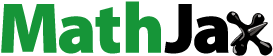
ABSTRACT
Immunotherapy has brought high hopes for cancer treatment, and attracted tremendous resources from the biopharmaceutical community. Here we analyze cancer immunotherapy-related patents granted by the United States Patent and Trademark Office in the past decade (2006–2016). A total of 2,229 patents were identified in 13 subfields. The growth of patent number in this field has outpaced the background rate, with cytokine-related therapies, immune checkpoint inhibitors, and natural killer cell therapies growing the most rapidly. The top 15 assignees possess 27.6% (616) of the patents. Amgen is the largest patent holder, followed by Novartis, and then by Chugai Seiyaku. The top assignees have focused on different subfields, and collaborated with each other for technology development. Our competitive analysis reveals that Novartis, Chugai Seiyaku, and Abbvie lead in both patent number and average quality of patents. Meanwhile, Immunomedics owns a high-quality though relatively small patent portfolio in single-chain variable fragment technology, which is not the focus of the abovementioned forerunners. Overall, our analysis illustrates an ecosystem where industry giants and smaller-size players each occupies a niche. Selection and succession are expected to continue for years in this young ecosystem.
Introduction
Immunotherapy has been heralded as our best chance to terminate cancers.Citation1 Surprisingly favorable clinical outcomes of cancer immunotherapyCitation2-7 have ignited fervent competitions to claim inventions in this field. Broadly defined, cancer immunotherapy includes cytokine-related therapies, immune checkpoint inhibitors, immune cell-based therapies (such as chimeric antigen receptor T cell, or CAR-T), cancer vaccines (active and passive), antibody-drug conjugates, oncolytic viruses, and other immunity-based technologies.
One of the most significant advances in cancer immunotherapy is the approval of check point inhibitors by the FDA (US Food and Drug Administration), including PD-1/PD-L1 inhibitors Pembrolizumab, Nivolumab, Atezolizumab, Avelumab, and Durvalumab. These entities have significantly improved on the treatment of melanoma, Hodgkin lymphoma, renal cancer, bladder cancer, non-small cell lung cancer (NSCLC), and head and neck cancer. Another class of checkpoint inhibitor targets CTLA-4, (Ipilimumab), which has been approved for the treatment of melanoma.Citation8 In year 2016 alone, FDA approved 5 new molecular entities, 17 efficacy supplements, and 5 companion diagnostics for cancer immunotherapy.Citation9 Despite the successes of immunotherapies in FDA clearance, these novel treatments may cause serious adverse effects in certain patients. Adequate management is required to balance between therapeutic benefits and damages to the patients.Citation10-13 Cancer immunotherapies other than checkpoint inhibitors have also faced challenges. For instance, the clinical benefits of several monoclonal antibodies have been called into question.Citation13
Recently, the scope of cancer immunotherapy has continued to expand to other therapeutic paradigms, including but not limited to personalized medicine,Citation14,15 combination of targeted and immunotherapies,Citation16 novel delivery methods,Citation17 cancer stem cells,Citation18 epigenetics,Citation19 liquid biopsy,Citation20 and novel biomaterials.Citation16 The cross-discipline integrations signify the rapidly growing influence, practicability, and adaptability of cancer immunotherapy. These exciting developments may not be reflected in the current patent landscape because of the time lapse between scientific innovation and technological implementation (and the subsequent patent application), but could possibly be observed in collaborations between key players in the field. Meanwhile, challenges remain for cancer immunotherapy. An important issue is to screen for a patient population suitable for a certain treatment scheme. Appropriate biomarkers are required to ensure safety and efficacy of immunotherapeutics.Citation21-24 With increasing applications of immunotherapeutics, resistance to current drugs is expected to occur.Citation25 Multiple therapeutic options should be available for one indication to overcome drug resistance. This future need could further foster the development of cancer immunotherapy, which could be observed in the patenting activities in this field.
The patenting activities in different subfields reflect strategies for product development. For instance, a company may be actively patenting cell-based therapies but lacking strength in checkpoint inhibitors. Another company could partner with a business ally to co-develop a critical vaccine technology, and jointly file patent applications. The number and quality of patents owned by an industry player indicate its competitive edge in the technology field. Such information is precious yet difficult to find for the field of cancer immunotherapy.
Patenting is the most effective means of excluding market competitions. Unlike consumer electronics, a biopharmaceutical product is usually protected by a small number of patents. Standard essential patents and patent pooling are seldom seen in the biopharma industry. The key to success of a biopharmaceutical product is to prove its safety and therapeutic benefits in clinical trials, which are stringently regulated and the outcomes are unpredictable. Such stringency and unpredictability have consumed enormous human and financial resources, and greatly increased the risk in product development.
