ABSTRACT
Botulinum neurotoxins (BoNTs) produced by the spore-forming, gram-positive, anaerobic bacterium Clostridium botulinum are the most toxic substances known and cause botulism, flaccid paralysis, or death. Owing to their high lethality, BoNTs are classified as category A agents by the Centers for Disease Control (CDC). Currently, there are no vaccines available to protect against BoNTs, so the rapid development of a safe and effective vaccine is important. DNA-based vaccines have recently drawn great attention because they can be developed quickly and can be applied in mass vaccination strategies to prevent disease outbreaks. Here, we report on the immunogenic and protective efficacy of a DNA vaccine, encoding a 50-kDa carboxy-terminal fragment of the BoNT serotype E heavy chain, which is delivered via an intradermal route. This plasmid DNA vaccine induced robust humoral and cellular BoNT/E-specific immune responses and completely protected animals against lethal challenge with BoNT/E. These results not only indicate that DNA vaccines could be further developed as safe and effective candidates for vaccines against BoNTs but also suggest a possible approach for developing vaccines that protect against bio-threat toxins.
Introduction
Botulism is a neuroparalytic disease characterized by progressive muscle weakness that can be fatal when it leads to respiratory failure.Citation1 Natural intoxication with botulinum neurotoxins (BoNTs) commonly results from eating food contaminated with the toxin (foodborne botulism), contamination of a wound by Clostridium botulinum (C. botulinum) spores (wound botulism), and colonization of the gastrointestinal tract by C. botulinum after ingestion of its spores (intestinal botulism).Citation2 This disease can also occur when humans overdose on BoNT injections that are used for cosmetic and therapeutic purposes (iatrogenic botulism) or, potentially, when BoNTs are used as agents for bioterrorism and biological warfare.Citation3,Citation4
There are eight known immunologically distinct serotypes of BoNTs, categorized as serotypes A through H.Citation5,Citation6 Five of these, BoNT/A,/B,/E,/F, and/H, cause human disease and most cases of human botulism are associated with BoNT/A,/B, and/E. Each BoNT is produced as a 150 kDa single chain that is cleaved to form a 50 kDa N-terminal light chain and a 100 kDa C-terminal heavy chain, which are linked to one another by disulfide bonds. The light chain is a zinc protease that mediates the toxic activity of BoNTs and the heavy chain comprises the receptor-binding and translocation domains. Following binding of the C-terminal half of the heavy chain to its receptor, the N-terminal half of the heavy chain promotes translocation of the light chain into the cytosol, where it acts as an endopeptidase.Citation7,Citation8 Once inside a cell, BoNTs cause proteolytic cleavage of three specific proteins that are involved in the release of the neurotransmitter acetylcholine by nerve cells. BoNT serotypes A and E cleave the synaptosomal-associated protein 25 (SNAP-25), while serotype C cleaves syntaxin, and serotypes B, D, F, and G cleave vesicle-associated membrane proteins, such as synaptobrevins. Cleavage of these proteins in neuromuscular junctions prohibits the release of neurotransmitters and consequently leads to muscular paralysis.Citation9
Vaccination is an effective strategy for protecting against BoNTs because it elicits neutralizing antibodies that prevent binding of the toxins to receptors on nerve cells. A pentavalent (serotype A, B, C, D, and E) botulinum toxoid vaccine has been developed and administered as an investigational vaccine to people at risk for botulism.Citation10 This vaccine was derived from formalin-inactivated, partially purified BoNT serotypes A to E. However, use of this pentavalent vaccine was discontinued because of its poor performance and problems with its tolerability.Citation11 Therefore, efforts are needed to develop an improved, next-generation vaccine against botulism.
DNA vaccines can elicit a protective immune response by delivering genes that encode vaccinating antigens.Citation12 DNA vaccines offer many advantages over traditional vaccines, including simple production, easy storage, and convenient distribution. The manufacturing and quality-control processes needed to produce plasmid DNA in bacteria are well established, allowing DNA vaccines to be produced safely and at a low cost. DNA vaccines are advantageous because genetic manipulation can be performed quickly, which saves time during development. Further, because they are easy to develop, have a low cost, and are safe, DNA vaccines offer promising next-generation vaccines that can be rapidly deployed on a massive scale in response to bioterrorism emergencies or infectious disease pandemics.Citation13
Studies of subunit vaccines have demonstrated that the BoNT C-terminal domains of the heavy chains are sufficient to generate protective immunity against BoNTs.Citation14 In addition, DNA vaccines encoding C-terminal fragments of the heavy chains of serotypes A, B, E, and F successfully neutralize toxin activity.Citation15,Citation16 However, protective immunity gained by using these DNA vaccines was mainly evaluated using intramuscular vaccination. The purpose of this study was to further evaluate the humoral and cellular responses induced by a DNA vaccine delivered via an intradermal route, with the vaccine consisting of the C-terminal fragment of the heavy chain of BoNT serotype E.
