ABSTRACT
The development of viral vector vaccines against various pathogens for which conventional vaccination approaches are not applicable has been a priority for a number of years. One promising approach is the insertion of immunodominant conservative cytotoxic T-cell (CTL) epitopes into the genome of a viral vector, which then delivers these epitopes to target cells, inducing immunity. Many different viruses have been assessed as viral vectors for CTL-based vaccines, but only a few of them are clinically relevant, mainly because of safety issues and limited knowledge about their performance in humans. In this regard, the use of licensed cold-adapted live attenuated influenza vaccine (LAIV) viruses as a vector delivery system has clear advantages for CTL-based vector vaccines against other respiratory pathogens: LAIV is known to induce all arms of the adaptive immune system and is administered via nasal spray, and its production process is relatively easy and inexpensive. Here we present the first results of the use of an LAIV backbone for designing a CTL epitope-based vaccine against respiratory syncytial virus (RSV). The chimeric LAIV-RSV vaccine candidates were attenuated in mice and induced strong, fully functional CTL immunity in this animal model.
Introduction
One of the major achievements in modern vaccinology is the improvement in structural approaches to the targeting of specific antigens of various pathogens. An epitope-based vaccination approach seeks to stimulate immunity to one or several epitopes of the pathogen of interest. This approach most commonly targets linear epitopes and can be used for immunotherapeutic and immunoprophylactic purposes.Citation1–Citation3 Numerous gene delivery systems exist serving different purposes.Citation4 Both non-viral and viral delivery systems can be used for epitope-based vaccination. The non-viral vector systems use artificial vehicles for DNA or RNA injection and are administered directly to the organism (DNA vaccine principles). The viral vectors are natural or engineered live viruses that carry foreign genetic material to target cells. The type of delivery system can be characterized in terms of target cell type, localization, duration of immune responses and co-stimulatory effects. There are numerous live attenuated vaccine viruses derived from human strains that preserve a host tissue tropism and a replication machinery adapted to human cells.Citation5
For respiratory infections that cannot be prevented by conventional vaccination approaches, a live attenuated influenza vaccine (LAIV) virus seems to be an attractive delivery system for the immunogenic epitopes of the pathogen.Citation6 LAIVs induce cross-reactive influenza immunity.Citation7 Intranasal administration of LAIV mimics upper respiratory infection with influenza virus, without clinical symptoms of disease.Citation8 The infection activates both systemic and local immune responses, such as viral interference,Citation9 natural killer cell activation,Citation10 cross-reactive T-cell expansionCitation11 and secretory IgA antibody generation.Citation12 There are well developed reverse genetic techniques for constructing an influenza virus vector.Citation13,Citation14 Here we report a proof-of-concept design of an LAIV vector-based T-cell epitope vaccine against respiratory syncytial virus (RSV), which is the most common cause of acute respiratory tract infections in children and the elderly.Citation15 Although there are currently no licensed RSV products on the market, significant advances in RSV vaccine development have been achieved during the past decade.Citation16 However, data on the design of CD8+ cytotoxic T-cell (CTL) epitope-based RSV vaccines are still limited. Study of prototype LAIV-RSV chimeric vaccines containing H2-Kd-specific CTL epitopes in a murine model will provide an immunological basis for further development of human T-cell epitope-based vaccines on the LAIV viral vector.
Results and discussion
During the past 20 years, a number of successful recombinant vaccines based on an influenza virus vector have been generated and studied in animal models.Citation13 Despite the wide use of influenza viruses as vectors for vaccines against a variety of infectious agents and some types of cancer, the issue of the safety of such vaccines in mass vaccination of the population remains unresolved. The majority of studies have concentrated on either A/WSN/33 or A/PR/8/34 virus as backbone; however, their unknown passage histories make it unlikely that the chimeric vaccines based on these backbones will be widely used as live virus vector vaccines. It should be noted that the majority of the reported studies have assessed the performance of recombinant viruses expressing full proteins of foreign pathogens, which is relevant for the establishment of an antibody-based immune response. Data on the development of T-cell epitope-based vaccines against respiratory pathogens are limited. The insertion of T-cell epitopes rather than full-length genes makes it possible to construct recombinant vaccines against multiple viruses. In this study, a licensed cold-adapted (ca) LAIV backbone was used to generate the first CTL-based LAIV viral vector vaccine against RSV.
