ABSTRACT
Adjuvants are considered a necessary component for HBV therapeutic vaccines but few are licensed in clinical practice due to concerns about safety or efficiency. In our recent study, we established that a combination protocol of 3-day pretreatments with GM-CSF before a vaccination (3 × GM-CSF+VACCINE) into the same injection site could break immune tolerance and cause over 90% reduction of HBsAg level in the HBsAg transgenic mouse model. Herein, we further investigated the therapeutic potential of the combination in AAV8–1.3HBV-infected mice. After 4 vaccinations, both serum HBeAg and HBsAg were cleared and there was a 95% reduction of HBV-positive hepatocytes, in addition to the presence of large number of infiltrating CD8+ T cells in the livers. Mechanistically, the HBV-specific T-cell responses were elicited via a 3 × GM-CSF+VACCINE-induced conversion of CCR2-dependent CD11b+ Ly6Chi monocytes into CD11b+CD11c+ DCs. Experimental depletion of Ly6Chi monocytes resulted in a defective HBV-specific immune response thereby abrogating HBV eradication. This vaccination strategy could lead to development of an effective therapeutic protocol against chronic HBV in infected patients.
Introduction
HBV (Hepatitis B Virus) infection is a global threat and a major cause of chronic hepatitis, and hepatocellular carcinoma.Citation1 Current therapies based on interferon-α (IFN-α) and/or nucleotide/nucleoside analogs fail to clear viral infection in the majority of chronic hepatitis B (CHB) patients.Citation2,3 Immunological tolerance underlies viral persistence during chronic HBV infection.Citation4 Consequently, immunotherapeutic vaccine effectiveness is linked to ability to break the tolerance. Several candidate HBV therapeutic vaccines are based on DNA, peptide, dendritic cells, and recombinant proteins. Notably, appropriate adjuvants are necessary for these vaccines. In animal models, alum,Citation5 saponin,Citation6 and CpGCitation7 are used as effective adjuvants to enhance the efficiency of the HBV vaccine. However, safety concerns limit the extension to clinical practice. By contrast, several drugs and cytokines for treating human diseases, such as levamisoleCitation8 and GM-CSFCitation9 are also proven adjuvants that can evoke effective immune responses to HBV vaccines.
GM-CSF is a potent haematopoietic growth factor that has been used in clinical practice for more than 20 y. It can stimulate and enhance the production and activation of myeloid dendritic cells (DC).Citation10 Since GM-CSF promotes the function of antigen presenting cells (APCs), it has been used as an immunostimulatory adjuvant for experimental anti-tumor and anti-virus vaccines.Citation11,12 However, several contradictory studies indicated that the inappropriate use of GM-CSF could induce suppressive immune responses.Citation13 The underlying mechanisms remain to be defined, but the induction of immature DCs might be involved.Citation14,15 The capture of HBV antigens by DC before they had sufficient time to mature could have undesirable immunosuppressive effects.Citation16 With this consideration, we hypothesized that pre-treatment with GM-CSF for more than one day could promote DCs to become fully mature such that subsequent HBV vaccination could evoke a potent HBV-specific immune response in animals.
In a recent work, we designed a novel protocol by using a combination of GM-CSF and HBsAg vaccine and evaluated its effectiveness in an HBsAg-expressing transgenic mouse model (official model designation, Tg [Alb-1 HBV] Bri 44). One course constitutes daily GM-CSF for 3 times, followed by HBsAg vaccination (3 × GM-CSF+VACCINE). The mice were given 3 courses at 2-week intervals.Citation17 The HBsAg transgenic mice with HBV sequences encoding for viral proteins pre-S, S, and X did not respond to a conventional HBsAg vaccine. In contrast, we observed that the 3 × GM-CSF+VACCINE treatment achieved 90% reduction of HBsAg level in the model via the activation of HBsAg specific CD8+T cells.Citation18
Currently, the mouse is the most widely used experimental animal because of its short generation time and the fact that the mouse genome is easy to manipulate.Citation19 Various mouse models for persistent HBV infection have been generated in the past years, and these include HBsAg transgenic mice,Citation17 human liver chimeric mice for HBV infection,Citation20 and HBV genome delivery through hydrodynamic injection or through viral vector.Citation21,22 However, each model has its limitations. For example, 2 major limitations of HBV transgenic mice are that HBV replicates from an integrated transgene that cannot be cleared and mouse hepatocytes do not establish covalently closed circular DNA (cccDNA).Citation23 Thus, the goals of therapeutic vaccination, namely, HBV clearance and elimination of cccDNA, cannot be achieved in this model. Human hepatocyte chimeric mice with livers efficiently replaced with human hepatocytes become susceptible for HBV infection and allow the formation of HBV cccDNA, but these chimeric mice are difficult to generate.Citation24 Hydrodynamic injection (HDI) of the HBV genome in mice can cause significant hepatocyte damage.Citation25 Furthermore, HBV replication and antigen expression after HDI is transient and HBV-specific immune responses are induced at 2 weeks after injection.Citation21
Recently, a recombinant adeno-associated virus (AAV) carrying 1.3 copies of the HBV genome (AAV8–1.3HBV) was used to establish chronic HBV infection.Citation22,26 In AAV8–1.3HBV-transduced mice, the HBV genome was transduced into the hepatocytes and thus mimicked the natural HBV transcription template. Serum HBV DNA, HBeAg and HBsAg persisted for more than 10 months and no significant liver inflammation was observed; these observations were similar to those seen in chronic hepatitis B (CHB) patients in the immunotolerant stage.Citation27 Therefore, these AAV8–1.3HBV-infected mice have presented numerous interesting models for chronic HBV infection.Citation26 In this study, we further explore the immunopotential of 3 × GM-CSF +VACCINE and its underlying mechanism in this mouse model infected with AAV8–1.3HBV.
Results
Strategy for the 3 × GM-CSF+VACCINE immunization in the AAV8–1.3HBV mouse model
The schema of the proposed strategy for the 3 × GM-CSF+VACCINE immunization as applied in the AAV8–1.3HBV mouse model is illustrated in . The AAV8–1.3HBV infected mice were treated subcutaneously with 10 μg of GM-CSF once per day for 3 d and then subcutaneously injected with 1 μg of VACCINE at the same site. Treatment of all mice started on day 0, and 3 booster treatments were given, starting on days 14, 28, and 49. Mice treated with phosphate-buffered saline (PBS), GM-CSF, or VACCINE alone and GM-CSF/VACCINE co-administration were used as controls. Blood samples and local draining lymph nodes were obtained from each mouse at 24, 48, and 72 h post-vaccination. CD11 is commonly used as a mouse myeloid cells and DC marker.Citation28,29 CD11b+Ly6ChiCCR2+ monocytes and CD11b+CD11c+DC were analyzed by flow cytometry at each time point. Mice were killed 14 d after the fourth immunization. Serum samples were collected for IL-12, MCP-1, HBV DNA, HBeAg and HBsAg detection. Spleen cells were stimulated with 10 μg/mL HBsAg for 18 h in vitro. IFN-γ+CD8+ T cells were analyzed by flow cytometry. Liver tissue was obtained from each killed mouse. The liver sections were stained with hematoxylin-eosin for histological analysis. HBcAg- and CD8-positive cells in liver tissue were determined by immunohistochemistry (IHC).