Results
In vitro expression of DNA vaccine
The DNA vaccines examined in this study included the SV40 enhancer upstream of the codon-optimized HCt/E coding region, which was fused to the IgM signal peptide. To confirm the expression ability of the DNA vaccine, BoNT/HCt/E plasmids were transfected into mammalian 293T cells and encoded HCt/E proteins were observed. An immunoblot assay revealed that BoNT/HCt/E was able to promote expression of full-length HCt/E protein. As shown in , cells transfected with the codon-optimized HCt/E expressed a protein of approximately 48 kDa, corresponding to the C-terminal heavy chain domain fused to a 20 amino acid IgM signal peptide. As control cells, we transfected 293T cells with a pcDNA 3.1 vector backbone.
Figure 1. In vitro expression of the BoNT/HCt/E DNA vaccine. BoNT/HCt/E plasmid was transfected into 293T cells and cells were harvested 48 h post-transfection for expression of HCt/E. (A) Western blot analysis of cell lysate. The proteins were separated by SDS-PAGE and expression of HCt/E protein was detected using mouse polyclonal antibodies against HCt/E. Lane 1. purified HCt/E protein (1 μg); Lane 2, vector only control cells; Lanes 3, cells transfected with BoNT/HCt/E plasmids (7 μg). (B) Secretion of HCt/E proteins in cell culture supernatants. Secreted HCt/E proteins were assayed with capture ELISA. Data represent the mean ± standard deviation.
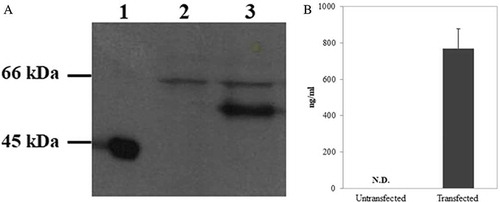
In order to determine if HCt/E could be stably expressed in and secreted from mammalian cells after transfection with BoNT/HCt/E, an enzyme-linked immunosorbent assay (ELISA) was performed. HCt/E protein was found in the culture supernatant and the level of secreted protein was approximately 770 ng/ml (). As expected, no HCt/E protein was detected in the supernatant of control cells.
Immunization with intradermal electroporation
To assess the ability of the DNA vaccine to induce a humoral immune response, Balb/c mice (n = 5) were immunized by an intradermal injection, followed by electroporation. Mice were vaccinated three times with 50 μg of BoNT/HCt/E plasmid at two-week intervals. After vaccination, blood sera were obtained and used to detect binding antibody titers by ELISA. BoNT/HCt/E-specific titers increased after each vaccination cycle. As shown in , no antibody was detected with the pre-immunized control group. However, the antibody titer was ten-fold higher with three injections than that obtained with two injections. The geometric mean of BoNT/HCt/E-specific titers induced by two and three injections were 1.9 × 103 and 2.2 × 104 respectively. Our data clearly demonstrated the ability of the BoNT/HCt/E DNA vaccine to induce a strong humoral immune response following intradermal electroporation delivery.
Figure 2. HCt/E-specific antibody production in mice immunized with BoNT/HCt/E DNA vaccine by intradermal electroporation. Mice were immunized 3 times (week 0, 2, 4) with 50 μg of DNA vaccine. Anti-HCt/E IgG levels are expressed as endpoint titers. Each symbol represents data from an individual mouse. The bar represents the mean titer for each group.
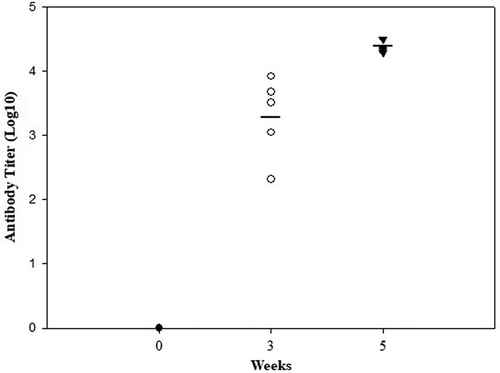
Antibody isotype profiles
To investigate the immune response elicited by the BoNT/HCt/E DNA vaccine, serum samples from vaccinated mice were collected to analyze the specific IgG subtypes using quantitative ELISA. As shown in , BoNT/HCt/E induced higher level of IgG1 and IgG2a antibody responses in week eight compared with the pre-immunized control group. Compare to the control group, there was significant induction of IgG1 (p < 0.05). Induction of IgG2a was also observed though induction did not reach statistical significance. The increase in HCt/E-specific IgG1 and IgG2a after immunization indicated that both Th1 and Th2 immune responses were stimulated. However, the increased level of IgG1 was noticeable and the resulting IgG1/IgG2a ratio was 4.0, suggesting the development of a strong humoral response after BoNT/HCt/E immunization.
