Abstract
Streptococcus agalactiae (group B Streptococcus, GBS) is a leading cause of severe invasive disease in neonate, elderly, and immunocompromised patients worldwide. Despite recent advances in the diagnosis and intrapartum antibiotic prophylaxis (IAP) of GBS infections, it remains one of the most common causes of neonatal morbidity and mortality, causing serious infections. Furthermore, recent studies reported an increasing number of GBS infections in pregnant women and elderly. Although IAP is effective, it has several limitations, including increasing antimicrobial resistance and late GBS infection after negative antenatal screening. Maternal immunization is the most promising and effective countermeasure against GBS infection in neonates. However, no vaccine is available to date, but two types of vaccines, protein subunit and capsular polysaccharide conjugate vaccines, were investigated in clinical trials. Here, we provide an overview of the GBS vaccine development status and recent advances in the development of immunoassays to evaluate the GBS vaccine clinical efficacy.
Introduction
Group B streptococcus (Streptococcus agalactiae; GBS) is an opportunistic gram-positive pathogen and one of the most common causes of life-threatening bacterial infections worldwide. In the human neonates, GBS infection commonly results in the development of pneumonia, sepsis, and meningitis.Citation1,Citation2 Despite considerable advances in the diagnosis, prevention, and treatment of neonatal GBS infections, it remains an important public concern globally. Additionally, an increasing number of GBS infections in pregnant women and non-pregnant adults typically with underlying medical conditions, has been reported. In the first meeting of the Product Development for Vaccines Advisory Committee (PDVAC) convened by the World Health Organization (WHO) in 2014, GBS was identified as an important pathogen leading to a large burden of disease worldwide and a high priority for the development of a vaccine.Citation2 Although vaccination is the most promising strategy for the prevention of GBS infection, currently no licensed GBS vaccine is available in the market. Here, we reviewed and described the studies investigating this pathogen and potential future directions of GBS vaccine development and assay methods for the evaluation of the clinical efficacy of GBS vaccines.
Disease burden and clinical spectrum of GBS infections
GBS has been identified as a major cause of invasive infections during the first three months of life since the 1970s. The incidence of invasive GBS infections varies geographically, ranging from 0.02 per 1,000 live births in Southeast Asia to 1.21 per 1,000 live births in Africa.Citation3 GBS infection cases in the neonates and infants can be divided into two categories: an early-onset disease (EOD), which occurs within 0–6 days after birth, and a late-onset disease (LOD), which occurs within 7–90 days after birth. GBS is a common colonizer of digestive and female genital tract in approximately one-third of human population. Maternal colonization and subsequent neonatal acquisition of GBS is an established risk factor for GBS sepsis during the early periods of life.Citation4 Therefore, intrapartum antibiotic prophylaxis (IAP) strategy to reduce the neonatal acquisition of GBS has been applied, and the incidence of EOD declined from 1.8 cases per 1,000 live births in 1990 to 0.23 cases per 1,000 live births in 2015. However, IAP has had no impact on the incidence of LOD and only a limited impact on disease development in pregnant women. The incidence of LOD in the United States has remained stable since 1990 at approximately 0.3 to 0.4 per 1,000 live births.Citation5–Citation9 Moreover, approximately 60–80% of LOD occurred in infants whose mothers had negative results in the GBS screening at 35–37 weeks’ gestation.Citation10,Citation11 This may be due to the recolonization of GBS shortly before the delivery in these individuals, and their infants may acquire GBS from breast milk or diverse community/nosocomial sources after birth.Citation12
In neonates and infants younger than 3 months, GBS causes invasive bacterial diseases including sepsis, meningitis, and pneumonia (). An unexpected, rapidly progressing sepsis is the dominant manifestation of a GBS infection (80–85%) in EOD, while both primary bacteremia (65%) and meningitis (25–30%) are common in LOD.Citation8,Citation13–Citation15 Localized GBS infections, such as skin and soft tissue infections, bone and joint infections, and urinary tract infections, occasionally occur in LOD cases as well.Citation13 GBS is vertically transmitted to neonates during labor and delivery, so maternal GBS carriage is an important risk factor for EOD, particularly with obstetric complications, such as preterm rupture of membrane, preterm delivery, and prolonged rupture of membrane. GBS carriage rates in pregnant women vary geographically between 6.5% and 43.6%, with approximately 20–30% of pregnant women carrying GBS in the developed countries.Citation12,Citation16,Citation17 In about 50% of the cases, the infection is transmitted to their babies, leading to invasive diseases in 1% of neonatal carriers.Citation18
Table 1. Disease spectrum and serotype distribution of group B streptococcal (GBS) infection according to the age of the patient.
Although GBS infection has been primarily recognized as a pediatric disease, it has also emerged as an important pathogen colonizing pregnant and non-pregnant adults, particularly older ones or adults with underlying medical conditions. A two-to-four-fold increase in the incidence of invasive GBS diseases in adults has been observed over the past two decades, reaching 25.4 cases per 100,000 adults.Citation19 Approximately 5% of adults with these disease experienced relapse with an average of 13-week intervals.Citation20 Moreover, more than 50% of fatal GBS infections occur in elderly people.Citation19 Common clinical manifestations of adult GBS diseases includes skin and soft tissue infections, primary bacteremia, bone and joint infections, pneumonia, etc. ().Citation20 Contrary to the pregnancy-related cases, which occur in the otherwise healthy women, most of the non-pregnant adult patients with GBS infections have underlying medical conditions.Citation19,Citation20 Old age (≥65 years), diabetes mellitus, liver cirrhosis, stroke, and cancer are considered the common risk factors increasing the invasiveness of GBS infections among non-pregnant adults.Citation19,Citation20 GBS colonization rate at the genital and gastrointestinal tract ranges from 20% to 35% in adults irrespective of age.Citation20 However, in bed-ridden elderly people, GBS has been shown to colonize the dental plaque and pharynx as well,Citation20 increasing the probability of GBS pneumonia development in these patients.
Serotype distribution and antimicrobial resistance
Capsular polysaccharide (CPS) is an important virulence factor of encapsulated bacteria, including GBS, and has been related to the bacterial disease clinical manifestations and invasiveness.Citation21 Among 10 distinct serotypes, more than 90% of EOD are caused by serotypes Ia, Ib, II, III, and V, while LODs are caused predominantly by serotype III ().Citation17 Similar to the neonates and infants, maternal carriage has been associated with the GBS disease development in the pregnant women, with five-fold increase in the risk of disease development, including genitourinary tract infection, chorioamnionitis, and endometritis, compared with that in the non-pregnant women.Citation18 Pregnancy-associated GBS infections may lead to poor pregnancy outcomes, including spontaneous abortion, stillbirth, and preterm birth. Serotype distribution of clinical and colonized isolates was shown to be well correlated in pregnant women, and they were determined to be similar to those leading to the development of EOD ().Citation17,Citation18 In adults, serotype V (27.5%) was shown to be a predominant serotype, followed by Ia (24.3%) and III (16.5%).Citation19
Similar to pneumococci, some GBS capsular serotypes commonly colonize the gastrointestinal/genital tract, but rarely cause the development of invasive diseases. Other serotypes, however, are more likely to cause invasive diseases with each episode of colonization.Citation22–Citation26 Previously, the invasive disease-causing potential of each GBS capsular serotype was investigated, based on the invasive odds ratio (OR) ().Citation22–Citation26 Invasive OR is calculated by referring to all other serotypes as follows: OR = (ad)/(bc), where a is the number of invasive A serotypes, b is the number of carriage A serotypes, c is the number of invasive non-A serotypes, and d is the number of carriage non-A serotypes.Citation21 An OR of 1 indicates that the serotype is equally likely to cause invasive disease or be recovered from carriage, an OR>1 indicates an increased probability for a serotype to cause invasive disease, and an OR<1 indicates a reduced probability for a serotype to cause invasive disease. These studies demonstrated that serotype III is the predominant invasive serotype with high invasive OR (1.8-4.2).Citation22–Citation26 In a study conducted in Hong Kong, the virulence of serotype III was further assessed at subtype level, and serotype III-subtype 4 GBS was shown to be more highly invasive compared with other subtypes (invasive OR, 19.4).Citation26
Table 2. Serotype (ST)-specific invasive diseases potential of group B streptococci.Citation14,Citation22–Citation25
As recommended by the United States Centers for Disease Control and Prevention (CDC) guidelines for the IAP for the prevention of neonatal GBS infections, penicillin or ampicillin is administered to the pregnant women before delivery.Citation27 For the patients allergic to β-lactam agents, either erythromycin or clindamycin can be used as an alternative agent. However, an increasing rate of resistance to erythromycin and clindamycin has been observed among clinical GBS isolates ().Citation28–Citation33 High resistance rate against macrolide antibiotics was reported in South Korea (51.8%) and China (74.1%).Citation28,Citation29 In South Korea, serotype V was predominantly shown to have a high macrolide resistance rate, reflecting clonal spread with the selective advantage of antimicrobial resistance.Citation28 However, in China, more than 70% of GBS were resistant to macrolide antibiotics, irrespective of serotype.Citation29 In South Korea, 42.9% of the GBS isolates were shown to carry ermB, while in China, 52.3% of the isolates carried this gene, which provided a high level of resistance to both erythromycin and clindamycin.Citation28,Citation29
Table 3. Resistance rates (%) to macrolide antibiotics in group B streptococcus isolates.
Virulence factors for a GBS vaccine
GBS expresses numerous virulence factors that are involved in its colonization, adherence, invasion, and immune evasion,Citation34–Citation36 and these may be used as potential vaccine candidates. Biochemical and molecular analyses of these factors can provide a better understanding of the infectious process, further assisting the development of new diagnostic techniques, specific antimicrobial compounds, and effective vaccines.
Capsular Polysaccharide (CPS)
GBS expresses a unique CPS that is the most well-studied virulence factor contributing to the evasion of host immune defense mechanisms by protecting the bacteria from opsonophagocytosis by immune cells.Citation12,Citation37,Citation38 CPS can also increase the invasiveness of GBS by enhancing biofilm formation, inhibiting the binding of antimicrobial peptides and neutrophil extracellular traps (NET), and affecting bacterial adherence to the epithelium and mucus.Citation39–Citation41 Moreover, a correlation between the presence of CPS-specific antibodies in serum and the increased risk of GBS EOD and LOD development was reported,Citation42–Citation44 and CPS is considered the best target for the development of GBS vaccine.
