ABSTRACT
Some insoluble aluminum salts are commonly used in injectable vaccines as adjuvants to accelerate, prolong, or enhance the antigen-specific immune responses. Data from previous studies testing the nasal mucosal vaccine adjuvant activity of aluminum salts are conflicting. The present study is designed to further assess the feasibility of using aluminum salts in injectable vaccines as nasal mucosal vaccine adjuvants. Using Alhydrogel®, the international scientific standard of aluminum (oxy)hydroxide gels, and ovalbumin or 3 × M2e-HA2, a synthetic influenza virus fusion protein, as antigens, we showed in a mouse model that when dosed intranasally Alhydrogel® enables antigens adsorbed on it to induce stronger antigen-specific immune responses in both serum samples (e.g., specific IgG) and nasal and lung mucosal secretions (i.e., specific IgA) in all immunized mice, as compared with nasal immunization with the antigens alone. Rerouting insoluble aluminum salts in injectable vaccines may represent a viable approach for (nasal) mucosal vaccine adjuvant discovery.
Introduction
Appropriate vaccine adjuvants are often needed in new generation, protein subunit vaccines to enhance their immunogenicity.Citation1 Certain insoluble aluminum salts, e.g., aluminum (oxy)hydroxide and aluminum (hydroxy)phosphate, are commonly used in many human vaccines as adjuvants, with excellent safety profiles.Citation2,Citation3 Aluminum salt-adjuvanted human vaccines are injectables and administered by intramuscular, subcutaneous, or intradermal injection. There has been effort to test the feasibility of using aluminum salts as nasal vaccine adjuvants to help antigens induce immune responses. Data from a study by Butler et al. (1970) testing the nasal mucosal adjuvant activity of aluminum salts were discouraging, as they reported that although the nose drops of fluid diphtheria toxoid (DT) stimulated both nasal secretory and serum antibodies in adult male volunteers, the nose-drops of liquid Alum-precipitated DT failed to stimulate specific antibody production in nasal secretion and serum samples,Citation4 indicating that the aluminum salt in the Alum-precipitated DT even inhibited the immunogenicity of the DT. However, Isaka et al. (1998) reported that nasal aluminum-adsorbed tetanus toxoid (aTT) induced stronger anti-TT antibodies in mouse sera than nasal TT alone.Citation5 Importantly, anti-TT IgA, though weak, was also detected in the nasal secretion of 3 of the 5 mice nasally dosed with aTT.Citation5 Similarly, Isaka et al. (2001) also reported that nasal aluminum-adsorbed DT induced anti-DT IgA in the nasal secretion of 2 of the 5 immunized mice, and anti-DT IgG in the serum samples of all immunized mice.Citation6 Therefore, it appeared that the aluminum salts in the aluminum-adsorbed TT and the aluminum-adsorbed DT when given nasally helped the antigens adsorbed on them to induce antigen-specific systemic immune response, and potentially mucosal immune response as well. The present study is designed to further assess the nasal mucosal vaccine adjuvant activity of aluminum salts in a mouse model. The Alhydrogel® aqueous suspension (2%, w/v) was chosen as the aluminum salt adjuvant, as Brenntag Biosector's Alhydrogel® was elected as the international standard preparation for aluminum hydroxide gels.Citation7,Citation8 Known as an effective mucosal vaccine adjuvant,Citation9-19 monophosphoryl lipid A (MPLA) was chosen as a positive control for nasal mucosal vaccine adjuvant. Ovalbumin (OVA) and a recombinant influenza virus fusion protein (3 × M2e-HA2), comprised of influenza virus matrix 2 protein ectodomain peptides and a centralized influenza hemagglutinin stem region, were used as antigens.Citation20 It was showed that 3 × M2e-HA2-specific immune responses induced by 3 × M2e-HA2 adjuvanted with cholera toxin B subunit (CTB) (i.e., CTB chemically linked to 3 × M2e-HA2) are protective against multiple strains of influenza viruses.Citation20
Results and discussion
Certain insolule aluminum salts such as aluminum (oxy)hydroxide are commonly used in injectable human vaccines as adjuvants. Results from previous studies testing the feasibility of using aluminum salts as nasal vaccine adjuvants are contradictory.Citation4-6 While Butler et al.’s study in adult male volunteers showed that the aluminum salt in the nose-drops of Alum-precipitated diphtheria toxoid did not help the DT to stimulate specific antibodies in the nasal secretion or serum samples of the volunteers,Citation4 Isaka et al. (1998, 2001) reported that nasally administered aluminum-adsorbed tetanus toxoid (aTT) or aluminum-adsorbed DT induced antigen-specific antibodies (e.g., IgG) in the serum samples of all immunized mice, as well as specific secretory IgA in the nasal secretion of some of the immunized mice (i.e., 2 or 3 of the 5 immunized mice).Citation5,Citation6 Those studies used different antigens (i.e., DT vs. TT) in different models (i.e., adult male volunteers vs. mice), and the vaccines were prepared differently (i.e., aluminum-precipitated DT vs. aluminum-adsorbed DT or TT). It remains unclear which of the above differences was mainly responsible for the conflicting results reported.
