ABSTRACT
Lassa virus (LASV) is an ambisense RNA virus in the Arenaviridae family and is the etiological agent of Lassa fever, a severe hemorrhagic disease endemic to West and Central Africa.Citation1,2 There are no US Food and Drug Administration (FDA)-licensed vaccines available to prevent Lassa fever.Citation1,2 in our previous studies, we developed a gene-optimized DNA vaccine that encodes the glycoprotein precursor gene of LASV (Josiah strain) and demonstrated that 3 vaccinations accompanied by dermal electroporation protected guinea pigs from LASV-associated illness and death. Here, we describe an initial efficacy experiment in cynomolgus macaque nonhuman primates (NHPs) in which we followed an identical 3-dose vaccine schedule that was successful in guinea pigs, and a follow-on experiment in which we used an accelerated vaccination strategy consisting of 2 administrations, spaced 4 weeks apart. In both studies, all of the LASV DNA-vaccinated NHPs survived challenge and none of them had measureable, sustained viremia or displayed weight loss or other disease signs post-exposure. Three of 10 mock-vaccinates survived exposure to LASV, but all of them became acutely ill post-exposure and remained chronically ill to the study end point (45 d post-exposure). Two of the 3 survivors experienced sensorineural hearing loss (described elsewhere). These results clearly demonstrate that the LASV DNA vaccine combined with dermal electroporation is a highly effective candidate for eventual use in humans.
KEYWORDS:
Introduction
Viruses in the Arenaviridae family are enveloped, primarily rodent-borne viruses, with some newly discovered members that have been isolated from reptiles.Citation3-5 The rodent-borne arenaviruses include highly pathogenic New World members, such as Machupo and Junin virus, endemic to South America, and Old World members, such as Lassa virus (LASV), endemic to West Africa.Citation1,2 LASV has been classified as a Category A biological threat agent by the US Centers for Disease Control and Prevention. The arenavirus genome consists of 2 single-stranded RNA segments that encode a total of 4 viral proteins, including the viral polymerase and zinc (Z) binding proteins on the Large (L) segment, and the nucleoprotein (NP) and glycoprotein precursor (GPC) structural proteins on the small (S) segment. The GPC gene product is post-translationally cleaved by a host protease, thus expanding the viral protein repertoire to include glycoproteins (GP) 1 and 2.Citation1,6 The RNA genomes of arenaviruses use a unique “ambisense” protein coding strategy, meaning that they have the capability of encoding their proteins from either end of the RNA segment in a virus-complementary or virus-sense manner.Citation1,7 While considerable sequence diversity exists within and between strains of LASV, the predicted glycosylation sites on the GPC gene and the GP1/GP2 cleavage site appears to be conserved.Citation8
Infection with LASV can result in Lassa fever, an acute disease characterized by fever, malaise, and exudative pharyngitis with progression to mucosal bleeding, edema and coagulopathy. According to the CDC, approximately 100,000 to 300,000 cases of Lassa fever occur per year in the endemic regions of Central and West Africa, and the disease accounts for approximately 10–16% of all hospital admissions per year.Citation9 The presumed case fatality rate is fairly low, causing approximately 5,000 deaths per year among identified cases, but a much higher case fatality rate of 55% was postulated based on serologic surveys showing that LASV-specific IgM, which has historically been associated with acute disease, can persist in LASV survivors for at least one year and; therefore may not be a reliable indicator of recent infection.Citation10 LASV infection not only causes acute disease, but also leads to serious and long-lasting sequelae in about 30% of survivors to include unilateral or bilateral sudden-onset sensorineural hearing loss.Citation11-16 At present, there are no vaccines or antiviral drugs approved by the FDA for treating infection with LASV. While ribavirin is approved for use in treating hemorrhagic fever caused by arenaviruses under the compassionate use provisions for investigational new drugs, it is not widely available and must be donated by the manufacturer. The efficacy of this therapeutic against Lassa fever is also questionable and has not been shown to reduce post-recovery deafness.Citation17,18 There have been some promising reports of small molecule inhibitors with efficacy against LASV in cell culture and in small animal models,Citation19-24 but as yet, there are no therapeutics available for use in humans, even in a compassionate use indication. There is clearly a need to develop effective preventative and therapeutic medical countermeasures to respond to or prevent outbreaks of Lassa fever in Africa and to counter potential acts of bioterrorism.
Animal models for Lassa fever include guinea pigs and NHP. Experimental LASV (Josiah strain) infection of cynomolgus macaques typically results first in a hemorrhagic phase in which NHP succumb approximately 14 d post-exposure (range 11–18 days); and a neurologic phase in which NHP develop neurological signs including tremors, ataxia and seizures approximately 21 d post-exposure (range 19–23).Citation25 and USAMRIID unpublished data In our experience, NHP that develop neurological signs seldom recover fully from disease; they either succumb as a consequence of or require euthanasia due to seizures, or continue to experience neurological signs to the study end point. Rarely, NHP will survive infection without experiencing severe signs and fully recover by the study end point.
Our laboratories are engaged in development of DNA-based strategies against viruses of biodefense interest. DNA-based vaccines have several advantages over other methods of vaccination. They are generally regarded as safe, they are relatively simple to produce, and thus can be manufactured quickly to best respond to need. In addition, DNA plasmid vaccines are relatively stable and don't require rigorous cold-chain maintenance, making them an ideal strategy for deployment environments.Citation26,27 DNA vaccines have also been traditionally considered to be good stimulators of cell-mediated immunity, thus are well suited for LASV studies because cellular immunity appears to be of greater importance than humoral immunity for protection against LASV.Citation28-32 For our LASV vaccine design efforts, we chose the GPC gene of LASV as our target due to the fact that there are important conserved regions of this gene (as discussed earlier), and that post-translational cleavage of GPC into GP1 and GP2 in the host could also potentially allow for the generation of GPC, GP1 and GP2-specific immune responses from a single plasmid. Using this vaccine, we demonstrated the LASV (Josiah strain) codon-optimized GPC plasmid when delivered by intradermal (ID) electroporation (EP), completely protected guinea pigs against LASV-associated viremia, disease and death.Citation33 Here, we describe the outcomes of experiments to describe the efficacy of a primate-optimized version of this vaccine in an NHP model, administered via a novel dermal electroporation device.