Structure and biosynthesis
GBS expresses at least 10 structurally and antigenically different types of CPS (Ia, Ib, II, III, IV, V, VI, VII, VIII, and IX) ().Citation45,Citation46 All identified CPSs of GBS are high-molecular weight polymers with the short side-chain capped terminally with a sialic acid (N-acetylneuraminic acid) residue. Pneumococcal type 14 polysaccharide (Pn14) is structurally related to GBS type III polysaccharide (GBS-III), except for the presence of a terminal sialic acid residue in the side chain.Citation47–Citation50 However, immunoglobulin G (IgG), induced by the presence of Pn14, poorly cross-reacts with GBS-III polysaccharide, suggesting that the sialic acid-dependent functional epitope may provide the protective immunity.Citation47,Citation51 Moreover, sialylated CPS of GBS is recognized as a critical structural moiety for the attachment to human brain microvascular endothelium and evasion of human immune system.Citation52,Citation53
Figure 1. Genetic organization of the cps locus in Streptococcus agalactiae. (A) Comparative cps gene organization in nine serotypes: Ia (AB028896.2), Ib (AAJS01000021.1), II (ALQD01000015.1), III (AF163833.1), IV (AF355776.1), V (AF349539.1), VI (AF337958.1), VII (LT671990.1), VIII (ALST01000010.1), and IX (LT671992.1). Gene designations are indicated on each arrow. Similarity between the genes is indicated by the same or similar colors. Gene names are the same as those used in a previous study,Citation45 except for cpsP, cpsS, and cpsQ. (B) Predicted CPS functions based on the results of previous studies and sequence comparisons.
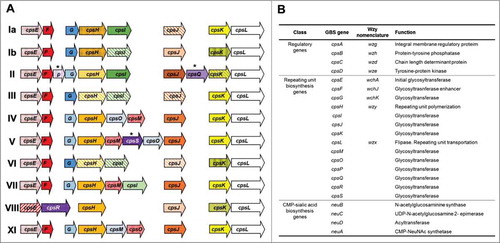
Based on the genetic structure of the GBS-CPS synthesis loci, the genes involved in CPS synthesis are located at the same chromosomal locus (cps) and are generally synthesized through the Wzx/Wzy-dependent pathway, similar to the pneumococcal CPS and Salmonella O-antigen synthesis (, A, and B).Citation37,Citation54 The locus contains the conserved genes (cpsA-D), whose products are involved in the regulation of capsule synthesis and the determination of the length of the repeating unit.Citation37,Citation55,Citation56 CpsA is a membrane anchoring protein and functions as a key regulator of CpsD phosphorylation.Citation56 CpsB, -C, and -D compose a phosphoregulatory system, where the CpsD autokinase phosphorylates its C-terminal tyrosine residues in a CpsC-dependent manner.Citation55 Capsule synthesis is initiated by the transfer of monosaccharide phosphate to a membrane-associated undecaprenyl-phosphate by CpsE,Citation57–Citation60 and the additional sugars are sequentially added to form a repeat unit through the activity of different transferases, such as CpsF-G, CpsI-K, and CpsM-S.Citation37,Citation60 The repeat unit is transferred to the outside of the cytoplasmic membrane by flippase (CpsL) and polymerized to form the mature CPS by polymerase (CpsH). High molecular weight CPS is covalently linked to GlcNAc C-6 of peptidoglycan, but the genetic and enzymatic mechanisms involved in polysaccharide-PGN ligation remain unclear.Citation61
Figure 2. Biochemical capsular polysaccharide (CPS) structure of Streptococcus agalactiae. Association of the encoded sugar transferases and polymerases (cpsH) with each corresponding CPS structure. Links between two sugars are represented as black lines (β1→4), red lines (β1→4), blue lines (β1→6), and green lines (α2→3).
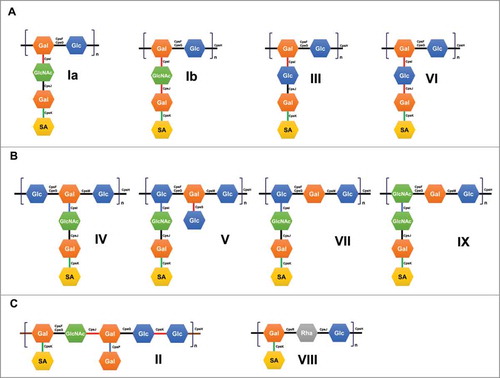
Figure 3. Representative Wzx/Wzy-dependent capsular polysaccharide (CPS) biosynthesis pathway in the group B streptococcal serotype III. (A) Serotype III cps gene organization and putative functions of the gene products.Citation37 (B) Biochemical steps during the CPS synthesis. Galactose-1-phosphate is initially transferred to an undecaprenyl-phosphate by CpsE and the repeat unit is rapidly assembled by glycosyltransferases. Individual repeat units are translocated across the cytoplasmic membrane by flippase (cpsL) and linked to form lipid-linked CPS by polymerase (cpsH).
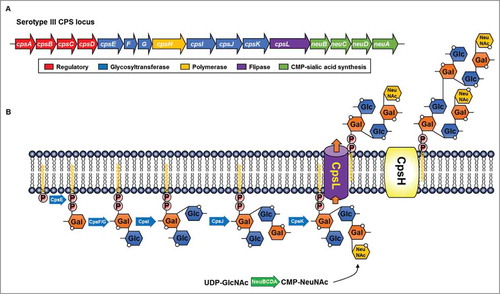
CPS vaccines
Around 1920, Avery and Heidelberger performed a series of studies establishing the bacterial capsule as a critical virulence factor of the encapsulated bacteria.Citation62–Citation69 Clinical trials investigating a multivalent polysaccharide vaccine demonstrated its high efficacy in humans against pneumococcal disease. Hexavalent, 14-valent, and 23-valent pneumococcal polysaccharide vaccines (PPVs) were licensed in 1947, 1977, and 1983, respectively, for the vaccination of adults and children.Citation70 GBS-CPS vaccine underwent clinical trials in healthy adults including pregnant women in the 1980s.Citation71–Citation75 Although tetravalent GBS polysaccharide vaccine with serotypes Ia, Ib, II, and III was shown to be well-tolerated, the proportion of subjects with more than four-fold increase in the serotype-specific Ig titers compared with those in the unvaccinated group was only 33% for serotype Ia, 0% for serotype Ib, 17% for serotype II, and 70% for serotype III.Citation73 During the PDVAC meeting in 2014, it was concluded that the native CPS vaccine was ineffective due to its poor immunogenicity.Citation76
Around 1980, CPS coupling to protein carriers, which transforms T-cell-independent CPS antigens to T-cell-dependent antigenic vaccines that can elicit a larger IgG response than the native CPS vaccine, was developed by Robbins and Schneerson.Citation77,Citation78 Monovalent or multivalent GBS CPS-protein conjugated vaccines (GBS-PCV) were designed and evaluated in clinical trials.Citation77–Citation93 In healthy adults, trivalent conjugate vaccines with serotypes Ia, Ib, and III were well tolerated, significantly more immunogenic than the uncoupled CPSs, and induced predominantly the generation of the IgG antibodies, which promote opsonophagocytic killing and are effectively transferred across the placenta.Citation81,Citation94 Currently, two large companies are undertaking efforts to develop GBS conjugate vaccines. GlaxoSmithKline (GSK) has conducted phase I/II trials with a trivalent CPS-CRM197 GBS conjugate vaccine (serotypes Ia, Ib, and III), and this company plans to conduct a clinical trial of the pentavalent GBS vaccine with serotypes Ia, Ib, II, III, and V.Citation1 Pfizer has also announced a phase 1/2 trial of pentavalent conjugate vaccine with serotypes Ia, Ib, II, III, and V to evaluate its safety, tolerability, and immunogenicity (NCT03170609).
Surface anchoring adhesins
Although the production, coverage, safety and immunogenicity of CPS conjugate vaccines have been well established, they show several limitations, includingCitation95–Citation98 (1) their limited usability in the low-income countries due to the high cost,Citation96 (2) potential immune interference with other type of conjugate vaccines,Citation99 (3) the possibility of serotype replacement and switching following the vaccination,Citation37,Citation100–Citation102 and (4) an increase in the occurrence of the unencapsulated GBS.Citation103–Citation105 Therefore, a structurally conserved protein antigen-based vaccine against GBS has been investigated as alternative vaccines.Citation106,Citation107 Conserved bacterial surface proteins play important roles during different stages of infection and likely represent the promising universal vaccine candidates.Citation106,Citation107,Citation108 To initiate infection and invasion of a specific organs, bacterial pathogens must first be able to attach to an appropriate target tissue by specific multiple tropisms between bacterial surface ligands and host receptors. Surface-anchoring adhesion molecules of GBS may therefore represent good candidates for vaccine development.
Alp protein family
Most well-known gram-positive bacterial surface proteins are cell wall proteins covalently anchored to the peptidoglycan layer through an LPXTG motif by the activity of enzyme sortase A (SrtA).Citation109 The genome of GBS encodes at least 20–25 LPXTG-linked surface proteins.Citation110–Citation112 Proteomic analysis of GBS was conducted to identify the major surface proteins of GBS, and Rip protein, one of Alp family protein, was found to be the most abundant surface protein.Citation110 Furthermore, over 90% of GBS clinical isolates were found to express or encode at least one of the Alp protein family genes.Citation113 Seven members of the Alp family, including AlphaC, BetaC, Alp1, Alp2, Alp3 (R28), Alp4, and Rib, have been identified to date. They contain an N-terminal secretion signal sequence (S), N-terminal conserved domain (N), a variable number of tandemly arranged repeats of 70–80 amino acids (R), 8 to 10 repeats, and a C-terminal LPXTG cell-wall anchoring motif (A).Citation111 Certain domains of Alp proteins may display high sequence similarity, which provides a structural basis for their interactions with the same host receptor and cross-protective immunity.Citation114 The roles of AlphaC and BetaC proteins have been extensively studied, compared with those of the other members of the Alp family. AlphaC protein was shown to be an important ligand involved in the GBS binding to human cervical epithelial cells through its interaction with glycosaminoglycan (GAG).Citation115 MLKKIE sequence motif of BetaC protein binds to the Fc region of human IgA, predominantly found on the mucus surface (B).Citation116,Citation117 Additionally, it was also shown to bind to human factor H (FH) to protect GBS from opsonophagocytosis.Citation118 Rib protein shares several biochemical features with AlphaC protein, but no immunological cross-reaction with either AlphaC or BetaC proteins has been found.Citation119 The potential invasive properties of other Alp proteins have not been studied.
