ABSTRACT
Programmed death-1 (PD-1) is a cell surface receptor that functions as a T cell checkpoint and plays a central role in regulating T cell exhaustion. Binding of PD-1 to its ligand, programmed death-ligand 1 (PD-L1), activates downstream signaling pathways and inhibits T cell activation. Moreover abnormally high PD-L1 expression on tumor cells and antigen-presenting cells in the tumor microenvironment mediates tumor immune escape, and the development of anti-PD-1/PD-L1 antibodies has recently become a hot topic in cancer immunotherapy. Here, we review the structure of PD-1 and PD-L1, the function of the PD-1/PD-L1 signaling pathway, the application of PD-1 or PD-L1 monoclonal antibodies and future directions for anti-PD-1/PD-L1 antibodies with combination therapies. Cancer immunotherapy using PD-1/PD-L1 immune checkpoint blockade may require more studies, and this approach may be curative for patients with many types of cancer in the future.
KEYWORDS:
1. Introduction
Cancer is the malignant growth of tumors in the human body, and for many types of cancer, there is no cure. In previous decades, the main cancer treatment approaches were surgery, radiotherapy and chemotherapy. However, these treatments cannot distinguish between normal and abnormal cells, which causes unnecessary damage.
More than a century ago, researchers found that the immune system can target tumor cells. As knowledge of immunology increased, T cells were shown to act against tumor cells, with advantages that feature specificity, memory and adaptabilityCitation1. Furthermore, the application of antibodies that block T cell immune regulatory checkpoints is a type of immunotherapy, with breakthrough curative effectsCitation2. T cells become fully activated through a two-signal model requiring both antigen receptor signaling and CD28 costimulatory signaling. Antibody-mediated inhibition of cytotoxic T lymphocyte-associated antigen 4 (CTLA-4) was the first checkpoint protein blockade shown to be effective in cancer immunotherapy; CTLA-4 can translocate to the T cell surface and compete with CD28 for binding to CD80 and CD86, which results in inhibition of T cell proliferation and activation. Other T-cell-intrinsic checkpoints that mediate T cell inhibitory signaling include programmed cell death protein 1 (PD-1), T-cell immunoglobulin and mucin-domain containing-3 (TIM-3), and lymphocyte-activation gene-3 (LAG-3)Citation3–Citation5.
In recent years, many researchers are investigating the PD-1/programmed death-ligand 1 (PD-L1) pathway due to its remarkable clinical efficacy, durable response and low toxicity. The aim of cancer immunotherapies that target PD-1/PD-L1 signaling is to normalize the immune system rather than simply enhancing the function of immune cells in tumorCitation6. Regardless, such immune checkpoint therapy only benefits a fraction of patients, and many questions about this approach remain. This review focuses on the PD-1/PD-L1 signaling pathway and progress in its research because antibodies that regulate this pathway are the main immune checkpoint therapies clinically available and because there has been substantial progress in recent years. Accordingly, more information may be learned to provide opportunities to utilize other antitumor immunity antibodies or other methods in an effort to realize their potential for clinical applications.
2. Structure of PD-1 and PD-1 ligands
Human PD-1 (CD279), encoded by the PDCD1 gene, is a member of the immunoglobulin gene superfamily. This factor was named programmed cell death protein 1, because its expression was shown to be enhanced by apoptotic stimuli in two different cell lines (2B4.11 and LyD9t), and it participates in apoptosisCitation7. PD-1 is a type I transmembrane glycoprotein of 50–55 kD that contains a single extracellular IgV domain, a hydrophobic transmembrane domain and a cytoplasmic tail structure domain. The IgV domain consists of 20 amino acids separated from the plasma membrane and exhibits 23% homology with CTLA-4. The cytoplasmic tail contains two tyrosine motifs, an immune receptor tyrosine-based inhibitory motif (ITIM) and an immune receptor inhibitory tyrosine-based switch motif (ITSM). Studies have shown that ITSM is necessary to exert the immune suppressive function of PD-1 on active T cellsCitation8.
PD-L1 (B7-H1, CD274) and PD-L2 (B7-DC, CD273) belonging to the protein B7 family, are the ligands of PD-1Citation9,Citation10. PD-L1 and PD-L2 are type I glycoproteins containing IgV and the IgC structure domains, a hydrophobic transmembrane domain and a cytoplasmic tail structure domain. The genes encoding these ligands are both located on chromosome nine, and their sequences are highly conserved. Interaction between PD-1 and PD-L1 occurs in the tumor microenvironment. Briefly, PD-1 is highly expressed on active T cells, and the ligand, PD-L1, is expressed on some types of tumor cells and antigen presenting cells (APCs). Interaction between PD-1 and PD-L1 results in the phosphorylaton of tyrosine residues in the PD-1 cytoplasmic region of the ITSM structure domain, which causes recruitment of Src homology 2 domain-containing protein tyrosine phosphatase-2 (SHP-2). This in turn causes the downstream proteins spleen tyrosine kinase (Syk) and phospholipid inositol-3-kinase (PI3K) to become phosphorylated, which subsequently inhibits downstream signaling and T cell biological functions, including lymphocyte proliferation, cytokine secretion, and cytotoxic T lymphocyte (CTL) cytotoxicity. This interaction results in tumor-specific T cell exhaustion and apoptosis, which enables tumor cells to evade immune surveillance by T cells.