We selected two RSV M2-1 protein fragments which were enriched by H2-(d) T-cell epitopes: M2-1 protein 70–101 fragment (32 amino acids) and M2-1 protein 114–146 fragment (33 amino acids) (). Both fragments were combined into a cassette with the N-terminus leading sequence of P2A self-cleaving peptide (). The construct was inserted into the C-terminus of influenza H7N9 neuraminidase (NA) protein, so that the full length of the NA protein was translated and incorporated into the viral particle, whereas the cleaved RSV cassette remained inside an infected cell (). Another recombinant LAIV-RSV candidate was designed to produce a C-terminus truncated NS1 protein, which retained the N-terminus 126 amino acids, followed by the P2A self-cleavage site and the RSV cassette (). This C-terminus truncation was selected on the basis of the results of previous studies that had successfully generated attenuated and immunogenic influenza viruses.Citation17,Citation18 Cleavage of the RSV cassette upon translation in infected cells should facilitate the T-cell epitope-presenting pathway.
Figure 1. The LAIV-RSV constructs.
(a). Distribution of major histocompatibility complex (MHC) class I (green) and class II (blue) restricted epitopes over M2-1 protein. The epitopes dataset was queried from the Immune Epitope DataBase in April 2017 (host organism: BALB/c mouse; antigen: matrix M2-1; positive assays only). The yellow lengths denote the RSV cassette fragments. (b). Scheme for generating chimeric influenza NA gene carrying RSV cassette. (c). Scheme for generating chimeric influenza NS1 gene carrying RSV cassette. (d). Genome composition of LAIV viruses.P2A: porcine teschovirus-1 P2A self-cleavage site (GSGATNFSLLKQAGDVEENPG↓P).
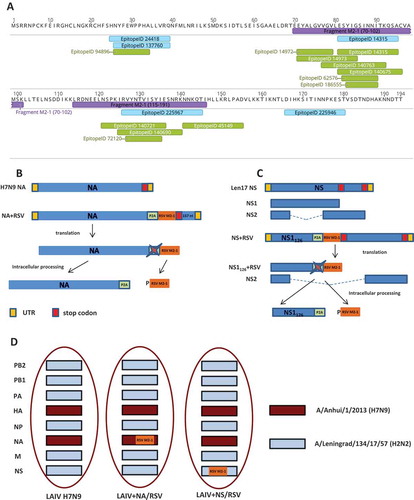
Two recombinant LAIV-RSV viruses based on H7N9 LAIV reassortant virus were rescued in this study. The H7N9 LAIV vector contained six genes coding for internal and non-structural proteins of Leningrad/17 master donor virus and hemagglutinin (HA) and NA genes of Anhui/2013 strain (). The first recombinant vaccine, LAIV+ NA/RSV, was generated using chimeric NA-RSV plasmid and the remaining plasmids identical to H7N9 LAIV. Similarly, the second recombinant virus, LAIV+ NS/RSV, was rescued from chimeric NS-RSV plasmid and the seven remaining plasmids of H7N9 LAIV. Importantly, the insertion of foreign epitopes into NA and NS1 genes did not affect the reproductive capacity of the vaccine virus in eggs at optimal temperature, suggesting that they could be used for egg-based vaccine production (). Both recombinant LAIV-RSV vaccines maintained the ts (temperature-sensitive) phenotype. However, in both cases, the insertion of the RSV cassette significantly reduced virus replication at low temperature in eggs, indicating that expression of modified viral genes might affect the ca phenotype (). The loss of the ca phenotype does not affect the safety of LAIV, since the attenuation of LAIV viruses is linked to the ts phenotype only.Citation19,Citation20 All three strains were unable to replicate in mouse lungs, confirming the attenuated phenotype of the viruses (). Surprisingly, the recombinant LAIV-RSV virus with the insertion of RSV cassette in the NS1 gene was not detected in the mouse upper respiratory tract, whereas the titer for the recombinant vaccine with the insertion in the NA gene was comparable to that for the control LAIV vector virus (). Previous studies have indicated that truncation of the NS1 protein to 126 amino acids reduces virus titer in mouse tissues by 100-fold;Citation21,Citation22 this may be the reason for these differences in virus infectivity in mice.
Table 1. Virological characteristics of recombinant LAIV-RSV viruses in vitro and in vivo.
