ABSTRACT
Streptococcus pneumoniae is an infectious pathogen mainly infecting host bodies through the respiratory system. An effective pneumococcal vaccine would be targeted to the mucosa and provide not only protection against invasive infection but also against colonization in the respiratory system. In the present work, we applied bacterium-like particles (BLPs) as an adjuvant for the development of a PspA mucosal vaccine, in which the PspA protein was displayed on the surface of BLPs. Intranasal immunization with the PspA-BLP pneumococcal vaccine, comprised of PspA2 from pneumococcal family 1 and PspA4 from pneumococcal family 2, not only induced a high level of serum IgG antibodies but also a high level of mucosal SIgA antibodies. Analysis of binding of serum antibodies to intact bacteria showed a broad coverage of binding to pneumococcal strains expressing PspA from clade 1 to 5. Immunization with the PspA-BLP vaccine conferred protection against fatal intranasal challenge with both PspA family 1 and family 2 pneumococcal strains regardless of serotype. Therefore, the PspA-BLP pneumococcal vaccine was demonstrated to be a promising strategy for mucosal immunization to enhance both systemic and mucosal immune responses.
Introduction
Streptococcus pneumoniae is a leading cause of infectious bacterial disease in children. It is estimated over one million deaths are caused by pneumonia in children younger than five years old every year, and about 33% of the deaths are due to the infection of Streptococcus pneumoniae.Citation1,Citation2 Pneumococcal disease is preventable, and the widespread use of pneumococcal polysaccharide-based vaccines has remarkably reduced the incidence of this disease associated with vaccine-covered serotypes, especially conjugate pneumococcal vaccine which could induce T cell response and longer immunity has successfully reduced pediatric pneumococcal disease incidence.Citation3–Citation7 However, the limited serotype coverage of this type of vaccine currently cannot completely protect people against all of the more than 90 serotypes of S. pneumoniae, and the replacement of non-vaccine coverage serotypes in pneumococcal infections is of great concern with the use of polysaccharide-based vaccines.Citation8–Citation10 Therefore, developing an alternative vaccine with broad coverage against pneumococcal diseases would be beneficial. Protein-based pneumococcal vaccines are promising candidates due to the level of conservation compared with polysaccharides. Many pneumococcal proteins have been studied for years with some positive results.Citation11–Citation13
Among all the proteins on the surface of S. pneumoniae, pneumococcal surface protein A (PspA) is one of the best vaccine candidates. PspA is a choline-binding protein, which interferes with complement deposition on the surface of S. pneumoniae and binds human lactoferrin in order to inhibit being killed by it. Based on its gene variations, PspA has been classified into three families, including six clades. Family 1 includes clades 1 and 2; family 2 includes clades 3, 4 and 5; family 3 includes only clade 6.Citation14,Citation15 Although PspA is variable, antibodies to PspA are highly cross-reactive and protective, especially in the same family.Citation16,Citation17 In previous studies, more than 96% of clinically isolated pneumococcal strains were shown to express family 1 or 2 PspA.Citation8,Citation18–Citation21 Many studies have shown that PspA could elicit protection against pneumococcal infections including bacteremia, sepsis, pneumonia and nasal carriage in animal models.Citation17,Citation22–Citation25 It has been reported selection of only single PspA Fragments could induce broad-ranging cross-reactivity.Citation17,Citation26 Other studies have reported that broad protective immunity is elicited by fusion pneumococcal PspA vaccine comprising of one PspA fragment from family 1 and the other PspA fragment from family 2.Citation27,Citation28 There is also intranasal immunization with PspA fused with a flagellin which could enhance cross-protective immunity against challenge in mice.Citation29
In order to overcome the variability of PspA, a combination of two PspA antigens, one from family 1 and the other from family 2, was proposed in order to best optimize a strategy for eliciting protection against a majority of pneumococcal strains.Citation30–Citation32 Mucosal immunization would be a good choice for the prevention of S. pneumoniae infection, which is expected to be effective against colonization. In our previous study, we developed a mucosal pneumococcal vaccine candidate based on pneumolysin mutant, in which bacterium-like particles (BLPs) were applied as a mucosal adjuvant and carrier. Intranasal immunization of mice with pneumolysin mutant adjuvanted with BLP not only induced a high level of serum IgG antibodies but also a high level of mucosal SIgA antibodies in lung lavage.Citation33 Unfortunately, in the following study, we found that the pneumolysin based vaccine couldn’t provide efficient protection against challenge. In this study, we applied this antigen delivery system to a more promising protein, PspA. Due to gene variations of PspA, it is a good idea to incorporate two PspA protein antigens from PspA family 1 and family 2 into the BLP-based pneumococcal vaccine in order to obtain broad coverage of protection. With this aim, in this study, PspA-BLP pneumococcal vaccine was designed to include PspA proteins from two different families, PspA2 from family 1 and PspA4 from family 2, and formulated with a BLP adjuvant also acting as a carrier. The PspA-BLP pneumococcal vaccine was developed as a serotype-independent prophylactic vaccine against S. pneumoniae.
