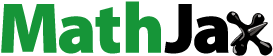
ABSTRACT
While broadly neutralizing antibodies (bnAbs) are a promising preventative and therapeutic tool for HIV infection, production is difficult and expensive. Production of antibody-like fragments in bacterial cytoplasm provides a cheaper alternative. This work explored the transplantation of the complementarity determining regions of the anti-HIV bnAbs PGT121 and 10E8 onto a single-chain variable fragment (scFv) scaffold, previously discovered through a novel screening platform. The scaffolded 10E8 scFv, but not the scaffolded PGT121 scFv, was soluble in bacterial cytoplasm, enabling efficient production in bacteria. Three additional multimeric constructs employing the scaffolded 10E8 scFv were also generated and soluble versions produced in bacteria. However, the constructs were found to have substantially lost anti-HIV binding function and had completely abrogated neutralizing activity. Overall, while this study provides a proof-of-concept for anti-HIV bnAb construct production in bacterial cytoplasm, future refinement of these technologies will be required to realize the goal of producing inexpensive and effective bnAb-like tools for the control of HIV.
Introduction
Current ART is effective, but requires daily administration and remains unaffordable for many communities worldwide (UNAIDS, 2016). Cheaper, safe and effective interventions are crucial to both treat more patients and help reduce HIV transmission. Broadly neutralizing antibodies (bnAbs) with potent activity against multiple different strains have the potential to become an important biomedical tool in HIV control.Citation1-6 Furthermore, some macaques receiving bnAbs exhibit improved control of Simian-Human Immunodeficiency virus infection after circulating Ab levels wane.Citation5,Citation7 Small-scale human studies of delivering bnAbs to HIV-infected subjects have produced similar results.Citation8-11 Due to the promising nature of bnAbs, larger scale clinical trials for the therapeutic use of VRC01 in patients receiving ART are currently underwayCitation11 as well as a large 5400-subject efficacy trial for VRC01 as a preventative in uninfected individuals (ClinicalTrials.gov ID: NCT02716675).
While the use of bnAb interventions is gaining momentum, the cost of widespread use as a preventative or treatment for HIV, especially in low-resource settings, may be prohibitive. Estimates suggest that the production cost of Abs may be in the order of $78-$300/gram, although these costs may reduce over time.Citation12,Citation13 The high cost is largely a result of the substantial bnAb production costs in mammalian cell lines and expensive downstream processing required for purification.Citation14
To reduce costs of bnAb production, new platforms for production are being explored. Other eukaryotic platforms, such as plantCitation15,Citation16 or yeast cellsCitation17 are often cheaper to grow and maintain than mammalian systems. Cheaper still is the alternative provided by rapidly-growing prokaryotic bacteria. Recent technologies allow the production of Abs in the periplasmic space of gram-negative bacteria, such as Escherichia coli, which is an oxidizing environment more suited to Ab folding and disulphide bond formation than the harsh environment of bacterial cytoplasm.Citation18,Citation19 However, this creates problems in yield since export of Ab products to the periplasm through the inner membrane is a rate-limiting step.Citation20
A major difficulty with bacterial cytoplasmic production of mAbs is the severely reducing environment.Citation21 Full length IgG Abs produced in bacterial cytoplasm cannot form disulphide bonds and are usually insoluble within bacteria. To circumvent the issue of disulphide bonds required for IgG molecules, Ab constructs such as single-chain variable fragment (scFv) Ab constructs can be produced.Citation22,Citation23 These fragments lack the constant region (Fc) of full-length Abs, but still bind to antigens through their complementarity determining regions (CDRs). ScFv constructs are able to mediate neutralization functions against viruses like HIVCitation24,Citation25 and are a potential tool in the control of the pandemic.
ScFv molecules can also potentially resolve the solubility issues that limit the production of IgG molecules in bacteria. A novel cytoplasmic display platform, termed “Retained Display” (ReD), was previously developed in order to select fully-human scFv that were both stable and soluble in the bacterial cytoplasm.Citation26 The human germline heavy chain gene IGHV3-23 formed the basis for all constructs in the screen, due to its intrinsic stability.Citation27,Citation28 IGHV3-23 was then fused with numerous light chain families in order to produce scFv in combinations that remained soluble in bacterial cytoplasm.Citation26 The most common useful pairing was a IGHV3-23 and IGLV3-1 fusion. Previous screens demonstrated that scFvs based on a IGHV3-23 and IGLV3-1 fusion tolerated CDR3 diversificationCitation26, suggesting it may be a good candidate to act as a scaffold for the transplantation of CDR sequences of known bnAbs. We aimed to determine if the scFv made from the fusion of IGHV3-23 and IGLV3-1 could be used to produce an scFv with the CDRs of 2 well-characterized and potent anti-HIV bnAbs termed 10E8Citation29 and PGT121.Citation30
The single Fv arm of scFvs reduces the stability of the interaction in comparison to standard antibodies with two arms for antigen interaction and may result in some loss of scFv potency. To help mitigate this, scFvs can be combined together to make diabodies or tandem scFv. Diabodies have short linkers (3-12 amino acids) that force the two scFv to dimerize and stabilize.Citation31 Diabodies have been shown to have higher affinities than scFv, suggesting improved binding.Citation32,Citation33 Tandem scFv, in contrast, have longer linkers that allow for more flexibility during binding. Both diabodies and tandem scFv can be produced with two identical scFvs, producing monospecifics, or with different scFvs, producing bispecifics.Citation34 Both monospecifics and bispecifics are designed to improve avidity over that of scFvs by increasing the number of binding sites. However, bispecifics are adaptable and can also employ a second non-neutralizing scFv arm to help stabilize binding.
In this study, the IGHV3-23 and IGLV3-1 fusion scFv previously identified by ReD screeningCitation26 underwent CDR transplantation in order to produce soluble scFvs with the HIV-binding capacity of the bnAbs PGT121 and 10E8. Ultimately, 4 different 10E8 scFv structures, soluble in bacterial cytoplasm, were generated: a simple scaffolded 10E8 scFv, a monospecific 10E8 diabody (10E8-10E8) and two bispecifics involving the scaffolded 10E8 scFv were also produced. One bispecific diabody employed 10E8 and A12, an anti-HIV VHH camelid antibodyCitation35,Citation36 (10E8-A12) and the other was a bispecific diabody containing a novel non-neutralizing, strongly binding anti-HIV scFv known as Ab49 (10E8-Ab49). All constructs were then tested in vitro for their ability to bind a broad range of HIV envelope antigens and for their ability to neutralize HIV.