In an effort to further assess the feasibility of using the traditional injectable aluminum salt-based vaccine adjuvants as nasal mucosal vaccine adjuvants, we used Alhydrogel® and 2 model antigens, OVA and 3 × M2e-HA2, a recombinant influenza virus fusion protein, and evaluated the specific antibody responses induced by the antigens in serum and nasal and lung secretory samples in a mouse model. The antigens were adsorbed on the surface of the Alhydrogel®, as aluminum salt-adjuvanted vaccines are commonly prepared by adsorbing protein antigens onto commercially available aluminum salt-based vaccine adjuvants such as Alhydrogel® or Adju-Phos®.Citation21 OVA was adsorbed on Alhydrogel® by mixing them at an Alhydrogel® to OVA weight ratio of 4:1. The adsorption efficiency of the OVA reached 100% when the weight ratio was 1:1 or above. However, the 4:1 weight ratio preparation was the most stable (data not shown). The 3 × M2e-HA2 was adsorbed onto the Alhydrogel® by mixing them at a weight ratio of 10:1 (Alhydrogel®:3 × M2e-HA2). The adsorption efficiency of 3xM2e-HA2 reaches ∼100% at this ratio. The size (X50, i.e., particle dimensions corresponding to 50% of the cumulative undersize distribution) of the OVA/Alhydrogel® particles in suspension was 12.93 ± 0.52 μm, and 10.01 ± 1.38 μm for the 3 × M2e-HA2/Alhydrogel®. The X50 value of the Alhydrogel® itself was 5.96 ± 0.34 μm. Data from many previous studies showed that MPLA has a strong nasal mucosal vaccine adjuvant activity.Citation10-19 Therefore, antigens mixed with MPLA were chosen as positive controls. OVA or 3 × M2e-HA2 was mixed with MPLA at a weight ratio of 1:1 and used to immunize mice. The particle size in the OVA/MPLA vaccine preparation was 207.9 ± 9.3 nm, and 211.9 ± 5.5 nm in the 3 × M2e -HA2/MPLA preparation. The mean diameter of the MPLA micelles (in an aqueous solution) was 202.3 ± 3.8 nm. Mice were immunized 3 times, 2 weeks apart, and the immune responses were measured 2 weeks after the last immunization.
Shown in and are the OVA-specific antibody levels (i.e., mucosal IgA and serum IgG1, IgG2a) in mice nasally immunized with OVA adjuvanted with Alhydrogel®. Although nasal OVA alone did not induce any significant OVA-specific IgA response in the nasal and lung secretions of the mice, nasal OVA/Alhydrogel® induced OVA-specific IgA responses in the nasal wash and the bronchoalveolar lavage (BAL) samples of all the immunized mice (). Nasal OVA/Alhydrogel® was also more immunogenic than nasal OVA alone in inducing OVA-specific serum IgG1 (). As expected, nasal OVA/MPLA was more immunogenic than nasal OVA alone, induced stronger OVA-specific serum IgG1 and IgG2a and OVA-specific IgA in nasal wash and BAL samples, as compared with nasal OVA alone (). shows the release of IL-4 and IFN-γ by splenocytes isolated from mice nasally immunized with OVA/Alhydrogel®, OVA/MPLA, or OVA alone after in vitro restimulation with OVA. As expected, the splenocytes from mice nasally immunized with OVA alone did not release any IL-4 or IFN-γ (). However, the splenocytes from mice nasally immunized with OVA/Alhydrogel® or OVA/MPLA released IL-4 (). Only the splenocytes from mice nasally immunized with OVA/MPLA released IFN-γ (), which is expected because MPLA is known to help boost type 1 T helper (Th1) response.Citation9,Citation22 Aluminum salts such as Alhydrogel® in injectable vaccines are known to only potentiate Th2 response. Based on our data in , it appears that Alhydrogel® also only potentiates Th2 response when dosed intranasally. In other words, changing the route of adiminstration from injection to intranasal dosing did not sigificantly change the type of immune responses induced by an aluminum salt-adjuvanted vaccine.
Figure 1. OVA-specific IgA levels in the nasal wash (A) and BAL samples (B) of mice intranasally immunized with OVA-adsorbed Alhydrogel®. Mice (n = 5) were dosed on days 0, 14, and 28 with OVA/Alhydrogel®, OVA/MPLA, OVA alone, or sterile PBS. The dose of OVA was 5 μg per mouse, 5 μg for MPLA, and 20 μg for Alhydrogel®. The anti-OVA IgA levels (OD450 values) in nose wash and BAL samples were measured 14 d after the third immunization (*p < 0.05, vs. OVA; #p < 0.05, OVA/Alhydrogel® vs. OVA/MPLA).
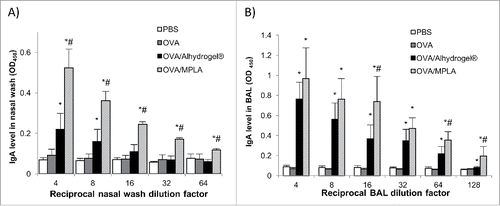
Figure 2. OVA-specific IgG1 (A) and IgG2a (B) levels in the sera of mice intranasally immunized with OVA-adsorbed Alhydrogel®. Mice (n = 5) were dosed on days 0, 14 and 28 with OVA/Alhydrogel®, OVA/MPLA, OVA alone, or sterile PBS. The dose of OVA was 5 μg per mouse, 5 μg for MPLA and 20 μg for Alhydrogel®. The anti-OVA IgG1 and IgG2a levels (OD450 values) in serum samples were measured 14 d after the third immunization (*p < 0.05, vs. OVA; #p < 0.05, OVA/Alhydrogel® vs. OVA/MPLA).