Figure 3. Determination of antibody isotype in mice immunized with BoNT/HCt/E DNA vaccine. The sera were collected one week after the last immunization and analyzed for the subclass of antibody. Serum samples were diluted 1:10000. Data represent the mean ± standard deviation. Significant results are marked asterisks: * p < 0.05.
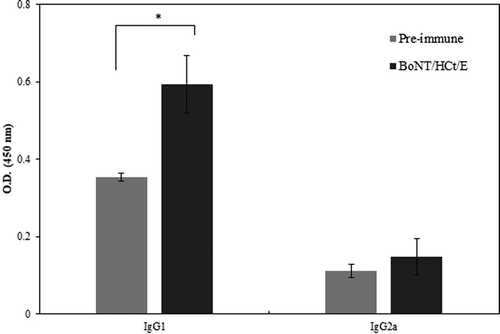
Cell-mediated immune response
We next investigated whether the BoNT/HCt/E DNA vaccine could induce a cell-mediated immune response in mice. Generally, the IFN-γ cytokine has been defined as a marker of the Th1 response. Splenocytes from vaccinated or control mice were in vitro stimulated with recombinant HCt/E protein and IFN-γ secretion was detected by the ELISPOT assay. As shown in , vaccinated mice presented significantly higher levels of IFN-γ when compared to samples collected from control mice (p < 0.05). These results suggested that the cell-mediated immune response was effectively induced by the BoNT/HCt/E DNA vaccine via intradermal electroporation administration.
Protection against challenge with BoNT/E
Because BoNT/HCt/E vaccination induced robust immunity, we evaluated whether vaccinated mice directly administered 50 LD50 of BoNT/E would be protected from intoxication. To perform this evaluation, three separate groups of mice were vaccinated with two 50 μg doses of DNA vaccine, three 50 μg doses of DNA vaccine, or two 100 μg doses of DNA vaccine. The survival data in demonstrates that the three 50 μg doses of DNA vaccine provided 100% protection. In comparison, two 50 μg doses of DNA vaccine protected only 20% of the mice against the toxin challenge. In addition, two 100 μg doses of DNA vaccine protected up to 80% of vaccinated mice.
Figure 5. Survival and antibody titers following immunization with BoNT/HCt/E DNA vaccine. Mice were immunized two or three times at 2-week intervals with various doses of DNA vaccine intradermally via electroporation, followed by intraperitoneal challenge with 50 LD50 of active BoNT/E. (A) Survival curves show the percentage of mice alive after challenge. Data shown are from one of two experiments. (B) Anti-HCt/E IgG levels are expressed as endpoint titers. Sera were obtained one week before challenged. Each symbol represents data from an individual mouse. The bar represents the mean titer for each group. Control group mice were immunized with vector only. Significant results are marked asterisks: ** p < 0.01.
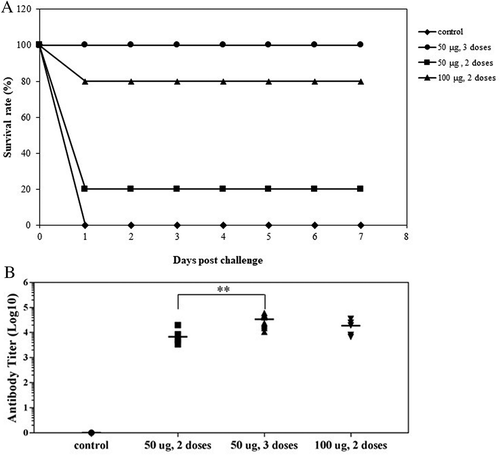
In order to further evaluate the protective ability of the DNA vaccine, serum antibody titers for individual mice one week before challenge were analyzed by ELISA and the geometric mean titers for each group in the study were determined. The sera results are shown in and imply that a trend exists between antibody level and survival rate. The geometric mean titers of an antibody in BoNT/HCt/E mice that were vaccinated with three doses of 50 μg was 2.9 x 104, which protected all mice from the effects of 50 LD50. Mice vaccinated with two 50 μg doses of BoNT/HCt/E produced an antibody response of 6.8 x 103. The antibody response in mice vaccinated with two 100 μg doses of BoNT/HCt/E tended to be higher than in mice vaccinated with two 50 μg doses of BoNT/HCt/E. The antibody response was 1.5 x 104, which protected four out of five mice. Thus, the most effective vaccination schedule was three 50 μg doses of BoNT/HCt/E given two weeks apart. Mice in the vector only control groups succumbed to botulism and died within one day post-challenge.