Results
Design of bnAb scFvs constructs for bacterial production
Selection of potential bnAb candidates for expression in the E. coli system was based on CDR binding profiles of individual Abs. Scaffolded constructs involved the transplanting of bnAb CDRs onto a heavy-light chain scFv fusion (IGHV3-23 and IGLV3-1) previously shown to be soluble in E. coli cytoplasm.Citation26 108E and PGT121 were chosen as candidates for two reasons. 1) These bnAbs are well characterized as being broad and potent, respectively and 2) they are predicted to bind to their epitopes only through CDR residues.
Scaffolded scFv fusion involved the transplantation of CDR residues only in an effort to maintain solubility by minimising alterations to the IGHV3-23 and IGLV3-1 chains. Thus only bnAbs that bound only through their CDR were considered. Examination of structural databases and previous studies determining bnAb-binding profiles suggested that 10E8 and PGT121 were potentially appropriate candidates. Unlike bnAbs such as VRC01Citation37, these bnAbs only bind through their CDR.Citation29,Citation30 shows VRC01 (top) binding to its antigen, with the binding residues located in the CDR highlighted in yellow. However, there are also non-CDR residues highlighted in green that are necessary for epitope recognition. In contrast, 10E8 (middle) and PGT121 (bottom) have all their binding residues (highlighted in yellow) located in the CDR.
Figure 1. (A) Detailed structure of VRC01 Ab (heavy chain in marron, light chain in blue) in complex with HIV-1 gp120Citation37 (purple). Residues bind the antigen both with the CDR (yellow) and outside of the CDR (green). (B) 10E8 Fab in complex with an HIV-1 gp41 peptideCitation29 (purple). All antigen-binding residues are within the CDR (yellow). (C) detailed structure of PGT121 Fab.Citation30 Resides that bind the Env antigen (yellow) are all within the CDR. Images were generated from the NCBI Structure database (MMDB ID: 83230, 103370, and 105068 respectively) using Swiss PDB viewer version 4.1.
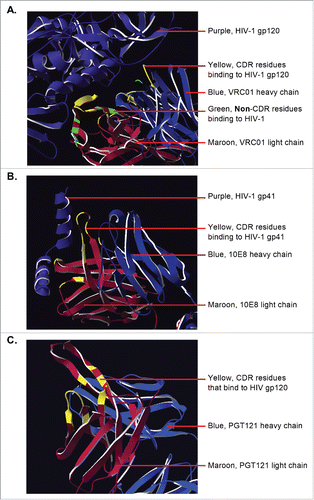
Furthermore, the heavy chain of 10E8 has high sequence identity with the IGHV3-23 heavy chain scaffold. This suggests that a construct built with this scaffold would both maintain solubility in bacterial cytoplasm, and recapitulate much of the WT 10E8 sequence. Changes were made to the scaffold, both to transplant the CDR onto the IGHV3-23 and IGLV3-1 and to make changes outside the CDR region to make the scaffold more reflective of WT 10E8. After the changes to the scaffold, the heavy chain of the scaffolded 10E8 scFv had 86.2% amino acid sequence identity with WT 10E8 and the light chain had 85.6% identity (, ).
Figure 2. Alignment of WT bnAb amino acid sequence with the sequence of the scaffold after CDR transplantation. Shown are the light chains and heavy chains that make up the scFv structures only, without the other domains listed in . (A) Shows the 10E8 heavy chain, (B) shows the 10E8 light chain. (C) Shows the PGT121 heavy chain, (B) shows the PGT121 light chain. The red boxes indicate the CDR sequence. Alignments were performed using ExPASy LALIGN software available from the SIB Bioinformatics Resource Portal. WT bnAb sequences were taken from Mouquet et al. for PGT121 (PDB: 4FQ1_L and 4FQ1_H)Citation30 and Huang et al. for 10E8 (Genbank AFS68396.1 and AFS68395.1).Citation29
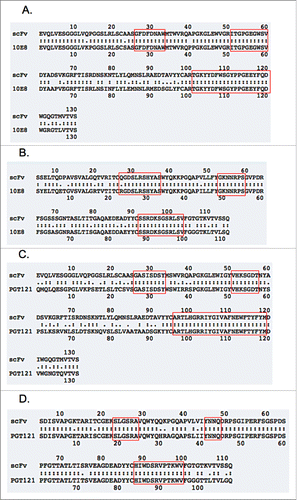
PGT121 was considered a more challenging candidate due to the substantial differences between its heavy chain and the scaffold IGHV3-23 that was used. The PGT121 scFv had only 68.7% amino acid sequence identity with WT PGT121, though the light chain had 86.9% identity (, ).
A number of useful domains were included as ways of simplifying purification (His6 tag), checking expression levels (FLAG tag and Biotinylation site) and increasing in vivo half-life (a G148-GA3 albumin binding domainCitation38). The domains and their functions are outlined in .
Figure 3. Schematic of bnAb scFv construct with useful domains indicated. The heavy (VH) and light chain (VL) scFv domains are shown in purple and orange. The His6 domain for purification is shown in yellow. The FLAG tag (amino acid sequence DYKDDDK) in light blue is used to check for expression, as is the biotinylation shown in dark blue used for detection by streptavidin/TMB reactions in ELISAs. The albumin-binding domain (purple) is for improved half-life in vivo.
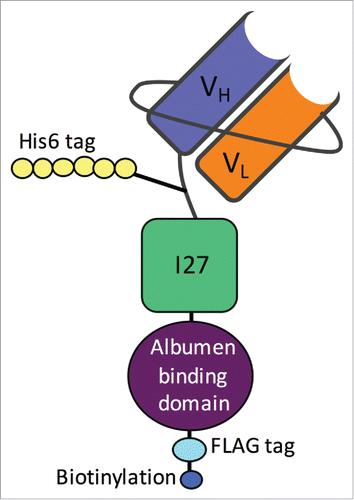
Expression and solubility of 10E8 and PGT121 constructs
Expressing the scaffolded 10E8 scFv in bacteria was successful. It was readily purified from the soluble fraction of the E. coli supernatant (). In contrast, scaffolded PGT121 scFv was problematic and initial attempts to express it were unsuccessful. As indicated by the SDS-gel, no PGT121 scFv is detected in the either the soluble or insoluble fractions of the elution usiing the pRhn vector (). Changing the expression vector of scaffolded PGT121 to the pAra5 vector led to some PGT121 expression, but the construct was only visualized in the insoluble fraction of the elution. Once again there was no trace of its presence in the soluble fraction, indicating that the PGT121 scFv as designed was insoluble (). In an attempt to improve expression of soluble PGT121 scFV, we restored the naturally truncated light chain of PGT121 to the germline sequence since this technique was previously shown not to interfere with neutralization function.Citation39 However, this modification did not make PGT121 soluble in this expression system ().