3. Expression and functions of PD-1 and PD-L1 in tumors
Similar to other inhibitory co-receptors, PD-1 is expressed on activated T cells, B cells, monocytes, dendritic cells (DCs), regulatory T cells (Tregs), and natural killer T cells (NKT) Citation11. PD-1 expression is defined as a hallmark of T cell exhaustion, which is well-defined in chronic virus infection and cancerCitation12. In many types of cancers, PD-1 is expressed on a large proportion of tumor infiltrating lymphocytes (TILs). Among CD4+ TILs, enhanced PD-1 expression is always observed on Treg cells, which may reflect their activation status, whereby the presence of actived Treg cells indicates that the tumor microenvironment (TME) is in an immunosuppressive state. For CD8+ TILs, increased PD-1 expression may reflect an anergic or exhausted T cell state, indicating a loss of CTL function. A recent study found that both mouse and human tumor associated macrophages (TAMs) express PD-1, which decreased their phagocytic capacity against tumor cells; conversely blockade of PD-1/PD-L1 increases phagocytosis and inhibits tumor growthCitation13.
PD-L1 is commonly upregulated in tumor cells, both in solid tumors and hemangiomas. PD-L1 is also expressed on T cells, B cells, macrophages, DCs, bone marrow-derived mast cells and some non-immune cellsCitation1Citation4. Type 1 and type 2 interferon can increase expression of PD-L1 on tumor cells and APCsCitation15,Citation16. In contrast, PD-L2 expression is greatly limited, as it is mainly expressed on activated macrophages and DCsCitation17. In addition to tumor cells, PD-L1 is expressed on TAMs, myeloid-derived suppressor cells (MDSCs) and DCs in the TME. Moreover, PD-L1 expression levels on TAMs have been associated with high CD4+ and CD8+ TIL levels in head and neck squamous cell carcinomaCitation18, and increased PD-L1 expression on MDSCs reportedly maintains their suppressive ability on T cell activation in colon cancerCitation19. In multiple myeloma (MM), PD-L1 is expressed on both plasma cell (PC) and DC subpopulations, and PD-L1+ PCs and CD141+ mature DCs inhibit the antitumor T cell response, which is the rationale for using anti-PD-1/PD-L1 antibodies to treat MM patientsCitation20.
The PD-1/PD-L1 pathway plays an important role in autoimmune diseases, virus infection, transplantation immunology, and tumor immunityCitation1,Citation21–Citation23. Under normal conditions, the PD-1/PD-L1 pathway induces and maintains peripheral immune tolerance and has a positive effect on preventing excessive tissue inflammation and autoimmune disease. However, with the occurrence and during the development of tumors, the combination of PD-1 and PD-L1 inhibits the host’s antitumor immunity, leading to tumor immune escape by 1) inhibiting TIL activation and inducing their apoptosis, 2) inhibiting CTL granular enzyme and perforin production, 3) decreasing the secretion of inflammatory cytokines, such as IFN-γ, IL-2, TNF-α, and promoting the secretion of the immune inhibitory cytokine IL-10, 4) stagnating the T cell cycle, leading to accumulation of cells in G0/G1 phase, and 5) promoting tumor cell epithelial materialization, tumor metastasis and infiltrationCitation24-Citation28(Summarized in ). Based on the molecular mechanisms of the PD-1/PD-L1 pathway, various types of anti-PD-1/PD-L1 antibodies have been applied to cure tumors, though this treatment has produced successful, durable, and long-lasting responses in only a fraction of patients.
Figure 1. PD-1 and PD-L1 interactions affect TIL function in the tumor microenvironment. In the tumor microenvironment, PD-L1 which can bind to PD-1, is abnormally highly expressed on tumor cells and APCs. Activation of PD-1/PD-L1 signaling can cause phosphorylation of tyrosine residues in the PD-1 cytoplasmic ITIM and ITSM structure domains, decreasing the antitumor activity of TILs by inducing apoptosis, inhibiting granular enzyme and perforin production, decreasing IFN-γ, IL-2, and TNF-α secretion, and stagnating the cell cycle. TCR: T cell receptor; MHC: major histocompatibility complex; APC: antigen presenting cell; ITIM: immune receptor tyrosine-based inhibitory motif; ITSM: immune receptor tyrosine-based switch motif; TIL: tumor-infiltrating lymphocytes. →:activation effect;“┤”inhibitory effect.
