ABSTRACT
Influenza A/H3N2 viruses are the most common and virulent subtypes for humans. Antigenic drift, changes in antigenicity through the accumulation of mutations in the hemagglutinin (HA) gene is chiefly responsible for the continuing circulation of A/H3N2 viruses, resulting in frequent updates of vaccine strains based on new variant analyses. In humans, these drift-related mutations are considered to be primarily caused by the immune pressure elicited by natural infection. Whether or not the immune pressure elicited by vaccination (vaccine pressure) can have a certain effect on drift-related mutations is unclear. Recently, our findings suggested the possible effect of vaccine pressure on HA mutations by directly comparing amino acid differences from the corresponding vaccine strains between isolates from vaccinated and unvaccinated patients. It is possible that influenza vaccine pressure selects variants genetically distant from the vaccine strains. Considering the effect of vaccine pressure on HA mutations would contribute to further understanding the mechanism of antigenic drift, which would be helpful for predicting future epidemic viruses.
Introduction
Epidemics caused by influenza viruses continue to have a major impact worldwide. This family of viruses is responsible for high rates of morbidity and mortality in humans and the socioeconomic burden is, therefore, significant. The two influenza viruses that cause significant infections in humans are A and B. Of these, the influenza A/H3N2 virus has remained one of the most significant causes of infection in humans since the 1968 Flu Pandemic. The higher rate of mutation and the global circulation pattern of A/H3N2 viruses, when compared to the A/H1N1 and B types, allow for the annual spread of new variants.Citation1,2 Influenza vaccines are important for the prevention of infection, and have been modified over several decades.Citation3 Currently, influenza vaccine strains have been updated based on recommendations by the World Health Organization (WHO).Citation4 However, because new H3N2 epidemic variants of next season are more difficult to predict, as compared with H1N1 and B, the protection offered by H3N2 vaccines has been suboptimal, particularly in recent years.Citation5-7 This difficulty in prediction is due to the fact that the mechanisms underlying the emergence of new variants, accompanied by many potential contributing factors, are still under question. Recently, we have obtained a finding suggesting that the immune pressure elicited by seasonal vaccination may affect the formation of H3N2 variants.Citation8 This review highlights the significance of our findings, along with the introduction of studies done by other researchers.
HA antigenic drift
Influenza viruses are highly mutable due to the lack of RNA proofreading, resulting in the natural selection of viruses with a survival advantage. The escape mechanisms of these genetic variants, based on antigenic changes, have been discussed over a prolonged period. The antigenic change of influenza viruses was initially observed several decades ago using anti-serum or anti-hemagglutinin (HA) antibody.Citation9,10 Moreover, it is now evident that HA mutations, which are favorable for survival, can be easily introduced through exposure to anti-HA monoclonal antibodies.Citation11 Thus, the high antigenicity of HA, a major surface protein, produces a strong immune pressure on the host, which allows influenza viruses to escape host immunity by changes in antigenicity, based on HA mutations.Citation12,13 This process is called antigenic drift. In H3N2 viruses, the globular head of the HA1 subunit contains five independent antigenic epitope regions (A to E),Citation14,15 and the HA mutations leading to antigenic drift are concentrated at these regions.Citation16 These drift-related mutations have been considered to be primarily caused by the immune pressure elicited by natural infection, but this leaves the question as to whether the immune pressure elicited by vaccination (vaccine pressure) has an effect on drift-related mutations.
The effect of vaccination on HA mutation in animals
There are several data regarding the effect of vaccine pressure on HA mutations in animals infected with influenza viruses. In dogs and horses, conflicting data on the differences in HA mutations between vaccinated and unvaccinated animals were obtained,Citation17,18 but the low sample numbers limit further evaluation of the issue. In contrast, in the field of avian influenza, nationwide vaccine programs for poultry were conducted in several countries due to the emergence of H5N1 highly pathogenic avian influenza (HPAI), which had a considerable economic impact. After the first report of HPAI in Indonesia in 2003, vaccine programs for poultry were performed in Indonesia and Hong Kong.Citation19,20 Subsequently, H5N1 viruses emerged that escaped vaccine pressure by the original vaccine strains, resulting in eventual vaccine failure. These viruses contained HA mutations that caused significant antigenic changes. Thus, the vaccination program for poultry induced the appearance of antigenic drift-related variants. Higher mutation rates of HA genes have been reported in countries applying H5 vaccination, when compared to countries not applying vaccination.Citation21
The effect of vaccination on HA mutation in humans
Data on the effect of vaccine pressure on HA mutations in humans have recently been reported.Citation22,23 The results of these studies are summarized as follows: (1) a phylogenetic method did not segregate H3N2 viruses isolated from vaccinated and unvaccinated patients, and (2) a deep sequencing method showed that within-host HA genetic diversity, based on single nucleotide polymorphism (SNP) analysis, was not significantly different between vaccinated and unvaccinated patients. A recent article reported by Debbink et al. suggested no relationship of SNP frequencies with hemagglutination inhibition (HI) titers after vaccination.Citation23 At the beginning, the researchers of the abovementioned studies had presumed that within vaccinated patients, after vaccination, the diverse genetic variants that are present within the host would narrow the population down, due to the selection of antigenic drift-related variants by vaccine pressure. In addition, they presumed that if the drift-related HA mutations were significantly different from those of unvaccinated patients, the clades would be segregated between isolates from vaccinated and unvaccinated patients.