Results
Protective efficacy of 3-doses vs 2-doses of the LASV DNA vaccine
Cynomolgus macaques were vaccinated by ID injection of the vaccine followed by ID-EP as described previously.Citation34,35 No inflammation at the vaccination sites or other adverse events were observed. Two separate studies were conducted to compare 3 or 2 vaccinations at 4-week intervals. Two of the 4 mock-vaccinated NHP in the 3-dose study and 5 of the 6 mock-vaccinated NHP in the 2-dose study infected with LASV succumbed during the hemorrhagic phase from 10–17 d post-exposure. The remaining mock-vaccinated NHP became critically ill, but survived the hemorrhagic phase (). Two of these survivors never fully recovered and developed chronic neurological illness characterized by reduced appetite, tremors, and ataxia. The third survivor partially recovered, retaining some neurological deficit which persisted until the study end point, 45 d post-exposure. Two of the 3 survivors developed hearing loss (Cashman et. al, Citationmanuscript in review). In contrast, all LASV-GPC DNA-vaccinated NHP, regardless of dose group, showed no signs of infection after exposure and survived to the study end point (). Morbidity scores, which are subjective measurements of disease severity based on observable signs, were assigned to each NHP daily, starting from the day of virus exposure. Likewise, final morbidity scores were assigned at the study end point (). A score of zero indicated the macaque was well; showing no outward signs of disease; whereas, a score of 10 indicated the NHP was severely ill and met euthanasia criteria. The LASV DNA-vaccinated NHP remained at zero on the morbidity scale for the duration of the study. Mock-vaccinated NHP became severely ill, as indicated by the increasing morbidity scores starting at approximately 5 d post-exposure. The 3 mock vaccinated NHP that survived the acute phase of disease had observable disease signs, thus morbidity scores were recorded for these NHP until the study end point ().
Figure 1. Survival curve, serum viremia, morbidity scores, and body weight and temperature changes for NHPs post-exposure. A) All of the DNA-vaccinated NHPs survived, while 2 of the 4 mock-vaccinated NHPs survived to the study end point. B) Overall morbidity scores assigned daily for each NHP as a subjective measure of observed responsiveness and presence or absence of disease signs; C) Serum viremia post-exposure as measured by plaque assay and expressed as the Log10 pfu/ml per blood sample collection day; D) Changes in body weight expressed as a percentage change from baseline weights for each NHP, then averaged per group; and E) Changes in body temperature as measured by rectal and/or temperature transponder chips and expressed as a percentage change from baseline temperatures for each NHP, then averaged per group.
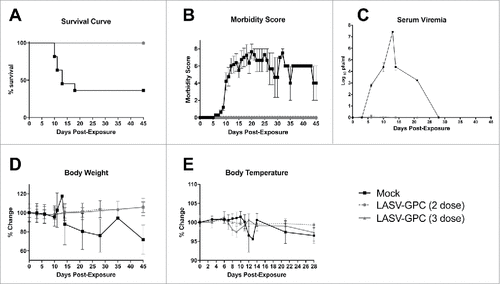
To identify virus levels in the blood post-exposure, serum viremia was measured using a standard plaque assay as described. All of the mock-vaccinated NHP had measureable serum viremias starting at day 3, and peaking between days 12 and 14, which corresponded with the development of fever (, ). Serum viremias were undetectable by plaque assay in the 3 surviving mock vaccinated NHP by day 28 (). In contrast, neither serum viremia nor fever was observed in any of the LASV DNA-vaccinated NHP at any timepoint (). One NHP had one plaque present at the 1 × 10−1 dilution at day 6 which falls below the limit of quantitation for the assay and is considered a false positive. Body weight was measured at each phlebotomy timepoint which revealed severe weight loss in the mock-vaccinated NHP beginning approximately 10 d post-exposure and continuing to the study end point in 2 of 3 mock-vaccinated survivors (). Elevated body temperatures for the mock vaccinated NHP were also observed when compared with the LASV-GPC vaccinated NHP (). Body temperature measurements were collected more frequently for the 3-dose study because scannable microchip transponders were used; whereas, only rectal temperatures were obtained during phlebotomy time points in the 2-dose study.
LASV-specific neutralizing antibody responses pre- and post-exposure
The neutralizing antibody response measured in NHP that received the LASV-GPC vaccine before exposure to LASV were modest, especially in those that received 2 doses of the vaccine (). The mock-vaccinated NHP did not develop neutralizing antibodies above background levels obtained before exposure. Following exposure to LASV, neutralizing antibody levels increased in the LASV DNA-vaccinated NHP, peaking approximately 21 d post exposure, then declining slightly at the study end point (). Neutralizing antibodies also were observed in the surviving mock-vaccinated NHP after exposure, but developed more slowly, becoming detectable by day 14, and continuing to rise to the study end point. No neutralizing antibodies were detected in the mock-vaccinated NHP that succumbed in the hemorrhagic phase. Since the mock-vaccinated survivors were chronically ill at the study end point, the presence of high levels of LASV-specific antibodies measured both by ELISA (data not shown) and PRNT was apparently insufficient to prevent or resolve the disease.
Blood hematology and chemistry measurements
Hematology and blood chemistry measurements were obtained on days 0, 3, 6, 10, 14, 21, 28, 35 and 45 post-exposure. There were clear differences observed between the LASV DNA-vaccinated and mock-vaccinated NHP with regard to cell counts post-exposure (). Initially, white blood cells (WBC) increased in the LASV DNA-vaccinated and decreased in the mock-vaccinated NHP early post-exposure (), followed by a rapid increase late in infection in the mock-vaccinated survivors compared with the LASV DNA-vaccinated NHP, which maintained stable WBC counts to the study end point. Neutrophil and eosinophil populations demonstrated similar trends, but neutrophil counts increased equally for all NHP at day 3 post-exposure, before differentiating by day 6 ( and , respectively). Lymphocyte and monocyte populations increased rapidly after exposure in the LASV DNA-vaccinated NHP, stabilizing by day 21, compared with mock vaccinated NHP ( and , respectively). Monocytes were unchanged in the mock vaccinated NHP early, but then increased between days 10 and 28 (). In contrast, lymphocyte numbers dropped steeply immediately after exposure until day 6 (). Surviving mock-vaccinated NHP returned lymphocyte numbers to baseline levels by the end of the study (). Both hemoglobin () and hematocrit () increased before becoming stable in the LASV DNA-vaccinated NHP and decreased consistently in the mock-vaccinated NHP. These measurements remained low until the study end point however, the mock-vaccinated NHP had normal red blood cell counts throughout the study (data not shown). Platelets increased in the LASV DNA-vaccinated NHP but dropped precipitously in the mock-vaccinates, which likely contributed to the hemorrhagic complications experienced by those who succumbed during that period (). The mock-vaccinated survivors eventually began to return platelet counts to baseline levels by day 28, and had elevated platelet counts at the study end point.