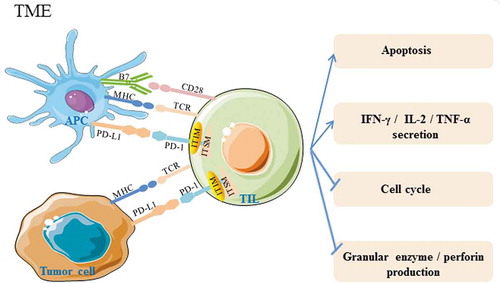
4. Clinical application of PD-1/PD-L1 blockade therapies
4.1 Anti-PD-1 antibodies
Many anti-PD-1 antibodies have been produced to date. Nivolumab (Opdivo), pembrolizumab (Keytruda) and cemiplimab (Libtayo) were approved by the FDA in September 2014, December 2014 and September 2018, respectively (). Pidilizumab, AMP-224, AMP-514 and PDR001 are still in the experimental phase of development.
Table 1. Summary of current anti-PD-1 and anti-PD-L1 antibodies approved by the FDA.
4.1.1 Nivolumab
Nivolumab (brand name: Opdivo, also known as BMS-936558 and MDX1106) is a humanized IgG4 anti-PD-1 monoclonal antibody that has a high affinity for PD-1, blocking the binding of PD-1 to its ligand PD-L1. In December 2014, the FDA accelerated the approval of nivolumab for treating unresectable or metastatic melanomaCitation29, And in March 2015, the FDA approved nivolumab to treatment of metastatic squamous non-small cell lung cancer (NSCLC)Citation30. In addition to melanoma and NSCLC, nivolumab has been demonstrated to be effective in a number of other malignancies, including, Hodgkin’s lymphomaCitation31 and hepatocellular carcinomaCitation32. The objective response rate (ORR) of nivolumab was found to be 23.7% in patients with NSCLC, with progression-free survival (PFS) of 91 daysCitation33. The overall survival (OS) of patients with Hodgkin’s lymphoma was approximately 80% at three years, and median the PFS ranged between 12 and 18 monthsCitation34.
4.1.2 Pembrolizumab
Pembrolizumab (brand name: Keytruda, also known as MK-3475 and lambrolizumab) is a humanized IgG4 kappa anti-PD-1 antibody with high affinity. In 2014, the FDA approved pembrolizumab to treat metastatic melanoma. A phase I clinical trial showed that pembrolizumab was safe and effective for the treatment of melanomaCitation35, and a phase II clinical trial showed that the curative effect of pembrolizumab for advanced melanoma was obvious when compared with that of ipilimumab (anti-CTLA-4 antibody)Citation36. The ORR of pembrolizumab in patients with advanced melanoma was reported to be 33%Citation37. In 2015, advanced NSCLC patient with no previously treatment were using pembrolizumab treatment and got the ORR as 18%Citation38. Afterwards, pembrolizumab was approved by the FDA in May 2017 for locally advanced or metastatic urothelial carcinoma. Indeed pembrolizumab improved overall survival compared with the combined performance of vinflunine, docetaxel and paclitaxel in patients with locally advanced or metastatic urothelial carcinomaCitation39. Furthermore, early clinical data showed that pembrolizumab also has great potential for the treatment of other tumors. The ORR of pembrolizumab in non-Hodgkin’s lymphoma was 53%Citation40, and in head and neck squamous cell carcinoma was found to be 19%Citation41.
4.1.3 Cemiplimab
Cemiplimab (brand name: Libtayo) is a high-affinity anti-PD-1 antibody that was the first checkpoint treatment specifically designed for advanced cutaneous squamous cell carcinoma (CSCC); it was very recently approved by the FDA. The result of a phase 1 study of cemiplimab treatment in advanced CSCC patients showed a durable response, as no disease recurrence was found more than 16 months after the treatmentCitation42. In an expansion phase I study, a 50% response was observed, and the response was durable. Moreover the results of the phase II study showed an objective response of 47%, with adverse events that were similar to other PD-1 inhibitorsCitation43.
4.2 Anti-PD-l1 antibodies
Several anti-PD-L1 monoclonal antibodies are commercially available. Atezolizumab, avelumab and duravulumab were approved by the FDA in September 2014, May 2016, and May 2017, respectively (). BMS-936559 and CK-301 are still in the experimental phase of development.
4.2.1 Atezolizumab
Atezolizumab (brand name: Tecentriq, also known as MPDL3280A) is a phage-derived human IgG1 monoclonal antibody with an Fc fragment. Atezolizumab blocks PD-L1 on the surface of tumors and exhibits promising antitumor activity. As the Fc fragment of atezolizumab was modified by gene engineering, the antibody-dependent cell-mediated cytotoxicity (ADCC) effect can be avoidedCitation44. In May 2016, the FDA approved atezolizumab as the first PD-L1 inhibitor for urothelial carcinoma. In addition, this drug has been shown to have significant curative effects in a wide variety of cancers, such as kidney cancer, bladder transitional cell carcinoma, and breast cancer. In the first stage, the safety and validity of 62 patients with renal cell carcinoma were evaluatedCitation45; the ORR of atezolizumab in the treatment of metastatic bladder transitional cell carcinoma and breast cancer was 26% and 10%, respectivelyCitation46,Citation47.