Figure 1. 3 × GM-CSF+VACCINE promotes the activation of CD11b+ CD11c+DC. (A) Schematic illustration of the full immunization workflow in AAV8–1.3HBV infected mice. (B, H) Three days after the second immunization, blood and inguinal lymph nodes were collected from the different immunization groups and analyzed. The percentages of CD11b+CD11c+ DC in blood (B) and inguinal lymph nodes (C) are shown. (D-F) Comparison of markers on CD11b+CD11c+ DC in blood: CD80 (D), MHC-I (E), and MHC-II (F). (G-H) Serum concentrations of IL-12 (G), and monocyte chemoattractant protein-1 (MCP-1, H).
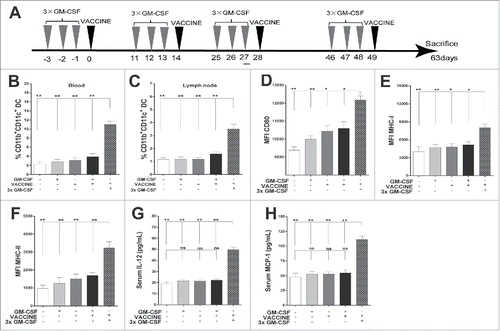
The 3 × GM-CSF+VACCINE promotes the activation of CD11b+ CD11c+DC
By design, the primary function of adjuvants is to activate antigen presenting cells (APCs), particularly DC, which are crucial to process and effectively present viral antigen and in turn to mount antigen-specific immune responses against invading pathogens.Citation30 To understand which APC subpopulation was activated by the 3 × GM-CSF+VACCINE regimen, we isolated APCs from peripheral blood and local lymph nodes of mice at 1 to 3 d after the second immunization of 3 × GM-CSF+VACCINE (). Mice that received the 3 × GM-CSF+VACCINE exhibited an approximately five- and threefold higher frequency of CD11b+CD11c+DC in the blood and local lymph nodes, respectively, compared with the control mice ( and ). However, this treatment did not alter the numbers of CD11b−PDCA-1+ plasmacytoid DC and CD11b+F4/80+ macrophages (Fig. S1A and S1B). To evaluate whether the 3 × GM-CSF+VACCINE promoted the activation of this newly increased DC population, we examined the expression levels of CD80, MHC-I and MHC-II ( to ) in the CD11b+CD11c+DC. The 3 × GM-CSF +VACCINE treatment significantly enhanced the expression levels of these functional molecules compared with the controls.
To further assess whether the 3 × GM-CSF+VACCINE induced a cytokine milieu environment containing cytokines in combinations that could subsequently influence the outcome of immune response, we examined several key inflammatory cytokines and chemokines in the serum using ELISA. The results showed that the 3 × GM-CSF+VACCINE treatments significantly enhanced the concentration of IL-12 () and of the monocyte chemo-attractant protein-1 (MCP-1), a chemokine that attracts monocytes and traffics them into an inflammatory site (). These results suggested that 3 × GM-CSF+VACCINE could markedly enhance the availability of immunogenic CD11b+CD11c+ DC in vivo.
The 3 × GM-CSF+VACCINE drives the production of CD11b+Ly6Chi monocytes
Monocytes can develop into DC in the presence of GM-CSF.Citation31 To evaluate whether the 3 pretreatments of GM-CSF (3 × GM-CSF) could influence monocyte differentiation into MoDC, we sorted blood CD11b+Ly6G− monocytes from wild type C57B/6 mice and treated them with GM-CSF once daily for 3 d (3 × GM-CSF) in vitro. Untreated cells and those treated once only with LPS or GM-CSF were used as controls. As shown in , × GM-CSF enriched the monocytes and promoted a higher rate of conversion to CD11c+ DC compared with the treatment with GM-CSF once. This was accompanied by higher levels of functional molecules CD80 (), MHC-I (), and MHC-II (), showing changes similar to those seen in vivo. To further characterize the function of these monocyte- derived DC (MoDC), we used the 3 × GM-CSF-induced MoDC for incubation with 10 μg/mL of HBsAg and purified splenic CD8+ T cells (2 × 105 cells/well) from AAV8–1.3HBV infected mice and further co-cultured them at a MoDC:T ratio of 1:10. As shown in and , the 3 × GM-CSF-induced MoDC promoted a significantly higher level of CD8+ T-cell proliferation than those induced by the single GM-CSF treatment.
Figure 2. 3 × GM-CSF+VACCINE drives the production of CD11b+ Ly6Chi monocytes. (A-F) CD11b+Ly6G−monocytes were sorted from PBMC of male wild-type C57BL/6 (n = 50) and treated in vitro with mGM-CSF (50 ng/mL) once and mGM-CSF (50 ng/mL) once per day for 3 d. LPS (1 μg/mL) was used as a positive control. (A) The percentage of CD11c+ DC induced by different cytokine formulations. (B-D) Comparison of CD80 (B), MHC-I (C), and MHC-II (D) on CD11c+ MoDC. (E, F) MoDC were further cultured for 72 hours with splenic CD8+T cells from AAV8–1.3HBV mice at a DC: T ratio of 1:10 and with HBsAg (10 μg/mL) then proliferative responses were assessed. Cells treated with 1μg/mL of CD3 and 100 ng/mL of CD28 were used as a positive control. (E) Representative flow cytometry results of CD8+ T cells proliferative experiment are shown. (F) The 3 × GM-CSF-induced MoDC promoted a significantly higher level of CD8+ T-cell proliferation than those induced by the single GM-CSF treatment. CD11b+Ly6Chi monocytes and CD11b+Ly6Clo monocytes in blood from AAV8–1.3HBV-infected mice that was collected 24 hours after the second immunization with 3 × GM-CSF+VACCINE were quantified by flow cytometry (G) and percentage of total CD11b+ cells (H). Numbers adjacent to outlined area indicate percent CD11b+Ly6Chi monocytes (top), and CD11b+Ly6Clo monocytes (bottom). Bars are shown as mean ± SEM. *, P<0.05; **, P<0.01; ***, P<0.001; ns, not significant.