Figure 4. Schematic representation of the group B streptococcal surface proteins. (A) Alp protein family. Signal peptide (S), conserved domain (CD), repeating domain (R), LPxTG cell-wall anchoring domain (green). (B) BetaC protein, containing IgA binding domain (blue) and factor H binding domain (brown). (C) Serine-rich repeat protein family. Ig-like domain (N1, N2, and N3), serine-rich repeat glycosylation domain (SRR1 and SRR2). (D) C5a peptidase. Protease activity domain (Protease), protease-associated domain (PA), fibronectin type III domain (Fn1, Fn2, Fn3). (E) Pilin proteins. Ig-like domain (D1-D3), pilin sorting motif (IPNTG, FPKTG, IPKTG), and a membrane-spanning hydrophobic domain (M).
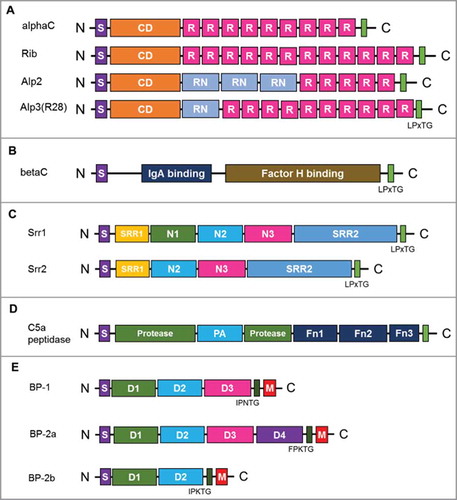
Preclinical vaccine investigations of the AlphaC, Alp3, and Rib proteins have been conducted, but the use of Alp proteins as universal vaccines has been limited due to the heterogeneity of the Alp sequence..Citation114,Citation120–Citation123 Nevertheless, MinervaX Inc. recently reported that the fusion protein of the highly immunogenic N-terminal domains of AlphC and Rib (GBS-NN) led to over 30-fold increase of GBS-NN-specific antibody in their phase I clinical trial with 240 healthy adult women (NCT02459262).Citation124
Serine-rich repeat proteins
Doro et al.Citation125 performed surfome analysis to identify GBS proteins with domains protruding from the bacterial surface. Among 43 surface-associated proteins identified using GBS COH1 strain (serotype III), serine-rich repeat 2 (Srr2) protein was shown to be the most abundant surface protein, which can be used to generate a protective immune response against GBS serotype III in mice. Serine-rich repeat (SRR) glycoproteins are a large and diverse family of adhesins found in most gram-positive bacteria.Citation126–Citation129 GBS expresses either one of two-allelic SRR proteins, Srr1 and Srr2,Citation130 with a highly conserved domain organization that includes a secretion signal sequence, two SRR domains that are glycosylated, a specialized fibrinogen binding domain between two SRR domains, and an LPXTG cell wall-anchoring motif (C). Both Srr1 and Srr2 identified in GBS can bind fibrinogen Aα chain through the “dock, lock, and latch” mechanism, and these interactions contributes to the pathogenesis of GBS meningitis and GBS colonization of the vaginal surface.Citation131–Citation133 An antigenic domain with 13 amino acids in Srr1 and Srr2, latch domain, was shown to be crucial for the pathogenesis of GBS diseases, and latch-peptide vaccination was demonstrated to provide serotype-independent protection against GBS infection in mice.Citation134
C5a peptidase (ScpB)
C5a peptidase is a highly conserved surface protein that is expressed on the surface of most GBS serotypes and can specifically inactivate a human phagocyte chemotaxin, C5a.Citation135–Citation138 C5a peptidase is also involved in GBS invasion, as it interacts with the human fibronectin through its RGD motif.Citation139,Citation140 This cell wall-anchoring protease contains N-terminal subtilisin-like protease domain, two RGD motifs targeting integrin, and three C-terminal fibronectin type III (Fn) domains (D).Citation137 C5a peptidase-deficient GBS pathogens were shown to be more rapidly cleared from mice supplemented with human C5a, suggesting that this peptidase is an important GBS virulence factor.Citation141 Recombinant C5a peptidase has been investigated as a universal protein vaccine or a carrier protein of GBS-CPS instead of the tetanus toxoid.Citation142,Citation143 In a murine model, antibodies raised against recombinant C5a peptidase were opsonic and enhanced phagocytic killing of various GBS serotypes. The immunization with C5a peptidase-conjugated GBS type III CPS led to an increase in the IgG immune response against both CPS and C5a peptidase. To enhance the immunogenicity of the recombinant C5a peptidase further, the researchers encapsulated it within microspheres composed of a lactic and glycolic acid co-polymer, which enabled this molecule to induce systemic and mucosal immune responses, offering protection against multiple GBS serotypes.Citation144,Citation145
Pilus
The genome sequences of five GBS serotypes were analyzed to identify pan-genome genes that encode putative surface-associated proteins and possible antigens suitable for the development of a universal GBS vaccine.Citation95,Citation146,Citation147 Among 396 core genes, pilin proteins were shown to induce a protective, serotype-independent immune response against GBS infection. Pili are long filamentous structures protruding from the bacterial surface, which are important for the bacterial virulence and disease pathogenesis.Citation148 Extensive genomic analyses of a large panel of GBS isolates revealed the presence of three pilus islands, PI-1, PI-2a, and PI-2b, which are further classified as pilus type 1, 2a, and 2b, respectively.Citation147,Citation149,Citation150 Each island encodes a pilus composed of three structural proteins, the major pilus subunit (backbone protein, BP) that forms the pilus shaft and two ancillary proteins that appear to be located at the pilus tip (AP1) and at the base (AP2) as anchor protein of the pilus to bacterial cell-wall.Citation151–Citation156 Although vaccination using either BP or AP1 induced protective immune responses against GBS, it was pilus type-specific and better in immunization with BP. Furthermore, at least six immunologically different variants were found in BP-2a, which limited BP for the use of vaccine development.Citation95,Citation149 Nuccitelli et al.Citation157 found that BP-2a variants share similar four Ig like domain (D1 to D4) and a D3 domain of BP-2a is a major epitope for a protective immune response. They further developed a six D3 fused chimeric protein from six BP-2a variants by using structural vaccine technology and showed strong protective immune responses against all six BP-2a variant carrying GBS strains. If this structural vaccine technology is further expanded to successfully include BP1 and BP-2b in a six D3 chimeric protein vaccine, a pilus is going to be a good vaccine candidate for a universal GBS protein vaccine.
Vaccine evaluation assays
Several vaccine candidates are under clinical and preclinical investigations, but the low baseline incidence of the primary endpoint of GBS invasive disease requires phase III clinical efficacy trials to be very large.Citation2 Based on a good correlation between immune response and clinical protection, some experts suggested that GBS vaccine can be approved based on the immunogenicity assay.Citation42,Citation158,Citation159 Similarly, Neisseria meningitides group C conjugate vaccine was successfully introduced in the UK on the basis of the immunogenicity assay results.Citation160 Therefore, the standardization of the clinical immunogenicity assays is urgently required for the development of GBS vaccines. The basic approach to the determination of vaccine immunogenicity is the measuring of antigen-specific antibody levels in the patient sera before and after vaccination, to determine whether an appropriate response has been induced.
For the PCV vaccine, two standard immunological methods, enzyme-linked immunosorbent assay (ELISA) and opsonophagocytic killing assay (OPKA) for measuring the quantity and quality of CPS-specific antibodies, are well established and accepted as the standard vaccine efficacy assays.Citation51,Citation161,Citation162 Although an immunogenicity assay for the analysis of GBS vaccine has been developed using certain modifications of the existing PCV vaccine assay, the modified protocol has not been standardized and validated in different laboratories to date, and consequently, the standardization of the GBS vaccine immunogenicity assays is necessary.
Antibody quantification
Standard ELISA can be used for the quantification of antibodies generated due to the immunization by protein-based vaccine.Citation163,Citation164 However, the capacity of this test to determine the levels of the antibodies against serotype-specific CPS antigens largely depends on the ability of the CPS immobilization on an ELISA plate, which can be accompanied by considerable technical difficulties, such as an inconsistent binding of immobilized CPS to the solid phase or a nonspecific serotype-independent binding with lower avidity.Citation85,Citation89,Citation91,Citation165 Despite the high degree of similarity between the repeat unit structure of CPS in different serotypes, their immunogenicity may quite differ. Therefore, methods used for the quantification of capsular serotype-specific antibody in serum must be not only sensitive, but also serotype-specific.Citation89 Baker and Kasper reported that the use of horse serum albumin-conjugated CPS obtained from different GBS serotypes as coating antigens results in at least 13- to 215-fold higher binding of antigens to the ELISA plates than when CPS alone is used and an improved sensitivity of the ELISA compared with that of the unconjugated CPS.Citation51,Citation81,Citation87,Citation89,Citation166 However, another study demonstrated that the chemical conjugation of CPSs and proteins as ELISA antigens can alter the antigenic structure of CPS, resulting in the reduction of antigenic specificity.Citation167 These results demonstrate that the design of specific ELISA protocol for the determination of serotype-specific GBS antibodies should be further optimized.
Assessment of functional antibodies
The quantity of antibodies generated against CPS or protein antigens highly correlates with the level of protection against GBS infections, but the functional quality of the antibodies induced by vaccines represents a critical determinant for the protection against GBS infections as well. Since the application of ELISA cannot differentiate between poorly functional antibodies with low avidity and the high-avidity antibodies, ELISAs may not be sufficient to determine the functional quality of antibodies.Citation168–Citation171 OPKA has been useful for the direct measurement of the protective capacity of antibodies, which function by opsonizing GBS for phagocytosis.Citation161 The classical OPKA is a tedious procedure for the examination of several serotype-specific OPKA in a large number of samples.Citation172,Citation173 For the clinical testing of pneumococcal vaccine efficacy, OPKA for pneumococcus has been modified to use a granulocytic cell line (HL60) that allows more convenient use of it with specificity and reproduciblity.Citation174 Additionally, this assay has been further simplified from a single OPKA to a multiplexed OPKA to reduce the assay time and the amount of serum required for the test.Citation175–Citation177 Evaluation of the functional efficacy of vaccine after immunization of pregnant women with GBS vaccine is performed in the newborns, in which extremely small amounts of serum can be obtained. With the technical advantages of the multiplexed OPKA, three-fold multiplexed OPKA for GBS (GBS-MOPA) has been developed, standardized, and validated to be used in newborns.Citation178 This standardized GBS-MOPA protocol enabled a practical, large-scale assessment of GBS vaccine immunogenicity against serotypes Ia, III, and V. An additional set of GBS-MOPA, covering all possible vaccine serotypes, is required to be developed.