4.2.2 Avelumab
Avelumab (brand name: Bavencio, also known as MSB0010718C) is a fully human anti-PD-L1 IgG1 monoclonal antibody; the drug was announced by Merck and Pfizer in November 2014. By blocking PD-L1, avelumab may reactivate T cells and induce ADCC with its native Fc region. Avelumab also demonstrated an objective response of 62.1% with metastatic Merkel cell carcinomaCitation48. Moreover, the ORR of advanced NSCLC was 12%Citation49.
4.2.3 Duravulumab
Duravulumab (brand name: Imfinzi) is a humanized monoclonal antibody that directly targets PD-L1, blocking PD-L1 and PD-1 interaction on T cells to prevent tumor immune escape and enhance immune responses. An ORR for duravulumab of 9.2% in the treatment of head and neck squamous cell carcinoma (HNSCC) has been reported. In addition, the 6-month progression-free survival rate was 20% in all HNSCC patients, and reached 25% in PD-L1 positive patientsCitation50. The ORR in NSCLC was 66.3% in the durvalumab groupCitation51.
5. New insights regarding PD-1/PD-L1 blockade for cancer treatment
5.1 Predictive biomarkers
When using anti-PD-1 or PD-L1 antibodies to treat cancer, some patients with low PD-L1 expression might be poor responders. Therefore, to personalize treatments and obtain an optimal treatment effect, biomarkers need to be identified. In patients with thymic epithelial tumor, those with a PD-1-positive microenvironment had a shorter mean estimated survival time than did negative controlCitation52, and in ovarian cancer and hepatocellular carcinoma, patients with high PD-L1 expression had significantly worse outcomes than did patients who had low or lacked PD-L1 expressionCitation53,Citation54. Moreover, increased soluble PD-L1 (sPD-L1) has been associated with a low 3-year overall survival rate in patients with diffuse large B-cell lymphoma (DLBCL)Citation55.
Although the FDA has approved a PD-L1 immunohistochemistry (IHC) test as a companion diagnostic for anti-PD-1 antibody treatment in advanced NSCLC, the expression pattern of PD-1 and PD-L1 is not a good predictive biomarker for diagnosing all cancer types because of the following reasons: 1) biopsy specimens from patients may not accurately identify PD-L1 expression; 2) PD-L1 expression levels might differ among patients; 3) biopsy collection times might be too long or involve poor storage conditions, and thus PD-L1 levels might not represent the real PD-L1 status; 4) inappropriate tissue handling or IHC techniques for assessing PD-L1 expression might lead to PD-L1 epitope degradation before fixation; 5) not all antibodies used for PD-L1 are suitable; 6) only membrane PD-L1 is functional, as it can interact with PD-1 on T cells, and it is unclear whether the amount of cytoplasmic PD-1 expression is associated with cancer progression; and 7) PD-L1 can be expressed by different cells in the TME, and it is also remain unclear whether its expression on different cells is related to cancer progressionCitation1Citation4,Citation56. Given the complexity of T cell regulation, which can be controlled by multiple signaling pathways, other components of the TME may be useful as predictive biomarkers together with PD-1/PD-L1 expression to help doctors select patients for optimal treatment.
5.2 Combination therapy
Currently, an increasing number of researchers are realizing that responses to anti-PD-1/PD-L1 antibody treatment and other immunotherapies are dependent on the TME, which can be distinguished as an immunogenic (hot) TME or a non-immunogenic (cold) TMECitation1. Large amounts of TILs and cytokines and high PD-L1 expression occur in hot tumors, whereas cold tumors show almost no PD-L1 expression and no T-cell infiltration. Therefore, some of the reasons why almost combination treatments with anti-PD-1/PD-L1 antibodies may provide better clinical results are as follows: 1) it will create a hot TME, for example, increasing PD-L1 expression or increasing TIL infiltration, which can enhance antitumor activity; 2) it will target other cell types in the TME, such as MDSCs, and provide an additive effect to the entire microenvironment; or 3) it may reduce the dosage or treatment time when compared with a single treatment, which can decrease the side effects of drugs. Overall, there are many combination therapy methods (), and these combination therapies have synergistic effects, which can improve the efficacy of therapies in a variety of cancers ().
Table 2. Combination therapies of anti-PD-1 antibodies in clinical trials.
Figure 2. Combination therapies with anti-PD-1/PD-L1 antibodies. Because of the complexity of the tumor microenvironment and the complexity of checkpoint regulation in TILs, only partially successful clinical results of PD-1/PD-L1 blockade have been achieved in certain tumors. Therefore, combination therapies are required. Ongoing studies, such as using PD-1/PD-L1 antibodies combined with other checkpoint antibodies, tumor vaccines, antiviral drugs, anti-microbiome modulation, chemotherapy and radiation therapies, or kinase or enzyme inhibitors are being developed. Combination therapies will be a future direction for cancer patient treatment.