Results
Analysis of binding of recombinant PspA2-PA and PspA4-PA to BLPs
With the aim of developing a pneumococcal particle vaccine for mucosal immunization, the BLP particles were used in this study. The BLPs was used as a carrier to display antigen on the surface, therefore, the binding of PspA2-PA or PspA4-PA to BLPs was an important indicator to assess the quality of the vaccine. PspA2-PA or PspA4-PA were produced by induction of a culture of E. coli BL21 transformed with pET-20b-PspA2-PA or pET-20b-PspA4-PA. After sonication and centrifugation of the harvest bacteria, supernatants containing PspA2-PA or PspA4-PA were incubated with BLP separately. Thereafter, the BLPs were collected and washed and suspended in PBS, and the binding of PspA2-PA or PspA4-PA with BLPs was analyzed by SDS-PAGE (). Compared with the BLP control, PspA2-PA and PspA4-PA were shown to obviously bind with BLPs. The amounts of PspA2-PA or PspA4-PA bound to the BLPs were determined according to the comparison with BSA standards by scanning of the SDS-PAGE gel (). Without PA, no PspA2 or PspA4 binding with BLPs was detected, while PspA2-PA or PspA4-PA could bind with BLPs efficiently with the capacity of about 45 μg PspA2-PA per 1 mg BLP and 60 μg PspA4-PA per 1 mg BLPs.
Figure 1. Identification of PspA2-PA and PspA4-PA binding with BLPs. (A) SDS-PAGE analysis of PspA2-PA and PspA4-PA binding with BLPs. Lane 1, protein molecular weight marker; lane 2, PspA2-PA binding with BLP; lane 3, BLP control; lane 4, PspA4-PA binding with BLP. (B) Binding capacity of PspA2-PA and PspA4-PA with BLPs. The amount of proteins binding to the BLPs was calculated by comparison with BSA standards in SDS-PAGE analysis.
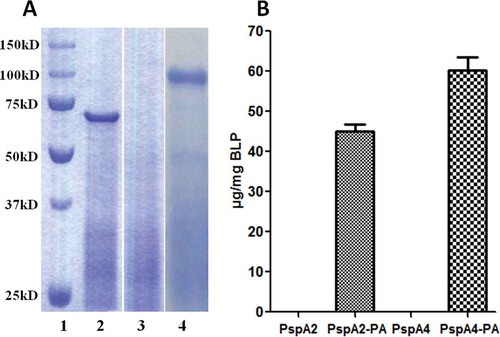
Analysis of antigenicity of PspA2-BLP and PspA4-BLP
According to the results of the SDS-PAGE analysis as shown in , PspA2-PA and PspA4-PA were confirmed to be bound with BLPs, called PspA2-BLP and PspA4-BLP, respectively. However, to further discuss whether the binding form of PspA2-PA and PspA4-PA in PspA2-BLP and PspA4-BLP would affect the antigenicity of the PspA2 and PspA4 antigens, and whether the binding BLP with antigen would affect the normal shape of BLPs, the PspA2-BLP and PspA4-BLP samples were observed and analyzed by confocal immunofluorescence microscopy with specific anti-PspA2 and anti-PspA4 antibodies. In contrast with the BLP unbound with antigen, PspA2-BLP and PspA4-BLP were shown to be recognized by the specific anti-PspA2 and anti-PspA4 antibodies; meanwhile, the shapes of the PspA2-BLP and PspA4-BLP particles were the same as that of the unbound BLP (). The results indicated that the binding of PspA2-PA and PspA4-PA with BLPs kept the antigenicity characteristic of the PspA2 and PspA4, and the binding of BLP with antigens did not affect the shape of BLP itself.
Figure 2. Analysis of antigenicity of PspA2-BLP and PspA4-BLP by confocal immunofluorescence microscopy. (A) PspA2-BLP, BLP. (B) PspA4-BLP, BLP. PspA2-BLP or PspA4-BLP was incubated with anti-PspA2 or anti-PspA4 antibody. BLP as the control and FITC-labeled secondary antibodies were used. The preparations were analyzed using bright field and fluorescence microscopy.
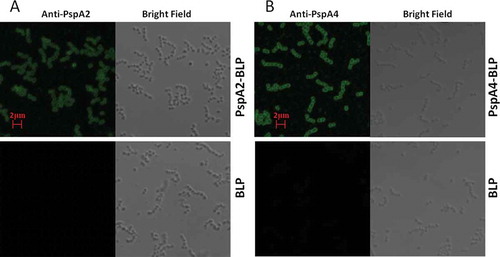
Intranasal immunization with PspA-BLP pneumococcal vaccine enhances both systemic and mucosal immune responses in mouse model
Samples obtained from immunized BALB/c mice with PspA-BLP pneumococcal vaccine were analyzed by ELISA against the individual PspA2 and PspA4 antigens. Specific anti-PspA2 and PspA4 antibody titers no mater IgG in serum or IgA in mucosa were significantly higher in the group immunized with PspA-BLP than the BLP control group as shown in . Compared with the BLP control group, intranasal immunization of PspA-BLP not only induced a significantly high level of specific IgG antibodies in serum but also induced a high level of specific SIgA antibodies in the mucosa. The results showed that the PspA-BLP pneumococcal vaccine was capable of inducing both mucosal immune responses in the respiratory tract and systemic immune responses in serum via mucosal immunization.
Antibodies induced by PspA-BLP pneumococcal vaccine bind to the surface of pneumococcal strains bearing different PspAs
To discuss whether the antibodies induced by PspA-BLP pneumococcal vaccine could bind intact bacteria, antibody binding ability analysis with intact bacteria was analyzed by flow cytometry. The experiment was done two times. The result was almost the same. Compared with BLP control group, FITC intensity increased in the PspA-BLP group in all the pneumococcal strains expressing PspA from clade 1 to 5, as shown in . It showed that antibodies induced by PspA-BLP pneumococcal vaccine could efficiently bind to the surface of intact Streptococcus pneumoniae regardless of PspA types.