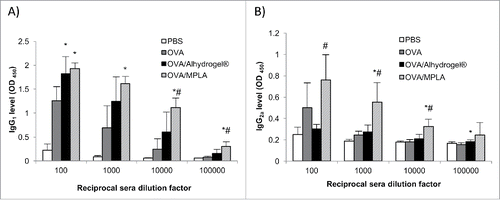
Figure 3. In vitro release of IL-4 and IFN-γ by splenocytes isolated from mice intranasally immunized with OVA-adsorbed Alhydrogel®. Mice (n = 5) were dosed on days 0, 14, and 28 with OVA/Alhydrogel®, OVA/MPLA, OVA alone, or sterile PBS. On day 42, splenocytes (2 × 106 cells/mL) isolated from individual mouse were stimulated with OVA (10 μg/mL) for 48 h. IL-4 and IFN-γ concentrations in cell culture medium were determined using ELISA kits (*p < 0.05, vs. OVA; #p < 0.05, OVA/MPLA vs. OVA/Alhydrogel®).
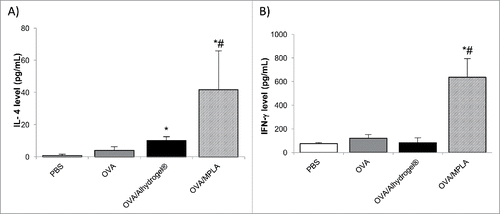
Shown in are the 3 × M2e-HA2-specific antibody levels (i.e., serum IgG and mucosal IgA) in mice intranasally immunized with 3 × M2e-HA2 adjuvanted with Alhydrogel®. Again, nasal 3 × M2e-HA2 alone was only weakly immunogenic, but nasal 3 × M2e-HA2/Alhydrogel® induced stronger specific serum IgG antibody response (p < 0.05, vs. 3 × M2e-HA2 alone), which was not significantly different from that induced by nasal 3 × M2e-HA2/MPLA (). Specific IgE was not detected in the serum samples of any of the immunized mice (data not shown), which is desirable, as IgE mediates a variety of allergic diseases.Citation23 Importantly, nasal 3 × M2e-HA2/Alhydrogel® also induced specific IgA responses in the nasal wash and the BAL samples of all the immunized mice (). In contrast, nasal 3 × M2e-HA2 alone did not induce any specific IgA response in the nasal secretion, and induced only a very weak IgA response in the lung secretion (i.e., BAL) (). Finally, the IgA levels induced by the 3 × M2e-HA2/Alhydrogel® in the nasal and lung secretions are not significantly different from that induced by nasal 3 × M2e-HA2/MPLA (). Clearly, nasal Alhydrogel® enabled 3 × M2e-HA2 to induce specific systemic and mucosal immune responses as well. However, different from the OVA/Alhydrogel® (), nasal 3 × M2e-HA2/Alhydrogel® was as effective as nasal 3 × M2e-HA2/MPLA in inducing 3 × M2e-HA2-specific IgA responses in mouse nasal wash and BAL samples (). It is noted that when the 3 × M2e-HA2 was used as the antigen, the dose of the Alhydrogel® was 50 μg/mouse, 20 μg/mouse when OVA was used as the antigen. The doses of the 3 × M2e-HA2 and OVA were both 5 μg/mouse.
Figure 4. Antigen (3 × M2e-HA2)-specific total IgG levels in the serum (A), and IgA levels in the nasal wash (B) and BAL (C) samples of mice intranasally immunized with 3 × M2e-HA2-adsorbed Alhydrogel®. Mice (n = 5) were dosed on days 0, 14 and 28 with 3 × M2e-HA2/Alhydrogel®, 3 × M2e-HA2/MPLA, 3 × M2e-HA2 alone or sterile PBS. The dose of 3 × M2e-HA2 was 5 μg per mouse, 5 μg for MPLA, and 50 μg for Alhydrogel®. The anti-3 × M2e-HA2 IgG (OD450 values) in the serum samples and IgA levels in the nose wash and BAL samples were measured 14 d after the third immunization (*p < 0.05, vs. 3 × M2e-HA2).