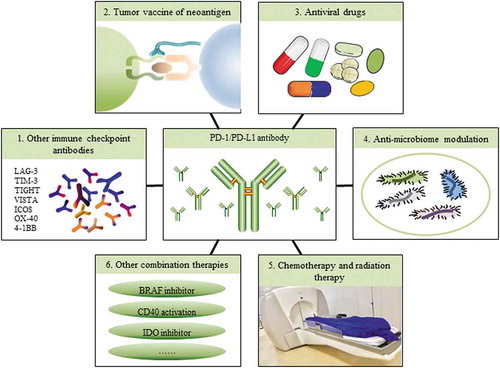
5.2.1 Combination with other immune checkpoint antibodies
CTLA-4 and PD-1 inhibit T cell activation in different waysCitation57. The first patient to use an immune checkpoint combination was treated with anti-PD-1 and CTLA-4 antibodies in 2009Citation2. In metastatic melanoma patients, the response rate for PD-1 and CTLA-4 blockade combination therapy was approximately 60% in phase II and phase III trials when compared with PD-1 blockade alone. Notably, a slightly higher 3-year survival rate was observed, but a higher frequency of toxicity was also foundCitation2. During 2018, three papers were published on lung cancer treatment with PD-1 plus CTLA-4 blockade. Showing a greater clinical benefit in high-tumor mutational burden patients when compared with low-tumor mutational burden patients, including improved objective responses, durable benefits, and progression-free survival ratesCitation58-Citation60. Therefore, the tumor mutational burden may be useful as a genomic determinant to identify patients who may benefit from PD-1 and CTLA-4 blockade combination immunotherapy.
Additional clinical trials and pre-clinical tumor model experiments evaluating other checkpoint proteins combined with anti-PD-1/PD-L1 antibodies are also being conducted. LAG-3, TIM-3, T cell immune receptor with Ig and ITIM domains (TIGIT), and V-domain Ig suppressor of T-cell activation (VISTA) are all checkpoint proteins in T cells. An investigational study using anti-LAG-3 with and without anti-PD-1 antibodies is currently recruiting patients to test its safety, tolerability and effectiveness for the treatment of solid tumors (NCT01968109, http://www.clinicaltrials.gov). Of note targeting TIM-3, TIGIT and VISTA combined with PD-1 pathway targeting improved antitumor immune responsesCitation61-Citation63. Inducible T cell costimulator (ICOS), TNF receptor superfamily member 4 (OX-40), and TNF receptor superfamily member 9 (4-1BB) are co-stimulatory molecules in T cells, and antibodies against these molecules combined with anti-PD-1/PD-L1 antibodies resulted in an enhanced immune response in tumor models (NCT02904226, http://www.clinicaltrials.gov)Citation64,Citation65. The use of low-dose combination checkpoint inhibition also appears to be an optimal approach for enhancing clinical benefits.
Unfortunately, patients may develop dermatitis, colitis, hepatitis, pancreatitis, pneumonitis and hypophysis after anti-CTLA-4 or anti-PD-1/PD-L1 antibody treatment. In fact, checkpoint combination therapies have notably high toxicity and side effects. For example, most melanoma patients experience immune-related adverse events (irAEs) during anti-PD-1/PD-L1 and anti-CTLA-4 antibody combination therapyCitation66. Therefore, further studies investigating the mechanisms of these checkpoint therapies may be required to ameliorate these toxicities.
5.2.2 Combination with neoantigen tumor vaccines
Several studies have shown that genomic information, especially a high mutation burden, helps to determine the response of PD-1/PD-L1 blockade. As it has many mutations that can be recognized as neoantigens, melanoma is a well-known hot tumor, and checkpoint immunotherapy has remarkable clinical effects. Whole-exome sequencing has been applied in NSCLC and mutations in its genomic landscape helped to determine response to anti-PD-1 antibodiesCitation67. In colon cancer, tumors with mismatch repair deficiency are highly sensitive to anti-PD-1 antibody treatment, and this deficiency indicate many mutant neoantigensCitation68, which are created by tumor cells because of mutations, and can be recognized by T cells. Although tumor vaccines can be prepared from cancer cells, parts of cancer cells, or pure tumor antigens, they always contain neoantigens from a tumor. However, questions regarding tumor vaccines from neoantigens have been raised, such as 1) How can neoantigen prediction be optimized?2) What is a good time for vaccine acquisition?3) What is the optimal burden and vaccine injection schedule?4) How do we select patients who will benefit most?Citation69,Citation70 Among melanoma patients using vaccines that targeted personal tumor neoantigens, those who had recurrent disease were then treated with anti-PD-1 therapy, and complete tumor regression and neoantigen-specific T cell expansion were found after this treatmentCitation71. These studies provide strong rationale for the further development of this combination approach, though more clinical tests and comprehensive studies identifying mechanisms are required.