To protect potentially massive returns, drug makers have raced to build strong patent portfolios. Patent analysis can provide a snapshot of patenting activities of the industry. A number of immunotherapy-related patent analyses have been available, covering such subfields as general anticancer therapies,Citation26 immune checkpoint inhibitors,Citation27,28 dendritic cell-based therapy,Citation29 oncolytic virus,Citation30 monoclonal antibodies,Citation31 and antibody-drug conjugates.Citation32 However, the patents of other important subfields, e.g. T cell-based therapy, vaccine, and bispecific antibody, remain unanalyzed. Furthermore, overview of the entire filed of immunotherapy and competitive analysis are still unavailable. The Unites States of America is the largest single pharmaceutical market. The advanced US legal system for intellectual property protection further increases the nation's attraction to inventors and potential patentees worldwide. Importantly, access to patent raw data from the United States Patent and Trademark Office (USPTO) has recently become feasible. Here we analyze US patents related to cancer immunotherapy. This analysis aims to address 3 important questions: (1) What is the patenting trend in each of the subfields of cancer immunotherapy? (2) Who are the major players and what are their relative strengths in the field? (3) Has any subfield or the entire field of immunotherapy been dominated by a small number of players? Our results suggest that the field of immunotherapy as a whole is still growing, with varying growth rates among different subfields. Although a few players possess relatively large numbers of patents, the field appears to remain dispersed. Joint development, cross-licensing activities, and mergers/acquisitions may occur for consolidation of this new technology field.
Results and discussion
Patent trend
shows that the number of immunotherapy-related patents trended upwards from 2006. The number of patents decreases slightly in 2016 because the 2016 data were incomplete (Materials and Methods). This upward trend reflects that considerable resources have been attracted to this promising field in recent years. The annual growth rate of immunotherapy-related patents, defined as the percentage of increase in patent number over the previous year, is shown in . The sharpest increase in immunotherapy-related patents occurred during 2008–2010, with an annual growth rate falling between 36–50%. Interestingly, a temporary decline in patent number followed in 2011 before the growth rate rebounded to ∼20% in 2012. The background growth rate (i.e. the growth rate of all of the issued US patents) exhibited a similar trend, but with a smaller variation. The background growth rate peaked at 2010 (27%). The slowdown from 2011 observed for immunotherapy-related patents also occurred to the background growth rate. Overall, immunotherapy-related patents have been increasing more rapidly than patents in other fields. We have also analyzed the number of cancer immunotherapy-related publications at PubMed and patent publications. shows that the growth in applications peaked at Year 2009 and Year 2014, whereas the growth of publications has been escalating since Year 2009. Academic activities usually lead industry applications by several years. The steady recent increase in cancer immunotherapy-related publication may foretell growth in patent number in the years to come.
Figure 1. (A) The numbers and of cancer immunotherapy-related US patents (B) the annual growth rates of cancer immunotherapy-related US patents, patent applications, and PubMed publications from Year 2006 to 2016, as compared with the growth rate of all issued US patents. Note that no growth rate is available for 2006 (the first year of the data set) and 2016 (data incomplete).
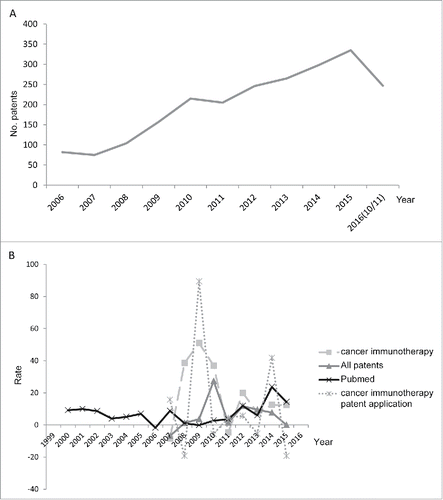
The majority of the analyzed patents (1,463/2,229) do not claim to target any specific cancer type. Two hundred 83 patents target one cancer type, while 483 patents target multiple cancer types. The top 10 cancer types that are explicitly targeted by the analyzed patents are shown in . Each of these cancer types is targeted by more than 150 patents, and the top 5 (colorectal cancer, breast cancer, lymphoma, lung cancer, and melanoma) are each targeted by more than 200 patents.
Figure 2. The number of patents that claim to target specific cancer types. Note that one patent can claim to target multiple cancer types.