Despite the reduced replication of the LAIV+ NS/RSV construct, this virus induced significant levels of RSV M2-182–90 epitope-specific CD8+ T-cells after immunization. Levels were identical to those induced by LAIV+ NA/RSV, suggesting that active replication of vaccine virus in mice was not required to stimulate CTLs against RSV-specific M2-182–90 epitope (). At the same time, both recombinant viruses stimulated an LAIV-specific response similar to that of the vector LAIV, which suggests that the insertion of foreign epitopes did not have a negative effect on anti-influenza CTL immunity (). On the other hand, no increase in the levels of CTLs reacting with M2-1126–145 peptide was detected after vaccination with LAIV-RSV constructs (). These data suggest that no CTLs specific to M2-1127–135 CD8+ epitope located inside the M2-1126–145 peptide were induced. The specificity of the T-cell response was confirmed by dimethyl sulfoxide (DMSO) control stimulation data ().
Figure 2. CD8+ T-cell response in mice after LAIV immunization measured by IFN-gamma ICS assay.
(a). Stimulation of cells by M2-182–90 peptide. (b). Stimulation with M2-1126–145 peptide. (c). Stimulation with LAIV vector whole virus. (d). Stimulation with DMSO. Bars denote median and interquartile range. P values for Mann-Whitney U test are indicated. ns: not significant (P ≥ 0.05). Red asterisks denote significant differences in levels of IFN-gamma+ CD8+ T cell between PBS and LAIV vaccine groups.
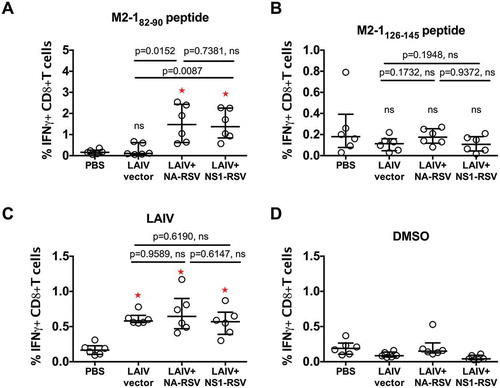
We further estimated the cytotoxic activity of RSV-specific CTLs induced by vaccination with LAIV-RSV constructs using a CTL in vivo assay. This method allows the functional activity of CTLs to be measured, i.e. their killing of target cells in the living organism. Immunized mice were treated with syngeneic peptide-loaded donor splenocytes and the number of recovered donor cells in the different immunization groups was assessed. In this assay, strong CTL activity was detected in vivo against M2-182–90-loaded target cells, in both LAIV+ NA/RSV and LAIV+ NS/RSV immunized animals, compared with LAIV vector-vaccinated mice (). As expected, there was little CTL killing activity against M2-1126–145-loaded target cells in both LAIV-RSV immunization groups (). Overall, the two locations of the RSV CTL cassette in the genome of LAIV virus induced similar CTL immune responses against influenza virus and foreign insert.
Figure 3. Comparison of in vivo CTL activity against RSV peptide-loaded targets in immunized mice.
(a). M2-182–90-peptide. (b). M2-1126–145 peptide.Bars denote median and interquartile range. Multiplicity adjusted P values are indicated. ns: not significant (P ≥ 0.05).
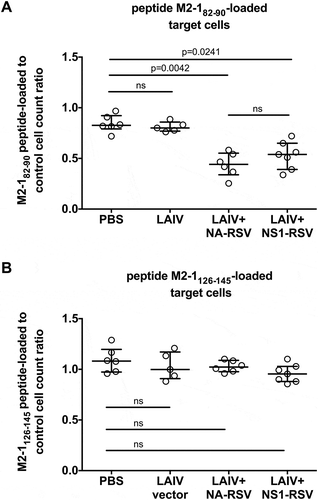
Many factors could influence the skewed CTL response to the M2-182–90 epitope. The first relates to the peptide processing steps: all RSV M2-1 fragments were combined into one cassette and synthesized evenly in an infected cell. To maximize the chance of correct epitope cleavage, we extended fragment lengths over the epitope sequence up to 4–6 residues. Thus, there is a low probability that M2-182–90 antigenic peptide has advantages in biosynthesis. Another factor is antigen presentation and the efficiency of epitope-specific T-cell expansion. M2-182–90 is a strongly immunodominant and protective epitope for BALB/c mice,Citation23 whereas M2-1127–135 epitope is subdominant and only about 0.3% of M2-1127–135-tetramer positive CD8+ T cells can be detected in murine spleens in the acute phase of RSV infection.Citation24 This is about one-third of the M2-182–90-specific T-cells found. McDermott and co-authors have shown strong immunodominance of M2-182–90 over M2-1127–135.Citation25 Interestingly, mutation of the M2-182–90 epitope did not elicit compensation by M2127-135-specific T-cell expansion, rather than another DbM187-195 epitope.Citation26 Thus, in LAIV infection there was no M2-1126–145 epitope-specific T-cell response, while M2-182–90-epitope-specifc T-cell expansion was pronounced. It is important to note that changes in RSV T-cell epitope composition can provoke pathological lesions during infection.Citation26 This was associated with M2-182–90 immunodominant epitope T-cell overcompensation when subdominant DbM187-195 epitope had been excluded. Thus, a combination of dominant and subdominant epitopes might be preferable for CTL activity “shaping”. Further studies are needed to investigate the balance of CTL activity in RSV vaccine development.