We have developed a network of physicians across Japan, who routinely collect influenza virus samples, along with patient information including vaccination history. We determined a total of 181 full-length sequences of HA genes of H3N2 viruses isolated from 82 vaccinated and 99 unvaccinated patients (2011–15, four Japanese influenza seasons) using a next-generation sequencer.Citation8 At the beginning, a phylogenetic analysis did not segregate the isolates from vaccinated and unvaccinated patients, as indicated in the aforementioned reports. We next tried to examine HA amino acid (AA) differences from the corresponding seasonal vaccine strains. As a result, deviation to a greater number of AA differences within HA1 antigenic epitope sites was found more significantly in the isolates from vaccinated than from unvaccinated patients, irrespective of seasons ( and ). A maximum of four AA differences of each isolate from the corresponding vaccine strain was observed with the epitope sites in the 2011–12, 2012–13, and 2013–14 seasons. Bias for the greater AA differences detected in vaccinated patients was confirmed in a total of the three seasons (P = 0.0005) (). In contrast to the 2011–14 seasons, a remarkable increase (six to fifteen) in the number of sites with an AA difference was found in the 2014–15 season. Similar to the previous seasons, there was a significant deviation to a greater number of AA differences in the isolates from vaccinated patients than from unvaccinated patients (P = 0.0096) (). In addition, the AA difference rates at epitope sites within HA1 sites were significantly higher in the isolates from vaccinated patients than from unvaccinated patients (P = 0.0332 for the 2011–14 seasons; P = 0.0575 for the 2014–15 season; P = 0.0316 for the 2011–15 seasons) (). In contrast, the AA difference rates at non-epitope sites were similar between the isolates from vaccinated and unvaccinated patients ().
Figure 1. Comparison of amino acid differences in HA1 epitope sites between influenza A/H3N2 isolates from vaccinated and unvaccinated patients in Japan. A maximum of four amino acid (AA) differences of each isolate from the corresponding vaccine strain was observed within the epitope sites in the 2011–12, 2012–13, and 2013–14 seasons. Six to fifteen in the number of sites with an AA difference was seen in the 2014–15 season. and show the results of the 2011–14 seasons (3 seasons in total) and 2014–15 season, respectively. This figure was created based on reference 8. AA, amino acid.
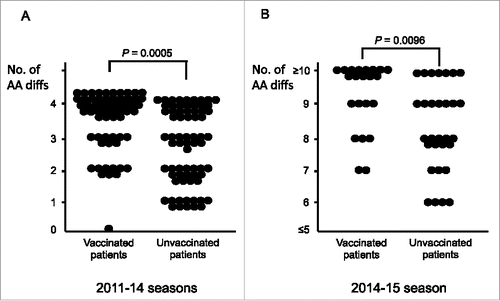
Figure 2. Comparison of amino acid difference rates in HA1 sites between influenza A/H3N2 isolates from vaccinated and unvaccinated patients in Japan. Based on the data provided in , amino acid (AA) difference rates at epitope sites (A) and non-epitope sites (B) were compared between the isolates from vaccinated and unvaccinated patients. This figure was created based on reference 8. Vac, vaccinated patients; Unvac, unvaccinated patients.