Figure 4. Binding of serum antibodies to intact pneumococci. Sera from mice immunized with PspA-BLP or BLP were tested for the ability to bind to pneumococcal strains expressing PspA from clade 1 (A), clade 2 (B), clade 3 (C), clade 4 (D) and clade 5 (E). Results are shown as fluoresence intensity for each group.
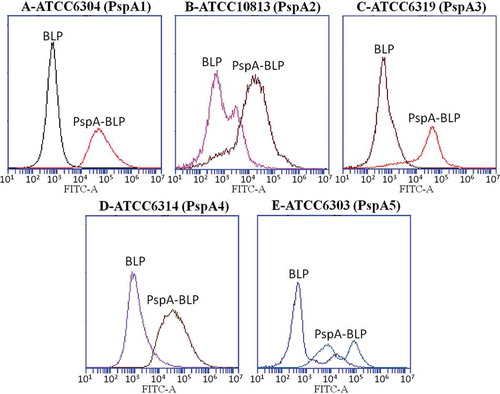
Intranasal immunization with PspA-BLP pneumococcal vaccine protects mice against fatal pneumococcal challenge
We discussed above that the PspA-BLP pneumococcal vaccine showed good immunogenicity via mucosal administration route. In the following study, we evaluated whether the PspA-BLP pneumococcal vaccine could confer protection against pneumococcal challenge. Considering the variability of PspA and in order to evaluate the breadth of coverage of the PspA-BLP pneumococcal vaccine, we determined its protective ability against two pneumococcal strains, one from PspA family 1 and the other from PspA family 2. In previous studies, more than 96% of clinically isolated pneumococcal strains were shown to express family 1 or 2 PspA.Citation8,Citation18–Citation21 To this aim, mice were immunized three times intranasally with the PspA-BLP vaccine, and mice immunized with BLP (intranasally) were included as negative control. Commercial 23-valent PPV (subcutaneously) was included as positive control, and mice were immunized only once in this group according the instruction of the vaccine. After vaccination of the PspA-BLP vaccine, BLP and 23-valent PPV, all the mice were anesthetized and challenged intranasally with 40 μl volume of Streptococcus pneumoniae containing 1.04 × 104 CFU of strain ATCC10813 (PspA family 1, clade 2) or 2.2 × 106 CFU of strain ATCC6303 (PspA family 2, clade 5). Mortality was monitored for 14 days after the pneumococcal challenge. As shown in , when challenged intranasally with ATCC10813 (PspA family 1), BLP negative immunization group didn’t showed any protection and all the mice were dead, while both 23-valent PPV and PspA-BLP vaccine groups showed great protection against lethal challenge. The survival rate of the PspA-BLP vaccine group reached 100% protection, which was better than that of the 23-valent PPV group. We then assessed the protective effect of the PspA-BLP vaccine against the PspA family 2 strain. When challenged intranasally with ATCC6303 (PspA family 2), the result as shown in was almost the same as that after challenge with ATCC10813 (PspA family 1). Once again the BLP negative control group didn’t show any protection, the PspA-BLP pneumococcal vaccine conferred 100% protection against challenge better than that of the 23-valent PPV group.
Figure 5. Protection against pneumococcal challenge with strains bearing heterologous PspAs. Mice were intranasally immunized with PspA-BLP pneumococcal vaccine and BLP as negative control. The 23-valent PPV was used as a positive control, which was immunized in mice via the subcutaneous route. Two weeks after the third immunization of PspA-BLP and BLP (six weeks after the first immunization of 23-valent PPV), the immunized mice were challenged intranasally with S. pneumoniae. (A) Immunized mice were challenged with the ATCC10813 pneumococcal strain (PspA family 1). (B) Immunized mice were challenged with the ATCC6303 pneumococcal strain (PspA family 2). Ten mice per group were examined in each challenge experiment. Mortality was monitored for 14 days, and survival curves are shown in the figure. **P < 0.01 versus BLP control group.
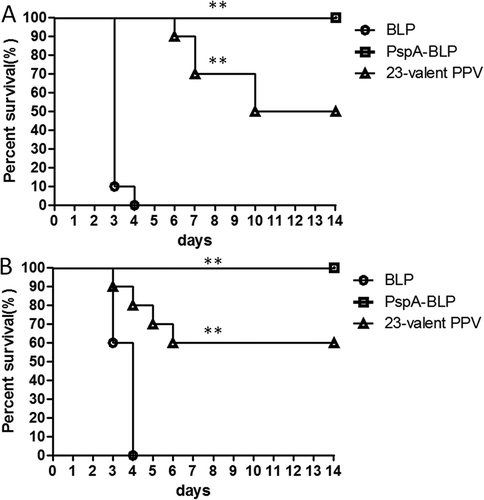
Taken together, these results showed that the PspA-BLP pneumococcal intranasal vaccine comprised of PspA2 fragment from family 1 and PspA4 fragment from family 2 could confer effective protection against challenge with pneumococcal strains from both PspA families 1 and 2 via a nature infection route, and the protective effect was greater than that provided by the 23-valent PPV.
Discussion
S. pneumoniae is an infectious pathogen, which infects the host mainly through the respiratory system. A potent pneumococcal vaccine should not only provide protection against invasive infection but also against colonization in the respiratory system. The currently available pneumococcal vaccines are based on the serotype-specific polysaccharide capsule of the bacterium.Citation34 As those vaccines are administered via the intramuscular injection route, they are generally poor inducers of mucosa immunity and are therefore less effective against infection at mucosal surfaces.Citation35,Citation36 In addition, these vaccines have limited serotype coverage and serotype replacement after vaccination. Therefore, developing more effective alternative vaccines against pneumococcal diseases is needed.