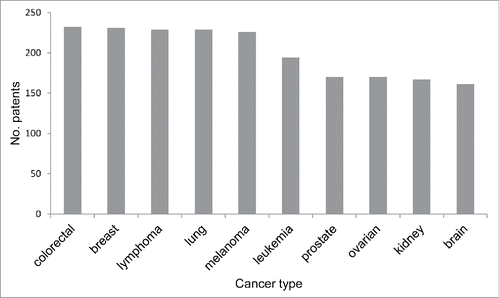
As expected, the 2,229 patents were mostly granted to US applicants (∼1600 patents), followed by Japanese, French, German, and Chinese applicants (). The high percentage (> 70%) of US-owned patents clearly indicates the dominance of US in this technology field. Also noteworthy is that Japan had the second largest number of immunotherapy-related patents (248), dwarfing France (122), Germany (106), Switzerland (87), and the United Kingdom (74). Of note, many biopharmaceutical companies have branches in both of the US and Europe. The observed distribution thus may not completely reflect the patenting activities of Europe-based companies.
Figure 3. The numbers of cancer immunotherapy-related patents (A) assigned to entities from different countries and (B) issued to top 20 inventors.
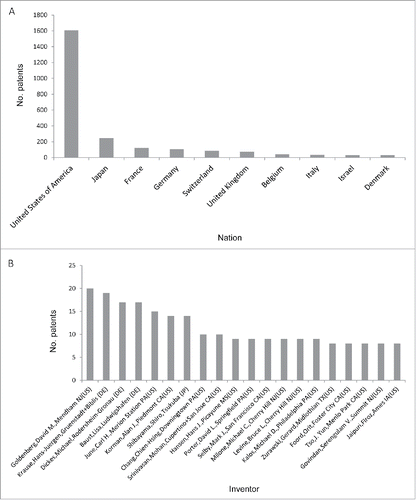
demonstrates the top 20 inventors of the 2,229 analyzed patents. As expected, most (16/20) of the top inventors were US citizens. Interestingly, only one Japanese inventor (Shiro Shibayama) made to this top 20 list, even though Japanese entities owned the second largest number of immunotherapy-related patents. By contrast, 3 German inventors (Hans-Juergen Krause, Michael Dickes, and Lisa Baust) were listed in the top 20, despite the smaller number of patents owned by German than by Japanese entities ( and ). These observations imply that the efforts in developing cancer immunotherapy technology are more scattered in Japan as compared with in Germany. In addition, the 3 German inventors might have been the employees of the same entity or entities undergoing a joint venture. We then analyzed the co-inventorship of these top 20 inventors. shows that David M. Goldenberg and Chien-Hsing Chang co-invented 10 patented technologies. Among the 10 patents, 5 were assigned to Immunomedics, 4 assigned to IBC Pharmaceuticals, and the remaining one assigned to both companies. Indeed, IBC Pharmaceuticals was founded as a result of a joint venture between Immunomedics and Beckman Coulter (http://www.immunomedics.com/ibc-pharmaceuticals.shtml). Smilarly, Carl H. June and David L. Porter, Michael C. Milone, and Bruce L. Levine co-invented in 9 patents in University of Pennsylvania (). Alan J. Korman co-invented with Mark J. Selby and Mohan Srinivasan, in a variety of entities (). Meanwhile, the 3 German inventors, Krause Hans-Juergen, Dickes Michael, and Baust Lisa co-invented in 17 patents, all of which were assigned to AbbVie (data not shown). This co-inventorship analysis revealed which entities the top inventors worked for, and how they collaborated with each other in the development of immunotherapy-related technologies.
Figure 4. The co-inventorship among the top 20 inventors. The names of the inventors are shown on both sides. The company/institution names in the middle indicate the assignees of the relevant patents. The numbers in the parentheses represent the numbers of patents when the inventors were affiliated with the company/institution.