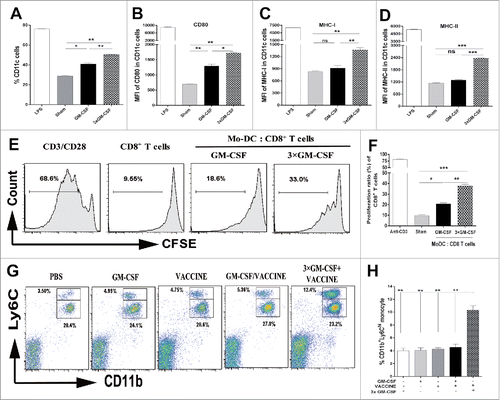
Figure 3. 3 × GM-CSF+VACCINE induces robust HBV specific CD8+ T-cell response in vivo. (A,B) 14 d after the fourth immunization, spleen cells were stimulated with 10 μg/mL HBsAg for 18 hours in vitro or with PMA (100 ng/mL) and Ionomycin (1 μg/mL) for 6 hours as a positive control. (A) Representative flow cytometry results of IFN-γ+/CD8+ T cells are shown. (B) The percentage of CD8+ T cells that were IFN-γ+ is presented. (C) IHC for CD8+ T cells (brown staining) on liver sections at 14 d after the fourth immunization. Scale bar represents 50 μm. (D) In vivo CTL strategy is illustrated. (E-F) Percentage of HBsAg-specific CTL activity in vivo is summarized. Bars are shown as mean ± SEM. *, P<0.05; **, P<0.01; ns, not significant.
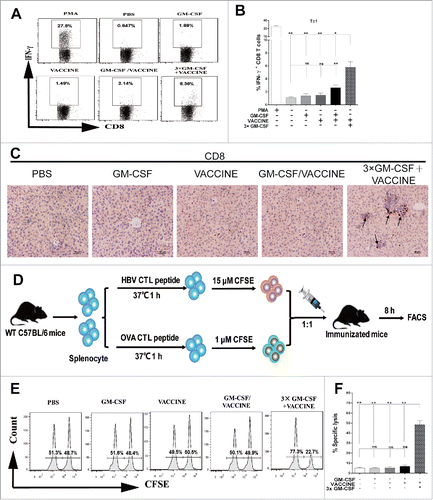
MoDC are continuously generated from the blood Ly6Chi monocytes in vivo;Citation32 therefore, we examined which subtype of monocyte assisted this process in HBV-infected mice. The monocytes in the peripheral blood were determined at 24, 48, and 72 h after the last immunization, wherein mice were immunized with 3 × GM-CSF+VACCINE twice at 2-week intervals. By 24 h, mice that received 3 × GM-CSF+VACCINE presented an approximately 3.5-fold higher frequency of CD11b+Ly6Chi monocytes in the blood than controls did( and ). Moreover, the frequency of CD11b+Ly6Clo monocytes did not change (Figs. S2A and S2B). CCR2 on Ly6Chi monocytes reportedly plays a guiding role and enables cells to traffic to inflammatory sites.Citation33 Correspondingly, an elevation in the serum level of MCP-1 (a CCR2 chemotactic ligand) was observed in the 3 × GM-CSF+VACCINE-treated group (). Echoing this finding, the 3 × GM-CSF+VACCINE significantly enhanced the expression of CCR2 on CD11b+Ly6Chi monocytes compared with other controls (Fig. S2C), indicating that MCP-1/CCR2 pairs may be essential for the Ly6Chi monocytes trafficking to the infected sites in immunized mice.
The 3 × GM-CSF+VACCINE induces robust HBV specific CD8+ T-cell response in vivo
A robust HBV-specific CD8+ T cell response plays a crucial role in eliminating virally infected hepatocytes.Citation34,35 We assessed the antigen specific cellular responses to the protocol shown in at 14 d after the last immunization. The 3 × GM-CSF+VACCINE induced significantly increased HBsAg-specific IFN-γ+CD8+ T cells in the periphery ( and ). Through IHC, a similar trend was found for CD8+ T cell infiltrates in the liver (). A HBV-specific CTL response is essential to clear the intracellular virus,Citation36,37 and was assessed as shown in . As shown in and , HBsAg-specific killing was almost 50% in the 3 × GM-CSF+VACCINE group, whereas the level of CTL response of mice that received control immunizations was less than 10%. Collectively, these results suggested that the 3 × GM-CSF+VACCINE could facilitate a robust HBsAg-specific CD8+ T-cell response in the AAV8–1.3HBV mouse model.
The 3 × GM-CSF+VACCINE enhances humoral response and induces HBV clearance in the mouse model
We next investigated the possible therapeutic effect of the 3 × GM-CSF+VACCINE in the AAV8–1.3HBV mouse model. The mice were immunized with the 3 × GM-CSF+VACCINE according to the designed immunization strategy (). Mice treated with PBS, GM-CSF or VACCINE alone and GM-CSF/VACCINE co-treated mice were used as controls. Fourteen days after the fourth vaccination, serum samples of immunized AAV8–1.8HBV mice were collected for HBeAg, HBsAg, HBV DNA and anti-HBsAg antibody tests. Liver tissues were collected for HBV DNA, IHC and pathology tests. The results demonstrated that serum HBeAg () and HBsAg () were cleared in the 3 × GM-CSF+VACCINE group, which was in marked contrast to the controls. Treatment with 3 × GM-CSF+VACCINE promoted substantial production of anti-HBsAg antibody, whereas no HBsAg-specific antibodies were detectable in controls (). To analyze whether 3 × GM-CSF+VACCINE influences IgG subclasses, we determined the levels of IgG1 and IgG2c at intervals during 24 weeks. After immunization with 3 × GM-CSF+VACCINE the AAV8–1.3HBV infected mice mounted strong IgG2c responses by weeks 9 and 12 and there were strong IgG1 responses on weeks 16, 20, and 24 (). To evaluate the long-term therapy with the 3 × GM-CSF+VACCINE for the HBV mouse model, we monitored the serum HBeAg and HBsAg levels for 24 weeks. No rebounds were found in either HBeAg or HBsAg in 3 × GM-CSF +VACCINE group ( and ). Notably, the serum level of ALT was about 2.5-fold higher in the 3 × GM-CSF+VACCINE group than in controls. Consistent with these findings, we observed a large number of lymphocytes trafficking in the 3 × GM-CSF+VACCINE-treated mice livers (). However, the elevation of ALT was transient and decreased to the baseline level after the termination of anti-HBV treatment (). Furthermore, serum and liver HBV DNA levels declined >2 log10IU/mL and >2 log10IU/g, respectively, in the 3 × GM-CSF +VACCINE group ( and ).