BLPs have been studied for many years as a mucosal vaccine carrier and adjuvant with some promising results.Citation37–Citation41 BLPs are non-living particles with a shape and size similar to that of bacteria, which are favorable for uptake by membranous cells on the mucosal surface and stimulation of host immunity. The BLPs could activate antigen-presenting cells which increase uptake of antigen. The capacity of recognition and activation of innate immunity by BLP was mediated through TLR2.Citation41
In our previous study, BLPs were applied as a mucosal adjuvant and carrier for a mucosal pneumococcal vaccine based on pneumolysin mutant. Intranasal immunization of mice with pneumolysin mutant adjuvanted with BLP demonstrated good immunogenicity by inducing not only a high level of serum IgG antibodies but also a high level of mucosal SIgA antibodies in bronchoalveolar washes.Citation33 The results indicated that the BLP technique could be a feasible strategy for the developing of a mucosal pneumococcal vaccine. Unfortunately, in the following study, we found that the pneumolysin based vaccine couldn’t provide efficient protection against challenge. In this study, we applied this antigen delivery system to a more promising protein, PspA. Although PspA is a promising pneumococcal vaccine candidate, it is not conserved in all clinical strains. PspA has been classified into three families including six clades based on the gene variations. In order to overcome the variability of PspA, a combination of two PspA antigens, one from family 1 and the other from family 2 was proposed to optimize a strategy for eliciting protection against the majority of pneumococcal stains. Considering the need for broad coverage of protection, in this study, we incorporated two PspA protein antigens into the BLP-based pneumococcal vaccine. This PspA-BLP pneumococcal vaccine included PspA proteins from two different families, PspA2 from family 1 and PspA4 from family 2. This new vaccine was formulated with the BLP adjuvant serving also as the carrier. We showed that intranasal immunization with the PspA-BLP vaccine efficiently induced not only PspA2- and PspA4-specific IgG in the serum but also PspA2- and PspA4-specific IgA in the mucosa. Analysis of binding of serum antibodies to intact bacteria showed efficient binding to pneumococcal strains expressing PspA from clade 1 to 5. Moreover, the PspA-BLP vaccine could provide effective protection against pneumococcal challenges by strains with different PspA families.
Although Pneumolysin is conserved in all clinical isolates, the Pneumolysin-BLP couldn’t induce sufficient protection against challenge. That lies in the protein itself. Pneumolysin is a secreted virulence factor and has cytotoxic activity resulting in cell lysis.Citation42 Anti-Pneumolysin antibodies could neutralize the function of Pneumolysin, but it couldn’t inhibit the propagation of S. pneumoniae. Pneumolysin is also responsible for the induction of inflammation which facilitate the clearance of bacteria by recruitment and activation of leukocytes.Citation43,Citation44 While PspA is on the surface of S. pneumoniae, anti-PspA antibodies could increase complement deposition on the surface of S. pneumoniae and inhibit binding lactoferrin, which facilitate the clearance of S. pneumoniae from host. Maybe that is the reason why PspA-BLP vaccine is better than Pneumolysin-BLP vaccine. Many studies have shown that PspA could elicit protection against pneumococcal infections including bacteremia, sepsis, pneumonia and nasal carriage in animal models. It has been reported selection of only single PspA Fragments could induce broad-ranging cross-reactivity.Citation17,Citation26 Considering the variability of PspA, some studies have reported that broad protective immunity was elicited by fusion pneumococcal PspA vaccine comprising of one PspA fragment from family 1 and the other PspA fragment from family 2.Citation27,Citation28 Some researchers applied intranasal immunization with PspA fused with a flagellin which could enhance cross-protective immunity against challenge in mice.Citation29 These results are accordance with our results that PspA vaccine comprising one or two PspA fragments could induce broad cross-protective immunity and it is feasible to apply PspA protein to mucosal immunization with adjuvant.
Although it is a feasible and promising strategy for mucosal immunization in animal model, it still has a long way to apply this vaccine in human. First, there is no vaccine available on the market with BLP adjuvant, we have to further to assess the safety and efficacy of this PspA-BLP mucosal vaccine. Second, we do not have a clear idea how to assess the efficacy of this PspA-BLP vaccine in human because there is only pneumococcal polysaccharide-based vaccine and no pneumococcal protein-based vaccine available on the market. Third, opsonophagocytosis could be an efficient means to evaluate the protective immune responses, but we haven’t established this experiment. Therefore, there are still many challenges ahead, but we will try our best to overcome all the difficulties to promote the development of pneumococcal mucosal vaccine.
In conclusion, the PspA-BLP vaccine comprised of PspA2 from pneumococcal family 1 and PspA4 from pneumococcal family 2 was demonstrated as a promising strategy for mucosal immunization to enhance both systemic and mucosal immune responses. It could confer broad coverage of protection against fatal intranasal challenge with PspA family 1 and family 2 strains regardless of serotype.
Materials and methods
Pneumococcal strains and culture conditions
S. pneumoniae strains used in this study were included ATCC6304 (PspA family 1, clade 1), ATCC10813 (PspA family 1, clade 2), ATCC6319 (PspA family 2, clade 3), ATCC6314 (PspA family 2, clade 4) and ATCC6303 (PspA family 2, clade 5). Pneumococci were cultivated in Todd-Hewitt broth culture medium added with 0.5% yeast extract. After culture, the Pneumococci were divided into small volume and stored at −80°C. For the determination of titer, the frozen bacteria were thawed and cultured on blood agar plates at 37°C containing 5% CO2 for 24 hours to count colony-forming units (CFU).