Concluding remarks and perspectives
Despite the remarkable advances in the prevention and treatment of GBS infections over the recent decades, invasive GBS infections are still important public health problems, particularly in the neonates and infants. Although several vaccine candidates are under clinical development, a key issue of the phase III trials is the low baseline incidence of the primary clinical endpoints of GBS infections in both neonates and elderly. Additionally, the optimization of the number, concentrations, and timing of maternal vaccination conferring protection against GBS infections in both pregnant women and neonates is complicated. Therefore, it is critical to develop a standardized immunogenicity assay and establish GBS serotype-specific protective cut-off values to succeed in the development of effective vaccines. Several efforts were made to modify the standard immunogenicity assay for pneumococcal PCV for the application in the GBS vaccine development, however, several concerns were highlighted here. First, no reference serum for the standardization of GBS ELISA is available, furthermore, the immobilization of CPS on ELISA plate has to be optimized, and finally, the low affinity of natural and non-specific binding antibodies. Moreover, standard immunogenicity assays should be further optimized and validated in multiple laboratories across different counties, together with the worldwide epidemiological studies of the GBS serotype and genotype distribution. After the introduction of pneumococcal PCV, new serotypes and serotype replacement were identified in the countries where PCV has been used nationwide. Due to this, the vaccine effectiveness and changes in the disease incidence should be constantly assessed and monitored before and after the licensing and implementation of GBS vaccine.
Disclosure of potential conflicts of interest
No potential conflicts of interest were disclosed.
Additional information
Funding
References
- Kobayashi M, Vekemans J, Baker CJ, Ratner AJ, Le Doare K, Schrag SJ. Group B streptococcus vaccine development: present status and future considerations, with emphasis on perspectives for low and middle income countries. F1000Res. 2016;5:2355. doi:10.12688/f1000research.9363.1.
- Kobayashi M, Schrag SJ, Alderson MR, Madhi SA, Baker CJ, Sobanjo-Ter Meulen A, Kaslow DC, Smith PG, Moorthy VS, Vekemans J. WHO consultation on group B Streptococcus vaccine development: Report from a meeting held on 27–28 April 2016. Vaccine. 2016;16:31236–1. doi:10.1016/j.vaccine.2016.12.029.
- Kwatra G, Cunnington MC, Merrall E, Adrian PV, Ip M, Klugman KP, Tam WH, Madhi SA. Prevalence of maternal colonisation with group B streptococcus: a systematic review and meta-analysis. Lancet Infect Dis. 2016;16:1076–84. doi:10.1016/S1473-3099(16)30055-X.
- Farley MM. Group B streptococcal disease in nonpregnant adults. Clin Infect Dis. 2001;33:556–61. doi:10.1086/322696.
- Zangwill KM, Schuchat A, Wenger JD. Group B streptococcal disease in the United States, 1990: report from a multistate active surveillance system. MMWR CDC Surveill Summ. 1992;41:25–32.
- Centers for Disease C, Prevention. Early-onset and late-onset neonatal group B streptococcal disease–United States, 1996–2004. MMWR Morb Mortal Wkly Rep. 2005;54:1205–8.
- Centers for Disease C, Prevention. Perinatal group B streptococcal disease after universal screening recommendations–United States, 2003–2005. MMWR Morb Mortal Wkly Rep. 2007;56:701–5.
- Phares CR, Lynfield R, Farley MM, Mohle-Boetani J, Harrison LH, Petit S, Craig AS, Schaffner W, Zansky SM, Gershman K, et al. Epidemiology of invasive group B streptococcal disease in the United States, 1999–2005. JAMA. 2008;299:2056–65. doi:10.1001/jama.299.17.2056.
- Jordan HT, Farley MM, Craig A, Mohle-Boetani J, Harrison LH, Petit S, Lynfield R, Thomas A, Zansky S, Gershman K, et al. Revisiting the need for vaccine prevention of late-onset neonatal group B streptococcal disease: a multistate, population-based analysis. Pediatr Infect Dis J. 2008;27:1057–64. doi:10.1097/INF.0b013e318180b3b9.
- Melin P. Neonatal group B streptococcal disease: from pathogenesis to preventive strategies. Clin Microbiol Infect. 2011;17:1294–303. doi:10.1111/j.1469-0691.2011.03576.x.
- Van Dyke MK, Phares CR, Lynfield R, Thomas AR, Arnold KE, Craig AS, Mohle-Boetani J, Gershman K, Schaffner W, Petit S, et al. Evaluation of universal antenatal screening for group B streptococcus. N Engl J Med. 2009;360:2626–36. doi:10.1056/NEJMoa0806820.
- Nuccitelli A, Rinaudo CD, Maione D. group B streptococcus vaccine: state of the art. Ther Adv Vaccines. 2015;3:76–90. doi:10.1177/2051013615579869.
- Edward MS, Nizet V, Baker CJ. Group B streptococcal infection. In: Remington JS, Klien JO, Baker CJ, Wilson CB, eds. Infectious diseases of the fetus and newborn infant,. Philadelphia: WB Saunders Co, 2006:1091–141.
- Berardi A, Rossi C, Lugli L, Creti R, Bacchi Reggiani ML, Lanari M, Memo L, Pedna MF, Venturelli C, Perrone E, et al. Group B streptococcus late-onset disease: 2003–2010. Pediatrics. 2013;131:e361–8. doi:10.1542/peds.2012-1231.
- Pena BM, Harper MB, Fleisher GR. Occult bacteremia with group B streptococci in an outpatient setting. Pediatrics. 1998;102:67–72. doi:10.1542/peds.102.1.67.
- Kim EJ, Oh KY, Kim MY, Seo YS, Shin JH, Song YR, Yang JH, Foxman B, Ki M. Risk factors for group B streptococcus colonization among pregnant women in Korea. Epidemiol Health. 2011;33:e2011010. doi:10.4178/epih/e2011010.
- Seale AC, Bianchi-Jassir F, Russell NJ, Kohli-Lynch M, Tann CJ, Hall J, Madrid L, Blencowe H, Cousens S, Baker CJ, et al. Estimates of the burden of group B streptococcal disease worldwide for pregnant women, stillbirths, and children. Clin Infect Dis. 2017;65:S200–S19. doi:10.1093/cid/cix664.
- Le Doare K, Heath PT. An overview of global GBS epidemiology. Vaccine. 2013;31:D7–D12. doi:10.1016/j.vaccine.2013.01.009.
- Edwards MS, Baker CJ. Group B streptococcal infections in elderly adults. Clin Infect Dis. 2005;41:839–47. doi:10.1086/432804.
- Sendi P, Johansson L, Norrby-Teglund A. Invasive group B streptococcal disease in non-pregnant adults – A review with emphasis on skin and soft-tissue infections. Infection. 2008;36:100–11. doi:10.1007/s15010-007-7251-0.
- Song JY, Nahm MH, Moseley MA. Clinical implications of pneumococcal serotypes: invasive disease potential, clinical presentations, and antibiotic resistance. J Korean Med Sci. 2013;28:4–15. doi:10.3346/jkms.2013.28.1.4.
- Berg S, Trollfors B, Lagergard T, Zackrisson G, Claesson BA. Serotypes and clinical manifestations of group B streptococcal infections in western Sweden. Clin Microbiol Infect. 2000;6:9–13. doi:10.1046/j.1469-0691.2000.00007.x.
- Martins ER, Pessanha MA, Ramirez M, Melo-Cristino J, Portuguese Group for the Study of Streptococcal I. Analysis of group B streptococcal isolates from infants and pregnant women in Portugal revealing two lineages with enhanced invasiveness. J Clin Microbiol 2007; 45:3224–9. doi:10.1128/JCM.01182-07.
- Bisharat N, Jones N, Marchaim D, Block C, Harding RM, Yagupsky P, Peto T, Crook DW. Population structure of group B streptococcus from a low-incidence region for invasive neonatal disease. Microbiology. 2005;151:1875–81. doi:10.1099/mic.0.27826-0.
- Madzivhandila M, Adrian PV, Cutland CL, Kuwanda L, Schrag SJ, Madhi SA. Serotype distribution and invasive potential of group B streptococcus isolates causing disease in infants and colonizing maternal-newborn dyads. PloS One. 2011;6:e17861. doi:10.1371/journal.pone.0017861.
- Ip M, Ang I, Fung K, Liyanapathirana V, Luo MJ, Lai R. Hypervirulent Clone of group B streptococcus Serotype III Sequence Type 283, Hong Kong, 1993–2012. Emerg Infect Dis. 2016;22:1800–3. doi:10.3201/eid2210.151436.
- Schrag S, Gorwitz R, Fultz-Butts K, Schuchat A. Prevention of perinatal group B streptococcal disease. Revised guidelines from CDC. MMWR Recomm Rep. 2002;51:1–22.
- Yoon IA, Jo DS, Cho EY, Choi EH, Lee HJ, Lee H. Clinical significance of serotype V among infants with invasive group B streptococcal infections in South Korea. Int J Infect Dis. 2015;38:136–40. doi:10.1016/j.ijid.2015.05.017.
- Lu B, Chen X, Wang J, Wang D, Zeng J, Li Y, Li D, Zhu F, Cui Y, Huang L. Molecular characteristics and antimicrobial resistance in invasive and noninvasive group B streptococcus between 2008 and 2015 in China. Diagn Microbiol Infect Dis. 2016;86:351–7. doi:10.1016/j.diagmicrobio.2016.08.023.
- Morozumi M, Wajima T, Kuwata Y, Chiba N, Sunaoshi K, Sugita K, Sakata H, Iwata S, Ubukata K. Associations between capsular serotype, multilocus sequence type, and macrolide resistance in Streptococcus agalactiae isolates from Japanese infants with invasive infections. Epidemiol Infect. 2014;142:812–9. doi:10.1017/S0950268813001647.
- von Both U, Ruess M, Mueller U, Fluegge K, Sander A, Berner R. A serotype V clone is predominant among erythromycin-resistant Streptococcus agalactiae isolates in a southwestern region of Germany. J Clin Microbiol. 2003;41:2166–9. doi:10.1128/JCM.41.5.2166-2169.2003.
- Lin FY, Azimi PH, Weisman LE, Philips JB, 3rd, Regan J, Clark P, Rhoads GG, Clemens J, Troendle J, Pratt E, et al. Antibiotic susceptibility profiles for group B streptococci isolated from neonates, 1995–1998. Clin Infect Dis. 2000;31:76–9. doi:10.1086/313936.
- Dutra VG, Alves VM, Olendzki AN, Dias CA, de Bastos AF, Santos GO, de Amorin EL, Sousa MÂ, Santos R, Ribeiro PC, et al. Streptococcus agalactiae in Brazil: serotype distribution, virulence determinants and antimicrobial susceptibility. BMC Infect Dis. 2014;14:323. doi:10.1186/1471-2334-14-323.