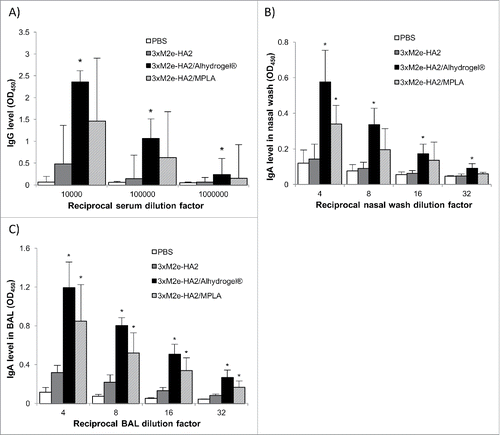
Taken together, using 2 different antigens, we showed that Alhydrogel®, an aluminum salt vaccine adjuvant commonly used in injectable vaccines, has nasal mucosal vaccine adjuvant activity. However, it appears that the relative systemic and mucosal adjuvant activity of the Alhydrogel® is to a certain extent affected by the antigen used. For example, when the influenza virus fusion protein antigen 3 × M2e-HA2 was used as antigen, nasal Alhydrogel® was largely as potent as nasal MPLA in potentiating specific immune responses. However, when OVA was used as antigen, nasal Alhydrogel® was not as potent as nasal MPLA. Of course, since the dose of Alhydrogel® used was 10-fold more than MPLA when 3 × M2e-HA2 was used as antigen, and only 4-fold more when OVA was used as antigen, the difference in the relative adjuvant activities observed may be in part due to the relative doses of the Alhydrogel® used in the immunization studies as well. It is known that the dose of adjuvant significantly affects the immune responses induced. In future studies, when aluminum salts such as Alhydrogel® are used as nasal mucosal vaccine adjuvants, the ratio of the aluminum salts to antigens in different vaccine preparations should be optimized. Nonetheless, our data strongly support that the traditional injectable aluminum (oxy)hydroxide adjuvant (i.e., Alhydrogel®) can be potentially used as a nasal mucosal vaccine adjuvant. Existing and new injectable vaccines adjuvanted with aluminum salts may be potentially administered intranasally to induce both systemic and mucosal immune responses. In fact, our preliminary data showed that intranasal and subcutaneous immunization of mice with the same 3 × M2e-HA2/Alhydrogel® both induced strong specific serum IgG responses, but specific secretory IgA response was only detected in the nasal wash and BAL samples of the intranasally immunized mice, not the subcutaneously immunized mice (data not shown), clearly demonstrating the advantages of nasal immunization.
Aluminum salt-containing adjuvants have close to a century of safety record. Local reactions such as redness, swelling and/or tenderness at the injection site are not infrequent,Citation24 but serious adverse effects attributable to aluminum salts are rare. Aluminum (oxy)hydroxide is poorly soluble in water (∼1 mg/L at 20oC), and thus when dispersed in water, aggregates to form 1–20 µm microparticles.Citation25 Data from a study by Flarend et al. (1997) indicated that all intramuscularly injected aluminum hydroxide may be slowly dissolved and absorbed eventually.Citation26,Citation27 Due to their large particle size (e.g., X50 of Alhydrogel®, 5.96 ± 0.34 μm), nasally dosed antigen-adsorbed insoluble aluminum salt particles are expected to largely remain in the nasal cavity, with limited deposition in the lung.Citation28,Citation29 There is the potential of brain exposure of aluminum to nasally administered aluminum salt-adjuvanted vaccines via the olfactory epithelium and olfactory bulb from the nasal aluminum salt adjuvant,Citation30 but Pauluhn (2009) reported that rats that were nose-only exposed to insoluble aluminum (oxy)hydroxide powders (particle size 25 or 40 μm as measured on the powder, primary powder size, 10 or 40 nm) for 4 weeks (5 d a week, 6 h a day, aluminum concentration as high as 28 mg/m3) did not show any significant change in brain aluminum level, as compared with rats that were not exposed to the aluminum (oxy)hydroxide.Citation31 In contrast, one month of continuous nasal exposure of rabbits to soluble aluminum in solutions, e.g., aluminum lactate or aluminum chloride (as ‘Gelfoam’), resulted in elevated aluminum in the brain.Citation32 Therefore, although experiments will need to be performed to confirm it, we suspect that the brain exposure to aluminum in nasally administrated insoluble aluminum salt-adjuvant vaccines will be limited, especially considering that during nasal immunization, a subject will be only transiently exposed to the insoluble aluminum salt in the vaccine for 1–3 times and at a dose significantly lower than in Pauluhn et al's study. Moreover, different from rodents, about 50% of whose nasal cavity is lined with olfactory epithelium, only 3% of the nasal cavity of primates and humans is lined with olfactory epithelium,Citation33 which will likely further limit nose to brain transport in humans, if any. However, although unlikely, it is possible that the nasal adjuvant activtiy of Alhydrogel® observed in mice may not be translatable to humans.
The mechanisms underlying the adjuvant activity of injectable aluminum salts are multi-fold.Citation34-40 For decades, it was thought to be the depot effect. Aluminum salts form an antigen depot at the site of injection, from where the antigens are slowly released.Citation34 It is now known that insoluble aluminum salts induce inflammation, thus recruiting and activating antigen-presenting cells (APCs) that capture antigens,Citation35 and the adsorption of soluble antigens on insoluble aluminum salt particles enables them to be more readily taken up by APCs.Citation35 Recent data also showed that the molecular target for the pro-inflammatory activity of aluminum salts is the NOD-like receptor protein 3 (NLRP3),Citation35-39 and activation of inflammasome leads to the production of proinflammatory cytokines. In addition, there are data showing that the induction of uric acid production is critical for the adjuvant activity of insoluble aluminum salts.Citation40 All the above mechanisms may be applicable to nasally administered insoluble aluminum salt-adjuvanted vaccines. The nasal mucosa has the nasopharynx-associated lymphoid tissue (NALT), which is a non-encapsulated lymophoid organ. NALT does not contain afferent lymphatics, but specialized cells in NALT may take up antigens that are released from the aluminum salt particles or antigen-adsorbed aluminum salt particles directly. The aluminum salt particles may facilitate the uptake of the antigens, activate inflammasome, and/or induce the production of uric acid, ultimately helping the antigens to induce specific mucosal and systemic immune responses.