Plasmid construction and production of PspA-PA fusion protein
In order to increase protein expression in Escherichia coli system, Gene fragments encoding PspA2-PA and PspA4-PA were optimized (The GenBank accession numbers for PspA2-PA are MH252423 and MH252424, and the GenBank accession numbers for PspA4-PA are MH252425 and MH252424). And the gene fragments with Escherichia coli preferred codons for the PspA2-PA and PspA4-PA were synthesized by gene synthesis company (GENERY Biotechnology). Protein anchor (PA) in the fusion proteins is acted as a protein anchor which could bind BLP carrier efficiently. It is the C-terminus of the lactococcal protein ACMA comprised of three lysin motif (LysM) domains.Citation45 The PspA2 part is comprised of N-terminal region of S. pneumoniae family 1 PspA (S pneumoniae strain RX1, GenBank: M74122.1) and PspA4 part is comprised of N-terminal region and proline-rich region of S. pneumoniae family 2 PspA (S. pneumoniae strain EF5668, GenBank: U89711.1). The cDNAs encoding PspA2-PA protein or PspA4-PA protein was sub-cloned into the pET-20b expression vector (Novagen). The resultant constructs, designated as pET-20b-PspA2-PA and pET-20b-PspA4-PA, were then transformed into E. coli BL21.
PspA-BLP pneumococcal vaccine preparation
Both recombinant proteins PspA2-PA and PspA4-PA were expressed in E. coli BL21 (DE3). After sonication and centrifugation of the harvest of the expressed bacteria, the supernatants containing PspA2-PA or PspA4-PA were filtered through 0.22-μm filters to remove cell debris. The cell-free extracts containing PspA2-PA or PspA4-PA fusion protein were then processed to bind with BLPs which were prepared as described elsewhere.Citation45 Briefly, Lactococcus lactis was cultured and harvested, then dealt with hot-acid to degrade the DNA and proteins in the bacteria, followed by three times of washing with phosphate buffered saline (PBS). After suspension of the treated sample, the BLPs carrier, one kind of non-living particles, were acquired.
Preparation of the PspA-BLP pneumococcal vaccine was produced as described previously in details.Citation45 Briefly, the supernatants containing PspA2-PA or PspA4-PA proteins were mixed with BLPs respectively for 30 min at room temperature. After centrifugation, the pellets harvested from the mixture were then washed with sterile PBS in order to remove unbound proteins. Then suspended the pellets to acquire PspA2-BLP and PspA4-BLP. The amounts of PspA2-PA or PspA4-PA proteins binding to the BLPs were determined by SDS-PAGE analysis compared with BSA, and the amounts of BLPs in the PspA2-BLP and PspA4-BLP were calculated according to the optical density at 600 nm (OD600). The binding of PspA2-PA or PspA4-PA to the surface of BLPs was confirmed by immunofluorescence microscopy, in which the specific anti-PspA2 and anti-PspA4 rabbit antibodies and FITC-labeled goat anti-rabbit antibodies were used (Beijing Dingguo Changsheng Biotech). PspA2-BLP was mixed together with PspA4-BLP according to the amount of BLPs at the ratio of 1:1 to form the final PspA-BLP pneumococcal vaccine adding 0.1% thiomersal as a preservative. Prior to the further animal experiments, the prepared PspA-BLP pneumococcal vaccine was divided into single-use volume and stored at 4°C. The graphical abstract of PspA-BLP preparation was shown in .
Animals and immunization
Six-week-old female BALB/c mice were acquired from the Changchun Institute of Biological Products company and were treated carefully in accordance with institutional guidelines. The study of animal experiment was approved by the Ethical Committee of Use of Laboratory Animals in Jilin University (No.JLUAP102326). For the vaccination, BALB/c mice were lightly anesthetized and then were intranasally administered 10 μl PspA-BLP pneumococcal vaccine per mouse into the nostrils containing 0.3 mg PspA2-BLP (about 13.5 μg PspA2-PA bound to 0.3 mg BLP) and 0.3 mg PspA4-BLP (about 18.0 μg PspA4-PA bound to 0.3 mg BLP) or 10 μl BLP as a negative control group. The two groups were vaccinated three times on day 0, 14 and 28 respectively. For a positive control, the 23-valent pneumococcal polysaccharide vaccine (23-valent PPV, Merck) was included, and this group was immunized only once with 100 μl 23-valent PPV vaccine per mouse on day 0 by the subcutaneous route. Six mice per group were used in this experiment. Fourteen days after the last vaccination, blood samples were obtained by orbital puncture. Serum samples were acquired by centrifugation of blood samples and then stored the serum at −80°C until further use. Bronchoalveolar washes were obtained by flushing the bronchus with 1 ml PBS with protease inhibitor (Roche). The lavage fluid was centrifuged and then stored at −80°C until further analysis.
Analysis of antibody responses against PspA2 and PspA4 antigens using ELISA
Antibody titers were detected by indirect ELISA as described before.Citation33 First, the ELISA plates were coated with His-tag purified PspA2 or PspA4 protein prepared in Jilin University. The plates were then blocked with bovine serum albumin (BSA) and washed. Serially diluted samples were added to the ELISA plates and incubated for 45 min at 37°C, followed by washing steps. Then the ELISA plates were performed with a goat anti-mouse horseradish peroxidase (HRP)-conjugated IgG (Beijing Dingguo Changsheng Biotech) for IgG antibody detection in serum and goat anti-mouse IgA-HRP conjugate (Life Science) for IgA antibody detection in bronchoalveolar washes. Titers processed are the reciprocal of the sample dilution corresponding with an OD of over 0.1 after background correction.