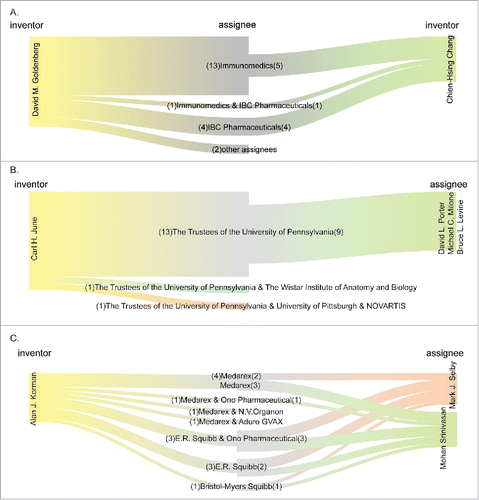
shows the distribution of patents among the 13 subfields of cancer immunotherapy. Cytokine-related patents accounted for the largest group (> 800 patents), followed by patents related to immune checkpoint inhibitors, natural killer cell, cancer vaccines, single-chain variable fragment, and so on. The disparity in patent numbers among different subfields partly resulted from the differences in the stage of technology development. Newly emerged therapies, such as IDO (indoleamine 2,3-dioxygenase) inhibitors, tumor-infiltrating lymphocytes (TILs), CAR-T (chimeric antigen receptor T cell) understandably included only small numbers of patents. In comparison, cytokine-related therapies have long been recognized as a potential treatment of cancer.Citation33-35 And the patenting activities indicate that interests in this subfield are long-lasting. Meanwhile, oncolytic virus-related patents represented only a small group although the potential of this technology had been explored early,Citation36-38 suggesting that the industry has not been passionate in further developing this subfield. This cautious attitude probably resulted from potential risks and unpredictable outcomes of oncolytic virus therapy.Citation39 The temporal development of these 13 subfields is shown in . The patent numbers in most of the groups increased over time, with patents related to cytokine, immune checkpoint, and natural killer cell growing most rapidly. Interestingly, year 2007–2008 seems to be a starting point of rapid growth for all these 3 subfields. In comparison, the growth of patents related to vaccines and T cell-based therapies seem to have slowed down since year 2010, whereas those related to antibody-drug conjugates and single-chain variable fragment continue to grow steadily.
Development strategies and relative strengths of the top 15 assignees
The next important question is which entities own the immunotherapy-related patents. shows the top 15 assignees of the 2,229 patents. Twelve of the entities were biopharmaceutical companies. Only 3 were academic institutes (the Regents of the University of California, the Trustee of the University of Pennsylvania, and the Dana-Farber Cancer Institute). Amgen, Novartis, and Chugai Seiyaku Kabushiki Kaisha possessed the largest numbers of immunotherapy-related patents. The top 15 assignees collectively owned 616 (27.6%) of the 2,229 patents. Amgen, the top assignee, owned only 88 of the patents, which represented 3.9% of the 2,229 patents, and 14.3% of the 616 patents. This observation suggests that the patent landscape of immunotherapy has remained dispersed, and that cross-licensing, joint venture, and merger/acquisition events might increase in the years to come. Indeed, collaborations among the top 15 assignees were observed. Two patents were co-owned by Novartis and Amgen, 16 were shared by Amgen and Pfizer, and another 5 by Pfizer and Bristol-Myers-Squibb (). lists the patents co-owned by these assignees. Apparently Amgen and Pfizer joined force to develop monoclonal antibodies against CTLA-4, CD40, and insulin-like growth factor I receptor. Meanwhile, Pfizer collaborated with Bristol-Meyer-Squibb to develop antibodies against OX40, integrin α5β1, and IL-6. This observation indicates that resourceful pharmaceutical giants have been using a multi-channel development strategy by partnering with different entities.
Figure 6. The numbers of cancer immunotherapy-related patents (A) owned individually and (B) co-owned by top 15 assignees. Note that the co-owned patents in (B) were counted as one patent assigned to each of the 2 co-owners in (A).
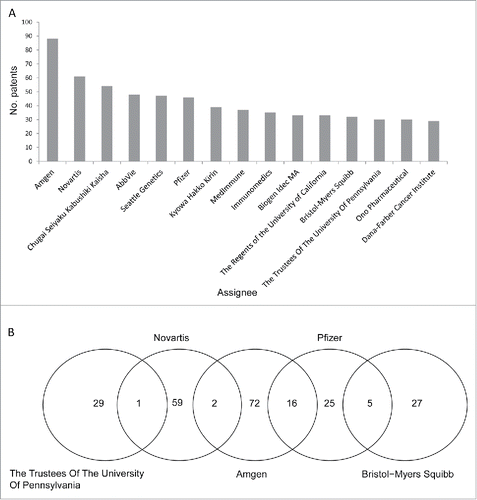
Table 1. Patents co-owned by top assignees.
To evaluate the relative technology strengths for each of the top 15 assignees, we generated the Radar Diagram (Materials and Methods). indicates that Amgen had strong standings in antibody, immune checkpoint, IDO inhibitor, and T cell-related therapies. In comparison, Novartis focused more on natural killer cell, cancer vaccines, dendritic cell, and TILs. Meanwhile, Seattle Genetics seemed to specialize in antibody-drug conjugates, while Ohno Pharmaceutical was focused on CAR-T therapies. Apparently the top 15 assignees have different roadmaps for technology development. This is consistent with our above proposition that the patent landscape of immunotherapy has remained dispersed. Further consolidation or collaborations are expected to occur.