To further assess the vaccine’s potency for protection, higher doses of active BoNT/E were also used in toxin challenges. As shown in , three 50 μg doses of BoNT/HCt/E protected mice from challenges at 100 and 200 LD50 of BoNT/E units. These data indicate that immunization with BoNT/HCt/E provides an effective protection against BoNT/E.
Figure 6. Protection against various amounts of active BoNT/E challenged in BoNT/HCt/E DNA vaccine immunized mice. Mice were immunized with 50 μg of DNA vaccine intradermally via electroporation three times at 2-week intervals. Animals were then challenged with 100 or 200 LD50 of active BoNT/E two weeks after the last immunization. Control group mice were immunized with vector only. Data shown are from one of two experiments.
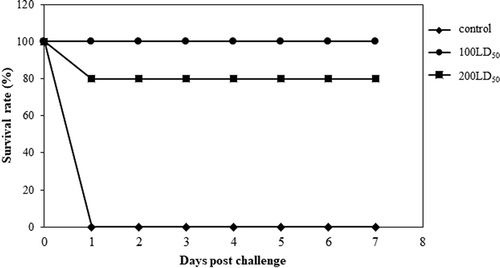
To compare the protective efficacy afforded by DNA vaccines and protein vaccines, we next immunized mice with HCt/E recombinant proteins. Immunization included three consecutive vaccinations, with a two-week interval between each immunization. As expected, mice immunized with 10 μg or 20 μg of purified HCt/E vaccine were fully protected against 200 LD50 of BoNT/E units (). One week after last immunization, sera were collected and anti-HCt/E IgG titers were measured. The high titer (3.0 x 104) antibodies were obtained when mice were immunized with three 20 μg dose of purified HCt/E vaccine, whereas immunization with a three 10 μg dose of purified HCt/E vaccine induced lower titers (2.4 x 104). Overall, these data indicate that protein vaccines display similar antibody titers when compared with three 50 μg dose of DNA vaccine.
Figure 7. Survival and antibody titers following immunization with HCt/E protein vaccine. Mice were immunized three times at 2-week intervals with 10 μg or 20 μg of purified HCt/E vaccine intramuscularly, followed by intraperitoneal challenge with 200 LD50 of active BoNT/E. The number of survivors are shown. Anti-HCt/E IgG levels are expressed as endpoint titers. Sera were obtained one week after last immunization. Each symbol represents data from an individual mouse. The bar represents the mean titer for each group.
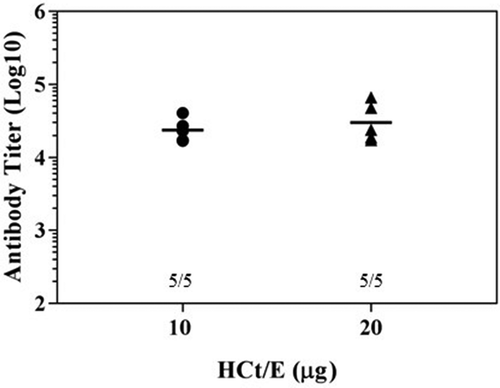
Discussion
The BoNTs are generally regarded as the most poisonous of all toxins; with lethal human doses of approximately one nanogram per kg of body weight.Citation5 BoNTs have been described as bioterrorism or biothreat agents because they have great potential for public health mass casualties and therefore, require broad-based public health preparedness efforts.Citation17 Because there are no FDA approved vaccines for botulism, it is important to develop vaccines against BoNTs for biodefense.
Several DNA vaccines have been developed as platforms for vaccines against botulism in animal model systems. As an appropriate vaccine candidate, plasmids containing the genes encoding carboxy-terminal fragments of BoNT heavy chains have proven to be efficient and safe immunogens for the generation of neutralizing antibodies, providing sufficient protection against various BoNTs serotypes. An early DNA vaccine study demonstrated that plasmid-based vaccination induced high carboxy-terminal fragments of BoNT/A antibodies and subsequently protected against a challenge with 25–100 LD50 of BoNT/A.Citation18 Later, Zeng et al. also reported that after injection with adenoviral vectors (2 x 107 pfu) encoding a human codon-optimized gene for BoNT/C HCt, the animals were completely protected against intraperitoneal challenge with 100 MLD50 of active BoNT/C.Citation19 However, previous studies with BoNT DNA vaccines have focused primarily on intramuscular electroporation delivery, whereas little data are available regarding immune responses associated with intradermal electroporation delivery of DNA vaccines for botulism.