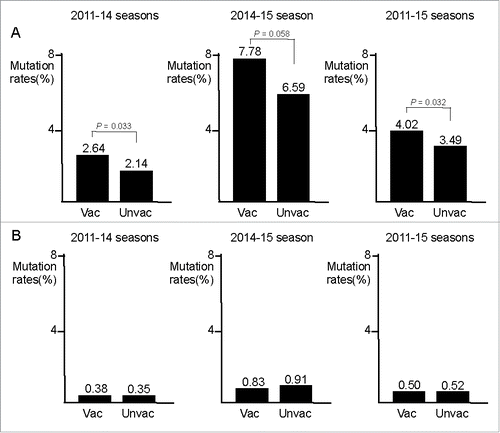
Detection ability of the effect of vaccination on HA mutation
The Dinis and Debbink research groups showed no difference in the SNP frequency of HA1 genes between isolates from vaccinated and unvaccinated patients.Citation22,23 In addition, these studies found that within-host HA genetic diversity was low (∼10%) and that even if SNPs were present, they were sparsely detected within HA1. Similarly, other studies also showed that HA genetic diversity after influenza infection was extremely low and sparse within the host.Citation24,25 An SNP analysis may not be appropriate for detecting the effect of vaccine pressure on HA mutation patterns.
As indicated by the two groups, we also showed that there was no phylogenetic segregation between isolates from vaccinated and unvaccinated patients. This result means that viruses that are able to infect vaccinated persons will cause epidemics, finally irrespective of vaccination status, although the development process is unclear about the intra-host and inter-host selection mechanisms. Therefore, viruses containing HA mutations that evade host immunity can be isolated from both vaccinated and unvaccinated patients, resulting in no segregation in the phylogenetic tree. We were first able to suggest the possible effect of vaccine pressure on HA mutations by directly comparing AA differences from the corresponding vaccine strains between isolates from vaccinated and unvaccinated patients.
The extent of AA differences was extremely different between the 2011–14 and 2014–15 seasons ( and ). AA mutation rates within the HA epitope sites dramatically increased in the 2014–15 season after a relatively moderate mutation rate during the 2011–14 seasons. Thus, our study included two heterogeneous influenza seasons in which there were considerable quantitative differences in HA mutations. Despite a substantial difference in the epidemic variants of these two periods, the AA difference pattern that was predominant for vaccinated patients was similar in both the periods. Our findings suggest that vaccine pressure works to select influenza variants genetically distant from vaccine strains (vaccine-related genetic drift), regardless of influenza seasons.
Is the effect of vaccination on HA mutation disturbed by human immune history?
Unlike poultry, humans are repetitively infected with different influenza viruses, and therefore, each person seems to have a different immune history to influenza infection, particularly to H3N2 infection due to its long-term circulation.Citation26 Even if humans are infected with the same epidemic viruses or receive the same seasonal vaccination, post-infection and post-vaccination immune responses, including the specificity and magnitude of antibodies elicited, are presumed to be different and complicated.Citation27,28 Our findings showed that the isolates from patients receiving vaccination with common vaccine strains exhibited the same pattern of genetic drift, as mentioned above. Finally, apart from the question of the different immune history of each person, our findings suggest that influenza vaccine pressure selects genetically distant variants from vaccine strains, because of which those variants can escape vaccine pressure.
An interesting hypothesis has been proposed regarding the relationship between population-wide immunity (herd immunity) and HA antigenic drift.Citation12 If vaccination coverage is higher than 80%, the influenza virus would not undergo additional HA mutations to escape vaccine pressure, resulting in the epidemic not spreading. On the other hand, HA antigenic drift is more likely to accelerate, as vaccination coverage increases within a coverage rate lower than 80%. This means that an intermediate vaccination coverage rate results in the highest magnitude of HA antigenic drift. The coverage rate of influenza vaccination in Japan is just the “intermediate”. Thus, the incomplete vaccination coverage in Japan is rather likely to accelerate HA mutations, through which the difference in HA mutations between isolates from vaccinated and unvaccinated patients might have been detected in our study. What, then, is the extent and pattern of HA mutations in countries in which influenza vaccination has never been conducted? This is a fascinating question.