Figure 4. SDS PAGE gels showing expression of 10E8 and PGT121 constructs. (A) Initial attempts to isolate scFv proteins from bacteria carrying the PGT121 and 10E8 geneblocks in a pRhn vector. ScFvs were eluted from columns during purification and the soluable fraction from two separate PGT121 preperations were run in lanes 1and 2 (expected size 44kDa), and the insoluble fractions from these respective preprations were run in lanes 3 and 4. Lane 5 and 6 shows the soluble fractions of two scaffolded 10E8 scFv preperations (expected size 54kDa); and column 7 shows insoluble the insolubale fraction of a scaffolded 10E8 scFv prepration. (B) PGT121 expression sequence geneblocks were moved into a new pAra5 vector and attempts to isolate PGT121 scFv were undertaken. Column 1 shows the soluble fraction of the initial truncated PGT121 scFv preperation (expected size 44kDa), 2 the insoluble fraction of a truncated PGT121 scFv preperation, 3 shows the soluble fraction of a restored PGT121 scFv preperation (expected size ∼46kDa), and 4 shows the insoluble fraction of a restored PGT121 scFv preperation. (C) Expression of the 10E8-A12 construct (lane 2, expected size 94kDa), the 10E8-10E8 construct (Lane 3, expected size 107kDa) and the 10E8-Ab49 construct (Lane 3, expected size 106.6kDa). Arrows indicate the relevant bands and their expected sizes. The far left column of all gels indicates the molecular weight marker used with its ladder band sizes given in kDa.
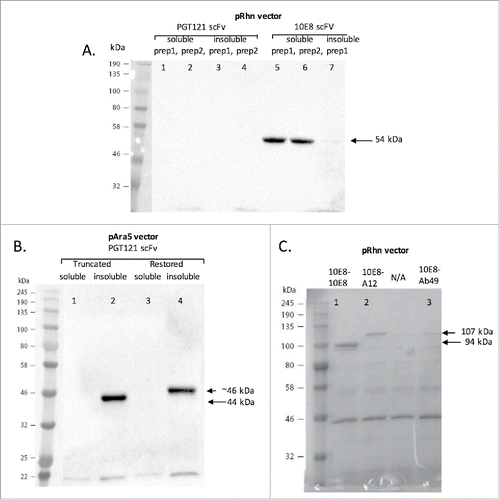
Since the scaffolded 10E8 scFv expressed well, we focused on generating additional monospecific and bispecific 10E8 constructs, shown diagrammatically in . Bispecific- and diabody-based have the potential to improve binding efficiency, which may translate to improved in vivo efficacy.Citation33,Citation40 The additional constructs made were (1) a monospecific 10E8 diabody (), (2) a tandem bispecific scFvs 10E8-Ab49 (Ab49 is an anti-HIV binding antibody, ), (3) a tandem bispecific scFv 10E8-A12 (A12 is a camelid anti-HIV neutralizing domain antibody, ). A control Ab49 scFv alone was also produced (). shows the expression of the 10E8 diabody and the10E8-A12 bispecific. The 10E8-Ab49 bispecific, and the Ab49 scFv as a control, were also successfully expressed (data not shown). Sufficient quantities of all soluble constructs (0.2-1mg of each construct) were readily produced for testing in both binding and neutralizing assays, as expected for a bacterial cytoplasmic platform.
Figure 5. Schematic of all bnAb scFv constructs. In all diagrams, light purple domains represent the 10E8 heavy chain, while dark purple indicates the 10E8 light chain. Dark blue and gold indicate the heavy and light chains of Ab49, respectively. Green represents the A12 VHH. (A) Shows thescaffolded 10E8 scFv, (B) the 10E8-10E8 diabody (C) the 10E8-Ab49 tandem scFv (D) the 10E8-A12 tandem scFv and (E) shows the Ab49 scFv control. The highest dilution of each Ab constructs in weight amounts are as follows: control Abs at 3.75ng, 10E8 scFv at 1.5ng, 10E8-10E8 at 2.35ng, 10E8-A12 at 6.68ng, 10E8-Ab49 at 2.67ng and the Ab49 scFv at 1.5ng.
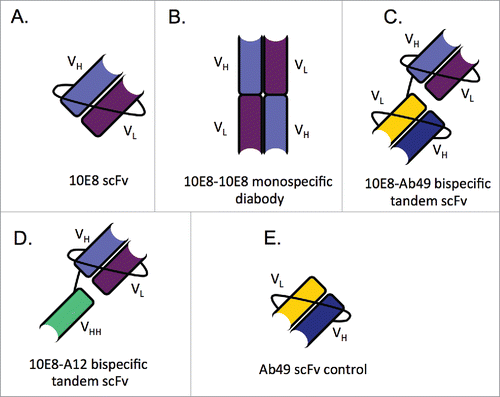
Binding of 10E8 constructs to different gp140 antigens
To determine whether the scaffolded 10E8-based scFv and diabody constructs retained their binding function against HIV envelope antigens, a series of ELISAs were performed. To assess the efficiency and breadth of the 10E8 constructs seven strains of HIV-1 gp140 across six different clades were evaluated, alongside whole HIV-1MN (a clade B strain) virion preparation inactivated with Aldrithiol-2 (AT-2)Citation41 abbreviated to WIV, since this antigen reflects a natural conformation of Env on the virion surface. Full-length wildtype (WT) PGT121 and VRC01 (which bind to V3 and CD4 binding sites within gp120 whereas 10E8 binds to the MPER region of gp41) were used as controls alongside the 10E8 constructs. WT full-length 10E8 IgG was biotinylated and employed to compare against the constructs. Ab49 scFv was also included as a control for the 10E8-Ab49 tandem scFv. Constructs were compared to each other in molar amounts rather than weights due to the differing size between both the constructs and the control Abs.