Figure 4. Responses of AAV8–1.3HBV infected mice to the complete 3 × GM-CSF +VACCINE regimen. 14 d after the fourth immunization, serum HBeAg (A) HBsAg (B), and HBsAb (C) were tested by ELISA. (D-G) At intervals up to 24 weeks, serum IgG subclasses (D), HBeAg (E), and HBsAg (F) and ALT (G) were measured by ELISA. 14 d after the fourth immunization, HBV DNA in livers (H) and serum samples (I) was analyzed with q-PCR. Dotted lines in (I) represent the assay limit of detection. (J-K) 14 d after the fourth immunization, liver-infiltrating lymphocytes were stained by H&E. Scale bar represents 50 μm (J). Liver sections were stained for HBcAg (brown staining) by IHC. Scale bar represents 50 μm (K). Symbols represent mean ± SEM. **, P<0.01; ns, not significant.
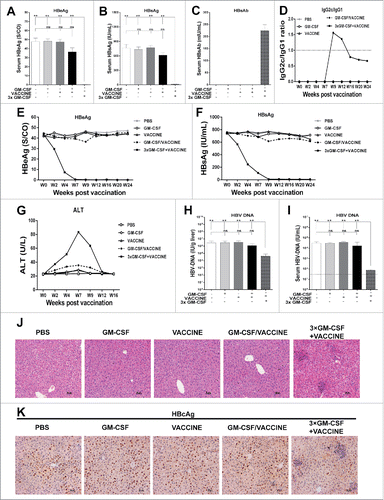
To further assess the therapeutic effects of this regimen, we analyzed the clearance of HBcAg-positive hepatocytes and lymphocyte infiltrations in the livers of immunized AAV8–1.3HBV mice. As shown in and , significant clearance of HBcAg-positive hepatocytes was observed after 3 × GM-CSF+VACCINE immunizations compared with controls. Taken together, these results highlighted a broader relevance for the robust anti-HBV responses induced by 3 × GM-CSF+VACCINE in the immune-tolerance model infected with AAV8–1.3HBV.
Depletion of Ly6Chi monocytes abolishes viral elimination of HBV model mice
Having demonstrated that the antigen specific CD8+T cell response was induced by CCR2+Ly6Chi monocytes, we determined whether the selective blockade of Ly6Chi monocytes would exert a detrimental effect on MoDC-mediated HBV clearance. We intraperitoneally injected INCB3344, a selective CCR2 antagonist,Citation38 into the HBV mouse model 1 h before the GM-CSF administration, and re-injected on days 2, 3, and 4. Mice treated with PBS or the DMSO vehicle were used as controls. Blood samples were collected, and peripheral blood mononuclear cells (PBMC) were analyzed 12 h after the fourth administration of INCB 3344. This pharmacological blockade of CCR2 prevented the generation of CD11b+Ly6Chi monocytes () and CD11b+CD11c+ DC ().
Figure 5. Blockage of Ly6Chi monocytes abrogates HBV clearance by the 3 × GM-CSF+VACCINE. The AAV8–1.3HBV infected mice were treated with CCR2 antagonist (INCB 3344, 30 mg/kg) by intraperitoneal injection one hour before GM-CSF, repeated at day 2, 3, and 4. The CD11b+Ly6Chi monocytes were measured 12 hours after the fourth INCB 3344 administration. (A) CD11b+Ly6Chi monocytes were quantified by percentage of total CD11b+ cells. (B) CD11b+ CD11c+ DC in blood were analyzed 48 hours after the fourth INCB 3344 administration and quantified by percentage of total CD11b+ cells. (C-F) IFN-γ+CD8+ T cells (C) in spleen, serum ALT (D), serum HBsAg (E), and serum HBV DNA (F) were measured on 14 d after the fourth vaccination. Dotted lines in (F) represent the assay limit of detection. (G) 14 d after the fourth vaccination, the liver sections HBcAg (brown staining) were stained by IHC. Scale bar represents 50 μm. Bars represent the mean ± SEM. *, P<0.05; **, P<0.01; ns, not significant.
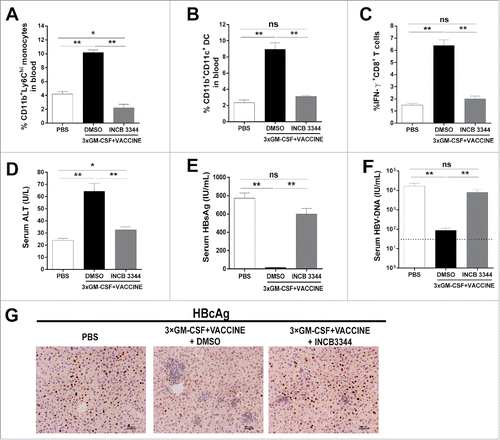
To assess the importance of CCR2+Ly6Chi monocytes, we examined whether the blockade of CCR2+Ly6Chi monocytes could abrogate the robust immune responses and abolish HBV clearance. The use of INCB3344 prevented the production of HBsAg specific IFN-γ+CD8+ T cells as well as the elevation of ALT level after 3 × GM-CSF+VACCINE treatments ( and ). Similar serum levels of viral load and HBsAg were found in PBS-and INCB3344-treated mice ( and ). As shown in , the blockade of CCR2 abrogated HBcAg-positive hepatocyte elimination. In summary, the induction of CCR2+Ly6Chi monocytes by 3 × GM-CSF+VACCINE immunization appeared to be crucial for the increased presence of CD11b+CD11c+DC and the subsequent induction of robust anti-HBV responses.
Discussion
Here we demonstrated that an enrichment of CD11b+Ly6Chi monocytes participated in the HBV-specific responses induced by multiple GM-CSF injections(once daily for 3 days) followed by a HBV vaccination at the same injected site, and resulted in viral clearance in the AAV8–1.3HBV-infected mice models. This strategy mainly promoted CD11b+Ly6Chi monocyte differentiation into CD11b+ CD11c+DC that elicited HBV specific CD8+ cellular responses and CTL in a CCR2-dependent manner. CCR2 dependence was implicated by CCR2-blockade that reduced CD11b+Ly6Chi monocytes and abrogated HBV clearance. Thus, the 3 × GM-CSF+VACCINE protocol could recruit more functionally relevant Ly6Chi monocytes and enhance DC functionally to boost HBsAg-specific CD8+ T cells and lead into the HBV clearance process in vivo.