Concluding remarks
Our study does not demonstrate whether the highly mutated variants detected in the vaccinated patients changed the antigenicity, causing antigenic drift. Therefore, it is indicated that antigenic differences between the isolates from vaccinated and unvaccinated patients should be further evaluated using sera after infection. Generally, sera from ferrets infected with a different influenza virus are used for antigenic characterization. On the other hand, whether or not HI data obtained from ferret anti-sera correctly reflect the antigenicity of circulating viruses infecting humans continues to be under question. Some researchers have evaluated the use of sera from humans for antigenic characterization of influenza viruses.Citation29,30 However, this issue does not seem to be properly resolved using human anti-sera, because, in humans, immune responses after infection or vaccination might be different in each person, as mentioned above.Citation26–28
It has been suggested that if the genetic compatibility of vaccine strains with epidemic variants can be achieved, influenza vaccines would work effectively to prevent infection.Citation6,31 This efficacy seems to be independent of the information on anti-sera, because it depends only on the genetic similarity between vaccine strains and circulating viruses. Recently, HA prediction using sequence data alone was performed based on a computational analysis, in which the authors stressed on the model's value in predicting future influenza variants.Citation32 Sequence data-oriented analysis would better contribute to the field of influenza vaccine selection. Vaccine pressure may impact on HA mutations of influenza A/H3N2 epidemic viruses, which are more difficult to predict when compared to those of A/H1N1 and B. Therefore, careful observation of AA differences in epidemic viruses from their corresponding vaccine strains would be meaningful in the quest for effectively utilizing these data for HA prediction.
Disclosure of potential conflicts of interest
The authors indicate no potential conflicts of interest.
Acknowledgments
We thank Kenjiro Shirane, Shinya Matsumoto, and Nozomi Noda for their technical support. We also thank Hiroyuki Sasaki and Donchon Kang for their support on our study.Citation8
We thank the following doctors for participating in our study8: Shunsuke Akimitsu, Masaaki Chinen, Ken-ichi Doniwa, Keisuke Egashira, Yasuhiko Hirata, Miki Hirata, Nobuo Hirotsu, Tsuneo Inoue, Norio Iwaki, Kyosuke Kaji, Seizaburo Kashiwagi, Naoki Kawai, Takashi Kawashima, Kunio Kondo, Hiroko Kondo, Haruo Kuroki, Woon Joo Lee, Tetsunari Maeda, Koji Maehara, Tsuyoshi Makino, Shinro Matsuura, Akiko Miyata, Masashi Miyazaki, Kouichi Mochizuki, Kazuo Mori, Atsuko Nabeshima, Shin Nagao, Masatoshi Nakao, Kiyoshi Nishikawa, Yoshinori Nishimoto, Tadahiko Ogasawara, Jun Ogawa, Kaoru Oguchi, Yasuo Ontachi, Ietaka Sato, Yasuo Sato, Keigo Shibao, Kunihisa Shimomura, Shizuo Shindo, Kotoko Sumimoto, Yoshio Takasaki, Takeshi Tana, Osame Tanaka, Yuriko Tarukawa, Noriko Tateno, Keita Tatsushima, Satuki Tomita, Hiroaki Tomori, Hiroshi Ukai, Yutaka Wakasa, Masahiro Yaekashiwa, Norio Yamaguchi, Hareaki Yamamoto, Yasuhito Yamanishi, Yuji Yamashita, Satoshi Yamauchi, Takashi Yokoyama, Takato Yokoyama, Gen Yoshida, Hiroyuki Yoshimine, and Midori Yoshimura.
Funding
Our studyCitation8 was supported by a grant-in-aid from the Ministry of Education, Culture, Sports, Science and Technology of Japan (26461505 to Y.C.).
References
- Carrat F, Flahault A. Influenza vaccine: the challenge of antigenic drift. Vaccine. 2007;25:6852-62. doi:10.1016/j.vaccine.2007.07.027.