In conclusion, using Alhydrogel® and 2 different protein antigens, we provide evidence in a mouse model that traditional injectable, insoluble aluminum salt-based vaccine adjuvants can be potentially used as nasal mucosal vaccine adjuvants.
Materials and methods
Materials
Alhydrogel®, a 2% (w/v) aqueous suspension of aluminum (oxy)hydroxide, was from InvivoGen (San Diego, CA). MPLA in a 1 mg/mL liquid solution was from Enzo Life Science (Farmingdale, NY). Recombinant influenza virus antigen 3 × M2e-HA2 was designed and purified as reported previously.Citation20 The endotoxin level from the 3 × M2e-HA2 in the final vaccine formulation was ∼3.57 EU/mL, measured using a ToxinSensor™ chromogenic limulus amebocyte lysate endotoxin assay kit from GenScript (Piscataway, NJ). OVA (from chicken egg white) and the 3,3’,5,5’-tetramethylbenzidine (TMB) substrate were from Sigma-Aldrich (St. Louis, MO). The BCA Protein Assay Kit was from ThermoFisher Scientific (Kansas, MO). Phosphate buffered saline (PBS), cell culture medium, antibiotics, and fetal bovine serum (FBS) were from Invitrogen (Carlsbad, CA). Horseradish peroxidase (HRP)-labeled, goat anti-mouse immunoglobulins (IgA, IgG, IgE, IgG1 and IgG2a) were from Southern Biotechnology Associates, Inc. (Birmingham, AL). Mouse IL-4 and IFN-γ ELISA kits were from BD Bioscience (San Jose, CA).
Vaccine formulations and immunization schedule
Animal studies were performed in accordance with the United States National Research Council Guide for the Care and Use of Laboratory Animals. The animal protocol was approved by the Institutional Animal Care and Use Committee at The University of Texas at Austin.
OVA as an antigen was adsorbed on Alhydrogel® by mixing them at a 1:4 ratio (OVA: Alhydrogel®, w/w) in normal saline and stirring at 4°C overnight. To determine the binding isotherm of OVA to Alhydrogel®, normal saline-diluted Alhydrogel® suspension and OVA were mixed to reach a final aluminum concentration of 0.25 mg/mL and OVA concentrations ranging from 0.025 to 4.5 mg/mL. After stirring for 16 h at 4°C, the adjuvant-protein mixtures in suspension were centrifuged at 14,000 g for 5 min at room temperature. The protein concentration in the supernatants was determined using the BCA Protein Assay kit. The amount of protein absorbed onto the Alhydrogel® was calculated by subtracting the amount of protein in the supernatant from the amount of the initially added protein. The adsorption efficiency of OVA to Alhydrogel® reached ∼100% when the Alhydrogel® to OVA weight ratio was equal or above 1:1 (data not shown). However, at the weight ratio of 4:1, the resultant OVA/Alhydrogel® preparation was most stable, having the least precipitation after 2 weeks of storage at 4oC (data not shown). BALB/c mice (6–8 weeks, female, Charles River Laboratories, Wilmington, MA) were randomized, lightly anesthetized with a ketamine and xylazine mixture, and then immunized intranasally by applying the OVA/Alhydrogel® liquid suspension to the nostrils of the mice using a fine pipet tip (20 μL total, 10 μL per nostril) 3 times, 2 weeks apart. Mice in control groups were intranasally dosed with OVA alone, OVA mixed with MPLA (OVA/MPLA), or left untreated. The doses of OVA, Alhydrogel®, and MPLA were 5, 20 and 5 μg/mouse/dose, respectively.
The 3 × M2e-HA2 antigen was adsorbed onto Alhydrogel® by mixing the protein in a normal saline solution and the Alhydrogel® in suspension at a 1:10 ratio (w/w) and stirring overnight at 4°C. The adsorption efficiency of 3 × M2e-HA2 to Alhydrogel® at such a ratio was determined to be 97.7 ± 0.1%. BALB/c mice (6–8 weeks, female) were immunized intranasally with the 3 × M2e-HA2/Alhydrogel® in suspension 3 times, 2 weeks apart. Mice in the control groups were intranasally dosed with 3 × M2e-HA2 alone, 3 × M2e-HA2 mixed with MPLA (3 × M2e-HA2/MPLA), or left untreated. The doses of the 3 × M2e-HA2, Alhydrogel®, and MPLA were 5, 50, and 5 μg/mouse/dose, respectively.