Conclusion
Our results show that safe and immunogenic cold-adapted influenza viruses that have been successfully used as live attenuated influenza vaccine for more than 30 years could be a promising platform for the design of T-cell epitope-based virus vector vaccines. As a proof-of-concept, we integrated a 139 amino acid RSV cassette into the NA and NS1 segments of LAIV virus using the 2A self-cleavage site and demonstrated that such constructs can stimulate fully functional RSV epitope-specific T-cell immunity in a murine model. Further studies of these constructs are now needed in a mouse RSV challenge model. Since foreign CTL-based inserts are relatively small, it is possible to incorporate epitopes from different pathogens on the same backbone, to create a multi-strain vaccine against influenza and other respiratory viruses.
Materials and methods
Cells, plasmids, peptides
Madin-Darby canine kidney (MDCK) and African monkey kidney cell lines (Vero) were obtained from the American Type Culture Collection and maintained in Dulbecco modified Eagle medium (DMEM) supplemented with 10% fetal bovine serum (FBS) (Hyclone) and serum-free OptiPro (Gibco) medium, respectively.
The RSV cassette containing human RSV A2 strain fragment from M2-1 protein (amino acids 70–101 and 114–146) was chemically synthesized by Eurogen Ltd (Russian Federation) (). This cassette also contained a porcine teschovirus-1 P2A auto cleavage site at the N-terminusCitation27 and a stop-codon at the C-terminus. A plasmid-based reverse genetics system developed for A/Leningrad/134/17/57 cold-adapted LAIV backbone was used to generate recombinant influenza viruses.Citation14 In addition, the HA and NA genes of A/Anhui/1/2013 (H7N9) virus were cloned into dual-promoter reverse genetics plasmids.
Human RSV strain A2 matrix protein peptides M2-182–90 (SYIGSINNI) and M2-1126–145 (RVYNTVISYIESNRKNNKQT) were chemically synthesized by Almabion Ltd (Russian Federation) with a purity of more than 80%, as measured by high-performance liquid chromatography. Peptides were reconstituted in DMSO at a concentration of 1 mM and stored at – 70°C in single-use aliquots.
Generation of recombinant LAIV-RSV viruses
The RSV cassette containing a porcine teschovirus-1 P2A self-cleavage site was inserted into either the NA gene of H7N9 virus or the NS1 gene of Len/17 virus (). For the NA-based construct, a 157-nucleotide 3’-end fragment was first duplicated to ensure correct incorporation of viral RNA into virions,Citation28 then the RSV cassette was inserted at the C-terminus of the NA protein (). For the NS1-based construct, the RSV cassette was inserted after amino acid 126 of NS1 protein, resulting in truncation of Len/17 NS1 protein. The NS2 protein remained unchanged. In both constructs, the self-cleavage P2A site separated the foreign sequence from the viral open reading frame, thus facilitating localization of the T-cell foreign epitopes inside the cells and activation of T-cell immunity. Recombinant influenza viruses, and the control LAIV reassortant strain, were rescued in Vero cells using a Neon electroporation system (Invitrogen). All viruses were fully sequenced using ABI BigDye Terminator v3.1 Cycle Sequencing kits and capillary-based 3130xl Series Genetic Analyzer (Applied Biosystems). Viruses were grown in 10-day-old embryonated chicken eggs at 33°C. Allantoic fluid was harvested, clarified by centrifugation and stored at – 70°C for further analyses.