Clinically, GM-CSF is the first cytokine documented to promote the differentiation of myeloid lineage cells into DC.Citation39 Given its effects, GM-CSF has been exploited as an adjuvant for cancer and virus vaccines in different animal models.Citation40,41 Our recent work demonstrated that the 3 × GM-CSF+VACCINE overcame the immune tolerance and elicited HBV-specific responses in the HBsAg transgenic mouse model. However, the underlying mechanism remained largely unknown. Murine monocytes comprise 2 populations, namely, the Ly6ChiCCR2+ inflammatory monocytes and the Ly6CloCCR2− circulating monocytes. Ly6Chi monocytes require the chemokine receptor CCR2 to migrate to inflammatory sites and up monocyte chemokine protein-1 (MCP-1) gradients.Citation42 The crucial role of Ly6Chi monocytes has been documented in preventing infectious diseases.Citation43,44 Here we identified Ly6ChiCCR2+ monocytes as a crucial effector to 3 × GM-CSF+VACCINE-induced HBV-specific response. Using a novel HBV mouse model, we demonstrated that 3 × GM-CSF+VACCINE significantly promoted the production of Ly6ChiCCR2+ monocytes and CD11b+CD11c+ DC. Moreover, we found that monocytes significantly developed into CD11c+DC when cultured in GM-CSF once for 3 d and then the 3 × GM-CSF-derived CD11c+ DC could markedly promote the proliferation of HBsAg specific CD8+ T cells. Interestingly, blockage of Ly6Chi monocytes could significantly reduce CD11b+CD11c+DC-induced HBV clearance. This finding implied that Ly6Chi monocytes may assist the increase in CD11b+CD11c+DC, and this enriches our understanding of the mode of action of the 3 × GM-CSF+VACCINE.
HBV-specific CD8+ T cells have been associated with the elimination of chronic HBV infections. Several inhibitory receptors, such as CTLA-4, PD-1, and LAG-3, contribute to the defective action of the anti-HBV CD8+ T cells in hepatitis B.Citation45 However, efforts to treat CHB infections with anti-PD-1 antibody achieved limited success,Citation46 indicating that these approaches may not be sufficient for reviving the function of the exhausted CD8+ T cells. Notably, in CHB patients, higher PD-1 expression was found on the intrahepatic virus-specific CTLsCitation47 and these were less susceptible to functional recovery by PD-1 depletionCitation48 when compared with circulating virus-specific CTLs. Therefore, freshly activating the naïve CD8+ T-cell repertoire becomes a more feasible approach. Interestingly, in this study, 3 × GM-CSF+VACCINE markedly induced the IFN-γ secretion of CD8+ T cells and augmented potent HBV-specific CTLs. The intrahepatic recruitment of HBV-specific CD8+ T-cell evidently leads to the killing of infected cells.Citation49 Correspondingly, we observed numerous recruited CD8+ T-cell infiltrated into the liver in the 3 × GM-CSF+VACCINE group. Furthermore, we noted that IL-12, a key cytokine in the initiation of effector T-cell development, was higher in the 3 × GM-CSF+VACCINE group than in other groups. These results demonstrated that the HBV clearance of AAV8–1.3HBV-infected mice could be attributed to the de novo HBV-specific CD8+ T cells arising after the 3 × GM-CSF+VACCINE treatments.
We believe that the current protocol might be more readily translatable to potential success in a clinical trial. The both commercial available GM-CSF and the alum-absorbed CHO-derived recombinant HBsAg vaccine have been approved to use with excellent safety profiles for more than 20 y. A pioneer clinical trial has demonstrated that the daily administration of GM-CSF to CHB patients significantly reduces HBV DNA levels in a safe and tolerable manner. However, the HBsAg seroconversion has not being observed.Citation50 Furthermore, HBsAg vaccine monotherapy for the CHB patients renders treatment has been demonstrated non-effective in a clinical study.Citation51 In this study, we observed that the 3 × GM-CSF+VACCINE regimen could promote HBsAg-specific CD8+ T cellular immune responses, HBsAb seroconversion and lead to the HBV clearance. Considering the safety and efficiency of this regimen, we expect that it may be readily acceptable in clinical practice. A recent study published by Bian and colleagues showed that preS1-containing vaccine could overcome immune tolerance more effectively than HBsAg vaccine did in HBV carrier mice.Citation26 Although there are not middle and/or large S protein vaccines licensed today, we would expect that the strategy of 3 × GM-CSF+preS vaccine could be very interest to test in human clinical trials if this vaccine becoming available.
This study has several limitations. First, how the hepatic immune populations respond to the 3 × GM-CSF+VACCINE regimen was not fully defined. Second, gene expression patterns associated with monocyte development into DC in response to the 3 × GM-CSF+VACCINE treatment were not defined. However, with the current pace of ongoing research, answers to these questions are expected soon.
In conclusion, the data presented here indicates that this GM-CSF-based HBV therapeutic vaccine promotes HBV-specific responses by enhancing Ly6Chi monocyte-derived CD11b+CD11c+DC in a CCR2-dependent manner in a HBV carrier mouse model. Accordingly, we suggest that the 3 × GM-CSF+VACCINE regimen presents a promising therapeutic strategy for CHB treatment.
Methods and materials
Experimental animals
Six- to eight-week-old C57BL/6 male mice were bought from Huafukang Laboratory Animal Co. Ltd (Beijing, China). All mice received 1 × 1010 TCID50 of AAV8–1.3HBV (genotype D, ayw, FivePlus, Beijing, China) virus through tail vein injection and were housed in environmentally-controlled conditions. All procedures were performed with the institutional animal use and care committee approval.
Vaccination
Alum-absorbed Chinese hamster ovary cell-derived recombinant HBsAg (VACCINE, thereafter) and recombinant human GM-CSF were kindly gifted by the Jingtan Biotech Corp. of China North Pharmaceutical Group (Shijiazhang, China). The AAV8–1.3HBV infected mice were treated subcutaneously with 10 μg of GM-CSF once per day for 3 days, then subcutaneously injected with 1 μg of VACCINE at the same site. All mice started treatment on day 0 and three booster 3-day courses were started on days 14, 28, 49. Mice treated with PBS, GM-CSF or VACCINE alone and GM-CSF/VACCINE were co-treated and used as controls.
Cultivating monocyte-derived DC
Blood CD11b+Ly6G− monocytes were isolated from male wild-type C57BL/6 mice (n = 50) by Easysep™ mouse monocyte isolation kit (STEMCELL, Canada). These cells were cultured in media supplied with recombinant mouse GM-CSF (50 ng/mL, PeproTech) once per day in a humidified incubators with 5% CO2. Monocytes were plated at 2 × 105/mL in 24-well cell culture plates. After 72 hours, the cells were collected and analyzed by FACS.