The size and size distribution of the Alhydrogel® and the antigen-adsorbed Alhydrogel® were measured using a Sympatec HELOS laser diffraction instrument equipped with a R3 lens (Sympatec GmbH, Germany). Antigen was mixed with MPLA at a 1:1 ratio (w/w) in normal saline and stirring at 4°C overnight, and the particle size and size distribution of the MPLA and antigen/MPLA were determined using a Malvern Zeta Sizer Nano ZS (Westborough, MA). In both animal studies, mice were killed 2 weeks after the last immunization to collect blood and spleen. Nasal wash and BAL samples were collected as described previously using 500 μL of sterile PBS.Citation41 Blood samples were centrifuged to collect sera. Spleens were used to prepare splenocyte suspensions from individual mouse as described previously.Citation42
Enzyme-linked immunosorbent assay (ELISA)
ELISA was performed as described previously.Citation43 Briefly, 96-wells ELISA plates were coated with 3 × M2e-HA2 or OVA (1 μg/mL) in 100 μL of 50 mM sodium bicarbonate buffer (pH 9.0) overnight at 4°C. Plates were then blocked with PBS containing 5% of horse serum albumin (blocking buffer) for 1 h at 37°C. Serum samples (100 μL) diluted with blocking buffer were added and incubated for 2 h at 37°C. Similarly, nasal wash and BAL samples (100 μL) diluted with blocking buffer were added and incubated for 2 h at 37°C. After washing with PBS-Tween 20, anti-mouse IgG, IgA, IgE, IgG1 or IgG2a diluted 5,000-fold in blocking buffer were then added into each well, and the plates were incubated for 1 h at room temperature. The plates were washed, and 100 μL TMB substrate was added. The reaction was stopped after 5 min with 50 μL H2SO4 stop solution. Plates were read at 450 nm using a BioTek Synergy HT Multi-Mode Microplate Reader (Winooski, VT).
Cytokine release from splenocytes after in vitro stimulation
Splenocytes (2 × 106 cells/mL) from individual mouse were cultured in RPMI-1640 media with 10% FBS (v/v), 100 U/mL penicillin, 100 μg/mL streptomycin, and 50 μM β-mercaptoethanol in the presence or absence of OVA (10 μg/mL) at 37oC, 5% CO2. For cytokine release assay, IL-4 and IFN-γ levels in culture media were measured using ELISA kits after 48 h of incubation.
Statistical analysis
Statistical analyses were conducted using analysis of variance (ANOVA) followed by Fischer's protected least significant difference procedure. A p-value of ≤ 0.05 (2-tail) was considered significant.
Disclosure of potential conflicts of interest
No potential conflicts of interest were disclosed.
Funding
This work was supported in part by research grants from the US. National Institute of Allergy and Infectious Diseases (AI105789 to ZC and AI072139 to MZ) and the Alfred and Dorothy Mannino Fellowship in Pharmacy at UT Austin (to ZC). ZC's work is also supported by the National Natural Science Foundation of China (81460454) and the Inner Mongolia Natural Science Fund (2014ZD05). SGT was supported in part by a Graduate Fellowship from UT Austin.
References
- Mbow ML, De Gregorio E, Valiante NM, Rappuoli R. New adjuvants for human vaccines. Curr Opin Immunol. 2010;22:411-6. PMID:20466528
- Bomford R. The comparative selectivity of adjuvants for humoral and cell-mediated immunity. I. Effect on the antibody response to bovine serum albumin and sheep red blood cells of Freund's incomplete and complete adjuvants, alhydrogel, Corynebacterium parvum, Bordetella pertussis, muramyl dipeptide and saponin. Clin Exp Immunol. 1980;39:426-34. PMID:6248282
- Harris JR, Soliakov A, Lewis RJ, Depoix F, Watkinson A, Lakey JH. Alhydrogel(R) adjuvant, ultrasonic dispersion and protein binding: a TEM and analytical study. Micron. 2012;43:192-200. PMID:21831642
- Butler WT, Rossen RD, Wenden RD. Effect of physical state and route of inoculation of diphtheria toxoid on the formation of nasal secretory and serum antibodies in man. J Immunol. 1970;104:1396-400. PMID:5463220
- Isaka M, Yasuda Y, Kozuka S, Miura Y, Taniguchi T, Matano K, Goto N, Tochikubo K. Systemic and mucosal immune responses of mice to aluminium-adsorbed or aluminium-non-adsorbed tetanus toxoid administered intranasally with recombinant cholera toxin B subunit. Vaccine. 1998;16:1620-6. PMID:9713937