As controls, we found WT full length 10E8 bound primarily only to the WIV (). The other control WT full length bnAbs PGT121 and VRC01 bound to 4/7 (C.1086, CN54, B.6240 and JRFL) and 5/7 (C.1086, CN54, B.6240, AD8 and JRFL) of the gp140 proteins tested respectively (, ) and to the WIV. There was poor binding across all control and Ab constructs for the gp140 proteins from HIV strains UG21 and BR29 ().
Figure 6. Binding of constructs and control full-length antibodies to a range of HIV gp140 antigens and whole inactivated virus. ELISAs were used to screen constructs against the gp140 portion of viral envelope and whole AT-2 inactivated virus (WIV). The relative ODs for ½ log dilutions of the constructs and antibodies are given against gp140 reagents obtained from the NIH, barring the AD8 gp140 and whole inactivated virus (). Constructs were compared to each other in molar amounts rather than weights due to the differing size between both the constructs and the control Abs. (A) Shows PGT121, (B) VRC01, (C) 10E8, (D) Ab49 scFv control, (E) 10E8 scFv, (F) 10E8-A12 tandem scFv, (G) 10E8-10E8 diabody, (H) 10E8-Ab49 tandem scFv. Statistical analyses are presented in the text of the results section.
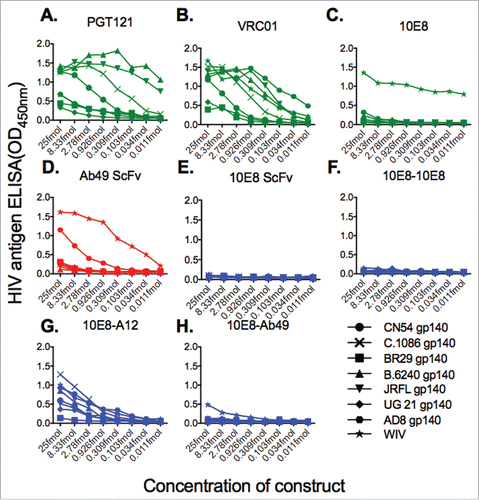
Scaffolded 10E8 scFv did not bind to any of the 7 gp140s nor to WIV (, p < 0.0001 compared to 10E8 wild type for WIV binding). The 10E8-10E8 monospecific diabody also did not bind to any of the 7 gp140s or WIV (, p < 0.0001 compared to 10E8 wild type for WIV binding), suggesting that the potential improved binding of 2 arms of 10E8 CDR did not resolve the substantial issues of the loss of binding in the scaffolded 10E8 scFv. The 10e8 scFv and scFv diabody constructs were tested at multiple different concentrations up to 3 times for each of the gp140 Env antigen with a consistent lack of ELISA binding as observed in .
The 10E8-Ab49 bispecific did not bind to any of the 7 gp140 protein strains tested. Although the 10E8-Ab49 bispecific had weak binding to the whole inactivated HIV (), this binding was significantly less than 10E8 wild type (p < 0.0001). The control Ab49 scFv alone had weak binding to the AD8 gp140 protein (as well as WIV), suggesting that the 10E8-Ab49 bispecific construct may have disrupted both the normal binding of 10E8 as well as binding of the Ab49scFv. Comparing the binding curves of Ab49 and 10E8-Ab49 (, ) shows that the tandem scFv had much less binding than the Ab49 scFv alone (p <0.0001). However, it was notable that Ab49 bound strongly to the WIV, and that both bispecific tandem scFv also showed moderate binding.
The 10E8-A12 bispecific did have significantly higher binding to all 7 of the gp140 antigens tested as well as the WIV compared to wild type 10E8 scFv (, all p <0.01). Since the scaffolded 10E8 scFv and 10E8-Ab49 bispecific had minimal binding to the 7 gp140s, this suggests that the A12 component of the bacteria-expressed bispecific retained its ability to bind gp140 in the plate-bound ELISA conformation.
Thus all constructs containing the scaffolded 10E8 scFv lost binding to multiple HIV-1 Env strains. The two binding arms of the diabody and tandem scFv did not appear to compensate for this loss of binding, though the data shows there was some retention of A12 binding, and to a lesser degree, Ab49 binding.
Neutralization of HIV pseudoviruses by 10E8 constructs
The ELISA results suggested that 10E8 binding to gp140 Env proteins may be impeded by the plate-bound confirmation of the antigens themselves. To further evaluate the loss of binding of the 10E8 constructs and determine whether 10E8's ability to inhibit virus entry was also abrogated, neutralization assays were performed. The 4 constructs (scaffolded 10E8 scFv, 10E8-10E8 diabody, and the tandem scFvs10E8-A12 and 10E8-Ab49) were tested against a range of tier 1 and 2 HIV-1 strains, along with WT 10E8 acting as a positive control. Negative controls for the neutralization assay included both a mouse leukaemia virus, which should not be neutralized and the Ab49 scFv construct, which retained ELISA binding capacity but does not contain a 10E8 scFv element. Neutralization was measured as the inhibitor concentration at which the luminescence reporter of HIV entry was reduced by 50% (IC50) compared to no Ab. WT 10E8 reached IC50 for 9 tested viruses. Most viruses were neutralized at concentrations <1.1 µg/ml of WT 10E8, except for the tier 2 clade C SHIV 1157ipd3N4, which required 8.77µg/ml to reach IC50 (). In contrast all 10E8 scFv constructs were poor neutralizers. All constructs achieved IC50 below 2.1µg/ml for the tier 1A MN.3 strain of pseudovirus. In some cases this represented a mild drop from an IC50 0.41 to 0.56 for the 10E8-A12 tandem scFv compared to WT, however it represented a 5 times reduction in neutralization for the 10E8-Ab49 tandem scFv. All constructs were also incapable of neutralizing 5 of the 9 strains and the successful neutralizations for the remaining 3 pseudoviruses had IC50 above 19µg/ml (), indicating 20–200 times loss of neutralization efficacy compared to WT 10E8, depending on the virus strain. The neutralization data indicate that all constructs suffered a loss of function on any virus that was more difficult to neutralize than tier 1A.
Discussion
The long-term goal is to produce a range of fully humanized, soluble bnAb-based constructs in the cytoplasm of E. coli for their cost-effective use to treat and prevent HIV. Although this was not achieved herein, we made some progress along this pathway. Two bnAb CDRs were transplanted onto a previously described scaffoldCitation26 to produce 10E8 and PGT121-like scFvs. One of the two bNAbs scFvs studied, soluble 10E8 expressed to high levels in bacteria and three additional 10E8-based constructs (10E8-10E8 diabody, and the tandem scFvs10E8-A12 and 10E8-Ab49) were also constructed and expressed. High yields of 0.2-1mg of these constructs were readily purified and tested for gp140-binding and neutralization ability. Although the results of the binding and neutralization studies were negative, the work provided a first step demonstrating that the production of bnAb-like constructs in bacteria cytoplasm was possible.