In vitro MoDC and T cell co-culture
Conventional splenic CD8+ T cells were purified from AAV8–1.3HBV infected mice by a MajoSort kit (Biolegend) and labeled with carboxyfluorescein succinimidyl ester (CFSE). 1 × GM-CSF-derived or 3 × GM-CSF-derived MoDC were incubated with10 μg/mL of HBsAg (Guikang, Shanghai, China) for 24 hours before mixed with the purified CD8+ T cells (2 × 105 cells/well) at a T:MoDC ratio of 10:1. After 3-day co-culture, cells were analyzed by FACS.
In vivo cytotoxic lysis assay
OVA-specific CTL epitope OVA257–264 (SIINFWKL) and HBsAg-specific CTL epitope S208–215 (ILSPFLPL; H-2b-restricted) were synthesized (Sangon, Shanghai, China). In vivo cytotoxic lysis assay was conducted as described previously.Citation18 Briefly, splenocytes from naïve C57BL/6 donor mice were labeled with 15 μM of CFSE and pulsed with 1 μg/mL of S208–215 (defined as CFSEhigh target cells). An equal fraction of splenocytes were labeled with 1 μM of CFSE and pulsed with 1 μg/mL of OVA257–264 (defined as CFSElow target cells) as a non-HBV target control. A mixture of CFSEhigh and CFSElow cells at a 1:1 ratio was adoptively transferred intravenously into immunized recipients at 2 × 107 cells per mouse on the 14th day after the fourth vaccination. Eight hours later, the splenocytes were isolated from the recipients and CFSE fluorescence intensities were analyzed.
Blockage of Ly6Chi monocyte with INCB 3344
The AAV8–1.3HBV infected mice were randomly divided into 3 groups (n = 5). PBS, INCB 3344 (30 mg/kg per day, MedChem Express, USA), or vehicle (10% dimethylsulfoxide, DMSO) was i.p. injected at 1 hour before GM-CSF inoculation. PBS, INCB 3344 or vehicle was injected again on days 2, 3, and 4. Ly6ChiCCR2+ monocytes were analyzed 24 hours later by FACS.
Biochemical, serological and HBV DNA analysis
Serum alanine aminotransferase activity (ALT) was determined by ALT kits (BioSino, Beijing, China). Serum HBsAg, HBsAb and HBeAg were measured by ELISA kits (Kehua, Shanghai, China). Serum IL-12 and MCP-1 were analyzed by ELISA kits (eBioscience, USA). Liver and serum HBV DNA was analyzed by real-time quantitative PCR kit (Sansure, Hunan, China).
Histology and immunohistochemistry (IHC) analysis
On day 14 after the fourth immunization, dissected liver samples were collected and fixed in 4% paraformaldehyde for 3 d before being embedded in paraffin wax, and cut into 5 to 10 μm thick slices. The liver sections were stained with hematoxylin-eosin for histology analysis and incubated with polyclonal rabbit antibody for IHC analysis.
Flow cytometry detection
Fluorescent labeled antibodies used for flow cytometric analysis were: CD8 (53–6.7), F4/80 (BM8), CD80 (16–10A1), MHC-I (AF6.88.5.5.3), PDCA-1 (eBio927), MHC-II (M5/114.15.2) from eBioscience; and CD11b (M1/70), Ly6C (HK1.4), CCR2 (SA203G11), IFN-γ (XMG1.2) from Biolegend; and CD11c (HL3) from BD. In cytokine detection, PMA (100 ng/mL) and ionomycin (1 μg/mL) were used as positive control stimulants. An LSRFortessa (BD Biosciences) was used for flow cytometry analyses.
Statistical analysis
Statistical analysis was performed using Student's t-test and P values less than 0.05 were deemed significant.
Abbreviations
ALT | = | alanine transaminase |
cccDNA | = | covalently closed circular DNA |
CHB | = | chronic hepatitis B |
DC | = | dendritic cells |
GM-CSF | = | granulocyte-macrophage colony-stimulating factor |
HBeAg | = | hepatitis B e antigen |
HBsAg | = | hepatitis B surface antigen |
HBV | = | hepatitis B virus |
Disclosure of potential conflict of interest
The authors declare having no potential conflicts of interest.
2016HV0580R1-s02.docx
Download MS Word (544.6 KB)Acknowledgments
We thank Ms. Ying Wang of Jingtan Biotech Corp. for providing valuable human recombinant HBV vaccines and human GM-CSF. We are also grateful to Mr. Wencong Yu, Ms. Xiaoyu Zhou, Ms. Shijie Zhang and Ms. Yue He for their helpful discussions on this work. We also wish to thank Dr. Douglas Lowrie for his careful proofread of this manuscript.
Funding
This work is supported by grants from the National Science and Technology Major Program of Infectious Diseases (2012ZX10002002004–001, 2012ZX10004701 and 2013ZX10002001) and Natural Science Foundation of China (31430027 and 81672016) to Dr. B. Wang.
References
- WHO. Guidelines for the prevention, care and treatment of persons with chronic hepatitis B infection. Geneva, World Health Organization 2015.