Binding of PspA-specific antibody to pneumococcal strains by flow cytometry
Antibody binding analysis was performed as described before.Citation28 In brief, pneumococcal strains stored at −80°C were thawed and cultured in THY media and then harvested by centrifugation. The pellets of the bacteria were washed two times and then incubated with heat-inactivated sera at 37°C for 30 minutes. After incubation, the samples were washed and then incubated with FITC-conjugated goat anti-mouse antibody (Tianjin Sungene Biotech) for 30 minutes on ice in the dark. Then the samples were washed and suspended in 1% formaldehyde for detection. Antibody binding was tested and analyzed by flow cytometry (BD Accuri C6 cytometer, BD Biosciences).
Protection against S. pneumoniae challenge
Mice immunized with PspA-BLP, BLP negative control and 23-valent PPV positive control were anesthetized with anaesthesia machine and challenged intranasally with 40 μl S. pneumoniae containing 1.04 × 104 CFU of strain ATCC10813 (PspA family 1, clade 2) or 2.2 × 106 CFU of strain ATCC6303 (PspA family 2, clade 5). S. pneumoniae challenges were performed 2 weeks after the final immunization of PspA-BLP and BLP negative control, but 6 weeks after the first immunization of the 23-valent PPV. Ten mice per group were used in this experiment. Mortality was monitored for 14 days after pneumococcal challenge.
Statistical analysis
Statistical analysis was performed using GraphPad Prism Software (GraphPad Software, La Jolla, CA, USA). Differences in antibody titers were calculated and compared by unpaired t-tests. P values < 0.05 were considered to be statistically significant. Results were expressed as the mean ± standard error of the mean (SEM) value. Statistical analysis of the survival curves was performed by log-rank Mantel-Cox test.
Disclosure of potential conflicts of interest
No potential conflict of interest was reported by the authors.
Ethical approval
This article does not contain any studies with human participants performed by any of the authors. All the experiments were approved by the Ethical Committee of Use of Laboratory Animals in Jilin University (no.JLUAP102326) and carried out in accordance with the approved guidelines. All applicable international, national, and/or institutional guidelines for the care and use of animals were followed.
Acknowledgments
We gratefully acknowledge Phuong Thi Sarkis for editorial support in the preparation of this manuscript.
References
- Walker CL, Rudan I, Liu L, Nair H, Theodoratou E, Bhutta ZA, O’Brien KL, Campbell H, Black RE. Global burden of childhood pneumonia and diarrhoea. Lancet. 2013;381:1405–1416. doi:10.1016/S0140-6736(13)60222-6.
- Chen K, Zhang X, Shan W, Zhao G, Zhang T. Serotype distribution of Streptococcus pneumoniae and potential impact of pneumococcal conjugate vaccines in China: A systematic review and meta-analysis. Hum Vaccin Immunother. 2018;14:1453–1463. doi:10.1080/21645515.2018.1435224.
- Thorrington D, Andrews N, Stowe J, Miller E, van Hoek AJ. Elucidating the impact of the pneumococcal conjugate vaccine programme on pneumonia, sepsis and otitis media hospital admissions in England using a composite control. BMC Med. 2018;16:13. doi:10.1186/s12916-018-1004-z.
- Akata K, Chang B, Yatera K, Kawanami T, Naito K, Noguchi S, Kido T, Mukae H. The distribution and annual changes in the Streptococcus pneumoniae serotypes in adult Japanese patients with pneumococcal pneumonia from 2011 to 2015. J Infect Chemother. 2017;23:301–306. doi:10.1016/j.jiac.2017.01.008.
- Kim GL, Seon SH, Rhee DK. Pneumonia and Streptococcus pneumoniae vaccine. Arch Pharm Res. 2017;40:885–893. doi:10.1007/s12272-017-0933-y.
- Wantuch PL, Avci FY. Current status and future directions of invasive pneumococcal diseases and prophylactic approaches to control them. Hum Vaccin Immunother. 2018:1–7. doi:10.1080/21645515.2018.1470726.
- Zhou H, He J, Wu B, Che D. Cost-effectiveness analysis of routine 13-valent pneumococcal conjugate vaccinations in Chinese infants. Hum Vaccin Immunother. 2018;14:1444-1452. doi:10.1080/21645515.2018.1438794.
- Croney CM, Coats MT, Nahm MH, Briles DE, Crain MJ. PspA family distribution, unlike capsular serotype, remains unaltered following introduction of the heptavalent pneumococcal conjugate vaccine. Clin Vaccine Immunol. 2012;19:891–896. doi:10.1128/CVI.05671-11.
- Deng X, Church D, Vanderkooi OG, Low DE, Pillai DR. Streptococcus pneumoniae infection: a Canadian perspective. Expert Rev Anti Infect Ther. 2013;11:781–791. doi:10.1586/14787210.2013.814831.
- Mera R, Miller LA, Fritsche TR, Jones RN. Serotype replacement and multiple resistance in Streptococcus pneumoniae after the introduction of the conjugate pneumococcal vaccine. Microb Drug Resist. 2008;14:101–107. doi:10.1089/mdr.2008.0782.
- Darrieux M, Goulart C, Briles D, Leite LC. Current status and perspectives on protein-based pneumococcal vaccines. Crit Rev Microbiol. 2015;41:190–200. doi:10.3109/1040841X.2013.813902.
- Moffitt KL, Malley R. Next generation pneumococcal vaccines. Curr Opin Immunol. 2011;23:407–413. doi:10.1016/j.coi.2011.04.002.