- Rajagopal L. Understanding the regulation of group B streptococcal virulence factors. Future Microbiol. 2009;4:201–21. doi:10.2217/17460913.4.2.201.
- Herbert MA, Beveridge CJ, Saunders NJ. Bacterial virulence factors in neonatal sepsis: group B streptococcus. Curr Opin Infect Dis. 2004;17:225–9. doi:10.1097/00001432-200406000-00009.
- Vornhagen J, Adams Waldorf KM, Rajagopal L. Perinatal group B streptococcal infections: Virulence factors, immunity, and prevention strategies. Trends Microbiol. 2017;25:919–31. doi:10.1016/j.tim.2017.05.013.
- Geno KA, Gilbert GL, Song JY, Skovsted IC, Klugman KP, Jones C, Konradsen HB, Nahm MH. Pneumococcal capsules and their types: Past, present, and future. Clin Microbiol Rev. 2015;28:871–99. doi:10.1128/CMR.00024-15.
- Croney CM, Nahm MH, Juhn SK, Briles DE, Crain MJ. Invasive and noninvasive Streptococcus pneumoniae capsule and surface protein diversity following the use of a conjugate vaccine. Clin Vaccine Immunol. 2013;20:1711–8. doi:10.1128/CVI.00381-13.
- D'Urzo N, Martinelli M, Pezzicoli A, De Cesare V, Pinto V, Margarit I, Telford JL, Maione D. Acidic pH strongly enhances in vitro biofilm formation by a subset of hypervirulent ST-17 Streptococcus agalactiae strains. Appl Environ Microbiol. 2014;80:2176–85. doi:10.1128/AEM.03627-13.
- Rosini R, Margarit I. Biofilm formation by Streptococcus agalactiae: influence of environmental conditions and implicated virulence factors. Front Cell Infect Microbiol. 2015;5:6. doi:10.3389/fcimb.2015.00006.
- Xia FD, Mallet A, Caliot E, Gao C, Trieu-Cuot P, Dramsi S. Capsular polysaccharide of group B streptococcus mediates biofilm formation in the presence of human plasma. Microbes Infect. 2015;17:71–6. doi:10.1016/j.micinf.2014.10.007.
- Baker CJ, Carey VJ, Rench MA, Edwards MS, Hillier SL, Kasper DL, Platt R. Maternal antibody at delivery protects neonates from early onset group B streptococcal disease. J Infect Dis. 2014;209:781–8. doi:10.1093/infdis/jit549.
- Baker CJ, Kasper DL. Correlation of maternal antibody deficiency with susceptibility to neonatal group B streptococcal infection. N Engl J Med. 1976;294:753–6. doi:10.1056/NEJM197604012941404.
- Baker CJ, Kasper DL, Tager I, Paredes A, Alpert S, McCormack WM, Goroff D. Quantitative determination of antibody to capsular polysaccharide in infection with type III strains of group B Streptococcus. J Clin Invest. 1977;59:810–8. doi:10.1172/JCI108703.
- Cieslewicz MJ, Chaffin D, Glusman G, Kasper D, Madan A, Rodrigues S, Fahey J, Wessels MR, Rubens CE. Structural and genetic diversity of group B streptococcus capsular polysaccharides. Infect Immun. 2005;73:3096–103. doi:10.1128/IAI.73.5.3096-3103.2005.
- Berti F, Campisi E, Toniolo C, Morelli L, Crotti S, Rosini R, Romano MR, Pinto V, Brogioni B, Torricelli G, et al. Structure of the type IX group B Streptococcus capsular polysaccharide and its evolutionary relationship with types V and VII. J Biol Chem. 2014;289:23437–48. doi:10.1074/jbc.M114.567974.
- Carboni F, Adamo R, Fabbrini M, De Ricco R, Cattaneo V, Brogioni B, Veggi D, Pinto V, Passalacqua I, Oldrini D, et al. Structure of a protective epitope of group B Streptococcus type III capsular polysaccharide. Proc Natl Acad Sci U S A. 2017;114:5017–22. doi:10.1073/pnas.1701885114.
- Yamamoto S, Miyake K, Koike Y, Watanabe M, Machida Y, Ohta M, Iijima S. Molecular characterization of type-specific capsular polysaccharide biosynthesis genes of Streptococcus agalactiae type Ia. J Bacteriol. 1999;181:5176–84.
- Kolkman MA, Wakarchuk W, Nuijten PJ, van der Zeijst BA. Capsular polysaccharide synthesis in Streptococcus pneumoniae serotype 14: molecular analysis of the complete cps locus and identification of genes encoding glycosyltransferases required for the biosynthesis of the tetrasaccharide subunit. Mol Microbiol. 1997;26:197–208. doi:10.1046/j.1365-2958.1997.5791940.x.
- Chaffin DO, Beres SB, Yim HH, Rubens CE. The serotype of type Ia and III group B streptococci is determined by the polymerase gene within the polycistronic capsule operon. J Bacteriol. 2000;182:4466–77. doi:10.1128/JB.182.16.4466-4477.2000.
- Guttormsen HK, Baker CJ, Nahm MH, Paoletti LC, Zughaier SM, Edwards MS, Kasper DL. Type III group B streptococcal polysaccharide induces antibodies that cross-react with Streptococcus pneumoniae type 14. Infect Immun. 2002;70:1724–38. doi:10.1128/IAI.70.4.1724-1738.2002.
- Carlin AF, Chang YC, Areschoug T, Lindahl G, Hurtado-Ziola N, King CC, Varki A, Nizet V. group B streptococcus suppression of phagocyte functions by protein-mediated engagement of human Siglec-5. J Exp Med. 2009;206:1691–9. doi:10.1084/jem.20090691.
- Chang YC, Olson J, Beasley FC, Tung C, Zhang J, Crocker PR, Varki A, Nizet V. group B streptococcus engages an inhibitory Siglec through sialic acid mimicry to blunt innate immune and inflammatory responses in vivo. PLoS Pathog. 2014;10:e1003846. doi:10.1371/journal.ppat.1003846.
- Schnaitman CA, Klena JD. Genetics of lipopolysaccharide biosynthesis in enteric bacteria. Microbiol Rev. 1993;57:655–82.
- Toniolo C, Balducci E, Romano MR, Proietti D, Ferlenghi I, Grandi G, Berti F, Ros IM, Janulczyk R. Streptococcus agalactiae capsule polymer length and attachment is determined by the proteins CpsABCD. J Biol Chem. 2015; 290:9521–32. doi:10.1074/jbc.M114.631499.
- Hanson BR, Runft DL, Streeter C, Kumar A, Carion TW, Neely MN. Functional analysis of the CpsA protein of Streptococcus agalactiae. J Bacteriol. 2012;194:1668–78. doi:10.1128/JB.06373-11.
- Cartee RT, Forsee WT, Bender MH, Ambrose KD, Yother J. CpsE from type 2 Streptococcus pneumoniae catalyzes the reversible addition of glucose-1-phosphate to a polyprenyl phosphate acceptor, initiating type 2 capsule repeat unit formation. J Bacteriol. 2005;187:7425–33. doi:10.1128/JB.187.21.7425-7433.2005.
- Al-Dabbagh B, Henry X, El Ghachi M, Auger G, Blanot D, Parquet C, Mengin-Lecreulx D, Bouhss A. Active site mapping of MraY, a member of the polyprenyl-phosphate N-acetylhexosamine 1-phosphate transferase superfamily, catalyzing the first membrane step of peptidoglycan biosynthesis. Biochemistry. 2008;47:8919–28. doi:10.1021/bi8006274.
- Barreteau H, Magnet S, El Ghachi M, Touze T, Arthur M, Mengin-Lecreulx D, Blanot D. Quantitative high-performance liquid chromatography analysis of the pool levels of undecaprenyl phosphate and its derivatives in bacterial membranes. J Chromatogr B Analyt Technol Biomed Life Sci. 2009;877:213–20. doi:10.1016/j.jchromb.2008.12.010.
- James DB, Gupta K, Hauser JR, Yother J. Biochemical activities of Streptococcus pneumoniae serotype 2 capsular glycosyltransferases and significance of suppressor mutations affecting the initiating glycosyltransferase Cps2E. J Bacteriol. 2013;195:5469–78. doi:10.1128/JB.00715-13.
- Larson TR, Yother J. Streptococcus pneumoniae capsular polysaccharide is linked to peptidoglycan via a direct glycosidic bond to beta-D-N-acetylglucosamine. Proc Natl Acad Sci U S A. 2017;114:5695–700. doi:10.1073/pnas.1620431114.
- Avery OT, Dubos R. The protective action of a specific enzyme against type III pneumococcus infection in mice. J Exp Med. 1931;54:73–89. doi:10.1084/jem.54.1.73.
- MacLeod CM, Hodges RG, Heidelberger M, Bernhard WG. Prevention of pneumococcal pneumonia by immunization with specific capsular polysaccharides. JEM. 1945;82:445–65. doi:10.1084/jem.82.6.445.
- Heidelberger M, Tyler JM. Cross-reactions of pneumococcal types. J Exp Med. 1964;120:711–9. doi:10.1084/jem.120.5.711.
- Heidelberger M. Precipitating cross-reactions among pneumococcal types. Infect Immun. 1983;41:1234–44.
- Heidelberger M, Rebers PA. Immunochemistry of the pneumococcal types II, V, and VI. The relation of type VI to type II and other correlations between chemical constitution and precipitation in antisera to type VI. J Bacteriol. 1960;80:145–53.
- Heidelberger M, Bernheimer AW. Cross-reactions of polysaccharides of fungi, molds, and yeasts in anti-pneumococcal and other antisera. Proc Nat Acad Sci USA. 1984;81:5247–9. doi:10.1073/pnas.81.16.5247.
- MacPherson CFC, Heidelberger M, Alexander HE, Leidy G. The specific polysaccharides of types A,B,C,D, and F Hemophilus influenzae. J Immunol. 1946;52:207–19.
- Heidelberger M. A “pure” organic chemists downward path. Annu Rev Microbiol. 1977;31:1–12. doi:10.1146/annurev.mi.31.100177.000245.
- Melegaro A, Edmunds WJ. The 23-valent pneumococcal polysaccharide vaccine. Part I. Efficacy of PPV in the elderly: a comparison of meta-analyses. Eur J Epidemiol. 2004;19:353–63. doi:10.1023/B:EJEP.0000024701.94769.98.