- Seo Y, Yano Y. Short- and long-term outcome of interferon therapy for chronic hepatitis B infection. World J Gastroentero 2014; 20:13284-92; PMID:25309065; https://doi.org/10.3748/wjg.v20.i37.13284
- Fung J, Lai CL, Seto WK, Yuen MF. Nucleoside/nucleotide analogues in the treatment of chronic hepatitis B. J Antimicrob Chemother 2011; 66:2715-25; PMID:21965435; https://doi.org/10.1093/jac/dkr388
- Tseng TC, Kao JH. Treating Immune-tolerant Hepatitis B. J Viral Hepat 2015; 22:77-84; PMID:25424771; https://doi.org/10.1111/jvh.12370
- Wen Y, Shi Y. Alum: an old dog with new tricks. Emerging microbes & infections 2016; 5:e25; PMID:27004761; https://doi.org/10.1038/emi.2016.40
- Parvez MK, Arbab AH, Al-Dosari MS, Al-Rehaily AJ. Antiviral natural products against chronic Hepatitis B: Recent developments. Curr Pharm Des 2016; 22:286-93; PMID:26561057
- Hu W, Huang H, Zhang TY, Mao YY, Wang XJ, Wang SQ. CpG oligodeoxynucleotide inhibits HBV replication in a hydrodynamic injection murine model. Antivir Ther 2015; 20:289-95; PMID:25279542; https://doi.org/10.3851/IMP2870
- Zhang W, Du X, Zhao G, Jin H, Kang Y, Xiao C, Liu M, Wang B. Levamisole is a potential facilitator for the activation of Th1 responses of the subunit HBV vaccination. Vaccine 2009; 27:4938-46; PMID:19549606; https://doi.org/10.1016/j.vaccine.2009.06.012
- Lin C, Zhu J, Zheng Y, Chen Y, Wu Z, Chong Y, Gao Z. Effect of GM-CSF in combination with hepatitis B vaccine on revacination of healthy adult non-responders. J Infect 2010; 60:264-70; PMID:20138189; https://doi.org/10.1016/j.jinf.2010.01.011
- Yu TW, Chueh HY, Tsai CC, Lin CT, Qiu JT. Novel GM-CSF-based vaccines: One small step in GM-CSF gene optimization, one giant leap for human vaccines. Human vaccines & immunotherapeutics 2016; 12:3020-8; PMID:27560197; https://doi.org/10.1080/21645515.2016.1221551
- Nemunaitis J. Vaccines in cancer: GVAX, a GM-CSF gene vaccine. Expert Rev Vaccines 2005; 4:259-74; PMID:16026242; https://doi.org/10.1586/14760584.4.3.259
- Hellerstein M, Xu Y, Marino T, Lu S, Yi H, Wright ER, Robinson HL. Co-expression of HIV-1 virus-like particles and granulocyte-macrophage colony stimulating factor by GEO-D03 DNA vaccine. Human vaccines & immunotherapeutics 2012; 8:1654-8; PMID:23111169; https://doi.org/10.4161/hv.21978
- Clive KS, Tyler JA, Clifton GT, Holmes JP, Mittendorf EA, Ponniah S, Peoples GE. Use of GM-CSF as an adjuvant with cancer vaccines: beneficial or detrimental?. Expert Rev Vaccines 2010; 9:519-25; PMID:20450326; https://doi.org/10.1586/erv.10.40
- Spearman P, Kalams S, Elizaga M, Metch B, Chiu YL, Allen M, Weinhold KJ, Ferrari G, Parker SD, McElrath MJ, et al. Safety and immunogenicity of a CTL multiepitope peptide vaccine for HIV with or without GM-CSF in a phase I trial. Vaccine 2009; 27:243-9; PMID:18996425; https://doi.org/10.1016/j.vaccine.2008.10.051
- Pilla L, Patuzzo R, Rivoltini L, Maio M, Pennacchioli E, Lamaj E, Maurichi A, Massarut S, Marchianò A, Santantonio C, et al. A phase II trial of vaccination with autologous, tumor-derived heat-shock protein peptide complexes Gp96, in combination with GM-CSF and interferon-alpha in metastatic melanoma patients. Cancer immunology, immunotherapy: CII 2006; 55:958-68; PMID:16215718; https://doi.org/10.1007/s00262-005-0084-8
- van de Laar L, Coffer PJ, Woltman AM. Regulation of dendritic cell development by GM-CSF: molecular control and implications for immune homeostasis and therapy. Blood 2012; 119:3383-93; PMID:22323450; https://doi.org/10.1182/blood-2011-11-370130
- Barone M, Maiorano E, Ladisa R, Cuomo R, Pece A, Berloco P, Caruso ML, Valentini AM, Iolascon A, Francavilla A, et al. Influence of ursodeoxycholate-enriched diet on liver tumor growth in HBV transgenic mice. Hepatology 2003; 37:880-6; PMID:12668981; https://doi.org/10.1053/jhep.2003.50175
- Wang X, Dong A, Xiao J, Zhou X, Mi H, Xu H, Zhang J, Wang B. Overcoming HBV immune tolerance to eliminate HBsAg-positive hepatocytes via pre-administration of GM-CSF as a novel adjuvant for a hepatitis B vaccine in HBV transgenic mice. Cellular & molecular immunology 2016; 13:850-61; PMID:26166767; https://doi.org/10.1038/cmi.2015.64
- Dembek C, Protzer U. Mouse models for therapeutic vaccination against hepatitis B virus. Med Microbiol Immunol 2015; 204:95-102; PMID:25523197; https://doi.org/10.1007/s00430-014-0378-6
- Bissig KD, Wieland SF, Tran P, Isogawa M, Le TT, Chisari FV, Verma IM. Human liver chimeric mice provide a model for hepatitis B and C virus infection and treatment. J Clin Invest 2010; 120:924-30; PMID:20179355; https://doi.org/10.1172/JCI40094
- Yang PL, Althage A, Chung J, Chisari FV. Hydrodynamic injection of viral DNA: a mouse model of acute hepatitis B virus infection. Proc Natl Acad Sci U S A 2002; 99:13825-30; PMID:12374864; https://doi.org/10.1073/pnas.202398599
- Yang D, Liu L, Zhu D, Peng H, Su L, Fu YX, Zhang L. A mouse model for HBV immunotolerance and immunotherapy. Cellular & molecular immunology 2014; 11:71-8; PMID:24076617; https://doi.org/10.1038/cmi.2013.43
- Akbar SK, Onji M. Hepatitis B virus (HBV)-transgenic mice as an investigative tool to study immunopathology during HBV infection. Int J Exp Pathol 1998; 79:279-91; PMID:10193311
- Chayama K, Hayes CN, Hiraga N, Abe H, Tsuge M, Imamura M. Animal model for study of human hepatitis viruses. J Gastroenterol Hepatol 2011; 26:13-8; PMID:21175788; https://doi.org/10.1111/j.1440-1746.2010.06470.x
- Huang LR, Gabel YA, Graf S, Arzberger S, Kurts C, Heikenwalder M, Knolle PA, Protzer U. Transfer of HBV genomes using low doses of adenovirus vectors leads to persistent infection in immune competent mice. Gastroenterology 2012; 142:1447-50e3; PMID:22426294; https://doi.org/10.1053/j.gastro.2012.03.006
- Bian Y, Zhang Z, Sun Z, Zhao J, Zhu D, Wang Y, Fu S, Guo J, Liu L, Su L, et al. Vaccines targeting PreS1 domain overcome immune tolerance in HBV carrier mice. Hepatology. 2017; [epub ahead of print]; PMID:28445927; https://doi.org/10.1002/hep.29239.