Figure 3. Changes in selected complete blood count counts after LASV exposure. A) White blood cells; B) Neutrophils; C) Lymphocytes; D) Monocytes; E) Eosinophils; F) Basophils; G) Hemoglobin; H) Hematocrit; I) Platelets.


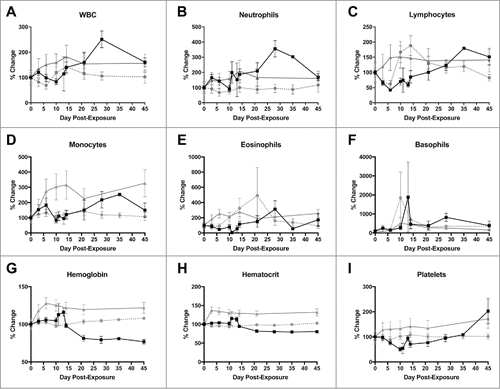
Differences in blood chemistries were observed, and are consistent with other reports for acute, hemorrhagic phase LASV disease in primates post-exposure.Citation25 Most blood chemistry parameters remained stable at near baseline levels for the LASV DNA-vaccinated NHP for the duration of the study ). In the mock-vaccinated NHP, creatinine, alanine aminotransferase (ALT) and aspartate aminotransferase (AST) became elevated during the hemorrhagic phase of disease , and ), indicating hepatic and pancreatic involvement. However, only alkaline phosphatase (ALP) remained elevated in the surviving NHP at the end of the study, which may reflect the chronically ill state of these survivors (). Albumin levels, despite total protein becoming highly elevated in mock-vaccinated NHP that survived the acute phase (), dropped precipitously and did not return to baseline by the end of study (), possibly inhibited by an inverse hypergammaglobulinemia in these animals.
Figure 4. Changes in selected blood chemistry values after LASV exposure. A) Blood urea nitrogen; B) Creatinine; C) Total bilirubin; D) Albumin; E) Total protein; F) Gamma-glutamyltransferase; G) Alanine aminotransferase (ALT); H) Aspartate aminotransferase (AST); and Alkaline phosphatase (ALP).


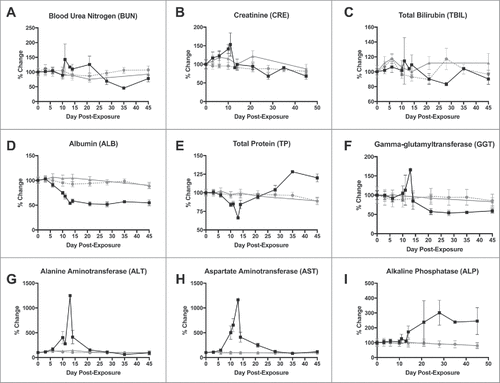
Pathologic findings
All of the LASV DNA-vaccinated NHP survived to the study end point and showed no identifiable LASV-specific lesions or corresponding immunoreactivity in any of the tissues collected. The only histologic finding was mild lymphoid follicle hyperplasia, an expected nonspecific finding indicative of an increased immune response to a stimulus, presumably recent LASV infection (, inset). The mock-vaccinated NHP could be categorized based on pathologic lesions into 2 groups: acute and chronic. Those that succumbed during the acute hemorrhagic phase demonstrated gross and histologic lesions consistent with previous reports of LASV infection in primatesCitation25 and also demonstrated the presence of LASV antigen in multiple tissues ().
Figure 5. Pathologic analysis of selected tissues in LASV-exposed NHPs. A) Spleen, peri-arteriolar lymphoid sheath (PALS) hyperplasia in a vaccinated macaque that survived LASV challenge, 4X magnification; Inset, spleen, PALS hyperplasia with a complete absence of LASV immunoreactivity; B) Kidney, positive cytoplasmic immunoreactivity in islet and exocrine epithelial cells Of an NHP that succumbed on Day 11 post-exposure, 20x; C) Lung, cytoplasmic immunoreactivity in low numbers of alveolar macrophages, pneumocytes and endothelial cells in an NHP that succumbed on Day 17 post-exposure, 20x; D) Liver, positive apical to membranous hepatocyte and cytoplasmic endothelial immunoreactivity in an NHP that succumbed on Day 11 post-exposure, 40x.
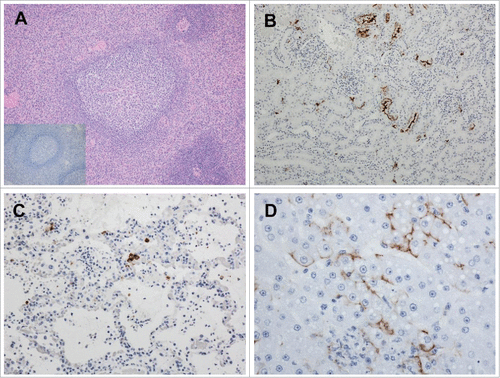
The chronically ill mock-vaccinated survivors had severe vascular lesions in multiple tissues to include the kidney, pancreas, mesentery, heart, testicles (in the male survivors), liver, gastrointestinal tract and ear consistent with a chronic-active perivasculitis to necrotizing and proliferative arteritis (data not shown). These lesions are not typically observed in NHPs that succumb during the LASV-associated hemorrhagic phase of disease.Citation25 Positive LASV immunoreactivity was identified in the testicles and pancreas of one mock-vaccinated survivor (data not shown). A detailed analysis of the pathology in these surviving NHP is described elsewhere (Cashman et al., Citationmanuscript in review).