5.2.3 Combination with antiviral drugs
Some human cancers are driven by oncogenes from integrated viruses, such as EBV in Burkitt’s lymphoma, Hodgkin’s lymphoma, gastric carcinoma, HPV in cervical cancer and head and neck cancer, HBV and HCV in hepatocellular carcinoma, and Merkel cell polyomavirus (MCPyV) in Merkel cell carcinoma (MCC) and chronic lymphocytic leukemia (CLL). No clinical trial data for PD-1/PD-L1 blockade combined with antiviral drugs are yet available; however, some preliminary studies indicate promise. MCC is a skin cancer, among which approximately 80% are associated with MCPyV, and high ORRs, prolonged durable responses, and good tolerability were found when using anti-PD-1 therapyCitation48. In addition, IHC and cytometry by time-of-flight (CyTOF) have been applied to examine the immune microenvironments in HBV-associated hepatocellular carcinomas, as more PD-1+ Tregs were found in HBV-related HCC than in non-viral-related HCCCitation72.
5.2.4 Combination with anti-microbiome modulation
In addition to the tumor itself, host factors such as the gastrointestinal microbiome can influence responses to checkpoint immunotherapy. Published in 2018, three notable studies have shown a relationship between healthy gut flora and anti-PD-1 treatment. The authors reported that in melanoma and epithelial cancer, “good” or “favorable” gut bacteria were required for a patient to respond to PD-1 blockade treatment and that antibiotic use could inhibit clinical benefits. Therefore, for anti-PD-1/PD-L1 antibody treatment, therapeutic responses of tumor patients may be improved through host gastrointestinal microbiome modulationCitation73-Citation75.
5.2.5 Combination with chemotherapy and radiation therapy
Chemotherapy kills tumor cells by inducing DNA damage, cell cycle arrest and ultimately apoptosis, whereas radiation therapy activates the type I IFN pathway in DCs to effectively prime tumor-specific T cells. After more mechanistic studies, it appears that these methods may help turn cold TME into hot TME, as they can create neoantigens of tumors during treatment. Therefore, the response has been encouraging when these two methods have been combined with anti-PD-1/PD-L1 antibodies. In NSCLC, promising results were shown in combination chemotherapy with anti-PD-1/PD-L1 antibodies, including a high overall response and long overall survival but not fewer irAEsCitation76. In metastatic melanoma, major tumor regression occurred when radiation and PD-L1 and CTLA-4 dual checkpoint blockade was usedCitation77. Although these antitumor results are remarkable, a considerable amount of work regarding the dosage, time and sequence is still needed in future clinical trials.
5.2.6 Other combination therapies
There are still many other combination therapies that are under development for application, and most are being tested in melanoma patients. For instance, a BRAF inhibitor used for the treatment of melanoma was combined with anti-PD-1 and anti-CTLA-4 antibodies, and the results showed that BRAF inhibitor therapy may affect subsequent clinical responses to checkpoint immunotherapyCitation78. CD40 activation can upregulate APC function, and convert cold tumors into hot tumors. In a pancreatic carcinoma mouse model treated with anti-PD-1 antibodies combined with anti-CD40/chemotherapy, the activity and durability of the response were extended when compared with anti-CD40/chemotherapy treatment aloneCitation79. Recently, IDO inhibitor and PD-1 antibody combination therapy exhibited a good antitumor response in hepatocellular carcinoma because checkpoint immunotherapy increased IDO induction, which can cause adaptive resistance in some patientsCitation80. In another phase 3 trial in advanced melanoma, the same IDO inhibitor plus an anti-PD-1 antibody had no increased benefit compared to the use of the anti-PD-1 antibody alone, indicating that combination therapy may not be necessary at all times; in particular no evidence to date from extensive trials supports the use of combination therapyCitation81.
6. Conclusion/expert opinion
Recently, cancer immunotherapy targeting PD-1 or PD-L1 has proven effective in causing durable antitumor immune responses with less toxicity in many types of tumors, We believe that PD-1/PD-L1 blockade therapy will be the major cancer immunotherapy method in the next few years, even though there is still much to be learned about this signaling pathway. Key questions remaining to be resolved include: 1) How to select PD-1/PD-L1-positive patient groups? What are their features of these patiens, and what efficient clinical detection method should be used? 2) How can the abundance of tumor infiltrating CD8+T cell be increased in TME? Especially the particular CD8+T cell that display tumor-reactive intratumoral TCR repertoires, but not bystander CD8+TILs that can not kill the tumor cell. 3) What is the mechanism by which PD-1 regulats on CTLs and Tregs, and what is the mechanism by which PD-L1 acts on tumor cells and APC cells in TME? Can we find more efficient inhibitors based on the mechanism? 4) Do we have any good treatment for PD-1/PD-L1- negative patients? Can other therapies or combination therapies with an anti-PD antibody approach be optimal for these patients? With a deeper understanding of personal genomic information, personalized markers in guiding anti-PD therapy alone or with other targets will be critical to achieve clinical results of such therapies, and more work needs to be performed to achieve clarity regarding these key questions. Similar to the tip of an iceberg, PD-1/PD-L1 blockade antitumor immunotherapy opens a new era of cancer treatment, and further work on safety and efficiency will be needed.