- Bedford T, Riley S, Barr IG, Broor S, Chadha M, Cox NJ, Daniels RS, Gunasekaran CP, Hurt AC, Kelso A, et al. Global circulation patterns of seasonal influenza viruses vary with antigenic drift. Nature. 2015;523:217-20. doi:10.1038/nature14460. PMID:26053121
- Barberis I, Myles P, Ault SK, Bragazzi NL, Martini M. History and evolution of influenza control through vaccination: from the first monovalent vaccine to universal vaccines. J Prev Med Hyg. 2016;57:E115−20. PMID:27980374
- Alan H, Ian B, Nancy C, Ruben O D, Siddhivinayak H, Daniel J, Jacqueline K, John M, Fernando M, Takato O, et al. Improving the selection and development of influenza vaccine viruses – Report of a WHO informal consultation on improving influenza vaccine virus selection, Hong Kong SAR, China, 18–20 November 2015. Vaccine. 2017;35:1104-9. doi:10.1016/j.vaccine.2017.01.018. PMID:28131392
- Belongia EA, Simpson MD, King JP, Sundaram ME, Kelley NS, Osterholm MT, McLean HQ. Variable influenza vaccine effectiveness by subtype: a systematic review and meta-analysis of test-negative design studies. Lancet Infect Dis. 2016;16:942-51. doi:10.1016/S1473-3099(16)00129-8. PMID:27061888
- Flannery B, Zimmerman RK, Gubareva LV, Garten RJ, Chung JR, Nowalk MP, Jackson ML, Jackson LA, Monto AS, Ohmit SE, et al. Enhanced Genetic Characterization of Influenza A(H3N2) Viruses and Vaccine Effectiveness by Genetic Group, 2014–2015. J Infect Dis. 2016;214:1010-9. doi:10.1093/infdis/jiw181. PMID:27190176
- Pebody RG, Warburton F, Ellis J, Andrews N, Thompson C, Wissmann von B, Green HK, Cottrell S, Johnston J, de Lusignan S, et al. Low effectiveness of seasonal influenza vaccine in preventing laboratory-confirmed influenza in primary care in the United Kingdom: 2014/15 mid-season results. Euro Surveill. 2015;20:21025. doi:10.2807/1560-7917.ES2015.20.5.21025. PMID:25677050
- Chong Y, Ikematsu H. Effect of seasonal vaccination on the selection of influenza A/H3N2 epidemic variants. Vaccine. 2017;35:255-63. doi:10.1016/j.vaccine.2016.11.084. PMID:27919631
- ARCHETTI I, HORSFALL FL. Persistent antigenic variation of influenza A viruses after incomplete neutralization in ovo with heterologous immune serum. J Exp Med. 1950;92:441-62. doi:10.1084/jem.92.5.441.
- Gerhard W, Webster RG. Antigenic drift in influenza A viruses. I. Selection and characterization of antigenic variants of A/PR/8/34 (HON1) influenza virus with monoclonal antibodies. J Exp Med. 1978;148:383-92. doi:10.1084/jem.148.2.383. PMID:359746
- Das SR, Hensley SE, Ince WL, Brooke CB, Subba A, Delboy MG, Russ G, Gibbs JS, Bennink JR, Yewdell JW. Defining influenza A virus hemagglutinin antigenic drift by sequential monoclonal antibody selection. Cell Host Microbe. 2013;13:314-23. doi:10.1016/j.chom.2013.02.008. PMID:23498956
- Boni MF. Vaccination and antigenic drift in influenza. Vaccine. 2008;26:C8−C14. doi:10.1016/j.vaccine.2008.04.011.
- Nelson MI, Holmes EC. The evolution of epidemic influenza. Nat Rev Genet. 2007;8:196-205. doi:10.1038/nrg2053.
- Wiley DC, Wilson IA, Skehel JJ. Structural identification of the antibody-binding sites of Hong Kong influenza haemagglutinin and their involvement in antigenic variation. Nature. 1981;289:373-8. doi:10.1038/289373a0. PMID:6162101
- Knossow M, Daniels RS, Douglas AR, Skehel JJ, Wiley DC. Three-dimensional structure of an antigenic mutant of the influenza virus haemagglutinin. Nature. 1984;311:678-80. doi:10.1038/311678a0.
- Smith DJ, Lapedes AS, de Jong JC, Bestebroer TM, Rimmelzwaan GF, Osterhaus ADME, Fouchier RAM. Mapping the antigenic and genetic evolution of influenza virus. Science. 2004;305:371-6. doi:10.1126/science.1097211.
- Hoelzer K, Murcia PR, Baillie GJ, Wood JLN, Metzger SM, Osterrieder N, Dubovi EJ, Holmes EC, Parrish CR. Intrahost evolutionary dynamics of canine influenza virus in naive and partially immune dogs. J Virol. 2010;84:5329-35. doi:10.1128/JVI.02469-09. PMID:20219907
- Murcia PR, Baillie GJ, Stack JC, Jervis C, Elton D, Mumford JA, Daly J, Kellam P, Grenfell BT, Holmes EC, et al. Evolution of equine influenza virus in vaccinated horses. J Virol. 2013;87:4768-71. doi:10.1128/JVI.03379-12.