Discussion
Lassa fever causes an enormous burden on the public health infrastructure in the endemic areas of Africa. Ribavirin and immune serum transfer have been used as post-exposure treatments with mixed results in humans.Citation36-38 Due to the large number of cases of Lassa fever that occur each year, a comprehensive preventative vaccine rather than post-exposure treatment would have a far greater impact on public health in the area. Our biodefense laboratory is engaged in research and development of DNA vaccines against agents of interest, including LASV. While other vaccine platforms, including inactivated whole virus, replication-competent candidates, a recombinant vesicular stomatitis virus-based vaccine, and a Mopeia/Lassa reassortant virus, have been or are currently being investigated, we believe that the DNA vaccination platform meets our needs.Citation39-52 From our perspective, DNA vaccines demonstrate attributes which make them attractive for use in remote/deployable areas such as ease of delivery, and speed of production, stability, safety, and lack of cold-chain accountability. We have observed promising results pairing DNA vaccines with ID-EP delivery. Electroporation is a physical technique used to increase cell permeability in vivo and enhance the uptake of DNA vaccines.Citation34,53-58 This delivery technology involves application of brief electrical pulses to the dermis, resulting in the creation of transient hydrophilic pathways within the lipid bi-layer membrane of mammalian cells. These perturbations allow the transport of DNA through cell membranes that were previously impermeable to such macromolecules. Historically, EP has been targeted at muscle tissue (IM-EP) and numerous clinical trials have successfully used this technology to enhance the delivery of DNA vaccines.Citation59-62
Targeting the skin for EP-enhanced vaccination is an attractive alternative to IM-EP not only because ID-EP is less invasive and has been shown to have a better acute tolerability than IM-EP,Citation35 but also because skin is a highly immunologically active organ.Citation63 The skin is rich in immune-active cell types that not only serve as targets for transfection with DNA plasmid vaccines, but also can be directly involved in mediating early immune system responses to DNA vaccines.Citation63
We previously demonstrated that 3 doses of the LASV DNA vaccine with ID-EP was more effective than IM-EP or gene gun at protecting guinea pigs from lethal infection.Citation33 Here, we demonstrated that the vaccine also protected NHPs from disease when an identical 3-dose vaccination schedule was used. In logistically challenging areas such as West Africa, as well as in biodefense scenarios, it is highly advantageous to have an effective vaccine requiring only one or 2 doses. We performed a study in which we vaccinated guinea pigs by ID-EP only once before LASV exposure, but we were only able to achieve partial protection with this abbreviated schedule (Cashman et al, unpublished data). In the study presented here, we were able to reduce the 3-dose schedule to 2-doses without loss of efficacy against virus challenge in NHP.
The immune correlates for protection against Lassa fever are not well-established either for NHPs or humans. We found that the LASV-GPC DNA vaccine elicited only modest levels of antibodies in both the 3-dose and 2-dose studies. Nevertheless, all LASV DNA-vaccinated NHP were protected from illness and death; thus, it is unlikely that the low titer neutralizing antibodies fully accounted for the protection observed. Cellular immunity rather than humoral immunity is thought to be the most important factor in protection against Lassa fever,Citation43,51,64 and our results support that theory. We acknowledge that although these results are promising, they are largely observational. Future planned studies will include more detailed analysis of the host humoral and cellular immune responses to vaccination before challenge to more fully investigate immune correlates of protection by the LASV DNA vaccine. In addition, we intend to examine the vaccine's ability to protect against other strains of LASV from geographically distinct areas to enable this platform to advance to safety and immunogenicity studies in humans.
Conclusion
The LASV DNA vaccine paired with ID-EP delivery is a promising vaccine platform that has been shown to completely protect guinea pigs and NHP against viremia, illness (acute and chronic), and death after LASV exposure. The ID-EP device used in this study has recently been developed into clinical trials evaluating several vaccine targets including HIV, influenza, ebola, and zika (NCT02431767, NCT01403155, NCT01405885, NCT02464670, and NCT02809443), demonstrating a favorable safety profile across a diverse range of target populations and clinical settings, and importantly, eliciting potent humoral and cellular immune responses in humans against the multi-valent vaccine antigens, thereby making this approach highly feasible for the development of an effective lassa vaccine for human use.
Materials and methods
Construction of codon-optimized Lassa Josiah GPC vaccine plasmid
The LASV, Josiah strain, GPC gene (Genbank Accession number AY628203.1) was optimized by GeneArt using a proprietary algorithm. In addition to codon usage optimization, negative cis-acting sites (such as splice sites, poly(A) signals, TATA boxes etc.) which may negatively influence expression were eliminated where relevant. The GC-content of the LASV GPC gene was adjusted to prolong mRNA half-life. Codon usage was adapted to the bias of Macaca fasicularis using a proprietary algorithm. The optimized sequence was synthetized and subcloned into the NotI/BglII site of expression vector pWRG7077Citation65 GeneArt (Germany). The plasmid was amplified by Aldevron (Fargo, ND) and provided as a 5 mg/ml solution in phosphate buffered saline, pH 7.4.
Vaccinations
Macaques were anesthetized and then received Mantoux-style shallow dermal injections of 100 µl containing 2.5 mg of DNA in solution at each of 4 different sites (both arms and both legs). Immediately after injection of the DNA solution, the injection site was electroporated using the CELLECTRA-3P device (Inovio Pharmaceuticals, Inc.). The CELLECTRA-3P ID-EP device allows for shallow intra-dermal/sub-cutaneous DNA delivery using a novel 3 electrode minimally invasive needle array.Citation66 This device consists of 3 needle electrodes, 3 mm in length, placed in an isosceles triangle formation mounted in a non-conductive material. The electroporation conditions were 0.2A constant current, 2 × 2 pulses, 52 ms in length with 3 sec between pairs of pulses. The depth of the electrodes results in full penetration of the tissue, reaching the epidermis, dermis and subcuticular layers. For the initial study, 3 vaccinations took place at 3 week intervals, followed by exposure to LASV 4 weeks after the last vaccination. The schedule for the second study consisted of 2 vaccinations at 4 week intervals, followed by exposure to LASV 5 weeks after the last vaccination. Blood samples were collected from each NHP just before each vaccination.