- Baker CJ, Edwards MS, Kasper DL. Immunogenicity of polysaccharides from type III, group B Streptococcus. J Clin Invest. 1978;61:1107–10. doi:10.1172/JCI109011.
- Kasper DL, Goroff DK, Baker CJ. Immunochemical characterization of native polysaccharides from group B streptococcus: the relationship of the type III and group B determinants. J Immunol. 1978;121:1096–105.
- Basham LE, Pavliak V, Li X, Hawwari A, Kotloff KL, Edelman R, Fattom A. A simple, quantitative, reproducible avidin-biotin ELISA for the evaluation of group B streptococcus type-specific antibodies in humans. Vaccine. 1996;14:439–45. doi:10.1016/0264-410X(95)00146-R.
- Larsen JW, Jr, Harper JS, 3rd, London WT, Baker CJ, Curfman BL, Kasper DL, Sever JL. Antibody to type III group B Streptococcus in the rhesus monkey. Am J Obstet Gynecol. 1983;146:958–62. doi:10.1016/0002-9378(83)90973-0.
- De Cueninck BJ, Eisenstein TK, McIntosh TS, Shockman GD, Swenson RM. Quantitation of in vitro opsonic activity of human antibody induced by a vaccine consisting of the type III-specific polysaccharide of group B streptococcus. Infect Immun. 1983;39:1155–60.
- Modjarrad K, Giersing B, Kaslow DC, Smith PG, Moorthy VS, Group WRVCE. WHO consultation on respiratory syncytial virus vaccine development report from a World Health Organization Meeting held on 23–24 March 2015. Vaccine. 2016;34:190–7. doi:10.1016/j.vaccine.2015.05.093.
- Miernyk KM, Butler JC, Bulkow LR, Singleton RJ, Hennessy TW, Dentinger CM, Peters HV, Knutsen B, Hickel J, Parkinson AJ. Immunogenicity and reactogenicity of pneumococcal polysaccharide and conjugate vaccines in alaska native adults 55–70 years of age. Clin Infect Dis. 2009;49:241–8. doi:10.1086/599824.
- Goldblatt D, Southern J, Andrews N, Ashton L, Burbidge P, Woodgate S, Pebody R, Miller E. The immunogenicity of 7-valent pneumococcal conjugate vaccine versus 23-valent polysaccharide vaccine in adults aged 50–80 years. Clin Infect Dis. 2009;49:1318–25. doi:10.1086/606046.
- Baker CJ, Rench MA, Fernandez M, Paoletti LC, Kasper DL, Edwards MS. Safety and immunogenicity of a bivalent group B streptococcal conjugate vaccine for serotypes II and III. J Infect Dis. 2003;188:66–73. doi:10.1086/375536.
- Paoletti LC, Wessels MR, Michon F, DiFabio J, Jennings HJ, Kasper DL. group B streptococcus type II polysaccharide-tetanus toxoid conjugate vaccine. Infect Immun. 1992;60:4009–14.
- Baker CJ, Paoletti LC, Rench MA, Guttormsen HK, Carey VJ, Hickman ME, Kasper DL. Use of capsular polysaccharide-tetanus toxoid conjugate vaccine for type II group B Streptococcus in healthy women. J Infect Dis. 2000;182:1129–38. doi:10.1086/315839.
- Wessels MR, Paoletti LC, Guttormsen HK, Michon F, D'Ambra AJ, Kasper DL. Structural properties of group B streptococcal type III polysaccharide conjugate vaccines that influence immunogenicity and efficacy. Infect Immun. 1998;66:2186–92.
- Paoletti LC, Kasper DL. Conjugate vaccines against group B Streptococcus types IV and VII. J Infect Dis. 2002;186:123–6. doi:10.1086/341073.
- Paoletti LC, Kennedy RC, Chanh TC, Kasper DL. Immunogenicity of group B Streptococcus type III polysaccharide-tetanus toxoid vaccine in baboons. Infect Immun. 1996;64:677–9.
- Kasper DL, Paoletti LC, Wessels MR, Guttormsen HK, Carey VJ, Jennings HJ, Baker CJ. Immune response to type III group B streptococcal polysaccharide-tetanus toxoid conjugate vaccine. J Clin Invest. 1996;98:2308–14. doi:10.1172/JCI119042.
- Vekemans J, Moorthy V, Friede M, Alderson MR, Sobanjo-Ter Meulen A, Baker CJ, Heath PT, Madhi SA, Mehring-Le Doare K, Saha SK, et al. Maternal immunization against Group B streptococcus: World Health Organization research and development technological roadmap and preferred product characteristics. Vaccine. 2018;17:31359–2. doi:10.1016/j.vaccine.2017.09.087.
- Baker CJ, Paoletti LC, Wessels MR, Guttormsen HK, Rench MA, Hickman ME, Kasper DL. Safety and immunogenicity of capsular polysaccharide-tetanus toxoid conjugate vaccines for group B streptococcal types Ia and Ib. J Infect Dis. 1999;179:142–50. doi:10.1086/314574.
- Paoletti LC, Rench MA, Kasper DL, Molrine D, Ambrosino D, Baker CJ. Effects of alum adjuvant or a booster dose on immunogenicity during clinical trials of group B streptococcal type III conjugate vaccines. Infect Immun. 2001;69:6696–701. doi:10.1128/IAI.69.11.6696-6701.2001.
- Brigtsen AK, Kasper DL, Baker CJ, Jennings HJ, Guttormsen HK. Induction of cross-reactive antibodies by immunization of healthy adults with types Ia and Ib group B streptococcal polysaccharide-tetanus toxoid conjugate vaccines. J Infect Dis. 2002;185:1277–84. doi:10.1086/340324.
- Baker CJ, Edwards MS. Group B streptococcal conjugate vaccines. Arch Dis Child. 2003;88:375–8. doi:10.1136/adc.88.5.375.
- Baker CJ, Paoletti LC, Rench MA, Guttormsen HK, Edwards MS, Kasper DL. Immune response of healthy women to 2 different group B streptococcal type V capsular polysaccharide-protein conjugate vaccines. J Infect Dis. 2004;189:1103–12. doi:10.1086/382193.
- Pannaraj PS, Edwards MS, Ewing KT, Lewis AL, Rench MA, Baker CJ. Group B streptococcal conjugate vaccines elicit functional antibodies independent of strain O-acetylation. Vaccine. 2009;27:4452–6. doi:10.1016/j.vaccine.2009.05.039.
- Heyderman RS, Madhi SA, French N, Cutland C, Ngwira B, Kayambo D, Mboizi R, Koen A, Jose L, Olugbosi M, et al. Group B streptococcus vaccination in pregnant women with or without HIV in Africa: a non-randomised phase 2, open-label, multicentre trial. Lancet Infect Dis. 2016;16:546–55. doi:10.1016/S1473-3099(15)00484-3.
- Kasper DL, Wessels MR, Guttormsen HK, Paoletti LC, Edwards MS, Baker CJ. Measurement of human antibodies to type III group B Streptococcus. Infect Immun. 1999;67:4303–5.
- Maione D, Margarit I, Rinaudo CD, Masignani V, Mora M, Scarselli M, Tettelin H, Brettoni C, Iacobini ET, Rosini R, et al. Identification of a universal Group B streptococcus vaccine by multiple genome screen. Science. 2005;309:148–50. doi:10.1126/science.1109869.
- Tettelin H, Medini D, Donati C, Masignani V. Towards a universal group B Streptococcus vaccine using multistrain genome analysis. Expert Rev Vaccines. 2006;5:687–94. doi:10.1586/14760584.5.5.687.
- Aguiar SI, Serrano I, Pinto FR, Melo-Cristino J, Ramirez M, Portuguese Surveillance Group for the Study of Respiratory P. Changes in Streptococcus pneumoniae serotypes causing invasive disease with non-universal vaccination coverage of the seven-valent conjugate vaccine. Clin Microbiol Infect. 2008;14:835–43. doi:10.1111/j.1469-0691.2008.02031.x.
- Gonzalez BE, Jacobs MR. The potential of human nasal colonization with Streptococcus pneumoniae as a universal pneumococcal vaccine. Am J Respir Crit Care Med. 2013;187:794–5. doi:10.1164/rccm.201302-0361ED.
- Findlow H, Borrow R. Interactions of conjugate vaccines and co-administered vaccines. Hum Vaccin Immunother. 2016;12:226–30. doi:10.1080/21645515.2015.1091908.
- Oliver MB, van der Linden MP, Kuntzel SA, Saad JS, Nahm MH. Discovery of Streptococcus pneumoniae serotype 6 variants with glycosyltransferases synthesizing two differing repeating units. J Biol Chem. 2015;290:26474–5. doi:10.1074/jbc.A113.480152.
- Chang B, Nariai A, Sekizuka T, Akeda Y, Kuroda M, Oishi K, Ohnishi M. Capsule switching and antimicrobial resistance acquired during repeated streptococcus pneumoniae Pneumonia episodes. J Clin Microbiol. 2015;53:3318–24. doi:10.1128/JCM.01222-15.
- Lachenauer CS, Kasper DL, Shimada J, Ichiman Y, Ohtsuka H, Kaku M, Paoletti LC, Ferrieri P, Madoff LC. Serotypes VI and VIII predominate among group B streptococci isolated from pregnant Japanese women. J Infect Dis. 1999;179:1030–3. doi:10.1086/314666.
- Persson E, Berg S, Trollfors B, Larsson P, Ek E, Backhaus E, Claesson BE, Jonsson L, Rådberg G, Ripa T, et al. Serotypes and clinical manifestations of invasive group B streptococcal infections in western Sweden 1998–2001. Clin Microbiol Infect. 2004;10:791–6. doi:10.1111/j.1469-0691.2004.00931.x.
- Yao K, Poulsen K, Maione D, Rinaudo CD, Baldassarri L, Telford JL, Sørensen UB; Members of the DEVANI Study Group, Kilian M. Capsular gene typing of Streptococcus agalactiae compared to serotyping by latex agglutination. J Clin Microbiol. 2013;51:503–7. doi:10.1128/JCM.02417-12.
- Ramaswamy SV, Ferrieri P, Flores AE, Paoletti LC. Molecular characterization of nontypeable group B streptococcus. J Clin Microbiol. 2006;44:2398–403. doi:10.1128/JCM.02236-05.
- Grandi G. Bacterial surface proteins and vaccines. F1000 Biol Rep. 2010;2:36.
- Rodriguez-Ortega MJ, Norais N, Bensi G, Liberatori S, Capo S, Mora M, Scarselli M, Doro F, Ferrari G, Garaguso I, et al. Characterization and identification of vaccine candidate proteins through analysis of the group A Streptococcus surface proteome. Nat Biotechnol. 2006;24:191–7. doi:10.1038/nbt1179.