Unfortunately, the constructs suffered a loss of function, such that the two bispecific tandem scFvs, 10E8-A12 and 10E8-Ab49, were only capable of binding to whole inactivated virus in ELISAs (). Even these bispecifics bound less well than the full-length WT 10E8. The Ab49 scFv alone was found to bind to whole MN HIV-1Citation41 substantially better than the 10E8-Ab49 tandem scFv, suggesting that combining the two different scFv reduced function rather than enhancing it. Some neutralization against a tier 1A MN.3 virus was detected for all the constructs, however there was greater than 20–200 times activity loss for all constructs against higher tier virus strains (). This indicates a near-complete loss of neutralization activity that appears to abrogate 10E8 breadth against all but the lowest tier viruses.
Interestingly, both WT 10E8 and the constructs could not bind well to gp140 antigens in ELISAs. The epitope that 10E8 targets is the MPER region of envelope and it is possible that the plate-bound conformation of gp140 in ELISAs somehow sterically hinders or obscures this crucial conserved epitope. The data support this in that whole virus inactivated by AT-2, a non-detergent reagent that does not disrupt envelope conformation, was required to show good WT 10E8 and construct binding in ELISAs. This suggests that future screens of any MPER-binding constructs may need to employ assays that do not require a plate-bound conformation of their envelope antigens.
While 10E8 has one of the widest breadths of all known bnAbsCitation29, PGT121 is less broad and is most notable for its remarkable potency.Citation42 Thus if scaffolded PGT121 scFv could be produced in this cytoplasmic system, even some loss of potency might still make it economically feasible to up-scale. To improve the solubility of the scaffolded PGT121 scFv, the vector expression system could be transformed into bacteria strains with the reductase path deleted. This deletion decreases the oxidation threshold of these E. coli, potentially making their cytoplasm more suited to the folding of soluble protein199, 224.
Although the reduced neutralization displayed by the bacteria-produced scFv constructs showed they would not make useful therapeutic tools at present, there are steps that could be taken to improve their efficacy. Anti-HIV bnAbs tend to include substantial levels of hypermutation compared to other Abs.Citation43 However the constructs were produced with less hypermutation to ensure that solubility would be retained (). For the 10E8 construct, sequence identity was around 85%. For PGT121, the heavy chain had only 88.5% similarity and 68.7% amino acid identity. Despite evidence that many hypermutations can be successfully reverted in bnAbsCitation39,Citation44, the scFv constructs lost neutralization activity. A careful screen may potentially increase efficacy without compromising solubility. Furthermore, the initial study describing the ReD techniques demonstrated that the IGHV3-23 and IGLV3-1 scFv fusion readily tolerated diversification of the CDR H3.Citation26 Thus ReD could be used to screen a library of 10E8 or PGT121 scaffolded scFvs. ReD uses the same bacterial platform for this type of screening and for expression, meaning the technologies are extremely compatible. Another way of improving the mono- and bispecific constructs would be by optimising the length of the linker between the scFvs. This has been show to be an important consideration in tandem scFvs production, exemplified in a study by Galimidi et al., showing dsDNA could be used as a “molecular ruler” to achieve optimized linker length.Citation45 Finally, the SDS-PAGE gels showed some impurities in the protein preparations. In future studies of more promising scFv candidates, further purification steps will be useful to improve neutralization function.
In conclusion, it proved difficult to express functional bNab scFv in bacteria by transplanting their CDR3 region onto a scaffold, lessons that should prove salutory to the field. Despite the functional setbacks encountered, we found that bispecific 10e8 scFv diabodies could be produced that retained some Env binding. Several approaches are now suggested to build upon this work. Our work represents the first step towards the potential for rapid production of bnAb-like scFv constructs in an inexpensive and high-yield bacterial expression system. As the tools for cost-effectively and reliably producing Ab constructs improves, so too will the potential for mass-distribution of these important therapeutic and preventative tools.
Methods
Transplantation of 10E8 and PGT121 CDRs into IGHV3-23 and IGLV3-1 scaffold
The CDRs of the anti-HIV bnAbs 10E8 and PGT121 were transplanted onto a scFv scaffold composed of a fusion of the germline human heavy chain IGHV3-23 and light chain IGLV3-1 that was previously found to be soluble in E. coli cytoplasm.Citation26 To improve the likelihood of a soluble scFv product retaining anti-HIV binding, changes were made to the sequence of both the IGHV3-23 and the IGLV3-1 to make them more in line with the bnAb sequence, including complete identity with the CDR ().
The new CDR-transplanted scaffold sequences and spacer domains were ordered as gene blocks (Integrated DNA Technologies, IDT). Domains for increased stability, purification and in vivo half-life were also incorporated into the constructs and the entire gene blocks were cloned into a rhamnose-inducible plasmid vector (pRhn from DNA2.0). The cloning process was a standard ligation where the pRhn vector was digested with XhoI/Xbal (New England Biolabs, NEB) double digest according to manufactures instructions. Briefly, XhoI/Xbal were combined with cutsmart buffer (NEB) and the plasmid vector and heated for 37°C for 1 hr. The enzymes were denatured at 80°C for 20 min after digest. The gene block insert was ligated into the vector using T4 ligase (NEB) according the manufacture's instructions. Briefly, T4 ligase was combined with ligation buffer (NEB) and a 3:1 ration of insert to vector. Vector+geneblock plasmids were transformed into a K12-derived Argentum strain of E. coli (Alchemy Biosciences) using electroporation. Positive colonies were selected on 30 µg/mL kanamycin agar and tested for correct gene block insert size by restriction digest screening.
Further 10E8 constructs (10E8-10E8 diabody, tandem scFvs 10E8-A12 and 10E8-Ab49) were produced as outlined above, using different gene blocks. The Ab49 arm is a non-neutralizing scFv discovered as part of an extensive in vitro ReD screen against a HIV-1 gp140 trimer. This screen produced many novel, soluble scFvs against HIV and the best binders were determined through ELISAs and affinity screens. Ab49 had consistently good binding against five different gp140 antigens and gp41.
Scaffolds containing the PGT121 CDR were first prepared as above, however PGT121 geneblocks were later cloned into an arabinose-inducible vector pAra5 similarly as described above, with minor changes. Ligations were performed by an XbaI/HindIII (NEB) double digest according to manufacturer's instructions.