Next, we analyzed the qualities (measured by the patent quality index “PQ6;" Materials and Methods) versus the numbers of patents owned by the top 15 assignees. The median values of patent number and PQ6 were used to delineate the data space into 4 quadrants (). The upper-right quadrant included entities that had relatively large numbers of high-quality patents, including Novartis, Chugai Seiyaku Kabushiki Kaisha, Abbvie, and Seattle Genetics. These companies have the potential of becoming leaders of the relevant subfields given sufficient time or strategic alliances for development. The lower-right quadrant harbored entities with smaller numbers of high-quality patents, including Immunomedics, Biogen Idec, and the Dana-Farber Cancer Institute. The patent numbers of these assignees would have to keep growing to join the forerunner group. Although Amgen had the largest number of patents, the average PQ6 score of its portfolio was slightly lower than the median. Nevertheless, the large patent portfolio could be a powerful weapon in the increasingly vehement competitions in cancer immunotherapy.
Analysis and perspective
The challenges of cancer immunotherapy also bring opportunities for technological innovations. First, current cancer immunotherapies are usually effective only in a subgroup of patients. Obviously, novel diagnostics are required to identify the patient population suitable for a certain immunotherapy scheme. To this end, liquid biopsy, novel biomarkers, omics-based tests, and the broad concept of precision medicine are expected to converge with cancer immunotherapy. Second, the adverse drug effects of immunotherapies suggest that adjunctive therapies to ameliorate such effects without compromising the efficacy of the primary therapy are needed. Alternatively, researchers may also consider developing future therapies with smaller side effects, or novel delivery methods to limit the range of drug actions. Third, the possibility of drug resistance foretells the needs for multiple therapeutic options, combinatorial therapies, and next-generation immunotherapies for each cancer type. This also indicates joint developments between major players of different expertise in the field. All of these directions are expected to emerge in the future patent landscape of cancer immunotherapy.
Concluding remarks
The development of cancer immunotherapy is clearly on the rise (), yet subfields differ from each other in patent number and growth rate (), reflecting uneven resource allocation by the community to different subfields. Meanwhile, major players in cancer immunotherapy apply divergent strategies in technology development, and specialize in different subfields (). Joint development thus may be mutually beneficial, as can be observed in the collaborations between top patent assignees ( and , ). The biotech giant, Amgen, has so far harvested more patents than any other competitors in the field of immunotherapy. Other companies, such as Novartis, Chugai Seiyaku Kabushiki Kaisha, and Immunomedics have fewer but relatively high-quality patents. (). The dynamic patent landscape of cancer immunotherapy is expected to keep changing rapidly in the foreseeable future, and is worth close attention from stakeholders in the field.
Methods
Patent search and classification
summarizes the analysis flow. To begin with, all of the patent documents in XML format were downloaded from the USPTO website at https://bulkdata.uspto.gov/. The XML files were then parsed using an in-house PERL script. The patent number, abstract, assignee(s), inventor(s), international patent classification codes, and claims were retrieved and dumped into an in-house MySQL database. Two rounds of keyword searches were then conducted in this database. The search space was limited to patents issued between January 2006 and October 11, 2016, and patents under IPC categories A、C、G01N and G06F. The first round of search (downward arrow from “In-house DB”) was based on immunotherapy-related keywords retrieved from a review article authored by Kohrt and colleagues (2016).Citation2 The synonyms, abbreviations, alias and/or full names of these keywords were retrieved from GeneCards and Wikipedia (S1 Table). The S1 Table keywords were then used to search against the title, abstract, and claims of each patent in the in-house database, which yielded Result (1). Note that certain keywords must be capitalized to be considered as correct keywords when appearing in a patent document. These include 19A, CD2, CLA, MICA, ML, NAIL, NK, SELL, PVR, TRAP, LIGHT, BLAST, T1, APRIL, H1, and TDO. A total of 4,491 patents were thus identified.
The second round of search (rightward arrow from “In-house DB”) was based on company names. The companies known to be developing immunotherapy were retrieved from a business intelligence report.Citation40 Merger and acquisition histories of interested companies were retrieved from Wikipedia. The names of immunotherapy-related subsidiaries of major companies were also considered. These company names were searched in the “assignee” column of the patent documents. Cancer-related wildcard keywords (cancer, tumor, tumor, *noma, *ioma, *toma, *sarcoma, leukemia, and *moma) and immunotherapy-related keywords (immune*,immune, antibod*, interferon, killer cell, NK cell, adoptive T, vaccine, cytokine, IDO inhibitor, CAR T, chimeric antigen receptor, indoleamine-2,3-dioxygenase, IL-1R, IL-2R, IL-7R, c-KIT, CCR, CXCR, and CX3CR1) were used to further limit the scope of the search space. 1,267 patents were identified by using this approach (Result (2)).