- European Association for the Study of the Liver. Lampertico P, Agarwal K, Berg T, Buti M, Janssen HLA, Papatheodoridis G, Zoulim F, Tacke F. EASL 2017 Clinical Practice Guidelines on the management of hepatitis B virus infection. J Hepatol 2017; 67:370-398; PMID:28427875; https://doi.org/10.1016/j.jhep.2017.03.021
- Singh-Jasuja H, Thiolat A, Ribon M, Boissier MC, Bessis N, Rammensee HG, Decker P. The mouse dendritic cell marker CD11c is down-regulated upon cell activation through Toll-like receptor triggering. Immunobiology 2013; 218:28-39; PMID:22445076; https://doi.org/10.1016/j.imbio.2012.01.021
- Mazzone A, Ricevuti G. Leukocyte CD11/CD18 integrins: biological and clinical relevance. Haematologica 1995; 80:161-75; PMID:7628754
- Sun H, Bi L, Zhou J, Zhou D, Liu Y, Jin G, Yan W. Modulation of the function of dendritic cells in adolescents with chronic HBV infection by IFN-lambda1. International journal of clinical and experimental pathology 2015; 8:1743-51; PMID:25973063
- Ko HJ, Brady JL, Ryg-Cornejo V, Hansen DS, Vremec D, Shortman K, Zhan Y, Lew AM. GM-CSF-responsive monocyte-derived dendritic cells are pivotal in Th17 pathogenesis. J Immunol 2014; 192:2202-9; PMID:24489100; https://doi.org/10.4049/jimmunol.1302040
- Tamoutounour S, Guilliams M, Montanana Sanchis F, Liu H, Terhorst D, Malosse C, Pollet E, Ardouin L, Luche H, Sanchez C, et al. Origins and functional specialization of macrophages and of conventional and monocyte-derived dendritic cells in mouse skin. Immunity 2013; 39:925-38; PMID:24184057; https://doi.org/10.1016/j.immuni.2013.10.004
- Neal LM, Knoll LJ. Toxoplasma gondii profilin promotes recruitment of Ly6Chi CCR2+ inflammatory monocytes that can confer resistance to bacterial infection. PLoS Pathog 2014; 10:e1004203; PMID:24945711; https://doi.org/10.1371/journal.ppat.1004203
- Iannacone M. Hepatic effector CD8(+) T-cell dynamics. Cellular & molecular immunology 2015; 12:269-72; PMID:25242274; https://doi.org/10.1038/cmi.2014.78
- Tang TJ, de Man RA, Kusters JG, Kwekkeboom J, Hop WC, van der Molen RG, Schalm SW, Janssen HL. Intrahepatic CD8 T-lymphocytes and HBV core expression in relation to response to antiviral therapy for chronic hepatitis B patients. J Med Virol 2004; 72:215-22; PMID:14695662; https://doi.org/10.1002/jmv.10565
- Iannacone M, Sitia G, Ruggeri ZM, Guidotti LG. HBV pathogenesis in animal models: recent advances on the role of platelets. J Hepatol 2007; 46:719-26; PMID:17316876; https://doi.org/10.1016/j.jhep.2007.01.007
- Guidotti LG, Ishikawa T, Hobbs MV, Matzke B, Schreiber R, Chisari FV. Intracellular inactivation of the hepatitis B virus by cytotoxic T lymphocytes. Immunity 1996; 4:25-36; PMID:8574849
- Chu HX, Kim HA, Lee S, Broughton BRS, Drummond GR, Sobey CG. Evidence of CCR2-independent transmigration of Ly6Chi monocytes into the brain after permanent cerebral ischemia in mice. Brain Res 2016; 1637:118-27; https://doi.org/10.1016/j.brainres.2016.02.030
- Zhan Y, Vega-Ramos J, Carrington EM, Villadangos JA, Lew AM, Xu Y. The inflammatory cytokine, GM-CSF, alters the developmental outcome of murine dendritic cells. Eur J Immunol 2012; 42:2889-900; PMID:22806691; https://doi.org/10.1002/eji.201242477
- Yan WL, Shen KY, Tien CY, Chen YA, Liu SJ. Recent progress in GM-CSF-based cancer immunotherapy. Immunotherapy 2017; 9:347-60; PMID:28303764; https://doi.org/10.2217/imt-2016-0141
- Choi HJ, Gwon YD, Jang Y, Cho Y, Heo YK, Lee HJ, Kim KC, Choi J, Lee JB, Kim YB. Effect of AcHERV-GmCSF as an Influenza Virus Vaccine Adjuvant. PLoS One 2015; 10:e0129761; PMID:26090848; https://doi.org/10.1371/journal.pone.0129761
- Shi C, Pamer EG. Monocyte recruitment during infection and inflammation. Nat Rev Immunol 2011; 11:762-74; PMID:21984070; https://doi.org/10.1038/nri3070
- Biswas A, Bruder D, Wolf SA, Jeron A, Mack M, Heimesaat MM, Dunay IR. Ly6C(high) monocytes control cerebral toxoplasmosis. J Immunol 2015; 194:3223-35; PMID:25710908; https://doi.org/10.4049/jimmunol.1402037
- Ngo LY, Kasahara S, Kumasaka DK, Knoblaugh SE, Jhingran A, Hohl TM. Inflammatory monocytes mediate early and organ-specific innate defense during systemic candidiasis. J Infect Dis 2014; 209:109-19; PMID:23922372; https://doi.org/10.1093/infdis/jit413
- Bertoletti A, Ferrari C. Adaptive immunity in HBV infection. J Hepatol 2016; 64:S71-83; PMID:27084039; https://doi.org/10.1016/j.jhep.2016.01.026
- Bengsch B, Martin B, Thimme R. Restoration of HBV-specific CD8+ T cell function by PD-1 blockade in inactive carrier patients is linked to T cell differentiation. J Hepatol 2014; 61:1212-9; PMID:25016223; https://doi.org/10.1016/j.jhep.2014.07.005
- Golden-Mason L, Palmer B, Klarquist J, Mengshol JA, Castelblanco N, Rosen HR. Upregulation of PD-1 expression on circulating and intrahepatic hepatitis C virus-specific CD8+ T cells associated with reversible immune dysfunction. J Virol 2007; 81:9249-58; PMID:17567698; https://doi.org/10.1128/JVI.00409-07
- Nakamoto N, Cho H, Shaked A, Olthoff K, Valiga ME, Kaminski M, Gostick E, Price DA, Freeman GJ, Wherry EJ, et al. Synergistic reversal of intrahepatic HCV-specific CD8 T cell exhaustion by combined PD-1/CTLA-4 blockade. PLoS Pathog 2009; 5:e1000313; PMID:19247441; https://doi.org/10.1371/journal.ppat.1000313
- Ferrari C. HBV and the immune response. Liver international: official journal of the International Association for the Study of the Liver 2015; 35(Suppl 1):121-8; PMID:25529097; https://doi.org/10.1111/liv.12749
- Martin J, Bosch O, Moraleda G, Bartolome J, Quiroga JA, Carreno V. Pilot study of recombinant human granulocyte-macrophage colony-stimulating factor in the treatment of chronic hepatitis B. Hepatology 1993; 18:775-80; PMID:8406350
- Lok AS, Pan CQ, Han SH, Trinh HN, Fessel WJ, Rodell T, Massetto B, Lin L, Gaggar A, Subramanian GM, et al. Randomized phase II study of GS-4774 as a therapeutic vaccine in virally suppressed patients with chronic hepatitis B. J Hepatol 2016; 65:509-16; PMID:27210427; https://doi.org/10.1016/j.jhep.2016.05.016