In this study, we described the humoral and cellular immune responses induced by a DNA vaccine (BoNT/HCt/E) encoding the HCt/E protein, which was delivered intradermally with electroporation in Balb/c mice. Skin is an attractive site for DNA vaccine delivery because it is rich in potent antigen-presenting cells, such as Langerhans cells and dendritic cells. Skin antigen-presenting cells have the capacity for internalization, processing, and presentation of foreign antigens to the lymph nodes, resulting in the induction of both humoral and cellular immune responses.Citation20
Previous subunit vaccine studies have shown that protection of BoNT/E was antibody-mediated and antibody level has associated with protection.Citation21,Citation22 For example, using purified BoNT/E subunit vaccine candidate, Zichel et al. showed a significant correlation between the antibody titer and protection efficacy with increasing vaccine dose and number of vaccinations.Citation22 Consistent with previous reports, our findings also indicated that there was a positive relationship between serum levels of anti-HCt/E IgG antibodies and protective responses. Antibody titers were approximately 6.8 x 103 after two 50 μg injections of BoNT/HCt/E that protected one out of five mice against 50 LD50 of active BoNT/E, whereas 100% protection was obtained after three injections, producing approximately 2.9 x 104 titer values. We also demonstrated survival of four out of five mice following two 100 μg injections of BoNT/HCt/E and antibody titers were approximately 1.5 x 104. These results were then compared to recombinant protein vaccine data. Although both DNA and protein vaccines produced a similar level of antibody titer, protein vaccine obtained 100% protection against 200 LD50 challenge of toxin, whereas DNA vaccine protected 80% of mice. One possible explain for this slightly less protective effect is that protein vaccine may favor the induction of stronger antibody responses and antibody-mediated protection against toxin. In addition, it is worth noticing that in our results that the number of immunizations appeared to be a more important factor than the total injection volume of DNA vaccine for inducing more efficient protection, as shown in the 50 and 100 μg dose experiments.
To better understand the BoNT/HCt/E-induced immune response, antibody isotype analysis was performed using quantitative ELISA. It is generally known that IgG1 production is associated with Th2 immune responses, whereas Th1 immune responses are related to the induction of IgG2a.Citation23,Citation24 Earlier work suggests that the type of immune response following immunization with an intramuscular injection of BoNT DNA vaccine varied between studies. BoNT DNA vaccines have induced a predominately Th2-type immune response in some studies,Citation15,Citation16 or mixed Th1- and Th2-type immune responses in other groups.Citation25 In this study, intradermally delivered BoNT/HCt/E vaccine elicited both HCt/E-specific IgG1 and IgG2a with a skew toward IgG1, indicative of a robust Th2-type response in mice. These immunoglobulin type differences may be partially explained by the magnitude of expression, secretion of antigen, antigen itself, or the delivery route, which vary from one study to another.Citation15
In our study, we performed IFN-γ ELISPOT as the biomarker to measure BONT/HCt/E-specific T cell immune responses after splenocytes from immunized mice were stimulated in cultured medium with HCt/E antigen. Because antigen-specific T cell are required for stimulation of B cells, generating a strong cell-mediated immune response may result in increased antibody production.Citation26 The ELISPOT assay results demonstrated that an increase in the number of IFN-γ-secreting cells in the immunized mice relative to the control mice. It may be therefore be beneficial for our DNA vaccine to activate antibody-producing plasma cells and memory B cells. Our results are consistent with a recent study, which reported that strong IFN-γ-secreting T cell responses were elicited by a trivalent DNA vaccine targeting the heavy chain C-terminal fragment botulinum BoNT antigens.Citation27
In conclusion, this study demonstrated that intradermal administration of BoNT/HCt/E via electroporation induced robust immune responses and effectively protected mice against challenge with lethal doses of BoNT/E. Our results may contribute to the further development of safe and efficacious botulinum DNA vaccines. However, more studies are needed to optimize dosing regimens and formulations in nonhuman primates and these experiments will further define DNA vaccine effects.