Results (1) and (2) were combined. The total of 5,758 (4,491+ 1,267) patents were manually curated to further screen out irrelevant and distantly related patents. Finally, 2,229 patents were retained. These patents were then classified into 13 groups based on subfield-related keywords (S2 Table).Citation2 Note that one patent can be classified into multiple groups. The sum of patent numbers across the 13 groups was thus larger than 2,229.
Patent quality analysis
Patent quality was measured by PQ(6).Citation41,42 PQ(6) is the average of 6 normalized measurements: number of forward citations, number of backward citations, number of claims, family size, generality index, and grant lag. The numbers of forward citations, backward citations, and claims were retrieved from the downloaded patent data. Family size, defined as the number of patents in an INPADOC family (https://www.epo.org/searching-for-patents/helpful-resources/first-time-here/patent-families/inpadoc.html), was obtained from the Espacenet Patent Search web page (http://www.epo.org/searching-for-patents/technical/espacenet.html#tab1). Family size, the number of forward/backward citations, and the number of claims were separately normalized by dividing the measure of the interested patent by the maximal measure of the patent cohort. Note that a maximal family size of 490 is given by the Espacenet if the actual size exceeds this number. A total of 15 out of the 616 patents that belonged to the top 15 assignees were given the family size of 490. The generality indexCitation42 was defined as
where Spj is the percentage of forward citations received by patent p from 4-digits IPC technology class j.
Grant lag was defined as:
where Δt was the time between the date of filing and the date of issuance (days);
MaxΔti was the maximal Δt of patent cohort i. Both grant lag and the number of backward citations were extracted from the downloaded patent documents.
Relative strength analysis
Radar Diagrams were used to exhibit the relative strengths of the top 15 patent assignees in different subfields. The patents owned by the top 15 assignees (total 616 patents) were classified into 13 subfields as mentioned above. For an assignee, the strength in a subfield was defined as the proportion of the assignee's patents over the total number of patents owned by the top 15 assignees in this subfield. Therefore, each assignee had 13 strength measures, with each corresponding to one subfield.
Disclosure of potential conflicts of interest
The authors declare that they have no competing interests.
Author contributions
FCC conceived of and designed the study. CPL conducted data collection and analysis. CPL and FCC drafted the manuscript.
Supplementary_information.zip
Download Zip (52.2 KB)Funding
This study was supported by the intramural funding of National Health Research Institutes, Taiwan (105-IPHS-PP06 and 106-IPHS-PP06).
References
- Farkona S, Diamandis EP, Blasutig IM. Cancer immunotherapy: The beginning of the end of cancer? BMC Med. 2016;14:73. doi:10.1186/s12916-016-0623-5. PMID:27151159.
- Kohrt HE, Tumeh PC, Benson D, Bhardwaj N, Brody J, Formenti S, Fox BA, Galon J, June CH, Kalos M, et al. Immunodynamics: A cancer immunotherapy trials network review of immune monitoring in immuno-oncology clinical trials. J Immunother Cancer. 2016;4:15. doi:10.1186/s40425-016-0118-0. PMID:26981245.
- Sharon E, Streicher H, Goncalves P, Chen HX. Immune checkpoint inhibitors in clinical trials. Chin J Cancer. 2014;33:434–44. doi:10.5732/cjc.014.10122. PMID:25189716.
- Emens LA, Butterfield LH, Hodi FS Jr, Marincola FM, Kaufman HL. Cancer immunotherapy trials: Leading a paradigm shift in drug development. J Immunother Cancer. 2016;4:42. doi:10.1186/s40425-016-0146-9. PMID:27437105.
- Melero I, Berman DM, Aznar MA, Korman AJ, Pérez Gracia JL, Haanen J. Evolving synergistic combinations of targeted immunotherapies to combat cancer. Nat Rev Cancer. 2015;15:457–72. doi:10.1038/nrc3973. PMID:26205340.
- Gedye C, van der Westhuizen A. & John T. Checkpoint immunotherapy for cancer: Superior survival, unaccustomed toxicities. Intern Med J. 2015;45:696–701. doi:10.1111/imj.12653. PMID:25444021.
- Zavala VA, Kalergis AM. New clinical advances in immunotherapy for the treatment of solid tumours. Immunology. 2015;145:182–201. doi:10.1111/imm.12459. PMID:25826229.