Purification of antibody fragments from E. coli
Cultures were grown at 37°C and protein expression was induced by 0.2% arabinose once OD600 had been reached. Induced culture was shaken for a further two hours at 25°C before permeablization as previously describedCitation26 with 0.5% n-octyl-β-D-thioglucopyranoside in Luria broth for 10 min at 25°C. Permeabilized cells were pelleted and the antibody-containing supernatant collected. For all products used in ELISAs and neutralization assays, a simple column purification using HisTrap™ HP columns (VWR) was performed according the manufacturer's instructions. The yields of the protein preparations that were used in the neutralization and binding assays were measured by nanodrop, with the concentrations confirmed using a BSA standard. The yields were as followed: 10E8 scFv 0.5mg, 10E8-A12 0.43mg, 10E8-10E8 0.18mg, 10E8-Ab49 0.22 mg and 0.2mg of 10E8-Ab49.
Visualization of purified antibody protein
Ab construct proteins were visualized by protein and Western blot gels. Samples were mixed with a 3:1 ratio of Laemmli loading buffer and loaded onto pre-cast polyacrylamide gel (BioRad Laboratories) according to manufacturer's instructions. Gels were run at 100V for approximately 30 min in a BioRad Mini-PROTEAN® cell II. Gels were removed from their casing and fixed in methanol and acetic acid for 1 hr in the dark, with the first 30 min rolling on a vial roller. Where transfer was necessary for Western blot, proteins were transferred onto nitrocellulose in 3 Whatman buffer at 100V for 1 hr. Transfers were then blocked in 5% milk TBS-T, with tween at 0.5%, overnight. Detection was done with the primary Ab rat-anti-Biotin used at 1/1000 with anti-rat AF488 as the secondary Ab. Abs were washed off at each step 4 times for 10 min in the dark. Gels were visualized using a BioRad Pharos molecular imager.
ELISA-based analyses for binding of constructs to HIV Env proteins
ELISA plates were coated with a range of gp140, gp120 and gp41 HIV Env proteins (NIH AIDS reagent program, see for catalogue numbers) at 100 ng/well overnight at 4°C. Plates were blocked with PBS+0.1%Tween 20+5%BSA for 1 hr. After washing, dilutions of Ab constructs or WT 10E8 were added and incubated for 4 hr at 37°C. After washing away Abs, streptavidin (BD Biosciences) was incubated for 1 hr at 37°C. ELISAs were visualized using TMB (Thermo Fischer Scientific). The TMB reactions were stopped with the addition of 50 µl of 1M sulphuric acid and quantified on a CLARIOstar plate reader (BMG Labtech). No antigen negative controls were used to determine background binding.
Table 1. Further description of antigens used in the ELISAs.
Table 2. Neutralization of pseudoviruses by 10E8 constructs compared to WT 10E8.
Statistical analyses of ELISA binding data
To compare the kinetics of OD values between different NAbs, we used a standard dose-response sigmoidal curve as below:in which:
Min | = | = minimum background value |
Max | = | = maximum OD value |
X | = | = log of concentration of test antibody |
IC50 | = | = concentration that will give half of the maximum OD value |
We found no difference in the Min value between different Nab constructs without antigen (in negative control sample, p = 0.18, Kruskal-Wallis test). The Min value was then fixed based on the value of the no antigen (negative) control. We then tested if IC50 and Max can be shared between different NAb types. This was done using an F-test for testing a nested model (GraphPad Prism version 7).
Analyses of antibody construct neutralization of HIV pseudoviruses
Neutralization assays were performed as previously described.Citation46 Briefly, pseudotyped viruses were incubated for 1h r at 37°C with various dilutions of antibody constructs and WT 10E8 as a control and then used to infect TZM-bl cells pre-treated with DEAE-dextran (10 µg/ml). Cells were incubated at 37°C for 48hrs and then lysed with the Bright-Glo luciferase assay system (Promega). A long terminal repeat (LTR) driven luciferase reporter gene in TZM-bl cells generated the luciferase signal. Infection was quantified by measuring luciferase activity with a Multiscan MCC/340 luminometer, Model 347 (MTX Lab Systems). Neutralization was measured as the reduction in luciferase activity compared with that of untreated controls.
Disclosure of potential conflicts of interest
Dr. Ben Kiefel and Dr. Keith Niven are employees of the company Affinity BIO and Dr Wendy Winnall holds stock in Affinity BIO. Affinity BIO holds patents in the field of antibody engineering.