Virus exposure
LASV (Josiah strain) was diluted to a concentration of 1000 pfu/ml in sterile physiological saline. Macaques were given a single IM injection of 1000 pfu LASV, were monitored daily for disease progression and were killed when moribund according to IACUC-approved euthanasia criteria. Blood samples were collected on days 0, 3, 6, 10, 14, 28 and 45 post-exposure. Blood samples were analyzed for CBC, blood chemistry and serum viremia. Research was conducted under an IACUC approved protocol in compliance with the Animal Welfare Act, PHS Policy, and other Federal statutes and regulations relating to animals and experiments involving animals. The facility where this research was conducted is accredited by the Association for Assessment and Accreditation of Laboratory Animal Care, International and adheres to principles stated in the Guide for the Care and Use of Laboratory Animals, National Research Council, 2011.Citation67
Analysis of viremia
Serum samples collected just before exposure (day 0), and the post-exposure schedule described above were assayed for viremia via a standard plaque assay with minor modifications.Citation68 Briefly, Vero cells, seeded in 6-well cell culture plates, were adsorbed with gentle rotation at 37°C, 5% CO2 with 10-fold serial dilutions of serum for 1 h, then an overlay of 0.8% molecular grade agarose in EBME (basal medium Eagle with Earle's salts) with 10% fetal bovine serum and 20 μg/ml gentamicin was applied to each well and allowed to solidify. Overlayed cells were incubated at 37°C, 5% CO2 for 4 days, then stained with a neutral red overlay (Invitrogen, Carlsbad, CA). After an overnight incubation at 37°C in the staining overlay, plaques were counted and recorded.
Plaque-reduction neutralization test (PRNT)
Neutralizing capabilities of antibodies in the sera collected pre- and post-exposure were analyzed by a standard PRNT with some modifications.Citation69 Briefly, twofold dilutions of sera (in 100 µl volumes) were incubated for 1 h at 37°C with LASV diluted to approximately 100 pfu per serum dilution. After incubation, each serum dilution/virus mixture was then added to Vero cells seeded to approximately 90% confluency in 6-well cell tissue culture plates. The remainder of the procedure was performed as described above for the standard plaque assay. Plaques were counted and compared with control wells containing cells infected with 100 pfu LASV pre-incubated with a LASV naïve primate serum. Neutralizing antibody titers yielding a 50% reduction in plaques were determined.
Blood chemistry and hematology analysis
Serum samples collected pre- and post-exposure were analyzed for glucose, blood urea nitrogen, creatinine, uric acid, calcium, albumin, total protein, alanine aminotransferase (ALT), aspartate aminotransferase (AST), alkaline phosphatase (ALP), total bilirubin, gamma glutamyl transferase, and amylase. Approximately 100 μl of serum was applied to a General Chemistry 13-panel rotor and evaluated in a Piccolo point-of-care blood chemistry analyzer (Abaxis). Values for each serum sample were recorded and compiled. For the first study, CBC analysis was performed on a Hemavet 950FS Instrument (Drew Scientific), using an approximate volume of 75 µl of EDTA-treated whole blood. The Hemavet Instrument was not available for the second study, thus an Advia 120 Hematology Instrument (Siemens) was used. Values for each blood sample were recorded and compiled. Since different instruments were used to obtain CBC data, all graphs were generated using percent change from baseline readings.
Pathologic analysis of tissues
Tissues were trimmed and processed according to standard protocols. Sections were trimmed at 5–6 µm thickness and stained with hematoxylin and eosin. Immunohistochemistry was performed on replicate tissue sections for both partial and full necropsies using an Envision kit (Dako). A monoclonal antibody specific for LASV GP1 was used at a dilution of 1:15000. After deparaffinization and peroxidase blocking an antigen retrieval step was performed using a TRIS/EDTA buffer in a steamer for 30 minutes. Tissue sections were then covered with primary antibody and incubated at room temperature for 30 minutes, rinsed and peroxidase-labeled polymer (secondary antibody) applied for 30 minutes. Slides were rinsed again and a substrate-chromogen solution (DAB, Dako) was applied for 5 minutes. The slides were rinsed in distilled water and counterstained with hematoxylin for 2 minutes, dehydrated, cleared with xyless and then coverslipped. Slides were evaluated using a Nikon Eclipse 600 light microscope.
Disclosure of potential conflicts of interest
No potential conflicts of interest were disclosed.
Acknowledgments
The authors are grateful for the assistance of members of the Veterinary Medicine Division at USAMRIID for assistance during the vaccination phase of these studies. We also thank Heather Esham for guidance with primate phlebotomy in BSL-4 during the 3-dose study. The excellent work of the technicians in the Pathology Division for tissue preparation are highly appreciated. Opinions, interpretations, conclusions, and recommendations are those of the author and are not necessarily endorsed by the US Army.
Funding
Both of the NHP studies described in this work were funded by the Military Infectious Disease Research Program (MIDRP).
References
- Buchmeier MJ, De La Torre JC, Peters CJ. Arenaviridae: The viruses and their replication. In: Knipe DM, Howley PM, Griffin DE, Lamb RA, Martin A, Roizman B, et al., editors. Fields virology.. New York: Lippincott, Williams & Wilkins; 2007:1792-827.
- Enria DA, Mills JN, Bausch DG, Shieh WJ, Peters CJ. Arenavirus infections. In: Guerrant RL, Walker DH, Weller PF, editors. Tropical infectious diseases: Principles, pathogens & practice.. New York: Elsevier; 2011. p. 1130.
- Bodewes R, Kik MJ, Raj VS, Schapendonk CM, Haagmans BL, Smits SL, Osterhaus AD. Detection of novel divergent arenaviruses in boid snakes with inclusion body disease in The Netherlands. J Gen Virol. 2013;94:1206-10. doi:10.1099/vir.0.051995-0. PMID:23468423
- Hetzel U, Sironen T, Laurinmaki P, Liljeroos L, Patjas A, Henttonen H, Vaheri A, Artelt A, Kipar A, Butcher SJ, et al. Isolation, identification, and characterization of novel arenaviruses, the etiological agents of boid inclusion body disease. J Virol. 2013;87:10918-35. doi:10.1128/JVI.01123-13. PMID:23926354
- Stenglein MD, Sanders C, Kistler AL, Ruby JG, Franco JY, Reavill DR, Dunker F, Derisi JL. Identification, characterization, and in vitro culture of highly divergent arenaviruses from boa constrictors and annulated tree boas: Candidate etiological agents for snake inclusion body disease. mBio. 2012;3:e00180-12. doi:10.1128/mBio.00180-12. PMID:22893382
- Lenz O, ter Meulen J, Klenk HD, Seidah NG, Garten W. The Lassa virus glycoprotein precursor GP-C is proteolytically processed by subtilase SKI-1/S1P. Proc Natl Acad Sci U S A. 2001;98:12701-5. doi:10.1073/pnas.221447598. PMID:11606739
- Bishop DHL. Viruses with ambisense RNA genomes. In: Notkins AL, Oldstone MBA, editors. Concepts in viral pathogenesis II.. New York (NY): Springer New York; 1986. p. 32-9.