Materials and methods
DNA vaccine construction
The nucleotide sequence encoding the C-terminal fragment of the heavy chain (HCt/E) of the BoNT serotype E from C. botulinum was obtained from GenBank (accession no. EES 49627.1) and its codons were optimized for expression in human cells using Genescript OptimumGeneTM. The codon-optimized HCt/E gene was then chemically synthesized (GeneScript, Piscataway, USA) and cloned into the pUC57 vector alongside an immunoglobulin M (IgM) signal sequence to increase secretion. The HCt/E gene containing the signal sequence was then cloned into a pcDNA 3.1 vector (Invitrogen, Carlsbad, USA) between its EcoRI and ApaI restriction sites to obtain an HCt/E protein expression vector. Expression from this vector was controlled by the CMV promoter and the enhancer from simian virus 40, which was inserted between the BglII and NruI sites located upstream of the vector’s coding region. This plasmid is referred to as BoNT/HCt/E.
Protein expression and antibodies
The amplified HCt/E gene was ligated into the pET14b vector to generate the recombinant expression vector, pET 14b-His-HCt/E. The recombinant plasmids were further transformed into E. coli strain BL21 (Rosetta) and grown in LB media containing ampicillin until reaching a cell density of 0.6 OD600. Induction of genes expressing HCt/E sequences was performed using 0.25 mM isopropyl-β-D-thiogalactopyranoside (IPTG) at 30°C for 12 h. The induced bacteria cells were harvested and lysed. The protein in the supernatants was purified by Ni-NPA spin column (Qiagen, Duesseldorf, Germany). HCt/E was eluted and cleaved to remove the His-tag via thrombin. Protein concentration was measured by a BCA protein assay kit (Pierce, Waltham, USA). Antibodies specific for the HCt/E were purified from polled immune serum using Protein A affinity chromatography (MbaSelect SuRe, GE Healthcare, Chicago, USA). The specificity of HCt/E antibodies against HCt/E was tested using an ELISA assay as previously described.Citation28 Briefly, the plates were coated with HCt/E protein (1 μg/ml) overnight. Then the plates were blocked with 1% skim milk in PBS. Serially diluted antibodies were incubated for 1 h at 37°C. HCt/E-specific antibodies were revealed with horseradish peroxidase-conjugated goat anti-mouse IgG followed by 3,3ʹ,5,5ʹ-tetramethylbenzidine (TMB) as a substrate and stop solution and lastly read the absorbance.
In vitro expression of DNA vaccine
BoNT/E/HCt was expressed in 293T cells following transfection using Vivagen (Seoul, Korea), according to the manufacturer’s instructions. Forty-eight hours post-transfection, cells were harvested and cell lysates were prepared for western blot analysis. The HCt/E protein was separated by electrophoresis on a 4–20% polyacrylamide gel. The HCt/E protein was detected using a mouse anti-HCt/E antibody and goat anti-mouse IgG conjugated to horseradish peroxidase (Santa Cruz Biotech, Santa Cruz, USA). Proteins were identified using Western Lighting Plus-ECL (PerkinElmer, Waltham, USA).
Capture ELISA
The quantity of HCt/E protein secreted into the cell culture supernatant was determined 48 h post-transfection. Microtiter plates were coated with anti-HCt/E monoclonal antibody (10 μg/ml) overnight at 4°C. The plates were then washed three times with wash buffer (PBS containing 0.02% Tween 20) and blocked with 1% skim milk for 1 h. Serial dilutions of cell supernatants in blocking buffer were applied to the plates and then incubated at 37°C for 1 h. After another wash, mouse anti-HCt/E antibody (10 μg/ml) was added to the plates and incubated for an additional 1 h at 37°C. Binding products were detected by incubation with horseradish peroxidase-conjugated goat anti-mouse secondary antibody. After a wash step, the bound antibody was detected colorimetrically using TMB as a substrate and the A405 was measured. The amount of secreted HCt/E protein for each sample was determined using a standard curve.
Immunization of mice
Groups of female Balb/c mice (6 to 8 weeks of age, n = 5–6) were purchased from Samtako (Osan, Korea). DNA vaccines were delivered into mice by intradermal administration of naked plasmid DNA combined with electroporation. Mice were immunized in the skin three times at two-week intervals. As a control, mice were injected with control vector (pcDNA 3.1) as above. Electroporation experiments were performed as previously described.Citation28 Briefly, mice were anesthetized intraperitoneally with Zoletil (2 mg/kg) and sites on the flank were shaved. Immediately after injection of the plasmid DNA, two-needle array electrodes (BTX, Hollison, USA) were placed over the injection site. Using a BTX ECM-830 electroporator (Hollison, USA), electric pulses of 90 V/mm and 25 ms in length were generated, followed by three pulses with reversal of polarity after each pulse. For protein vaccine immunization, E coli-derived HCt/E was formulated in 2% alhydrogel (InvivoGen, San Diego, USA). Mice were immunized intramuscularly three times at two-week intervals with 10 μg or 20 μg of recombinant protein.