References
- Mascola JR, Stiegler G, VanCott TC, Katinger H, Carpenter CB, Hanson CE, Beary H, Hayes D, Frankel SS, Birx DL, et al. Protection of macaques against vaginal transmission of a pathogenic HIV-1/SIV chimeric virus by passive infusion of neutralizing antibodies. Nat Med. 2000;6(2):207-10. https://doi.org/10.1038/72318. PMID:10655111
- Veazey RS, Shattock RJ, Pope M, Kirijan JC, Jones J, Hu Q, Ketas T, Marx PA, Klasse PJ, Burton DR, et al. Prevention of virus transmission to macaque monkeys by a vaginally applied monoclonal antibody to HIV-1 gp120. Nat Med. 2003;9(3):343-6. https://doi.org/10.1038/nm833. PMID:12579198
- Hessell AJ, Rakasz EG, Poignard P, Hangartner L, Landucci G, Forthal DN, Koff WC, Watkins DI, Burton DR. Broadly Neutralizing Human Anti-HIV Antibody 2G12 Is Effective in Protection against Mucosal SHIV Challenge Even at Low Serum Neutralizing Titers. Plos Pathog. 2009;5(5):e1000433. https://doi.org/10.1371/journal.ppat.1000433. PMID:19436712
- Hessell AJ, Jaworski JP, Epson E, Matsuda K, Pandey S, Kahl C, Reed J, Sutton WF, Hammond KB, Cheever TA, et al. Early short-term treatment with neutralizing human monoclonal antibodies halts SHIV infection in infant macaques. Nat Med. 2016;22(4):362-8. https://doi.org/10.1038/nm.4063. PMID:26998834
- Barouch DH, Whitney JB, Moldt B, Klein F, Oliveira TY, Liu J, Stephenson KE, Chang HW, Shekhar K, Gupta S, et al. Therapeutic efficacy of potent neutralizing HIV-1-specific monoclonal antibodies in SHIV-infected rhesus monkeys. Nature. 2013;503(7475):224-8. PMID:24172905
- Moldt B, Rakasz EG, Schultz N, Chan-Hui PY, Swiderek K, Weisgrau KL, Piaskowski SM, Bergman Z, Watkins DI, Poignard P, et al. Highly potent HIV-specific antibody neutralization in vitro translates into effective protection against mucosal SHIV challenge in vivo. Proc Natl Acad Sci USA. 2012;109(46):18921-5. https://doi.org/10.1073/pnas.1214785109. PMID:23100539
- Shingai M, Nishimura Y, Klein F, Mouquet H, Donau OK, Plishka R, Buckler-White A, Seaman M, Piatak M Jr, Lifson JD, et al. Antibody-mediated immunotherapy of macaques chronically infected with SHIV suppresses viraemia. Nature. 2013;503(7475):277-80. PMID:24172896
- Caskey M, Klein F, Lorenzi JC, Seaman MS, West AP, Buckley N, Kremer G, Nogueira L, Braunschweig M, Scheid JF, et al. Viraemia suppressed in HIV-1-infected humans by broadly neutralizing antibody 3BNC117. Nature. 2015;522(7557):487-91. https://doi.org/10.1038/nature14411. PMID:25855300
- Caskey M, Klein F, Nussenzweig MC: Broadly Neutralizing Antibodies for HIV-1 Prevention or Immunotherapy. N Engl J Med. 2016;375(21):2019-21. https://doi.org/10.1056/NEJMp1613362. PMID:27959740
- Lynch RM, Boritz E, Coates EE, DeZure A, Madden P, Costner P, Enama ME, Plummer S, Holman L, Hendel CS, et al. Virologic effects of broadly neutralizing antibody VRC01 administration during chronic HIV-1 infection. Sci Translat Med. 2015;7(319):319ra206. https://doi.org/10.1126/scitranslmed.aad5752
- Ledgerwood JE, Coates EE, Yamshchikov G, Saunders JG, Holman L, Enama ME, DeZure A, Lynch RM, Gordon I, Plummer S, et al. Safety, Pharmacokinetics, and Neutralization of the Broadly Neutralizing HIV-1 Human Monoclonal Antibody VRC01 in Healthy Adults. Clin Exp Immunol. 2015;182(3):289-301. https://doi.org/10.1111/cei.12692. PMID:26332605
- Kelley B: Industrialization of mAb production technology The bioprocessing industry at a crossroads. Mabs. 2009;1(5):443-52. https://doi.org/10.4161/mabs.1.5.9448. PMID:20065641
- Felo M, Christensen B, Higgins J: Process cost and facility considerations in the selection of primary cell culture clarification technology. Biotechnol Prog. 2013;29(5):1239-45. https://doi.org/10.1002/btpr.1776. PMID:23847160
- Frenzel A, Hust M, Schirrmann T. Expression of recombinant antibodies. Front Immunol. 2013;4:217. https://doi.org/10.3389/fimmu.2013.00217. PMID:23908655
- Schirrmann T, Al-Halabi L, Dubel S, Hust M: Production systems for recombinant antibodies. Front Biosci. 2008;13:4576-94. https://doi.org/10.2741/3024. PMID:18508530
- Virdi V, Depicker A. Role of plant expression systems in antibody production for passive immunization. Int J Dev Biol. 2013;57(6-8):587-93. https://doi.org/10.1387/ijdb.130266ad. PMID:24166441
- Buckholz RG, Gleeson MA: Yeast systems for the commercial production of heterologous proteins. Biotechnology. 1991;9(11):1067-72. https://doi.org/10.1038/nbt1191-1067. PMID:1367623
- Seo MJ, Jeong KJ, Leysath CE, Ellington AD, Iverson BL, Georgiou G: Engineering antibody fragments to fold in the absence of disulfide bonds. Prot Sci 2009;18(2):259-267. https://doi.org/10.1002/pro.31
- Huston JS, Levinson D, Mudgett-Hunter M, Tai MS, Novotný J, Margolies MN, Ridge RJ, Bruccoleri RE, Haber E, Crea R. Protein engineering of antibody binding sites: Recovery of specific activity in an anti-digoxin single-chain Fv analogue produced in Escherichia coli. Proc Natl Acad Sci USA . 1988;85(16):5879-83. https://doi.org/10.1073/pnas.85.16.5879. PMID:3045807
- de Marco A. Strategies for successful recombinant expression of disulfide bond-dependent proteins in Escherichia coli. Microb Cell Fact 2009;8:26. https://doi.org/10.1186/1475-2859-8-26. PMID:19442264
- Lee YJ, Jeong KJ. Challenges to production of antibodies in bacteria and yeast. J Biosci Bioeng. 2015;120(5):483-90. https://doi.org/10.1016/j.jbiosc.2015.03.009. PMID:25912450
- Martineau P, Jones P, Winter G: Expression of an antibody fragment at high levels in the bacterial cytoplasm. J Mol Biol. 1998;280(1):117-27. https://doi.org/10.1006/jmbi.1998.1840. PMID:9653035
- Proba K, Worn A, Honegger A, Pluckthun A: Antibody scFv fragments without disulfide bonds made by molecular evolution. J Mol Biol. 1998;275(2):245-53. https://doi.org/10.1006/jmbi.1997.1457. PMID:9466907
- Wang LX, Mellon M, Bowder D, Quinn M, Shea D, Wood C, Xiang SH. Escherichia coli surface display of single-chain antibody VRC01 against HIV-1 infection. Virology. 2015;475:179-86. https://doi.org/10.1016/j.virol.2014.11.018. PMID:25482819