- Bowen MD, Rollin PE, Ksiazek TG, Hustad HL, Bausch DG, Demby AH, Bajani MD, Peters CJ, Nichol ST. Genetic diversity among Lassa virus strains. J Virol. 2000;74:6992-7004. doi:10.1128/JVI.74.15.6992-7004.2000. PMID:10888638
- Centers for Disease CaP. Lassa fever fact sheet. 2016. https://www.cdc.gov/vhf/lassa/pdf/factsheet.pdf
- Branco LM, Grove JN, Boisen ML, Shaffer JG, Goba A, Fullah M, Momoh M, Grant DS, Garry RF. Emerging trends in lassa fever: Redefining the role of immunoglobulin M and inflammation in diagnosing acute infection. Virol J. 2011;8:478. doi:10.1186/1743-422X-8-478. PMID:22023795
- Cummins D. Rats, fever and sudden deafness in Sierra Leone. Trop Doct. 1992;22:83-4. PMID:1604723
- Ibekwe TS, Okokhere PO, Asogun D, Blackie FF, Nwegbu MM, Wahab KW, Omilabu SA, Akpede GO. Early-onset sensorineural hearing loss in Lassa fever. Eur Arch Otorhinolaryngol. 2011;268:197-201. doi:10.1007/s00405-010-1370-4. PMID:20809263
- Liao BS, Byl FM, Adour KK. Audiometric comparison of Lassa fever hearing loss and idiopathic sudden hearing loss: Evidence for viral cause. Otolaryngol Head Neck Surg. 1992;106:226-9. doi:10.1177/019459989210600303. PMID:1589210
- Macher AM, Wolfe MS. Historical Lassa fever reports and 30-year clinical update. Emerg Infect Dis. 2006;12:835-7. doi:10.3201/eid1205.050052. PMID:16704848
- Okokhere PO, Ibekwe TS, Akpede GO. Sensorineural hearing loss in Lassa fever: Two case reports. J Med Case Rep. 2009;3:36. doi:10.1186/1752-1947-3-36. PMID:19178735
- Ter Meulen J, Lukashevich I, Sidibe K, Inapogui A, Marx M, Dorlemann A, Yansane ML, Koulemou K, Chang-Claude J, Schmitz H. Hunting of peridomestic rodents and consumption of their meat as possible risk factors for rodent-to-human transmission of Lassa virus in the Republic of Guinea. Am J Trop Med Hyg. 1996;55:661-6. doi:10.4269/ajtmh.1996.55.661. PMID:9025695
- Snell NJ. Ribavirin–current status of a broad spectrum antiviral agent. Expert Opin Pharmacother. 2001;2:1317-24. doi:10.1517/14656566.2.8.1317. PMID:11585000
- Fisher-Hoch SP, Gborie S, Parker L, Huggins J. Unexpected adverse reactions during a clinical trial in rural west Africa. Antiviral Res. 1992;19:139-47. doi:10.1016/0166-3542(92)90073-E. PMID:1444324
- Cashman KA, Smith MA, Twenhafel NA, Larson RA, Jones KF, Allen RD 3rd, Dai D, Chinsangaram J, Bolken TC, Hruby DE, et al. Evaluation of Lassa antiviral compound ST-193 in a guinea pig model. Antiviral Res. 2011;90:70-9. doi:10.1016/j.antiviral.2011.02.012. PMID:21371508
- Dai D, Burgeson JR, Gharaibeh DN, Moore AL, Larson RA, Cerruti NR, Amberg SM, Bolken TC, Hruby DE. Discovery and optimization of potent broad-spectrum arenavirus inhibitors derived from benzimidazole. Bioorg Med Chem Lett. 2013;23:744-9. doi:10.1016/j.bmcl.2012.11.095. PMID:23265895
- Larson RA, Dai D, Hosack VT, Tan Y, Bolken TC, Hruby DE, Amberg SM. Identification of a broad-spectrum arenavirus entry inhibitor. J Virol. 2008;82:10768-75. doi:10.1128/JVI.00941-08. PMID:18715909
- Gowen BB, Smee DF, Wong MH, Hall JO, Jung KH, Bailey KW, Stevens JR, Furuta Y, Morrey JD. Treatment of late stage disease in a model of arenaviral hemorrhagic fever: T-705 efficacy and reduced toxicity suggests an alternative to ribavirin. PloS One. 2008;3:e3725. doi:10.1371/journal.pone.0003725. PMID:19008960
- Gowen BB, Wong MH, Jung KH, Sanders AB, Mendenhall M, Bailey KW, Furuta Y, Sidwell RW. In vitro and in vivo activities of T-705 against arenavirus and bunyavirus infections. Antimicrob Agents Chemother. 2007;51:3168-76. doi:10.1128/AAC.00356-07. PMID:17606691
- Mendenhall M, Russell A, Juelich T, Messina EL, Smee DF, Freiberg AN, Holbrook MR, Furuta Y, de la Torre JC, Nunberg JH, et al. T-705 (favipiravir) inhibition of arenavirus replication in cell culture. Antimicrob Agents Chemother. 2011;55:782-7. doi:10.1128/AAC.01219-10. PMID:21115797
- Hensley LE, Smith MA, Geisbert JB, Fritz EA, Daddario-DiCaprio KM, Larsen T, Geisbert TW. Pathogenesis of Lassa fever in cynomolgus macaques. Virol J. 2011;8:205. doi:10.1186/1743-422X-8-205. PMID:21548931
- Dupuy LC, Schmaljohn CS. DNA vaccines for biodefense. Expert Rev Vaccines. 2009;8:1739-54. doi:10.1586/erv.09.132. PMID:19943766
- Saade F, Petrovsky N. Technologies for enhanced efficacy of DNA vaccines. Expert Rev Vaccines. 2012;11:189-209. doi:10.1586/erv.11.188. PMID:22309668
- Pannetier D, Reynard S, Russier M, Journeaux A, Tordo N, Deubel V, Baize S. Human dendritic cells infected with the nonpathogenic mopeia virus induce stronger T-cell responses than those infected with Lassa virus. J Virol. 2011;85:8293-306. doi:10.1128/JVI.02120-10. PMID:21632749
- Flatz L, Rieger T, Merkler D, Bergthaler A, Regen T, Schedensack M, Bestmann L, Verschoor A, Kreutzfeldt M, Brück W, et al. T cell-dependence of Lassa fever pathogenesis. PLoS Pathog. 2010;6:e1000836. doi:10.1371/journal.ppat.1000836. PMID:20360949
- Russier M, Pannetier D, Baize S. Immune responses and Lassa virus infection. Viruses. 2012;4:2766-85. doi:10.3390/v4112766. PMID:23202504