- Tai SS. Streptococcus pneumoniae protein vaccine candidates: properties, activities and animal studies. Crit Rev Microbiol. 2006;32:139–153. doi:10.1080/10408410600822942.
- Hollingshead SK, Becker R, Briles DE. Diversity of PspA: mosaic genes and evidence for past recombination in Streptococcus pneumoniae. Infect Immun. 2000;68:5889–5900.
- Briles DE, Hollingshead SK, Swiatlo E, Brooks-Walter A, Szalai A, Virolainen A, McDaniel LS, Benton KA, White P, Prellner K, et al. PspA and PspC: their potential for use as pneumococcal vaccines. Microb Drug Resist. 1997;3:401–408. doi:10.1089/mdr.1997.3.401.
- Nabors GS, Braun PA, Herrmann DJ, Heise ML, Pyle DJ, Gravenstein S, Schilling M, Ferguson LM, Hollingshead SK, Briles DE, et al. Immunization of healthy adults with a single recombinant pneumococcal surface protein A (PspA) variant stimulates broadly cross-reactive antibodies to heterologous PspA molecules. Vaccine. 2000;18:1743–1754.
- Moreno AT, Oliveira ML, Ferreira DM, Ho PL, Darrieux M, Leite LC, Ferreira JMC, Pimenta FC, Andrade ALSS, Miyaji EN. Immunization of mice with single PspA fragments induces antibodies capable of mediating complement deposition on different pneumococcal strains and cross-protection. Clin Vaccine Immunol. 2010;17:439–446. doi:10.1128/CVI.00430-09.
- Brandileone MC, Andrade AL, Teles EM, Zanella RC, Yara TI, Di Fabio JL, Hollingshead SK. Typing of pneumococcal surface protein A (PspA) in Streptococcus pneumoniae isolated during epidemiological surveillance in Brazil: towards novel pneumococcal protein vaccines. Vaccine. 2004;22:3890–3896. doi:10.1016/j.vaccine.2004.04.009.
- Beall B, Gherardi G, Facklam RR, Hollingshead SK. Pneumococcal pspA sequence types of prevalent multiresistant pneumococcal strains in the United States and of internationally disseminated clones. 2000; 38:3663–3669.
- Hollingshead SK, Baril L, Ferro S, King J, Coan P, Briles DE. Pneumococcal surface protein A (PspA) family distribution among clinical isolates from adults over 50 years of age collected in seven countries. J Med Microbiol. 2006;55:215–221. doi:10.1099/jmm.0.46268-0.
- Qian J, Yao K, Xue L, Xie G, Zheng Y, Wang C, Shang Y, Wang H, Wan L, Liu L, et al. Diversity of pneumococcal surface protein A (PspA) and relation to sequence typing in Streptococcus pneumoniae causing invasive disease in Chinese children. Eur J Clin Microbiol Infect Dis. 2012;31:217–223. doi:10.1007/s10096-011-1296-9.
- Daniels CC, Briles TC, Mirza S, Hakansson AP, Briles DE. Capsule does not block antibody binding to PspA, a surface virulence protein of Streptococcus pneumoniae. Microb Pathog. 2006;40:228–233. doi:10.1016/j.micpath.2006.01.007.
- Ferreira DM, Oliveira ML, Moreno AT, Ho PL, Briles DE, Miyaji EN. Protection against nasal colonization with Streptococcus pneumoniae by parenteral immunization with a DNA vaccine encoding PspA (Pneumococcal surface protein A). Microb Pathog. 2010;48:205–213. doi:10.1016/j.micpath.2010.02.009.
- Briles DE, Hollingshead SK, Paton JC, Ades EW, Novak L, van Ginkel FW, Benjamin WH. Immunizations with pneumococcal surface protein A and pneumolysin are protective against pneumonia in a murine model of pulmonary infection with Streptococcus pneumoniae. J Infect Dis. 2003;188:339–348. doi:10.1086/376571.
- Briles DE, Hollingshead SK, King J, Swift A, Braun PA, Park MK, Ferguson LM, Nahm MH, Nabors GS. Immunization of humans with recombinant pneumococcal surface protein A (rPspA) elicits antibodies that passively protect mice from fatal infection with Streptococcus pneumoniae bearing heterologous PspA. J Infect Dis. 2000;182:1694–1701. doi:10.1086/317602.
- Goulart C, Darrieux M, Rodriguez D, Pimenta FC, Brandileone MC, de Andrade AL, Leite LCC. Selection of family 1 PspA molecules capable of inducing broad-ranging cross-reactivity by complement deposition and opsonophagocytosis by murine peritoneal cells. Vaccine. 2011;29:1634–1642. doi:10.1016/j.vaccine.2010.12.074.
- Piao Z, Akeda Y, Takeuchi D, Ishii KJ, Ubukata K, Briles DE, Tomono K, Oishi K. Protective properties of a fusion pneumococcal surface protein A (PspA) vaccine against pneumococcal challenge by five different PspA clades in mice. Vaccine. 2014;32:5607–5613. doi:10.1016/j.vaccine.2014.07.108.
- Lu J, Sun T, Wang D, Dong Y, Xu M, Hou H, Kong FT, Liang C, Gu T, Chen P, et al. Protective immune responses elicited by fusion protein containing PsaA and PspA fragments. Immunol Invest. 2015;44:482–496. doi:10.3109/08820139.2015.1037956.