Antibody titer measurements
To measure antibody production, sera were collected at various time points and stored at −20°C until assayed. 96-well plates were coated with HCt/E protein (1 μg/ml) purified from E. coli, incubated overnight at 4°C, and then blocked for 1 h with block buffer (2% skim milk in PBS). Serial dilutions of mouse serum samples in block buffer were then added. The plates were incubated at 37°C for 1 h and washed three times. Peroxidase-conjugated goat anti-mouse IgG was added and bound antibodies were detected colorimetrically. Endpoint titers were reported as the highest dilution of each sample with an A450 ≥ 0.1 after subtraction of the A450 of the serum samples from unvaccinated mice at the same dilution.
Immunoglobulin isotyping
Antibody isotypes were identified using Isotyping Kits for Immunoglobulins (eBioscience, San Diego, USA) following the manufacturer’s instructions. The sera were collected one week after the last immunization and analyzed for the antibody subclass. Serum samples were diluted 1:10,000. Microplates were read by a Microplate Spectrophotometer (Molecular Devices, USA) at a wavelength of 450 nm.
ELISPOT assay
IFN-γ responses specific to the HCt/E were assessed using a mouse IFN-γ-specific ELISPOT kit following the manufacturer’s protocol (R&D Systems, Minneapolis, USA). Suspensions of spleen cells from immunized mice were added to the wells (2 × 105 cells per well) and re-stimulated for 48 h with the antigen (10 μg/ml). After extensive washing, the plates were incubated with biotinylated anti-mouse IFN-γ antibody at 4°C for 16 h and then streptavidin-alkaline phosphatase for 2 h at room temperature. Finally, spots were detected using BCIP/NBT Chromogen as the substrate and the number of IFN-γ-producing cells was counted using the EliSpot Reader System ERL02 (AID, Strassberg, Germany).
Vaccine efficacy
Mice were challenged by intraperitoneal injection with different dosages of active BoNT/E two weeks after their last vaccination. The control group received the plasmid pcDNA 3.1. The mice were monitored every day for seven days for intoxication symptoms or death in order to determine the status of vaccine protection. Surviving mice were humanely euthanized after one week. The animal experiments conducted for this study were approved by the Institutional Animal Care and Use Committee (IACUC) of our institution (approval ID: Add-iacuc-16–27).
Statistical analysis
Data were analyzed using GraphPad Prism software (San Diego, USA). Based on the type of data, a Student’s t-test or one-way ANOVA, followed by Tukey’s test were performed. A p < 0.05 was considered statistically significant.
Disclosure of potential conflicts of interest
No potential conflict of interest was reported by the authors.
Additional information
Funding
References
- Sobel J. Botulism. Clinic Infect Dis. 2005;41:1167–1173. doi:10.1086/444507.
- CDC. Botulism. Altlanta (GA): Centers of disease control and prevention 2017; 2017.
- Wheeler A, Smith HS. Botulinum toxins: mechanism of action, antinociception and clinical application. Toxicology. 2013;306:124–146. doi:10.1016/j.tox.2013.02.006.
- Middlebrook JL. Production of vaccines against leading biowarfare toxins can utilize DNA scientific technology. Adv Drug Delivery Rev. 2005;57:1415–1423. doi:10.1016/j.addr.2005.01.016.
- Aron SS, Schechter R, Inglesby TV, Henderson DA, Barlett JG, Ascher MS, Eitzen E, Fine AD, Hauer J, Layton M, et al. Botulinum toxin as biological weapon: medical and public health management. JAMA. 2001;285:1059–1070.
- Barash JR, Arnon SS. A novel strain of Clostridium botulinum that produces type B and type H botulinum toxins. J Infect Dis. 2014;209:183–191. doi:10.1093/infdis/jit449.
- Simpson LL. Identification of the major step in botulinum toxin action. Annu Rev Pharmacol Toxicol. 2004;44:167–193. doi:10.1146/annurev.pharmtox.44.101802.121554.
- Dickerson TJ, Janda KD. The use of small molecules to investigate molecular mechanism and therapeutic targets for treatment of botulinum neurotoxin A intoxication. ACS Chem Bio. 2006;6:359–369. doi:10.1021/cb600179d.
- Montecucco C, Rossetto O, Schiavo G. Presynaptic receptor arrays for clostridial neurotoxins. Trends Microbiol. 2004;12:442–446. doi:10.1016/j.tim.2004.08.002.