In vitro studies
The infectious activity of the recombinant LAIV-RSV viruses was compared with that of the LAIV vector control virus using embryonated chicken eggs incubated at different temperatures (33°C, 38°C and 26°C) and MDCK cells at permissive temperature (33°C). Virus titers in eggs and MDCK cells were calculated using the Reed and Muench method and expressed in terms of log10 50% infectious dose (EID50)/ml and log10TCID50/ml, respectively. A virus was considered to be temperature-sensitive (ts phenotype) if the infectious titer at 33°C was at least 5.0 log10EID50 higher than at 38°C. A virus was considered to be cold-adapted (ca phenotype) if the infectious titer at 26°C was not more than 3.0 log10EID50 lower than at 33°C.
Murine studies
Animal studies were performed in accordance with the European Convention for the Protection of Vertebrate Animals used for Experimental and other Scientific Purposes (Strasbourg, 18.3.1986) and with the approval of the Institute of Experimental Medicine Ethics Committee (protocol #2/17 approved 15.02.2017). Female BALB/c mice of 6–8 weeks old were obtained from the Stolbovaya breeding laboratory and nursery of the Scientific Center for Biomedical Technologies of the Federal Medical and Biological Agency (Moscow region, Russian Federation).
Groups of mice were immunized intranasally with LAIV strains at a dose of 106 log10EID50, in a volume of 50 μl, twice at 21 days interval. Replication of vaccine viruses in the respiratory tract of the mice (nasal turbinates, lungs) was assessed in four animals on day 3 after the first immunization by determining viral titers in homogenized tissues using limiting dilutions in eggs. Mice in the placebo group received phosphate-buffered saline (PBS) in same regimen.
Virus/epitope-specific T-cells were counted by intracellular cytokine staining (ICS) assay and quantified as the percentage of gamma-interferon (IFN) positive cells in the CD8 + T-cell subset. The mice were euthanized on day 21 after the second vaccine dose. Splenocytes were isolated in Hanks’ Balanced Salt Solution (Biolot) supplemented with 2% heat-inactivated FBS (Gibco) and 25 mM HEPES, and red blood cells were lysed with ammonium-chloride-potassium lysing buffer. One million cells were plated in complete DMEM (high glucose, Gibco) supplemented with antibiotic-antimycotic solution (Gibco), 50µM 2-mercaptoethanol (Amresco), 25mM HEPES (Biolot), and 10 U/ml human rIL-2 (Roncoleukin) in U-bottom well microplates. Cells were stimulated with sucrose-gradient purified virus at multiplicity of infection 3.0 in 100 µL of complete DMEM without FBS. For peptide stimulation, the same procedures were performed without the addition of virus. After incubation for one hour, 50 µL of complete DMEM with 30% FBS was added to a final FBS concentration of 10%. After 16–18 hours, GolgiPlug™ solution (BD) alone or with 1 μM peptide or DMSO (for peptide stimulation experiments) was added, followed by a further five hours incubation. ICS was performed using a Cytofix/Cytoperm kit (BD), in accordance with the manufacturer’s protocol. Samples were stained with live/dead fixable stain (Invitrogen), anti-CD4 (Biolegend), anti-CD8 (Biolegend) and anti-IFN-gamma antibody-conjugates (BD). Samples were fixed in 1% paraformaldehyde and analysed by Navios flow cytometer (Beckman Coulter).
An in vivo cytotoxicity assay was performed as described by Durward et al.Citation29 with modifications. Briefly, target cells were prepared from splenocytes of naïve BALB/c mice (1x108 splenocytes in 10 ml complete DMEM supplemented with 10% FBS) and loaded with either 1 μM peptide (M2-182–90 or M2-1126–145) or an equal volume of peptide diluent (DMSO) for one hour at 37 ℃, with occasional mixing. Next, cells were washed in PBS and stained with different concentrations of carboxyfluorescein succinimidyl ester CFSE in PBS (40, 20, 10 or 5 mM CFSE for control and 2 or 3 peptides, respectively). Cells were then washed, resuspended in Hanks solution and mixed in equal amounts to a final concentration of 1 × 108 cells/ml. Ten million prefiltered target cells were administered in 100 µL to anaesthetized mice by retro-orbital injection. After 16–18 hours, the mice were sacrificed; splenocytes were harvested and assessed by flow cytometry (SupFigure.pdf). The ratio of peptide-loaded to control target cells was calculated as a normalized measure of epitope-specific cytotoxicity between immunization groups. The ratio in placebo group samples (PBS) was assumed as survival at basal epitope-specific cytotoxicity.