- Postow MA, Callahan MK, Wolchok JD. Immune Checkpoint Blockade in Cancer Therapy. J Clin Oncol. 2015;33:1974–82. doi:10.1200/JCO.2014.59.4358. PMID:25605845.
- Blumenthal GM, Pazdur R. Approvals in 2016: The march of the checkpoint inhibitors. Nat Rev Clin Oncol. 2017;14:131–2. doi:10.1038/nrclinonc.2017.15. PMID:28218255.
- Hassel JC, Heinzerling L, Aberle J, Bähr O, Eigentler TK, Grimm MO, Grünwald V, Leipe J, Reinmuth N, Tietze JK, et al. Combined immune checkpoint blockade (anti-PD-1/anti-CTLA-4): Evaluation and management of adverse drug reactions. Cancer Treat Rev. 2017;57:36–49. doi:10.1016/j.ctrv.2017.05.003. PMID:28550712.
- Eigentler TK, Hassel JC, Berking C, Aberle J, Bachmann O, Grünwald V, Kähler KC, Loquai C, Reinmuth N, Steins M, et al. Diagnosis, monitoring and management of immune-related adverse drug reactions of anti-PD-1 antibody therapy. Cancer Treat Rev. 2016;45:7–18. doi:10.1016/j.ctrv.2016.02.003. PMID:26922661.
- Kumar V, Hassel JC, Berking C, Aberle J, Bachmann O, Grünwald V, Kähler KC, Loquai C, Reinmuth N, Steins M, et al. Current Diagnosis and Management of Immune Related Adverse Events (irAEs) Induced by Immune Checkpoint Inhibitor Therapy. Front Pharmacol. 2017;8:49. doi:10.3389/fphar.2017.00049. PMID:28228726.
- Booth CM, Del Paggio JC. Approvals in 2016: Questioning the clinical benefit of anticancer therapies. Nat Rev Clin Oncol. 2017;14:135–6. doi:10.1038/nrclinonc.2017.18. PMID:28218259.
- Bethune MT, Joglekar AV. Personalized T cell-mediated cancer immunotherapy: Progress and challenges. Curr Opin Biotechnol. 2017;48:142–52. doi:10.1016/j.copbio.2017.03.024. PMID:28494274.
- Capietto AH, Jhunjhunwala S, Delamarre L. Characterizing neoantigens for personalized cancer immunotherapy. Curr Opin Immunol. 2017;46:58–65. doi:10.1016/j.coi.2017.04.007. PMID:28478383.
- Gotwals P, Cameron S, Cipolletta D, Cremasco V, Crystal A, Hewes B, Mueller B, Quaratino S, Sabatos-Peyton C, Petruzzelli L, et al. Prospects for combining targeted and conventional cancer therapy with immunotherapy. Nat Rev Cancer. 2017;17:286–301. doi:10.1038/nrc.2017.17. PMID:28338065.
- Francis DM, Thomas SN. Progress and opportunities for enhancing the delivery and efficacy of checkpoint inhibitors for cancer immunotherapy. Adv Drug Deliv Rev. 2017;114:33–42. doi:10.1016/j.addr.2017.04.011. PMID:28455187.
- Fiori ME, Villanova L, De Maria R. Cancer stem cells: At the forefront of personalized medicine and immunotherapy. Curr Opin Pharmacol. 2017;35:1–11. doi:10.1016/j.coph.2017.04.006. PMID:28527911.
- Barrero MJ. Epigenetic Strategies to Boost Cancer Immunotherapies. J Mol Sci. 2017;18(6):1108. doi:10.3390/ijms18061108.
- Quandt D, Dieter Zucht H, Amann A, Wulf-Goldenberg A, Borrebaeck C, Cannarile M, Lambrechts D, Oberacher H, Garrett J, Nayak T, et al. Implementing liquid biopsies into clinical decision making for cancer immunotherapy. Oncotarget. 2017;8(29):48507–20. PMID:28501851.
- Yuan, J, Hegde PS, Clynes R, Foukas PG, Harari A, Kleen TO, Kvistborg P, Maccalli C, Maecker HT, Page DB, et al. Novel technologies and emerging biomarkers for personalized cancer immunotherapy. J Immunother Cancer. 2016;4:3. doi:10.1186/s40425-016-0107-3. PMID:26788324.
- Gnjatic S, Bronte V, Brunet LR, Butler MO, Disis ML, Galon J, Hakansson LG, Hanks BA, Karanikas V, Khleif SN, et al. Identifying baseline immune-related biomarkers to predict clinical outcome of immunotherapy. J Immunother Cancer. 2017;5:44. doi:10.1186/s40425-017-0243-4. PMID:28515944.