- Isaka M, Yasuda Y, Taniguchi T, Kozuka S, Matano K, Maeyama J, Morokuma K, Ohkuma K, Goto N, Tochikubo K. Comparison of systemic and mucosal responses of mice to aluminium-adsorbed diphtheria toxoid between intranasal administration and subcutaneous injection. Nagoya Med J. 2001;45:5-15
- Stewart-Tull DES. Recommendations for the Assessment of Adjuvants (Immunopotentiators). In: Gregoriadis G, Allison AC, Poste G, eds. Immunological adjuvants and vaccines. Boston, MA: Springer US, 1989:213-26
- Stewart-Tull DES. The assessment and use of adjuvants. In: Gregoriadis G, Allison AC, Poste G, eds. Vaccines: Recent trends and progress. Boston, MA: Springer US, 1991:85-92
- Casella CR, Mitchell TC. Putting endotoxin to work for us: monophosphoryl lipid A as a safe and effective vaccine adjuvant. Cell Mol Life Sci. 2008;65:3231-40. PMID:18668203
- Childers NK, Miller KL, Tong G, Llarena JC, Greenway T, Ulrich JT, Michalek SM. Adjuvant activity of monophosphoryl lipid a for nasal and oral immunization with soluble or liposome-associated antigen. Infect Immun. 2000;68:5509-16. PMID:10992447
- Sasaki S, Hamajima K, Fukushima J, Ihata A, Ishii N, Gorai I, Hirahara F, Mohri H, Okuda K. Comparison of intranasal and intramuscular immunization against human immunodeficiency virus Type 1 with a DNA-Monophosphoryl lipid A adjuvant vaccine. Infect Immun. 1998;66:823-6. PMID:9453648
- Kamphuis T, Shafique M, Meijerhof T, Stegmann T, Wilschut J, de Haan A. Efficacy and safety of an intranasal virosomal respiratory syncytial virus vaccine adjuvanted with monophosphoryl lipid A in mice and cotton rats. Vaccine. 2013;31:2169-76. doi:10.1016/j.vaccine.2013.02.043. PMID:23499594
- Baldridge JR, Yorgensen Y, Ward JR, Ulrich JT. Monophosphoryl lipid A enhances mucosal and systemic immunity to vaccine antigens following intranasal administration. Vaccine. 2000;18:2416-25. doi:10.1016/S0264-410X(99)00572-1. PMID:10738099
- Nathaniel FP, Sacci JB, Alving CR, Richardson EC. Enhancement by lipid A of mucosal immunogenicity of liposome-associated cholera toxin. Rev Infect Dis. 1984;6:563-6. doi:10.1093/clinids/6.4.563. PMID:6474016
- Blanco JCG, Boukhvalova MS, Pletneva LM, Shirey KA, Vogel SN. A recombinant anchorless respiratory syncytial virus (RSV) fusion (F) protein/monophosphoryl lipid A (MPL) vaccine protects against RSV-induced replication and lung pathology. Vaccine. 2014;32:1495-500. doi:10.1016/j.vaccine.2013.11.032. PMID:24252693
- Couch RB, Atmar RL, Cate TR, Quarles JM, Keitel WA, Arden NH, Wells J, Niño D, Wyde PR. Contrasting effects of type I interferon as a mucosal adjuvant for influenza vaccine in mice and humans. Vaccine. 2009;27:5344-8. doi:10.1016/j.vaccine.2009.06.084. PMID:19607949
- Hirano T, Kodama S, Kawano T, Maeda K, Suzuki M. Monophosphoryl lipid A induced innate immune responses via TLR4 to enhance clearance of nontypeable Haemophilus influenzae and Moraxella catarrhalis from the nasopharynx in mice. FEMS Immunol Med Microbiol. 2011;63:407-17. doi:10.1111/j.1574-695X.2011.00866.x. PMID:22092567
- Uddowla S, Freytag LC, Clements JD. Effect of adjuvants and route of immunizations on the immune response to recombinant plague antigens. Vaccine. 2007;25:7984-93. doi:10.1016/j.vaccine.2007.09.030. PMID:17933440
- VanCott TC, Kaminski RW, Mascola JR, Kalyanaraman VS, Wassef NM, Alving CR, Ulrich JT, Lowell GH, Birx DL. HIV-1 neutralizing antibodies in the genital and respiratory tracts of mice intranasally immunized with oligomeric gp160. J. Immunol. 1998;160:2000-12. PMID:9469464
- Li J, Arevalo MT, Chen Y, Posadas O, Smith JA, Zeng M. Intranasal immunization with influenza antigens conjugated with cholera toxin subunit B stimulates broad spectrum immunity against influenza viruses. Hum Vaccin Immunother. 2014;10:1211-20. doi:10.4161/hv.28407. PMID:24632749
- Thakkar SG, Cui Z. Methods to Prepare Aluminum Salt-Adjuvanted Vaccines. Methods Mol Biol. 2017;1494:181-99. doi:10.1007/978-1-4939-6445-1_13. PMID:27718194
- Drachenberg KJ, Wheeler AW, Stuebner P, Horak F. A well‐tolerated grass pollen‐specific allergy vaccine containing#8232;a novel adjuvant, monophosphoryl lipid A, reduces allergic symptoms after only four preseasonal injections. Allergy. 2001;56:498-505. doi:10.1034/j.1398-9995.2001.056006498.x. PMID:11421893
- Platts-Mills TA, Schuyler AJ, Erwin EA, Commins SP, Woodfolk JA. IgE in the diagnosis and treatment of allergic disease. J. Allergy Clin Immunol. 2016;137:1662-70. doi:10.1016/j.jaci.2016.04.010. PMID:27264001