- Baize S, Marianneau P, Loth P, Reynard S, Journeaux A, Chevallier M, Tordo N, Deubel V, Contamin H. Early and strong immune responses are associated with control of viral replication and recovery in lassa virus-infected cynomolgus monkeys. J Virol. 2009;83:5890-903. doi:10.1128/JVI.01948-08. PMID:19297492
- ter Meulen J. Lassa fever: Implications of T-cell immunity for vaccine development. J Biotechnol. 1999;73:207-12. doi:10.1016/S0168-1656(99)00122-4. PMID:10486929
- Cashman KA, Broderick KE, Wilkinson ER, Shaia CI, Bell TM, Shurtleff AC, Spik KW, Badger CV, Guttieri MC, Sardesai NY, et al. Enhanced efficacy of a codon-optimized DNA vaccine encoding the glycoprotein precursor gene of lassa virus in a guinea pig disease model when delivered by dermal electroporation. Vaccines. 2013;1:262-77. doi:10.3390/vaccines1030262. PMID:26344112
- Amante DH, Smith TR, Mendoza JM, Schultheis K, McCoy JR, Khan AS, Sardesai NY, Broderick KE. Skin transfection patterns and expression kinetics of electroporation-enhanced plasmid delivery using the CELLECTRA-3P, a portable next-generation dermal electroporation device. Hum Gene Ther Methods. 2015;26:134-46. doi:10.1089/hgtb.2015.020. PMID:26222896
- Diehl MC, Lee JC, Daniels SE, Tebas P, Khan AS, Giffear M, Sardesai NY, Bagarazzi ML. Tolerability of intramuscular and intradermal delivery by CELLECTRA((R)) adaptive constant current electroporation device in healthy volunteers. Hum Vaccin Immunother. 2013;9:2246-52. doi:10.4161/hv.24702. PMID:24051434
- Bausch DG, Hadi CM, Khan SH, Lertora JJ. Review of the literature and proposed guidelines for the use of oral ribavirin as postexposure prophylaxis for Lassa fever. Clin Infect Dis. 2010;51:1435-41. doi:10.1086/657315. PMID:21058912
- Hadi CM, Goba A, Khan SH, Bangura J, Sankoh M, Koroma S, Juana B, Bah A, Coulibaly M, Bausch DG. Ribavirin for Lassa fever postexposure prophylaxis. Emerg Infect Dis. 2010;16:2009-11. doi:10.3201/eid1612.100994. PMID:21122249
- McCormick JB, King IJ, Webb PA, Scribner CL, Craven RB, Johnson KM, Elliott LH, Belmont-Williams R. Lassa fever. Effective therapy with ribavirin. N Engl J Med. 1986;314:20-6. doi:10.1056/NEJM198601023140104. PMID:3940312
- Bredenbeek PJ, Molenkamp R, Spaan WJ, Deubel V, Marianneau P, Salvato MS, Moshkoff D, Zapata J, Tikhonov I, Patterson J, et al. A recombinant yellow fever 17D vaccine expressing lassa virus glycoproteins. Virology. 2006;345:299-304. doi:10.1016/j.virol.2005.12.001. PMID:16412488
- Carrion R Jr, Bredenbeek P, Jiang X, Tretyakova I, Pushko P, Lukashevich IS. Vaccine platforms to control arenaviral hemorrhagic fevers. J Vaccines Vaccin. 2012;3:1000160. doi:10.4172/2157-7560.1000160. PMID:23420494
- Carrion R Jr, Patterson JL, Johnson C, Gonzales M, Moreira CR, Ticer A, Brasky K, Hubbard GB, Moshkoff D, Zapata J, et al. A ML29 reassortant virus protects guinea pigs against a distantly related Nigerian strain of Lassa virus and can provide sterilizing immunity. Vaccine. 2007;25:4093-102. doi:10.1016/j.vaccine.2007.02.038. PMID:17360080
- Fisher-Hoch SP, Hutwagner L, Brown B, McCormick JB. Effective vaccine for lassa fever. J Virol. 2000;74:6777-83. doi:10.1128/JVI.74.15.6777-6783.2000. PMID:10888616
- Fisher-Hoch SP, McCormick JB. Towards a human Lassa fever vaccine. Rev Med Virol. 2001;11:331-41. doi:10.1002/rmv.329. PMID:11590670
- Fisher-Hoch SP, McCormick JB. Lassa fever vaccine. Expert Rev Vaccines. 2004;3:189-97. doi:10.1586/14760584.3.2.189. PMID:15056044
- Geisbert TW, Jones S, Fritz EA, Shurtleff AC, Geisbert JB, Liebscher R, Grolla A, Ströher U, Fernando L, Daddario KM, et al. Development of a new vaccine for the prevention of Lassa fever. PLoS Med. 2005;2:e183. doi:10.1371/journal.pmed.0020183. PMID:15971954
- Jiang X, Dalebout TJ, Bredenbeek PJ, Carrion R Jr, Brasky K, Patterson J, Goicochea M, Bryant J, Salvato MS, Lukashevich IS. Yellow fever 17D-vectored vaccines expressing Lassa virus GP1 and GP2 glycoproteins provide protection against fatal disease in guinea pigs. Vaccine. 2011;29:1248-57. doi:10.1016/j.vaccine.2010.11.079. PMID:21145373
- Lukashevich IS. Advanced vaccine candidates for Lassa fever. Viruses. 2012;4:2514-57. doi:10.3390/v4112514. PMID:23202493
- Lukashevich IS, Carrion R Jr, Salvato MS, Mansfield K, Brasky K, Zapata J, Cairo C, Goicochea M, Hoosien GE, Ticer A, et al. Safety, immunogenicity, and efficacy of the ML29 reassortant vaccine for Lassa fever in small non-human primates. Vaccine. 2008;26:5246-54. doi:10.1016/j.vaccine.2008.07.057. PMID:18692539
- Lukashevich IS, Patterson J, Carrion R, Moshkoff D, Ticer A, Zapata J, Brasky K, Geiger R, Hubbard GB, Bryant J, et al. A live attenuated vaccine for Lassa fever made by reassortment of Lassa and Mopeia viruses. J Virol. 2005;79:13934-42. doi:10.1128/JVI.79.22.13934-13942.2005. PMID:16254329