Disclosure of potential conflicts of interest
The authors have declared that no competing interest exists.
Additional information
Funding
References
- Sharma P, Allison JP. The future of immune checkpoint therapy. Science. 2015;348:56. doi:10.1126/science.aaa5139.
- Ribas A, Wolchok JD. Cancer immunotherapy using checkpoint blockade. Science. 2018;359:1350–55. doi:10.1126/science.aar4060.
- Ahmadzadeh M, Johnson LA, Heemskerk B, Wunderlich JR, Dudley ME, White DE, Rosenberg SA. Tumor antigen-specific CD8 T cells infiltrating the tumor express high levels of PD-1 and are functionally impaired. Blood. 2009;114:1537–44. doi:10.1182/blood-2008-12-195792.
- Fourcade J, Sun Z, Benallaoua M, Guillaume P, Luescher IF, Sander C, Kirkwood JM, Kuchroo V, Zarour HM. Upregulation of Tim-3 and PD-1 expression is associated with tumor antigen-specific CD8+ T cell dysfunction in melanoma patients. J Exp Med. 2010;207:2175–86. doi:10.1084/jem.20100637.
- Wang J, Sanmamed MF, Datar I, Su TT, Ji L, Sun J, Chen L, Chen Y, Zhu G, Yin W, et al. Fibrinogen-like protein 1 is a major immune inhibitory ligand of LAG-3. Cell. 2019;176:334–47.e12. doi10.1016/j.cell.2018.11.010.
- Sanmamed MF, Chen L. A paradigm shift in cancer immunotherapy: from enhancement to normalization. Cell. 2018;175:313–26. doi:10.1016/j.cell.2018.09.035.
- Ishida Y, Agata Y, Shibahara K, Honjo T. Induced expression of PD-1, a novel member of the immunoglobulin gene superfamily, upon programmed cell death. Embo J. 1992;11:3887–95.
- Chemnitz JM, Parry RV, Nichols KE, June CH, Riley JL. SHP-1 and SHP-2 associate with immunoreceptor tyrosine-based switch motif of programmed death 1 upon primary human T cell stimulation, but only receptor ligation prevents T cell activation. J Immunol. 2004;173:945–54. doi:10.4049/jimmunol.173.2.945.
- Freeman GJ, Long AJ, Iwai Y, Bourque K, Chernova T, Nishimura H, Fitz LJ, Malenkovich N, Okazaki T, Byrne MC, et al. Engagement of the PD-1 immunoinhibitory receptor by a novel B7 family member leads to negative regulation of lymphocyte activation. J Exp Med. 2000;192:1027–34.
- Latchman Y, Wood CR, Chernova T, Chaudhary D, Borde M, Chernova I, Iwai Y, Long AJ, Brown JA, Nunes R, et al. PD-L2 is a second ligand for PD-1 and inhibits T cell activation. Nat Immunol. 2001;2:261–68. doi:10.1038/85330.
- Keir ME, Butte MJ, Freeman GJ, Sharpe AH. PD-1 and its ligands in tolerance and immunity. Annu Rev Immunol. 2008;26:677–704. doi:10.1146/annurev.immunol.26.021607.090331.
- Pauken KE, Whery EJ. Overcoming T cell exhaustion in infection and cancer. Trends Immunol. 2015;36:265–76. doi:10.1016/j.it.2015.02.008.
- Gordon SR, Maute RL, Dulken BW, Hutter G, George BM, Mccracken MN, Gupta R, Tsai JM, Sinha R, Corey D, et al. PD-1 expression by tumour-associated macrophages inhibits phagocytosis and tumour immunity. Nature. 2017;545:495. doi:10.1038/nature22396.
- Patel SP, Kurzrock R. PD-L1 expression as a predictive biomarker in cancer immunotherapy. Mol Cancer Ther. 2015;14:847–56. doi:10.1158/1535-7163.MCT-14-0983.
- Eppihimer MJ, Gunn J, Freeman GJ, Greenfield EA, Chernova T, Erickson J, Leonard J. Expression and regulation of the PD-L1 immunoinhibitory molecule on microvascular endothelial cells. Microcirculation. 2002;9:133. doi:10.1080/713774061.
- Schreiner B, Mitsdoerffer M, Kieseier BC, Chen L, Hartung HP, Weller M, Wiendl H. Interferon-beta enhances monocyte and dendritic cell expression of B7-H1 (PD-L1), a strong inhibitor of autologous T-cell activation: relevance for the immune modulatory effect in multiple sclerosis. J Neuroimmunol. 2004;155:172. doi:10.1016/j.jneuroim.2004.06.013.