- Nguyen CT, Kim SY, Kim MS, Lee SE, Rhee JH. Intranasal immunization with recombinant PspA fused with a flagellin enhances cross-protective immunity against Streptococcus pneumoniae infection in mice. Vaccine. 2011;29:5731–5739. doi:10.1016/j.vaccine.2011.05.095.
- Darrieux M, Moreno AT, Ferreira DM, Pimenta FC, de Andrade AL, Lopes AP, Leite LCC, Miyaji EN. Recognition of pneumococcal isolates by antisera raised against PspA fragments from different clades. J Med Microbiol. 2008;57:273–278. doi:10.1099/jmm.0.47661-0.
- Darrieux M, Miyaji EN, Ferreira DM, Lopes LM, Lopes AP, Ren B, Briles DE, Hollingshead SK, Leite LCC. Fusion proteins containing family 1 and family 2 PspA fragments elicit protection against Streptococcus pneumoniae that correlates with antibody-mediated enhancement of complement deposition. Infect Immun. 2007;75:5930–5938. doi:10.1128/IAI.00940-07.
- Xin W, Li Y, Mo H, Roland KL, Curtiss R 3rd. PspA family fusion proteins delivered by attenuated Salmonella enterica serovar Typhimurium extend and enhance protection against Streptococcus pneumoniae. Infect Immun. 2009;77:4518–4528. doi:10.1128/IAI.00486-09.
- Lu J, Hou H, Wang D, Leenhouts K, Roosmalen MLV, Sun T, Gu T, Song Y, Jiang C, Kong W, et al. Systemic and mucosal immune responses elicited by intranasal immunization with a pneumococcal bacterium-like particle-based vaccine displaying pneumolysin mutant Plym2. Immunol Lett. 2017;187:41–46. doi:10.1016/j.imlet.2017.05.003.
- Cutts FT, Zaman SMA, Enwere G, Jaffar S, Levine OS, Okoko JB, Oluwalana C, Vaughan A, Obaro SK, Leach A, et al. Efficacy of nine-valent pneumococcal conjugate vaccine against pneumonia and invasive pneumococcal disease in The Gambia: randomised, double-blind, placebo-controlled trial. Lancet. 2005;365:1139–1146. doi:10.1016/S0140-6736(05)71876-6.
- Neutra MR, Kozlowski PA. Mucosal vaccines: the promise and the challenge. Nat Rev Immunol. 2006;6:148–158. doi:10.1038/nri1777.
- Srivastava A, Gowda DV, Madhunapantula SV, Shinde CG, Iyer M. Mucosal vaccines: a paradigm shift in the development of mucosal adjuvants and delivery vehicles. APMIS. 2015;123:275–288. doi:10.1111/apm.12351.
- Van Braeckel-Budimir N, Haijema BJ, Leenhouts K. Bacterium-like particles for efficient immune stimulation of existing vaccines and new subunit vaccines in mucosal applications. Front Immunol. 2013;4:282. doi:10.3389/fimmu.2013.00282.
- de Haan A, Haijema BJ, Voorn P, Meijerhof T, van Roosmalen ML, Leenhouts K. Bacterium-like particles supplemented with inactivated influenza antigen induce cross-protective influenza-specific antibody responses through intranasal administration. Vaccine. 2012;30:4884–4891. doi:10.1016/j.vaccine.2012.04.032.
- Keijzer C, Haijema BJ, Meijerhof T, Voorn P, de Haan A, Leenhouts K, van Roosmalen ML, van Eden W, Broere F. Inactivated influenza vaccine adjuvanted with bacterium-like particles induce systemic and mucosal influenza A virus specific T-cell and B-cell responses after nasal administration in a TLR2 dependent fashion. Vaccine. 2014;32:2904–2910. doi:10.1016/j.vaccine.2014.02.019.
- Saluja V, Amorij JP, van Roosmalen ML, Leenhouts K, Huckriede A, Hinrichs WL, Frijlink HW. Intranasal delivery of influenza subunit vaccine formulated with GEM particles as an adjuvant. Aaps J. 2010;12:109–116. doi:10.1208/s12248-009-9168-2.
- Ramirez K, Ditamo Y, Rodriguez L, Picking WL, van Roosmalen ML, Leenhouts K, Pasetti MF. Neonatal mucosal immunization with a non-living, non-genetically modified Lactococcus lactis vaccine carrier induces systemic and local Th1-type immunity and protects against lethal bacterial infection. Mucosal Immunol. 2010;3:159–171. doi:10.1038/mi.2009.131.
- Denoel P, Philipp MT, Doyle L, Martin D, Carletti G, Poolman JT. A protein-based pneumococcal vaccine protects rhesus macaques from pneumonia after experimental infection with Streptococcus pneumoniae. Vaccine. 2011;29:5495–5501. doi:10.1016/j.vaccine.2011.05.051.
- Marriott HM, Mitchell TJ, Dockrell DH. Pneumolysin: a double-edged sword during the host-pathogen interaction. Curr Mol Med. 2008;8:497–509.
- Lu J, Sun T, Hou H, Xu M, Gu T, Dong Y, Wang D, Chen P, Wu C, Liang C, et al. Detoxified pneumolysin derivative Plym2 directly protects against pneumococcal infection via induction of inflammatory cytokines. Immunol Invest. 2014;43:717–726. doi:10.3109/08820139.2014.930478.
- van Roosmalen ML, Kanninga R, El Khattabi M, Neef J, Audouy S, Bosma T, Kuipers A, Post E, Steen A, Kok J, et al. Mucosal vaccine delivery of antigens tightly bound to an adjuvant particle made from food-grade bacteria. Methods. 2006;38:144–149. doi:10.1016/j.ymeth.2005.09.015.