Statistical analyses
Data were analysed with the GraphPad Prism 6.0 software (GraphPad Software Inc). Statistical significance of was determined by nonparametric Mann-Whitney U test and Dunn’s multiple comparison test. Multiplicity adjusted P values were reported and P < 0.05 was considered significant.
Disclosure of potential conflicts of interest
No potential conflict of interest was reported by the authors.
Supplemental Material
Download MS Power Point (6.7 MB)Supplementary material
Supplemental data can be accessed here.
Additional information
Funding
References
- Balazs, AB, Chen J, Hong CM, Rao DS, Yang L, Baltimore D. Antibody-based protection against HIV infection by vectored immunoprophylaxis. Nature. 2011;481(7379):81–84. doi:10.1038/nature10660.
- Xue, C., Tian X, Li X, Zhou Z, Su X, Zhou R. Construction and characterization of a recombinant human adenovirus type 3 vector containing two foreign neutralizing epitopes in hexon. Virus Res. 2014;183:67–74. doi:10.1016/j.virusres.2014.01.027.
- Gu, L., Li ZC, Krendelchtchikov A, Krendelchtchikova V, Wu H, Matthews QL. Using multivalent adenoviral vectors for HIV vaccination. PLoS One. 2013;8(3):e60347. doi:10.1371/journal.pone.0060347.
- Nayerossadat N, Maedeh T, Ali PA. Viral and nonviral delivery systems for gene delivery. Adv Biomed Res. 2012;1:27. doi:10.4103/2277-9175.98152.
- Minor PD. Live attenuated vaccines: historical successes and current challenges. Virology. 2015;479-480:379–392. doi:10.1016/j.virol.2015.03.032.
- Isakova-Sivak I, Tretiak T, Rudenko L. Cold-adapted influenza viruses as a promising platform for viral-vector vaccines. Expert Rev Vaccines. 2016; 15(10): 1241–1243.
- Hoft, DF, Lottenbach KR, Blazevic A, Turan A, Blevins TP, Pacatte TP, Yu Y, Mitchell MC, Hoft SG, Belshe RB. Comparisons of the humoral and cellular immune responses induced by live attenuated influenza vaccine and inactivated influenza vaccine in adults. Clin Vaccine Immunol. 2017;24(1). doi:10.1128/CVI.00414-16.
- Jin H, Subbarao K. Live attenuated influenza vaccine. Curr Top Microbiol Immunol. 2015;386:181–204. doi:10.1007/82_2014_410.
- Laurie, KL, Guarnaccia TA, Carolan LA, Yan AWC, Aban M, Petrie S, Cao P, Heffernan JM, McVernon J, Mosse J, et al. Interval between infections and viral hierarchy are determinants of viral interference following influenza virus infection in a Ferret model. J Infect Dis. 2015;212(11):1701–1710. doi:10.1093/infdis/jiv260.
- Schultz-Cherry S. Role of NK cells in influenza infection. Curr Top Microbiol Immunol. 2015;386:109–120. doi:10.1007/82_2014_403.
- Mohn KGI, Zhou F, Brokstad KA, Sridhar S, Cox RJ. Boosting of cross-reactive and protection-associated T cells in children after live attenuated influenza vaccination. J Infect Dis. 2017;215(10):1527–1535. doi:10.1128/CVI.00037-16.
- van Riet E, Ainai A, Suzuki T, Hasegawa H. Mucosal IgA responses in influenza virus infections; thoughts for vaccine design. Vaccine. 2012;30(40):5893–5900. doi:10.1016/j.vaccine.2012.04.109.
- Li J, Arevalo MT, Zeng M. Engineering influenza viral vectors. Bioengineered. 2013;4(1):9–14. doi:10.4161/bioe.21950.
- Isakova-Sivak I, Chen LM, Matsuoka Y, Voeten JT, Kiseleva I, Heldens JG, den Bosch H, Klimov A, Rudenko L, Cox N.J, et al. Genetic bases of the temperature-sensitive phenotype of a master donor virus used in live attenuated influenza vaccines: a/Leningrad/134/17/57 (H2N2). Virology. 2011;412(2):297–305. doi:10.1016/j.virol.2011.01.004.