- Maruta Y, Kuwata T, Tanaka K, Alam M, Valdez KP, Egami Y, Suwa Y, Morioka H, Matsushita S. Cross-neutralization activity of single-chain variable fragment (scFv) derived from anti-V3 monoclonal antibodies mediated by post-attachment binding. Jpn J Infect Dis. 2016;69(5):395-404. https://doi.org/10.7883/yoken.JJID.2015.667. PMID:26902223
- Beasley MD, Niven KP, Winnall WR, Kiefel BR: Bacterial cytoplasmic display platform Retained Display (ReD) identifies stable human germline antibody frameworks. Biotechnol J. 2015;10(5):783-9. https://doi.org/10.1002/biot.201400560. PMID:25712138
- Visintin M, Settanni G, Maritan A, Graziosi S, Marks JD, Cattaneo A: The intracellular antibody capture technology (IACT): Towards a consensus sequence for intracellular antibodies. J Mol Biol. 2002;317(1):73-83. https://doi.org/10.1006/jmbi.2002.5392. PMID:11916379
- Tse E, Lobato MN, Forster A, Tanaka T, Chung GT, Rabbitts TH: Intracellular antibody capture technology: application to selection of intracellular antibodies recognising the BCR-ABL oncogenic protein. J Mol Biol. 2002;317(1):85-94. https://doi.org/10.1006/jmbi.2002.5403. PMID:11916380
- Huang J, Ofek G, Laub L, Louder MK, Doria-Rose NA, Longo NS, Imamichi H, Bailer RT, Chakrabarti B, Sharma SK, et al. Broad and potent neutralization of HIV-1 by a gp41-specific human antibody. Nature. 2012;491(7424):406-12. https://doi.org/10.1038/nature11544. PMID:23151583
- Mouquet H, Scharf L, Euler Z, Liu Y, Eden C, Scheid JF, Halper-Stromberg A, Gnanapragasam PN, Spencer DI, Seaman MS, et al. Complex-type N-glycan recognition by potent broadly neutralizing HIV antibodies. Proc Natl Acad Sci USA. 2012;109(47):E3268-77. https://doi.org/10.1073/pnas.1217207109. PMID:23115339
- Kufer P, Lutterbuse R, Baeuerle PA: A revival of bispecific antibodies. Trends Biotechnol. 2004;22(5):238-44. https://doi.org/10.1016/j.tibtech.2004.03.006. PMID:15109810
- Chen M, Wen K, Tao XQ, Xie J, Wang L, Li Y, Ding S, Jiang H. Cloning, expression, purification and characterization of a bispecific single-chain diabody against fluoroquinolones and sulfonamides in Escherichia coli. Protein Expr Purif. 2014;100:19-25. https://doi.org/10.1016/j.pep.2014.04.015. PMID:24816423
- Huang BC, Davern S, Kennel SJ: Mono and bivalent binding of a scFv and covalent diabody to murine laminin-1 using radioiodinated proteins and SPR measurements: Effects on tissue retention in vivo. J Immunol Meth. 2006;313(1-2):149-60. https://doi.org/10.1016/j.jim.2006.04.006
- Winnall WR, Beasley MD, Center RJ, Parsons MS, Kiefel BR, Kent SJ: The maturation of antibody technology for the HIV epidemic. Immunol Cell Biol. 2014;92(7):570-7. https://doi.org/10.1038/icb.2014.35. PMID:24797582
- Muyldermans S. Nanobodies: Natural single-domain antibodies. Annu Rev Biochem 2013;82:775-97. https://doi.org/10.1146/annurev-biochem-063011-092449. PMID:23495938
- Forsman A, Beirnaert E, Aasa-Chapman MM, et al.: Llama antibody fragments with cross-subtype human immunodeficiency virus type 1 (HIV-1)-neutralizing properties and high affinity for HIV-1 gp120. J Virology. 2008;82(24):12069-81. https://doi.org/10.1128/JVI.01379-08. PMID:18842738
- Zhou T, Georgiev I, Wu X, Yang ZY, Dai K, Finzi A, Kwon YD, Scheid JF, Shi W, Xu L, et al. Structural basis for broad and potent neutralization of HIV-1 by antibody VRC01. Science. 2010;329(5993):811-7. https://doi.org/10.1126/science.1192819. PMID:20616231
- Rozak DA, Orban J, Bryan PN. G148-GA3: A streptococcal virulence module with atypical thermodynamics of folding optimally binds human serum albumin at physiological temperatures. Biochim Biophys Acta. 2005;1753(2):226-33. https://doi.org/10.1016/j.bbapap.2005.10.005. PMID:16290081
- Sok D, Laserson U, Laserson J, Liu Y, Vigneault F, Julien JP, Briney B, Ramos A, Saye KF, Le K, et al. The effects of somatic hypermutation on neutralization and binding in the PGT121 family of broadly neutralizing HIV antibodies. PLoS Pathog. 2013;9(11):e1003754. https://doi.org/10.1371/journal.ppat.1003754. PMID:24278016
- Zhu Z, Zapata G, Shalaby R, Snedecor B, Chen H, Carter P: High level secretion of a humanized bispecific diabody from Escherichia coli. Biotechnology. 1996;14(2):192-6. PMID:9636323
- Rutebemberwa A, Bess JW, Brown B, Arroyo M, Eller M, Slike B, Polonis V, McCutchan F, Currier JR, Birx D, et al. Evaluation of aldrithiol-2-inactivated preparations of HIV type 1 subtypes A, B, and D as reagents to monitor T cell responses. AIDS Res Hum Retroviruses. 2007;23(4):532-42. https://doi.org/10.1089/aid.2006.0136. PMID:17506610
- Walker LM, Huber M, Doores KJ, Falkowska E, Pejchal R, Julien JP, Wang SK, Ramos A, Chan-Hui PY, Moyle M, et al. Broad neutralization coverage of HIV by multiple highly potent antibodies. Nature. 2011;477(7365):466-70. https://doi.org/10.1038/nature10373. PMID:21849977
- Derdeyn CA, Moore PL, Morris L. Development of broadly neutralizing antibodies from autologous neutralizing antibody responses in HIV infection. Curr Opin HIV AIDS. 2014;9(3):210-16. https://doi.org/10.1097/COH.0000000000000057. PMID:24662931
- Georgiev IS, Rudicell RS, Saunders KO, Shi W, Kirys T, McKee K, O'Dell S, Chuang GY, Yang ZY, Ofek G, et al. Antibodies VRC01 and 10E8 neutralize HIV-1 with high breadth and potency even with Ig-framework regions substantially reverted to germline. J Immunol. 2014;192(3):1100-06. https://doi.org/10.4049/jimmunol.1302515. PMID:24391217
- Galimidi RP, Klein JS, Politzer MS, Bai S, Seaman MS, Nussenzweig MC, West AP Jr, Bjorkman PJ, et al. Intra-spike crosslinking overcomes antibody evasion by HIV-1. Cell. 2015;160(3):433-46. https://doi.org/10.1016/j.cell.2015.01.016. PMID:25635457
- Montefiori DC. Evaluating neutralizing antibodies against HIV, SIV, and SHIV in luciferase reporter gene assays. Curr Prot Immunol. 2005; Chapter 12:Unit 12 11.