- Eickhoff TC, Myers M. Workshop summary. Aluminum in vaccines. Vaccine. 2002;20(Suppl 3):S1-4. doi:10.1016/S0264-410X(02)00163-9. PMID:12184358
- Hem SL, Hogenesch H. Relationship between physical and chemical properties of aluminum-containing adjuvants and immunopotentiation. Expert Rev Vaccines. 2007;6:685-98. doi:10.1586/14760584.6.5.685. PMID:17931150
- Flarend RE, Hem SL, White JL, Elmore D, Suckow MA, Rudy AC, Dandashli EA. In vivo absorption of aluminium-containing vaccine adjuvants using 26Al. Vaccine. 1997;15:1314-8. doi:10.1016/S0264-410X(97)00041-8. PMID:9302736
- Hem SL. Elimination of aluminum adjuvants. Vaccine. 2002;20(Suppl 3):S40-3. doi:10.1016/S0264-410X(02)00170-6. PMID:12184363
- Stuart BO. Deposition of inhaled aerosols. Arch Intern Med. 1973;131:60-73. doi:10.1001/archinte.1973.00320070056006. PMID:4569374
- Hatch TF. Distribution and deposition of inhaled particles in respiratory tract. Bacteriol Rev. 1961;25:237. PMID:13905321
- Djupesland PG, Mahmoud RA, Messina JC. Accessing the brain: the nose may know the way. J Cereb Blood Flow Metab. 2013;33:793-4. doi:10.1038/jcbfm.2013.41. PMID:23486291
- Pauluhn J. Pulmonary toxicity and fate of agglomerated 10 and 40 nm aluminum oxyhydroxides following 4-week inhalation exposure of rats: toxic effects are determined by agglomerated, not primary particle size. Toxicol Sci. 2009;109:152-67. doi:10.1093/toxsci/kfp046. PMID:19251949
- Perl DP, Good PF. Uptake of aluminium into central nervous system along nasal-olfactory pathways. Lancet. 1987;1:1028. doi:10.1016/S0140-6736(87)92288-4. PMID:2883359
- Harkema JR, Carey SA, Wagner JG. The nose revisited: A brief review of the comparative structure, function, and toxicologic pathology of the nasal epithelium. Toxicol Pathol. 2006;34:252-69. doi:10.1080/01926230600713475. PMID:16698724
- Glenny AT, Buttle, AH, Stevens MF. Rate of disappearance of diphtheria toxoid injected into rabbits and guinea pigs:toxoid precipitated with alum. J Pathol Bacteriol. 1931;34:267-75. doi:10.1002/path.1700340214.
- Tritto E, Mosca F, De Gregorio E. Mechanism of action of licensed vaccine adjuvants. Vaccine. 2009;27:3331-4. doi:10.1016/j.vaccine.2009.01.084. PMID:19200813
- Eisenbarth SC, Colegio OR, O'Connor W, Sutterwala FS, Flavell RA. Crucial role for the Nalp3 inflammasome in the immunostimulatory properties of aluminium adjuvants. Nature. 2008;453:1122-6. doi:10.1038/nature06939. PMID:18496530
- Franchi L, Nunez G. The Nlrp3 inflammasome is critical for aluminium hydroxide-mediated IL-1beta secretion but dispensable for adjuvant activity. Eur J Immunol. 2008;38:2085-9. doi:10.1002/eji.200838549. PMID:18624356
- Kool M, Petrilli V, De Smedt T, Rolaz A, Hammad H, van Nimwegen M, Bergen IM, Castillo R, Lambrecht BN, Tschopp J. Cutting edge: alum adjuvant stimulates inflammatory dendritic cells through activation of the NALP3 inflammasome. J Immunol. 2008;181:3755-9. doi:10.4049/jimmunol.181.6.3755. PMID:18768827
- Hornung V, Bauernfeind F, Halle A, Samstad EO, Kono H, Rock KL, Fitzgerald KA, Latz E. Silica crystals and aluminum salts activate the NALP3 inflammasome through phagosomal destabilization. Nat Immunol. 2008;9:847-56. doi:10.1038/ni.1631. PMID:18604214
- Kool M, Soullie T, van Nimwegen M, Willart MA, Muskens F, Jung S, Hoogsteden HC, Hammad H, Lambrecht BN. Alum adjuvant boosts adaptive immunity by inducing uric acid and activating inflammatory dendritic cells. J Exp Med. 2008;205:869-82. doi:10.1084/jem.20071087. PMID:18362170
- Tochikubo K, Isaka M, Yasuda Y, Kozuka S, Matano K, Miura Y, Taniguchi T. Recombinant cholera toxin B subunit acts as an adjuvant for the mucosal and systemic responses of mice to mucosally co-administered bovine serum albumin. Vaccine. 1998;16:150-5. doi:10.1016/S0264-410X(97)00194-1. PMID:9607023
- Stagg AJ, Burke F, Hill S, Knight SC. Isolation of mouse spleen dendritic cells. Dendritic Cell Protocols. 2001;64:9-22. doi:10.1385/1-59259-150-7:9.
- Bungener L, Geeraedts F, ter Veer W, Medema J, Wilschut J, Huckriede A. Alum boosts TH2-type antibody responses to whole-inactivated virus influenza vaccine in mice but does not confer superior protection. Vaccine. 2008;26:2350-9. doi:10.1016/j.vaccine.2008.02.063. PMID:18400340