- Marzi A, Feldmann F, Geisbert TW, Feldmann H, Safronetz D. Vesicular stomatitis virus-based vaccines against Lassa and Ebola viruses. Emerg Infect Dis. 2015;21:305-7. doi:10.3201/eid2102.141649. PMID:25625358
- McCormick JB, Mitchell SW, Kiley MP, Ruo S, Fisher-Hoch SP. Inactivated Lassa virus elicits a non protective immune response in rhesus monkeys. J Med Virol. 1992;37:1-7. doi:10.1002/jmv.1890370102. PMID:1619397
- Olschlager S, Flatz L. Vaccination Strategies against highly pathogenic arenaviruses: The next steps toward clinical trials. PLoS pathogens. 2013;9:e1003212. doi:10.1371/journal.ppat.1003212. PMID:23592977
- Mendoza JM, Amante DH, Kichaev G, Knott CL, Kiosses WB, Smith TR, Sardesai NY, Broderick KE. Elucidating the kinetics of expression and immune cell infiltration resulting from plasmid gene delivery enhanced by surface dermal electroporation. Vaccine. 2013;1:384-97. doi:10.3390/vaccines1030384. PMID:26344120
- Amante DH, Smith TR, Kiosses BB, Sardesai NY, Humeau LM, Broderick KE. Direct transfection of dendritic cells in the epidermis after plasmid delivery enhanced by surface electroporation. Hum Gene Ther Methods. 2014;25:315-6. doi:10.1089/hgtb.2014.061. PMID:25470335
- Broderick KE, Khan AS, Sardesai NY. DNA vaccination in skin enhanced by electroporation. Methods Mol Biol. 2014;1143:123-30. doi: 10.1007/978-1-4939-0410-5_8. PMID:24715285
- Kulkarni V, Rosati M, Jalah R, Ganneru B, Alicea C, Yu L, Guan Y, LaBranche C, Montefiori DC, King AD, et al. DNA vaccination by intradermal electroporation induces long-lasting immune responses in rhesus macaques. J Med Primatol. 2014;43:329-40. doi:10.1111/jmp.12123. PMID:24810337
- McCoy JB, Mendoza JM, Spik KW, Badger C, Gomez A, Schmaljohn CS, Sardesai NY, Broderick KE. A multi-head intradermal electroporation device allows for tailored and increased dose DNA vaccine delivery to the skin. Hum Vaccin Immunother. 2014;10:3039-47. doi:10.4161/hv.29671. PMID:25483486
- Roos AK, Moreno S, Leder C, Pavlenko M, King A, Pisa P. Enhancement of cellular immune response to a prostate cancer DNA vaccine by intradermal electroporation. Mol Ther. 2006;13:320-7. doi:10.1016/j.ymthe.2005.08.005. PMID:16185933
- Tollefsen S, Tjelle T, Schneider J, Harboe M, Wiker H, Hewinson G, Huygen K, Mathiesen I. Improved cellular and humoral immune responses against Mycobacterium tuberculosis antigens after intramuscular DNA immunisation combined with muscle electroporation. Vaccine. 2002;20:3370-8. doi:10.1016/S0264-410X(02)00289-X. PMID:12213407
- Lin F, Shen X, McCoy JR, Mendoza JM, Yan J, Kemmerrer SV, Khan AS, Weiner DB, Broderick KE, Sardesai NY. A novel prototype device for electroporation-enhanced DNA vaccine delivery simultaneously to both skin and muscle. Vaccine. 2011;29:6771-80. doi:10.1016/j.vaccine.2010.12.057. PMID:21199706
- Trimble CL, Morrow MP, Kraynyak KA, Shen X, Dallas M, Yan J, Edwards L, Parker RL, Denny L, Giffear M, et al. Safety, efficacy, and immunogenicity of VGX-3100, a therapeutic synthetic DNA vaccine targeting human papillomavirus 16 and 18 E6 and E7 proteins for cervical intraepithelial neoplasia 2/3: A randomised, double-blind, placebo-controlled phase 2b trial. Lancet. 2015;386:2078-88. doi:10.1016/S0140-6736(15)00239-1. PMID:26386540
- Bagarazzi ML, Yan J, Morrow MP, Shen X, Parker RL, Lee JC, Giffear M, Pankhong P, Khan AS, Broderick KE, et al. Immunotherapy against HPV16/18 generates potent TH1 and cytotoxic cellular immune responses. Sci Transl Med. 2012;4:155ra38. doi:10.1126/scitranslmed.3004414. PMID:23052295
- Di Meglio P, Perera GK, Nestle FO. The multitasking organ: Recent insights into skin immune function. Immunity. 2011;35:857-69. doi:10.1016/j.immuni.2011.12.003. PMID:22195743
- Lukashevich IS. The search for animal models for Lassa fever vaccine development. Expert Rev Vaccines. 2013;12:71-86. doi:10.1586/erv.12.139. PMID:23256740
- Schmaljohn C, Vanderzanden L, Bray M, Custer D, Meyer B, Li D, Rossi C, Fuller D, Fuller J, Haynes J, et al. Naked DNA vaccines expressing the prM and E genes of Russian spring summer encephalitis virus and Central European encephalitis virus protect mice from homologous and heterologous challenge. J Virol. 1997;71:9563-9. PMID:9371620
- Hirao LA, Wu L, Khan AS, Satishchandran A, Draghia-Akli R, Weiner DB. Intradermal/subcutaneous immunization by electroporation improves plasmid vaccine delivery and potency in pigs and rhesus macaques. Vaccine. 2008;26:440-8. doi:10.1016/j.vaccine.2007.10.041. PMID:18082294
- Academies NRCotN. Guide for the care and use of laboratory animals.. Washington (DC): The National Academies Press; 2011.
- Tomori O, Johnson KM, Kiley MP, Elliott LH. Standardization of a plaque assay for Lassa virus. J Med Virol. 1987;22:77-89. doi:10.1002/jmv.1890220110. PMID:3585290
- Hooper JW, Kamrud KI, Elgh F, Custer D, Schmaljohn CS. DNA vaccination with hantavirus M segment elicits neutralizing antibodies and protects against seoul virus infection. Virology. 1999;255:269-78. doi:10.1006/viro.1998.9586. PMID:10069952