brien-Simpson N, Rowe T, Nash A, McCluskey J, Vingadassalom D, Kleanthous H. Prospects for treatment of Porphyromonas gingivalis-mediated disease - immune-based therapy. J Oral Microbiol. 2015;7:29125. doi:10.3402/jom.v7.29125. PMID:26387645
- Holmgren J, Svennerholm AM. Vaccines against mucosal infections. Curr Opin Immunol. 2012;24:343–53. doi:10.1016/j.coi.2012.03.014. PMID:22580196
- Chen K, Cerutti A. Vaccination strategies to promote mucosal antibody responses. Immunity. 2010;33:479–91. doi:10.1016/j.immuni.2010.09.013. PMID:21029959
- Polak D, Wilensky A, Shapira L, Weiss EI, Houri-Haddad Y. Vaccination of mice with Porphyromonas gingivalis or Fusobacterium nucleatum modulates the inflammatory response, but fails to prevent experimental periodontitis. J Clin Periodontol. 2010;37:812–7. doi:10.1111/j.1600-051X.2010.01598.x. PMID:20670341
- Rhee JH, Lee SE, Kim SY. Mucosal vaccine adjuvants update. Clin Exp Vaccine Res. 2012;1:50–63. doi:10.7774/cevr.2012.1.1.50. PMID:23596577
- Lee SE, Kim SY, Jeong BC, Kim YR, Bae SJ, Ahn OS, Lee JJ, Song HC, Kim JM, Choy HE, et al. A bacterial flagellin, Vibrio vulnificus FlaB, has a strong mucosal adjuvant activity to induce protective immunity. Infect Immun. 2006;74:694–702. doi:10.1128/IAI.74.1.694-702.2006. PMID:16369026
- Nguyen CT, Kim SY, Kim MS, Lee SE, Rhee JH. Intranasal immunization with recombinant PspA fused with a flagellin enhances cross-protective immunity against Streptococcus pneumoniae infection in mice. Vaccine. 2011;29:5731–9. doi:10.1016/j.vaccine.2011.05.095. PMID:21696869
- Hong SH, Byun YH, Nguyen CT, Kim SY, Seong BL, Park S, Woo GJ, Yoon Y, Koh JT, Fujihashi K, et al. Intranasal administration of a flagellin-adjuvanted inactivated influenza vaccine enhances mucosal immune responses to protect mice against lethal infection. Vaccine. 2012;30:466–74. doi:10.1016/j.vaccine.2011.10.058. PMID:22051136
- Lee SE, Nguyen CT, Kim SY, Thi TN, Rhee JH. Tetanus toxin fragment C fused to flagellin makes a potent mucosal vaccine. Clin Exp Vaccine Res. 2015;4:59–67. doi:10.7774/cevr.2015.4.1.59. PMID:25649002
- Lim JS, Nguyen KC, Nguyen CT, Jang IS, Han JM, Fabian C, Lee SE, Rhee JH, Cho KA. Flagellin-dependent TLR5/caveolin-1 as a promising immune activator in immunosenescence. Aging Cell. 2015;14(5):907–15. doi:10.1111/acel.12383. PMID:26223660
- Frazer LT, O'Brien-Simpson NM, Slakeski N, Walsh KA, Veith PD, Chen CG, Barr IG, Reynolds EC. Vaccination with recombinant adhesins from the RgpA-Kgp proteinase-adhesin complex protects against Porphyromonas gingivalis infection. Vaccine. 2006;24:6542–54. doi:10.1016/j.vaccine.2006.06.013. PMID:16839648
- Bostanci N, Belibasakis GN. Porphyromonas gingivalis: an invasive and evasive opportunistic oral pathogen. FEMS Microbiol Lett. 2012;333:1–9. doi:10.1111/j.1574-6968.2012.02579.x. PMID:22530835
- Curtis MA, Kuramitsu HK, Lantz M, Macrina FL, Nakayama K, Potempa J, Reynolds EC, Aduse-Opoku J. Molecular genetics and nomenclature of proteases of Porphyromonas gingivalis. J Periodontal Res. 1999;34:464–72. doi:10.1111/j.1600-0765.1999.tb02282.x. PMID:10697803
- Nakayama K. Porphyromonas gingivalis cell-induced hemagglutination and platelet aggregation. Periodontol 2000. 2010;54:45–52. doi:10.1111/j.1600-0757.2010.00351.x. PMID:20712632
- Muramatsu K, Kokubu E, Shibahara T, Okuda K, Ishihara K. HGP44 induces protection against Porphyromonas gingivalis-Induced alveolar bone loss in mice. Clin Vaccine Immunol. 2011;18:888–91. doi:10.1128/CVI.00556-10. PMID:21430125
- Gibson FC, 3rd, Genco CA. Prevention of Porphyromonas gingivalis-induced oral bone loss following immunization with gingipain R1. Infect Immun. 2001;69:7959–63. doi:10.1128/IAI.69.12.7959-7963.2001. PMID:11705986
- Miyachi K, Ishihara K, Kimizuka R, Okuda K. Arg-gingipain A DNA vaccine prevents alveolar bone loss in mice. J Dent Res. 2007;86:446–50. doi:10.1177/154405910708600511. PMID:17452566