- Cogdill AP, Andrews MC, Wargo JA. Hallmarks of response to immune checkpoint blockade. Br J Cancer. 2017;117:1–7. doi:10.1038/bjc.2017.136. PMID:28524159.
- Milling L, Zhang Y, Irvine DJ. Delivering safer immunotherapies for cancer. Adv Drug Deliv Rev. 2017;114:79–101. PMID:28545888.
- Catani JPP, Riechelmann RP, Adjemian S, Strauss BE. Near future of tumor immunology: Anticipating resistance mechanisms to immunotherapies, a big challenge for clinical trials. Hum Vaccin Immunother. 2017;13:1109–11. doi:10.1080/21645515.2016.1269046. PMID:28059608.
- Ajay Dara ATS. Clearing the Fog of Anticancer Patents from 1993–2013_ Through an In-Depth Technology Landscape & Target Analysis from Pioneer Research Institutes and Universities Worldwide. PLOS ONE. 2014;9:e103847. doi:10.1371/journal.pone.0103847. PMID:25083710.
- Storz U. Intellectual property issues of immune checkpoint inhibitors. MAbs. 2016;8:10–26. doi:10.1080/19420862.2015.1107688. PMID:26466763.
- Collin M. Immune checkpoint inhibitors: A patent review (2010-2015). Expert Opin Ther Pat. 2016;26:555–64. doi:10.1080/13543776.2016.1176150. PMID:27054314.
- Kong X, Hu Y, Cai Z, Yang F, Zhang Q. Dendritic-cell-based technology landscape: Insights from patents and citation networks. Hum Vaccin Immunother. 2015;11:682–8. doi:10.1080/21645515.2015.1008857. PMID:25714961.
- Buonaguro FM, Tornesello ML, Izzo F, Buonaguro L. Oncolytic virus therapies. Pharm Pat Anal. 2012;1:621–7. doi:10.4155/ppa.12.65. PMID:24236929.
- Geng X, Kong X, Hu H, Chen J, Yang F, Liang H, Chen X, Hu Y. Research and development of therapeutic mAbs: An analysis based on pipeline projects. Hum Vaccin Immunother. 2015;11:2769–76. doi:10.1080/21645515.2015.1074362. PMID:26211701.
- Storz U. Antibody-drug conjugates: Intellectual property considerations. MAbs. 2015;7:989–1009. doi:10.1080/19420862.2015.1082019. PMID:26292154.
- Pardoll DM. Paracrine cytokine adjuvants in cancer immunotherapy. Annu Rev Immunol. 1995;13:399–415. doi:10.1146/annurev.iy.13.040195.002151. PMID:7612229.
- Salgaller ML, Lodge PA. Use of cellular and cytokine adjuvants in the immunotherapy of cancer. J Surg Oncol. 1998;68:122–38. doi:10.1002/(SICI)1096-9098(199806)68:2<122::AID-JSO10>3.0.CO;2-4. PMID:9624043.
- Egilmez NK, Kilinc MO, Gu T, Conway TF. Controlled-release particulate cytokine adjuvants for cancer therapy. Endocr Metab Immune Disord Drug Targets. 2007;7:266–70. doi:10.2174/187153007782794335. PMID:18220947.
- Garber K. China approves world's first oncolytic virus therapy for cancer treatment. J Natl Cancer Inst. 2006;98:298–300. doi:10.1093/jnci/djj111. PMID:16507823.
- Yu W, Fang H. Clinical trials with oncolytic adenovirus in China. Curr Cancer Drug Targets. 2007;7:141–8. doi:10.2174/156800907780058817. PMID:17346105.
- Rowan K. Oncolytic viruses move forward in clinical trials. J Natl Cancer Inst. 2010;102:590–5. doi:10.1093/jnci/djq165. PMID:20421567.
- Assessment of adenoviral vector safety and toxicity: Report of the National Institutes of Health Recombinant DNA Advisory Committee. Hum Gene Ther. 2002;13:3–13. doi:10.1089/10430340152712629. PMID:11779411.
- Kou Y-H, Chen C-L. Exploring the Business Opportunities in Cancer Immunotherapy (in Chinese). p. 137 (Development Center for Biotechnology; 2016.
- Squicciarini M, Dernis H, Criscuolo C. Measuring patent quality:indicators of technological and economic value. OECD Science, Technology and Industry Working Papers. 2013. doi:10.1787/5k4522wkw1r8-en
- Hall BH, Trajtenberg M. Uncovering GPTS with Patent Data NBER Working Papers No. 10901, 2004.