- Raynes JM, Young PG, Proft T, Williamson DA, Baker EN, Moreland NJ. Protein adhesins as vaccine antigens for Group A Streptococcus. Pathog Dis. 2018;76:fly061. doi:10.1093/femspd/fty016.
- Navarre WW, Schneewind O. Surface proteins of gram-positive bacteria and mechanisms of their targeting to the cell wall envelope. Microbiol Mol Biol Rev. 1999;63:174–229.
- Hughes MJ, Moore JC, Lane JD, Wilson R, Pribul PK, Younes ZN, Dobson RJ, Everest P, Reason AJ, Redfern JM, et al. Identification of major outer surface proteins of Streptococcus agalactiae. Infect Immun. 2002;70:1254–9. doi:10.1128/IAI.70.3.1254-1259.2002.
- Lindahl G, Stalhammar-Carlemalm M, Areschoug T. Surface proteins of Streptococcus agalactiae and related proteins in other bacterial pathogens. Clin Microbiol Rev. 2005;18:102–27. doi:10.1128/CMR.18.1.102-127.2005.
- Liu H, Zhang S, Shen Z, Ren G, Liu L, Ma Y, Zhang Y, Wang W. Development of a vaccine against Streptococcus agalactiae in fish based on truncated cell wall surface anchor proteins. Vet Rec. 2016;179:359. doi:10.1136/vr.103692.
- Gabrielsen C, Maeland JA, Lyng RV, Radtke A, Afset JE. Molecular characteristics of Streptococcus agalactiae strains deficient in alpha-like protein encoding genes. J Med Microbiol. 2017;66:26–33. doi:10.1099/jmm.0.000412.
- Maeland JA, Afset JE, Lyng RV, Radtke A. Survey of immunological features of the alpha-like proteins of Streptococcus agalactiae. Clin Vaccine Immunol. 2015;22:153–9. doi:10.1128/CVI.00643-14.
- Baron MJ, Bolduc GR, Goldberg MB, Auperin TC, Madoff LC. Alpha C protein of group B Streptococcus binds host cell surface glycosaminoglycan and enters cells by an actin-dependent mechanism. J Biol Chem. 2004;279:24714–23. doi:10.1074/jbc.M402164200.
- Jerlstrom PG, Talay SR, Valentin-Weigand P, Timmis KN, Chhatwal GS. Identification of an immunoglobulin A binding motif located in the beta-antigen of the c protein complex of group B streptococci. Infect Immun. 1996;64:2787–93.
- Jerlstrom PG, Chhatwal GS, Timmis KN. The IgA-binding beta antigen of the c protein complex of Group B streptococci: sequence determination of its gene and detection of two binding regions. Mol Microbiol. 1991;5:843–9. doi:10.1111/j.1365-2958.1991.tb00757.x.
- Jarva H, Hellwage J, Jokiranta TS, Lehtinen MJ, Zipfel PF, Meri S. The group B streptococcal beta and pneumococcal Hic proteins are structurally related immune evasion molecules that bind the complement inhibitor factor H in an analogous fashion. J Immunol. 2004;172:3111–8. doi:10.4049/jimmunol.172.5.3111.
- Stalhammar-Carlemalm M, Stenberg L, Lindahl G. Protein rib: a novel group B streptococcal cell surface protein that confers protective immunity and is expressed by most strains causing invasive infections. J Exp Med. 1993; 177:1593–603. doi:10.1084/jem.177.6.1593.
- Gravekamp C, Kasper DL, Paoletti LC, Madoff LC. Alpha C protein as a carrier for type III capsular polysaccharide and as a protective protein in group B streptococcal vaccines. Infect Immun. 1999;67:2491–6.
- Michel JL, Madoff LC, Kling DE, Kasper DL, Ausubel FM. Cloned alpha and beta C-protein antigens of group B streptococci elicit protective immunity. Infect Immun. 1991;59:2023–8.
- Gravekamp C, Horensky DS, Michel JL, Madoff LC. Variation in repeat number within the alpha C protein of group B streptococci alters antigenicity and protective epitopes. Infect Immun. 1996;64:3576–83.
- Erdogan S, Fagan PK, Talay SR, Rohde M, Ferrieri P, Flores AE, Guzmán CA, Walker MJ, Chhatwal GS. Molecular analysis of group B protective surface protein, a new cell surface protective antigen of group B streptococci. Infect Immun. 2002;70:803–11. doi:10.1128/IAI.70.2.803-811.2002.
- Lin SM, Zhi Y, Ahn KB, Lim S, Seo HS. Status of group B streptococcal vaccine development. Clin Exp Vaccine Res. 2018;7:76–81. doi:10.7774/cevr.2018.7.1.76.
- Doro F, Liberatori S, Rodriguez-Ortega MJ, Rinaudo CD, Rosini R, Mora M, Scarselli M, Altindis E, D'Aurizio R, Stella M, et al. Surfome analysis as a fast track to vaccine discovery: identification of a novel protective antigen for group B streptococcus hypervirulent strain COH1. Mol Cell Proteomics. 2009;8:1728–37. doi:10.1074/mcp.M800486-MCP200.
- Takamatsu D, Bensing BA, Cheng H, Jarvis GA, Siboo IR, Lopez JA, Griffiss JM, Sullam PM. Binding of the Streptococcus gordonii surface glycoproteins GspB and Hsa to specific carbohydrate structures on platelet membrane glycoprotein Ibalpha. Mol Microbiol. 2005;58:380–92. doi:10.1111/j.1365-2958.2005.04830.x.
- Bensing BA, Lopez JA, Sullam PM. The Streptococcus gordonii surface proteins GspB and Hsa mediate binding to sialylated carbohydrate epitopes on the platelet membrane glycoprotein Ibalpha. Infect Immun. 2004;72:6528–37. doi:10.1128/IAI.72.11.6528-6537.2004.
- Sullam PM, Hyun WC, Szollosi J, Dong J, Foss WM, Lopez JA. Physical proximity and functional interplay of the glycoprotein Ib-IX-V complex and the Fc receptor FcgammaRIIA on the platelet plasma membrane. J Biol Chem. 1998;273:5331–6. doi:10.1074/jbc.273.9.5331.
- Bensing BA, Khedri Z, Deng L, Yu H, Prakobphol A, Fisher SJ, Chen X, Iverson TM, Varki A, Sullam PM. Novel aspects of sialoglycan recognition by the Siglec-like domains of streptococcal SRR glycoproteins. Glycobiology. 2016;26:1222–34.
- Seo HS, Minasov G, Seepersaud R, Doran KS, Dubrovska I, Shuvalova L, Anderson WF, Iverson TM, Sullam PM. Characterization of fibrinogen binding by glycoproteins Srr1 and Srr2 of Streptococcus agalactiae. J Biol Chem. 2013;288:35982–96. doi:10.1074/jbc.M113.513358.
- Seo HS, Xiong YQ, Sullam PM. Role of the serine-rich surface glycoprotein Srr1 of Streptococcus agalactiae in the pathogenesis of infective endocarditis. PloS One. 2013;8:e64204. doi:10.1371/journal.pone.0064204.
- Seo HS, Mu R, Kim BJ, Doran KS, Sullam PM. Binding of glycoprotein Srr1 of Streptococcus agalactiae to fibrinogen promotes attachment to brain endothelium and the development of meningitis. PLoS Pathog. 2012;8:e1002947. doi:10.1371/journal.ppat.1002947.
- Wang NY, Patras KA, Seo HS, Cavaco CK, Rosler B, Neely MN, Sullam PM, Doran KS. Group B streptococcal serine-rich repeat proteins promote interaction with fibrinogen and vaginal colonization. J Infect Dis. 2014;210:982–91. doi:10.1093/infdis/jiu151.
- Lin SM, Jang AY, Zhi Y, Gao S, Lim S, Lim JH, Song JY, Sullam PM, Rhee JH. Seo H. Vaccination With a Latch Peptide Provides Serotype-Independent Protection Against group B streptococcus Infection in Mice. J Infect Dis. 2017;217:93–102. doi:10.1093/infdis/jix565.
- Chmouryguina I, Suvorov A, Ferrieri P, Cleary PP. Conservation of the C5a peptidase genes in group A and B streptococci. Infect Immun. 1996;64:2387–90.
- Bohnsack JF, Takahashi S, Hammitt L, Miller DV, Aly AA, Adderson EE. Genetic polymorphisms of group B streptococcus scpB alter functional activity of a cell-associated peptidase that inactivates C5a. Infect Immun. 2000;68:5018–25. doi:10.1128/IAI.68.9.5018-5025.2000.
- Brown CK, Gu ZY, Matsuka YV, Purushothaman SS, Winter LA, Cleary PP, Olmsted SB, Ohlendorf DH, Earhart CA. Structure of the streptococcal cell wall C5a peptidase. Proc Natl Acad Sci U S A. 2005;102:18391–6. doi:10.1073/pnas.0504954102.
- Kagawa TF, O'Connell MR, Mouat P, Paoli M, O'Toole PW, Cooney JC. Model for substrate interactions in C5a peptidase from Streptococcus pyogenes: A 1.9 A crystal structure of the active form of ScpA. J Mol Biol. 2009;386:754–72. doi:10.1016/j.jmb.2008.12.074.
- Beckmann C, Waggoner JD, Harris TO, Tamura GS, Rubens CE. Identification of novel adhesins from Group B streptococci by use of phage display reveals that C5a peptidase mediates fibronectin binding. Infect Immun. 2002;70:2869–76. doi:10.1128/IAI.70.6.2869-2876.2002.
- Cheng Q, Debol S, Lam H, Eby R, Edwards L, Matsuka Y, Olmsted SB, Cleary PP. Immunization with C5a peptidase or peptidase-type III polysaccharide conjugate vaccines enhances clearance of group B Streptococci from lungs of infected mice. Infect Immun. 2002;70:6409–15. doi:10.1128/IAI.70.11.6409-6415.2002.
- Bohnsack JF, Widjaja K, Ghazizadeh S, Rubens CE, Hillyard DR, Parker CJ, Albertine KH, Hill HR. A role for C5 and C5a-ase in the acute neutrophil response to group B streptococcal infections. J Infect Dis. 1997;175:847–55. doi:10.1086/513981.
- Cheng Q, Carlson B, Pillai S, Eby R, Edwards L, Olmsted SB, Cleary P. Antibody against surface-bound C5a peptidase is opsonic and initiates macrophage killing of group B streptococci. Infect Immun. 2001;69:2302–8. doi:10.1128/IAI.69.4.2302-2308.2001.