- Byrne MP, Smith LA. Development of vaccines for prevention of botulism. Biochemie. 2000;82:955–966. doi:10.1016/S0300-9084(00)01173-1.
- Sundeen G, Barbieri JT. Vaccines against botulism. Toxins. 2017;9:268. doi:10.3390/toxins9090268.
- Garmory HS, Perkins SD, Phillpotts RJ, Titball RW. DNA vaccines for biodefence. Adv Drug Delivery Rev. 2005;57:1343–1361. doi:10.1016/j.addr.2005.01.013.
- Tregoning JS, Kinnear E. Using plasmids as DNA vaccines for infectious diseases. Microbiol Spectr. 2014;2:PLAS-0028-2014.
- Smith LA. Botulism and vaccines for its prevention. Vaccine. 2009;27:D33–39. doi:10.1016/j.vaccine.2009.08.059.
- Bennett AM, Perkins SD, Holley PJ. DNA vaccination protects against botulism neurotoxi in type F. Vaccine. 2003;21:3110–3117.
- Trollet C, Pereira Y, Burgain A, Litzler E, Mezrahi M, Seguin J, Manich M, Popoff MR, Scherman D, Bigey P. Generation of high-titer neutralizing antibodies against botulinum toxins A, B, and E by electrotransfer. Infect Immun. 2009;77:2221–2229. doi:10.1128/IAI.01269-08.
- Smith TJ, Roxas-Duncan VI, Smith LA. Botulinum neurotoxins as biothreat agents. J Bioterr Biodef. 2014;S2:003.
- Clayton J, Middlebrook JL. Vaccination of mice with DNA encoding a large fragment of botulinum neurotoxin serotype A. Vaccine. 2000;18:1855–1862.
- Zeng M, Xu Q, Elias M, Pichichero ME, Simpson LL, Smith LA. Protective immunity against botulism provided by a single dose vaccination with an adenovirus-vectored vaccine. Vaccine. 2007;25:7540–7548. doi:10.1016/j.vaccine.2007.08.035.
- Kupper TS, Fuhlbrigge RC. Immune surveillance in the skin: mechanism and clinical consequences. Nat Rev Immunol. 2004;4:211–222. doi:10.1038/nri1310.
- Dux MP, Barent R, Sinha J, Gouthro M, Swanson T, Barthuli A, Iana M, Ross JT, Smith LA, Smith TJ, et al. Purification and scale up of a recombinant heavy chain fragment C of botulinum neurotoxin serotype E in Pichia pastoris GS115. Protein Expr Puri. 2006;45:359–367. doi:10.1016/j.pep.2005.08.015.
- Zichel R, Mimran A, Keren A, Barnea A, Steinberger-Levy I, Marcus D, Turgeman A, Reuveny S. Efficiency of a potential trivalent vaccine based on HC fragments of botulinum toxins A, B, and E produced in a cell-free expression system. Clin Vaccine Immunol. 2010;17:784–792. doi:10.1128/CVI.00496-09.
- Hess J, Schaible U, Raupach B, Kaufmann SH. Exploiting the immune system: toward new vaccines against intracellular bacteria. Adv Immunol. 2000;75:1–88.
- Visciano ML, Tagliamonte M, Tornesello ML, Buonaguro FM, Buonaguro L. Effects of adjuvants on IgG subclasses elicited by virus-like particles. J Transl Med. 2012;10:4. doi:10.1186/1479-5876-10-233.
- Jathoul AP, Holley JL, Garmory HS. Efficacy of DNA vaccines expressing the type F botulinum toxin Hc fragment using different promoters. Vaccine. 2004;22:3942–3946. doi:10.1016/j.vaccine.2004.04.006.
- Crotty S. A brief history of T cell help to B cell. Nat Rev Immunol. 2015;15:185–189. doi:10.1038/nri3803.
- Scott VL, Villarreal DO, Hutnick NA, Walters JN, Ragwan E, Bdeir K, Yan J, Sardesai NY, Finnefrock AC, Casimiro DR, et al. DNA vaccines targeting heavy chain C-terminal fragments of Clostridium botulinum neurotoxin serotypes A, B, and E induce potent humoral and cellular immunity and provide protection from lethal toxin challenge. Hum Vaccin Immunother. 2015;11:1961–1971. doi:10.1080/21645515.2015.1066051.
- Kim NY, Chang DS, Kim Y, Kim CH, Hur GH, Yang JM, Shin S. Enhanced immune response to DNA vaccine encoding Bacillus anthracis PA-D4 protects mice against anthrax spore challenge. PLoS One. 2015;10:e0139671. doi:10.1371/journal.pone.0139671.