- Swayne DE, Suarez DL, Spackman E, Jadhao S, Dauphin G, Kim-Torchetti M, McGrane J, Weaver J, Daniels P, Wong F, et al. Antibody titer has positive predictive value for vaccine protection against challenge with natural antigenic-drift variants of H5N1 high-pathogenicity avian influenza viruses from Indonesia. J Virol. 2015;89:3746-62. doi:10.1128/JVI.00025-15. PMID:25609805
- Connie Leung YH, Luk G, Sia S-F, Wu Y-O, Ho C-K, Chow K-C, Tang S-C, Guan Y, Malik Peiris JS. Experimental challenge of chicken vaccinated with commercially available H5 vaccines reveals loss of protection to some highly pathogenic avian influenza H5N1 strains circulating in Hong Kong/China. Vaccine. 2013;31:3536-42. doi:10.1016/j.vaccine.2013.05.076. PMID:23791547
- Cattoli G, Fusaro A, Monne I, Coven F, Joannis T, El-Hamid HSA, Hussein AA, Cornelius C, Amarin NM, Mancin M, et al. Evidence for differing evolutionary dynamics of A/H5N1 viruses among countries applying or not applying avian influenza vaccination in poultry. Vaccine. 2011;29:9368-75. doi:10.1016/j.vaccine.2011.09.127.
- Dinis JM, Florek NW, Fatola OO, Moncla LH, Mutschler JP, Charlier OK, Meece JK, Belongia EA, Friedrich TC. Deep Sequencing Reveals Potential Antigenic Variants at Low Frequencies in Influenza A Virus-Infected Humans. J Virol. 2016;90:3355-65. doi:10.1128/JVI.03248-15. PMID:26739054
- Debbink K, McCrone JT, Petrie JG, Truscon R, Johnson E, Mantlo EK, Monto AS, Lauring AS. Vaccination has minimal impact on the intrahost diversity of H3N2 influenza viruses. PLoS Pathog. 2017;13:e1006194. doi:10.1371/journal.ppat.1006194.
- Cushing A, Kamali A, Winters M, Hopmans ES, Bell JM, Grimes SM, Xia LC, Zhang NR, Moss RB, Holodniy M, et al. Emergence of Hemagglutinin Mutations During the Course of Influenza Infection. Sci Rep. 2015;5:16178. doi:10.1038/srep16178. PMID:26538451
- Sobel Leonard A, McClain MT, Smith GJD, Wentworth DE, Halpin RA, Lin X, Ransier A, Stockwell TB, Das SR, Gilbert AS, et al. Deep Sequencing of Influenza A Virus from a Human Challenge Study Reveals a Selective Bottleneck and Only Limited Intrahost Genetic Diversification. J Virol. 2016;90:11247-58. doi:10.1128/JVI.01657-16. PMID:27707932
- Fonville JM, Wilks SH, James SL, Fox A, Ventresca M, Aban M, Xue L, Jones TC, Le NMH, Pham QT, et al. Antibody landscapes after influenza virus infection or vaccination. Science. 2014;346:996-1000. doi:10.1126/science.1256427.
- Cobey S, Hensley SE. Immune history and influenza virus susceptibility. Curr Opin Virol. 2017;22:105-11. doi:10.1016/j.coviro.2016.12.004.
- Xie H, Li L, Ye Z, Li X, Plant EP, Zoueva O, Zhao Y, Jing X, Lin Z, Kawano T, et al. Differential Effects of Prior Influenza Exposures on H3N2 Cross-reactivity of Human Postvaccination Sera. Clin Infect Dis. 2017; 65:259-67. doi:10.1093/cid/cix269.
- Xie H, Wan X-F, Ye Z, Plant EP, Zhao Y, Xu Y, Li X, Finch C, Zhao N, Kawano T, et al. H3N2 Mismatch of 2014–15 Northern Hemisphere Influenza Vaccines and Head-to-head Comparison between Human and Ferret Antisera derived Antigenic Maps. Sci Rep. 2015;5:15279. doi:10.1038/srep15279. PMID:26472175
- Fonville JM, Fraaij PLA, de Mutsert G, Wilks SH, van Beek R, Fouchier RAM, Rimmelzwaan GF. Antigenic Maps of Influenza A(H3N2) Produced With Human Antisera Obtained After Primary Infection. J Infect Dis. 2016;213:31-8. doi:10.1093/infdis/jiv367. PMID:26142433
- DiazGranados CA, Dunning AJ, Kimmel M, Kirby D, Treanor J, Collins A, Pollak R, Christoff J, Earl J, Landolfi V, et al. Efficacy of high-dose versus standard-dose influenza vaccine in older adults. N Engl J Med. 2014;371:635-45. doi:10.1056/NEJMoa1315727. PMID:25119609
- Luksza M, Lässig M. A predictive fitness model for influenza. Nature. 2014;507:57-61. doi:10.1038/nature13087. PMID:24572367