- Griffiths C, Drews SJ, Marchant DJ. Respiratory syncytial virus: infection, detection, and new options for prevention and treatment. Clin Microbiol Rev. 2017;30(1):277–319. doi:10.1128/CMR.00010-16.
- Neuzil KM. Progress toward a respiratory syncytial virus vaccine. Clin Vaccine Immunol. 2016;23(3):186–188. doi:10.1128/CVI.00037-16.
- Talon J, Salvatore M, O'Neill RE, Nakaya Y, Zheng H, Muster T, Garcia-Sastre A, Palese P. Influenza A and B viruses expressing altered NS1 proteins: a vaccine approach. Proc Natl Acad Sci U S A. 2000;97(8):4309–4314. doi:10.1073/pnas.070525997.
- Steel J, Lowen AC, Pena L, Angel M, Solorzano A, Albrecht R, Perez DR, Garcia-Sastre A, Palese P. Live attenuated influenza viruses containing NS1 truncations as vaccine candidates against H5N1 highly pathogenic avian influenza. J Virol. 2009;83(4):1742–1753. doi:10.1128/JVI.01920-08.
- Maassab HF, Kendal AP, Abrams GD, Monto AS. Evaluation of a cold-recombinant influenza virus vaccine in ferrets. J Infect Dis. 1982;146(6):780–790.
- Wareing MD, Watson JM, Brooks MJ, Tannock GA. Immunogenic and isotype-specific responses to Russian and US cold-adapted influenza a vaccine donor strains A/Leningrad/134/17/57, A/Leningrad/134/47/57, and A/Ann Arbor/6/60 (H2N2) in mice. J Med Virol. 2001;65(1):171–177.
- Zhou B, Li Y, Belser JA, Pearce MB, Schmolke M, Subba AX, Shi Z, Zaki SR, Blau DM, Garcia-Sastre A, et al. NS-based live attenuated H1N1 pandemic vaccines protect mice and ferrets. Vaccine. 2010;28(50):8015–8025. doi:10.1016/j.vaccine.2010.08.106.
- Ferko B, Stasakova J, Sereinig S, Romanova J, Katinger D, Niebler B, Katinger H, Egorov A. Hyperattenuated recombinant influenza A virus nonstructural-protein-encoding vectors induce human immunodeficiency virus type 1 Nef-specific systemic and mucosal immune responses in mice. J Virol. 2001;75(19):8899–8908. doi:10.1128/JVI.75.19.8899-8908.2001.
- Kulkarni AB, Collins PL, Bacik I, Yewdell JW, Bennink JR, Crowe JE, Jr. Cytotoxic T cells specific for a single peptide on the M2 protein of respiratory syncytial virus are the sole mediators of resistance induced by immunization with M2 encoded by a recombinant vaccinia virus. J Virol. 1995;69(2):1261–1264.
- Lee S, Miller SA, Wright DW, Rock MT, Crowe JE. Tissue-specific regulation of CD8+ T-lymphocyte immunodominance in respiratory syncytial virus infection. J Virol. 2007;81(5):2349–2358. doi:10.1128/JVI.01910-06.
- McDermott DS, Knudson CJ, Varga SM. Determining the breadth of the respiratory syncytial virus-specific T cell response. J Virol. 2014;88(6):3135–3143. doi:10.1128/JVI.02139-13.
- Ruckwardt TJ, Luongo C, Malloy AM, Liu J, Chen M, Collins PL, Graham BS. Responses against a subdominant CD8+ T cell epitope protect against immunopathology caused by a dominant epitope. J Immunol. 2010;185(8):4673–4680. doi:10.4049/jimmunol.1001606.
- Kim, JH, Lee SR, Li LH, Park HJ, Park JH, Lee KY, Kim MK, Shin BA, Choi SY. High cleavage efficiency of a 2A peptide derived from porcine teschovirus-1 in human cell lines, zebrafish and mice. PLoS One. 2011;6(4):e18556. doi:10.1371/journal.pone.0018556.
- Fujii Y, Goto H, Watanabe T, Yoshida T, Kawaoka Y. Selective incorporation of influenza virus RNA segments into virions. Proc Natl Acad Sci U S A. 2003;100(4):2002–2007. doi:10.1073/pnas.0437772100.
- Durward M, Harms J, Splitter G. Antigen specific killing assay using CFSE labeled target cells. J Vis Exp. 2010;(45). PubMed: J Vis Exp. 2010 Nov 9;(45). pii: 2250. doi: 10.3791/2250.