- O'Brien-Simpson NM, Pathirana RD, Paolini RA, Chen YY, Veith PD, Tam V, Ally N, Pike RN, Reynolds EC. An immune response directed to proteinase and adhesin functional epitopes protects against Porphyromonas gingivalis-induced periodontal bone loss. J Immunol. 2005;175:3980–9. doi:10.4049/jimmunol.175.6.3980. PMID:16148146
- Sakai E, Naito M, Sato K, Hotokezaka H, Kadowaki T, Kamaguchi A, Yamamoto K, Okamoto K, Nakayama K. Construction of recombinant hemagglutinin derived from the gingipain-encoding gene of Porphyromonas gingivalis, identification of its target protein on erythrocytes, and inhibition of hemagglutination by an interdomain regional peptide. J Bacteriol. 2007;189:3977–86. doi:10.1128/JB.01691-06. PMID:17384191
- Kadowaki T, Baba A, Abe N, Takii R, Hashimoto M, Tsukuba T, Okazaki S, Suda Y, Asao T, Yamamoto K. Suppression of pathogenicity of Porphyromonas gingivalis by newly developed gingipain inhibitors. Mol Pharmacol. 2004;66:1599–606. doi:10.1124/mol.104.004366. PMID:15361547
- Tagawa H, Kiyama-Kishikawa M, Lee SY, Abiko Y. Inhibition of hemagglutinating activity by monoclonal antibody against Porphyromonas gingivalis 40-kDa outer membrane protein. Hybrid Hybridomics. 2004;23:183–6. doi:10.1089/1536859041224244. PMID:15312309
- Momoi F, Hashizume T, Kurita-Ochiai T, Yuki Y, Kiyono H, Yamamoto M. Nasal vaccination with the 40-kgdalton outer membrane protein of Porphyromonas gingivalis and a nontoxic chimeric enterotoxin adjuvant induces long-term protective immunity with reduced levels of immunoglobulin E antibodies. Infect Immun. 2008;76:2777–84. doi:10.1128/IAI.01502-07. PMID:18411288
- Lee YT, Kim KH, Ko EJ, Lee YN, Kim MC, Kwon YM, Tang Y, Cho MK, Lee YJ, Kang SM. New vaccines against influenza virus. Clin Exp Vaccine Res. 2014;3:12–28. doi:10.7774/cevr.2014.3.1.12. PMID:24427759
- Pabst R. Mucosal vaccination by the intranasal route. Nose-associated lymphoid tissue (NALT)-Structure, function and species differences. Vaccine. 2015;33:4406–13.
- Nagai Y, Shiraishi D, Tanaka Y, Nagasawa Y, Ohwada S, Shimauchi H, Aso H, Endo Y, Sugawara S. Transportation of sublingual antigens across sublingual ductal epithelial cells to the ductal antigen-presenting cells in mice. Clin Exp Allergy. 2015;45:677–86. doi:10.1111/cea.12329. PMID:24773115
- Kraan H, Vrieling H, Czerkinsky C, Jiskoot W, Kersten G, Amorij JP. Buccal and sublingual vaccine delivery. J Control Release. 2014;190:580–92. doi:10.1016/j.jconrel.2014.05.060. PMID:24911355
- Foged C. Subunit vaccines of the future: the need for safe, customized and optimized particulate delivery systems. Ther Deliv. 2011;2:1057–77. doi:10.4155/tde.11.68. PMID:22826868
- Moyle PM, Toth I. Modern subunit vaccines: development, components, and research opportunities. ChemMedChem. 2013;8:360–76. doi:10.1002/cmdc.201200487. PMID:23316023
- Reed SG, Orr MT, Fox CB. Key roles of adjuvants in modern vaccines. Nat Med. 2013;19:1597–608. doi:10.1038/nm.3409. PMID:24309663
- Choi EK, Kim SY, Kim SH, Paek YW, Kang IC. Proteolytic activity of Porphyromonas gingivalis attenuates MCP-1 mRNA expression in LPS-stimulated THP-1 cells. Microb Pathog. 2014;73:13–8. doi:10.1016/j.micpath.2014.05.004. PMID:24875922
- Yamamoto M, Briles DE, Yamamoto S, Ohmura M, Kiyono H, McGhee JR. A nontoxic adjuvant for mucosal immunity to pneumococcal surface protein A. J Immunol. 1998;161:4115–21; PMID: 9780183.
- Xu XC, Chen H, Zhang X, Zhai ZJ, Liu XQ, Qin A, Lu EY. Simvastatin prevents alveolar bone loss in an experimental rat model of periodontitis after ovariectomy. J Transl Med. 2014;12:284. doi:10.1186/s12967-014-0284-0. PMID:25269614
- Liu YF, Wu LA, Wang J, Wen LY, Wang XJ. Micro-computerized tomography analysis of alveolar bone loss in ligature- and nicotine-induced experimental periodontitis in rats. J Periodontal Res. 2010;45:714–9. doi:10.1111/j.1600-0765.2010.01290.x. PMID:20572916
- Jeong BC, Choi H, Hur SW, Kim JW, Oh SH, Kim HS, Song SC, Lee KB, Park KB, Koh JT. Repair of Cranial Bone Defects Using rhBMP2 and Submicron Particle of Biphasic Calcium Phosphate Ceramics with Through-Hole. Biomed Res Int. 2015;2015:926291. doi:10.1155/2015/926291. PMID:26491693