- Cheng Q, Stafslien D, Purushothaman SS, Cleary P. The group B streptococcal C5a peptidase is both a specific protease and an invasin. Infect Immun. 2002;70:2408–13. doi:10.1128/IAI.70.5.2408-2413.2002.
- Santillan DA, Rai KK, Santillan MK, Krishnamachari Y, Salem AK, Hunter SK. Efficacy of polymeric encapsulated C5a peptidase-based group B streptococcus vaccines in a murine model. Am J Obstet Gynecol. 2011;205:249 e1-8. doi:10.1016/j.ajog.2011.06.024.
- Santillan DA, Andracki ME, Hunter SK. Protective immunization in mice against group B streptococci using encapsulated C5a peptidase. Am J Obstet Gynecol. 2008;198:114 e1-6. doi:10.1016/j.ajog.2007.06.003.
- Tettelin H, Masignani V, Cieslewicz MJ, Donati C, Medini D, Ward NL, Angiuoli SV, Crabtree J, Jones AL, Durkin AS, et al. Genome analysis of multiple pathogenic isolates of Streptococcus agalactiae: implications for the microbial “pan-genome”. Proc Natl Acad Sci U S A. 2005;102:13950–5. doi:10.1073/pnas.0506758102.
- Lauer P, Rinaudo CD, Soriani M, Margarit I, Maione D, Rosini R, Taddei AR, Mora M, Rappuoli R, Grandi G, et al. Genome analysis reveals pili in group B streptococcus. Science. 2005;309:105. doi:10.1126/science.1111563.
- Rosini R, Rinaudo CD, Soriani M, Lauer P, Mora M, Maione D, Taddei A, Santi I, Ghezzo C, Brettoni C, et al. Identification of novel genomic islands coding for antigenic pilus-like structures in Streptococcus agalactiae. Mol Microbiol. 2006;61:126–41. doi:10.1111/j.1365-2958.2006.05225.x.
- Margarit I, Rinaudo CD, Galeotti CL, Maione D, Ghezzo C, Buttazzoni E, Rosini R, Runci Y, Mora M, Buccato S, et al. Preventing bacterial infections with pilus-based vaccines: the group B streptococcus paradigm. J Infect Dis. 2009;199:108–15. doi:10.1086/595564.
- Martins ER, Andreu A, Melo-Cristino J, Ramirez M. Distribution of pilus islands in Streptococcus agalactiae that cause human infections: insights into evolution and implication for vaccine development. Clin Vaccine Immunol, 2013;20:313–6. doi:10.1128/CVI.00529-12.
- Vengadesan K, Ma X, Dwivedi P, Ton-That H, Narayana SV. A model for group B Streptococcus pilus type 1: the structure of a 35-kDa C-terminal fragment of the major pilin GBS80. J Mol Biol. 2011;407:731–43. doi:10.1016/j.jmb.2011.02.024.
- Khare B, Krishnan V, Rajashankar KR, H IH, Xin M, Ton-That H, Narayana SV. Structural differences between the Streptococcus agalactiae housekeeping and pilus-specific sortases: SrtA and SrtC1. PloS One. 2011;6:e22995. doi:10.1371/journal.pone.0022995.
- Krishnan V, Dwivedi P, Kim BJ, Samal A, Macon K, Ma X, Mishra A, Doran KS, Ton-That H, Narayana SV. Structure of Streptococcus agalactiae tip pilin GBS104: a model for GBS pili assembly and host interactions. Acta Crystallogr D Biol Crystallogr. 2013;69:1073–89. doi:10.1107/S0907444913004642.
- Banerjee A, Kim BJ, Carmona EM, Cutting AS, Gurney MA, Carlos C, Feuer R, Prasadarao NV, Doran KS. Bacterial Pili exploit integrin machinery to promote immune activation and efficient blood-brain barrier penetration. Nat Commun. 2011;2:462. doi:10.1038/ncomms1474.
- Sheen TR, Jimenez A, Wang NY, Banerjee A, van Sorge NM, Doran KS. Serine-rich repeat proteins and pili promote Streptococcus agalactiae colonization of the vaginal tract. J Bacteriol. 2011;193:6834–42. doi:10.1128/JB.00094-11.
- Konto-Ghiorghi Y, Mairey E, Mallet A, Dumenil G, Caliot E, Trieu-Cuot P, Dramsi S. Dual role for pilus in adherence to epithelial cells and biofilm formation in Streptococcus agalactiae. PLoS Pathog. 2009;5:e1000422. doi:10.1371/journal.ppat.1000422.
- Nuccitelli A, Cozzi R, Gourlay LJ, Donnarumma D, Necchi F, Norais N, Telford JL, Rappuoli R, Bolognesi M, Maione D, et al. Structure-based approach to rationally design a chimeric protein for an effective vaccine against group B streptococcus infections. Proc Natl Acad Sci U S A. 2011;108:10278–83. doi:10.1073/pnas.1106590108.
- Lin FY, Philips JB, 3rd, Azimi PH, Weisman LE, Clark P, Rhoads GG, Regan J, Concepcion NF, Frasch CE, Troendle J, et al. Level of maternal antibody required to protect neonates against early-onset disease caused by group B Streptococcus type Ia: a multicenter, seroepidemiology study. J Infect Dis. 2001;184:1022–8. doi:10.1086/323350.
- Lin FY, Weisman LE, Azimi PH, Philips JB, 3rd, Clark P, Regan J, Rhoads GG, Frasch CE, Gray BM, Troendle J, et al. Level of maternal IgG anti-group B streptococcus type III antibody correlated with protection of neonates against early-onset disease caused by this pathogen. J Infect Dis. 2004;190:928–34. doi:10.1086/422756.
- Balmer P, Borrow R, Miller E. Impact of meningococcal C conjugate vaccine in the UK. J Med Microbiol 2002; 51:717–22. doi:10.1099/0022-1317-51-9-717.
- Romero-Steiner S, Frasch CE, Carlone G, Fleck RA, Goldblatt D, Nahm MH. Use of opsonophagocytosis for the serological evaluation of pneumococcal vaccines. Clin Vaccine Immunol. 2006;13:165–9. doi:10.1128/CVI.13.2.165-169.2006.
- Johnson SE, Rubin L, Romero-Steiner S, Dykes JK, Pais LB, Rizvi A, Ades E, Carlone GM. Correlation of opsonophagocytosis and passive protection assays using human anticapsular antibodies in an infant mouse model of bacteremia for Streptococcus pneumoniae. J Infect Dis. 1999;180:133–40. doi:10.1086/314845.
- Barrette RW, Urbonas J, Silbart LK. Quantifying specific antibody concentrations by enzyme-linked immunosorbent assay using slope correction. Clin Vaccine Immunol. 2006;13:802–5. doi:10.1128/CVI.00422-05.
- Granoff DM, Maslanka SE, Carlone GM, Plikaytis BD, Santos GF, Mokatrin A, Raff HV. A modified enzyme-linked immunosorbent assay for measurement of antibody responses to meningococcal C polysaccharide that correlate with bactericidal responses. Clin Diagn Lab Immunol. 1998;5:479–85.
- Guttormsen H-K, Wetzler LM, Finberg RW, Kasper DL. Immunologic memory induced by a glycoconjugate vaccine in a murine adoptic transfer model. InfecImmun. 1998;66:2026–32.
- Guttormsen HK, Baker CJ, Edwards MS, Paoletti LC, Kasper DL. Quantitative determination of antibodies to type III group B streptococcal polysaccharide. J Infect Dis. 1996;173:142–50. doi:10.1093/infdis/173.1.142.
- Bhushan R, Anthony BF, Frasch CE. Estimation of group B streptococcus type III polysaccharide-specific antibody concentrations in human sera is antigen dependent. InfectImmun. 1998;66:5848–53.
- Lee H, Nahm MH, Burton R, Kim KH. Immune response in infants to the heptavalent pneumococcal conjugate vaccine against vaccine-related serotypes 6A and 19A. Clin Vaccine Immunol. 2009;16:376–81. doi:10.1128/CVI.00344-08.
- Coughlin RT, White AC, Anderson CA, Carlone GM, Klein DL, Treanor J. Characterization of pneumococcal specific antibodies in healthy unvaccinated adults. Vaccine. 1998;16:1761–7. doi:10.1016/S0264-410X(98)00139-X.
- Yu X, Gray B, Chang SJ, Ward JI, Edwards KM, Nahm MH. Immunity to cross-reactive serotypes induced by pneumococcal conjugate vaccines in infants. J Infect Dis. 1999;180:1569–76. doi:10.1086/315096.
- Yu X, Sun Y, Frasch CE, Concepcion N, Nahm MH. Pneumococcal capsular polysaccharide preparations may contain non-C-polysaccharide contaminants that are immunogenic. Clin Diagn Lab Immunol. 1999;6:519–24.
- Nahm MH, Olander JV, Magyarlaki M. Identification of cross-reactive antibodies with low opsonophagocytic activity for Streptococcus pneumoniae. J Infect Dis. 1997;176:698–703. doi:10.1086/514093.
- Nahm MH, Ward J, Chang S, Yu XH. Young children may produce antibodies against Streptococcus pneumoniae that are reactive but not opsonophagocytic to cross-reactive serotypes. ICAAC 1997:207(G-85).
- Fleck RA, Romero-Steiner S, Nahm MH. Use of HL-60 cell line to measure opsonic capacity of pneumococcal antibodies. A review. Clin Diagn Lab Immunol. 2005;12:19–27.
- Nahm MH, Briles DE, Yu X. Development of a multi-specificity opsonophagocytic killing assay. Vaccine. 2000;18:2768–71. doi:10.1016/S0264-410X(00)00044-X.
- Kim KH, Yu J, Nahm MH. Efficiency of a pneumococcal opsonophagocytic killing assay improved by multiplexing and by coloring colonies. Clin Diagn Lab Immunol. 2003;10:616–21.
- Romero-Steiner S, Frasch C, Concepcion N, Goldblatt D, Kayhty H, Vakevainen M, Laferriere C, Wauters D, Nahm MH, Schinsky MF, et al. Multilaboratory evaluation of a viability assay for measurement of opsonophagocytic antibodies specific to the capsular polysaccharides of Streptococcus pneumoniae. Clin Diagn Lab Immunol. 2003;10:1019–24.
- Choi MJ, Noh JY, Cheong HJ, Kim WJ, Lin SM, Zhi Y, et al. Development of a multiplexed opsonophagocytic killing assay (MOPA) for group B Streptococcus. Hum Vaccin Immunother. 2018;14:67–73. doi:10.1080/21645515.2017.1377379.