- Yearley JH, Gibson C, Yu N, Moon C, Murphy E, Juco J, Lunceford J, Cheng J, Chow LQM, Seiwert TY, et al. PD-L2 expression in human tumors: relevance to anti-PD-1 therapy in cancer. Clinl Cancer Res. 2017;23:3158–67. doi:10.1158/1078-0432.CCR-16-1761.
- Mattox AK, Lee J, Westra WH, Pierce RH, Ghossein R, Faquin WC, Diefenbach TJ, Morris LG, Lin DT, Wirth LJ, et al. PD-1 expression in head and neck squamous cell carcinomas derives primarily from functionally anergic CD4(+) TILs in the presence of PD-L1(+) TAMs. Cancer Res. 2017;77:6365–74. doi:10.1158/0008-5472.CAN-16-3453.
- Lu C, Redd PS, Lee JR, Savage N, Liu K. The expression profiles and regulation of PD-L1 in tumor-induced myeloid-derived suppressor cells. Oncoimmunology. 2016;5:e1247135. doi:10.1080/2162402X.2016.1247135.
- Sponaas AM, Moharrami NN, Feyzi E, Standal T, Holth Rustad E, Waage A, Sundan A, Waisman A. PDL1 expression on plasma and dendritic cells in myeloma bone marrow suggests benefit of targeted anti PD1-PDL1 therapy. PLoS One. 2015;10:e0139867. doi:10.1371/journal.pone.0139867.
- Dai S, Jia R, Zhang X, Fang Q, Huang L. The PD-1/PD-Ls pathway and autoimmune diseases. Cell Immunol. 2014;290:72–79. doi:10.1016/j.cellimm.2014.05.006.
- McNally B, Ye F, Willette M, Flano E. Local blockade of epithelial PDL-1 in the airways enhances T cell function and viral clearance during influenza virus infection. J Virol. 2013;87:12916–24. doi:10.1128/JVI.02423-13.
- Lu PY, Youngblood BA, Austin JW, Mohammed AUR, Butler R, Ahmed R, Boss JM. Blimp-1 represses CD8 T cell expression of PD-1 using a feed-forward transcriptional circuit during acute viral infection. J Exp Med. 2014;211:515–27. doi:10.1084/jem.20130208.
- Shi F, Shi M, Zeng Z, Qi RZ, Liu ZW, Zhang JY, Yang YP, Tien P, Wang FS. PD-1 and PD-L1 upregulation promotes CD8+ T cell apoptosis and postoperative recurrence in hepatocellular carcinoma patients. Int JCancer. 2011;128:887. doi:10.1002/ijc.25577.
- Rollins MR, Gibbons Johnson RM. CD80 expressed by CD8+ T cells contributes to PD-L1-induced apoptosis of activated CD8+ T cells. J Immunol Res. 2017;2017:1–6. doi:10.1155/2017/7659462.
- Patsoukis N, Sari D, Boussiotis VA. PD-1 inhibits T cell proliferation by upregulating p27 and p15 and suppressing Cdc25A. Cell Cycle. 2012;11:4305–09. doi:10.4161/cc.22135.
- Mansfield AS, Aubry MC, Moser J, Harrington S, Dronca RS, Park SS, Dong H. Temporal and spatial discordance of programmed cell death-ligand 1 expression and lymphocyte tumor infiltration between paired primary lesions and brain metastases in lung cancer. Ann Oncol. 2016;27:1953–58. doi:10.1093/annonc/mdw289.
- Velcheti V, Rimm DL, Schalper KA. Sarcomatoid lung carcinomas show high levels of programmed death ligand-1 (PD-L1). J Thorac Oncol. 2013;8:803–05. doi:10.1097/JTO.0b013e318292be18.
- Robert C, Long GV, Brady B, Dutriaux C, Maio M, Mortier L, Hassel JC, Rutkowski P, McNeil C, Kalinka-Warzocha E, et al. Nivolumab in previously untreated melanoma without BRAF mutation. N Engl J Med. 2015;372:320–30. doi:10.1056/NEJMoa1412082.
- Brahmer J, Reckamp KL, Baas P, Crinò L, Eberhardt WEE, Poddubskaya E, Antonia S, Pluzanski A, Vokes EE, Holgado E, et al. Nivolumab versus docetaxel in advanced squamous-cell non–small-cell lung cancer. N Engl J Med. 2015;373:1627. doi:10.1056/NEJMoa1504627.
- Armand P, Engert A, Younes A, Fanale M, Santoro A, Zinzani PL, Timmerman JM, Collins GP, Ramchandren R, Cohen JB, et al. Nivolumab for relapsed/refractory classic hodgkin lymphoma after failure of autologous hematopoietic cell transplantation: extended follow-up of the multicohort single-arm phase II CheckMate 205 trial. J Clin Oncol. doi:10.1200/JCO.2017.76.0793
- El-Khoueiry AB, Sangro B, Yau T, Crocenzi TS, Kudo M, Hsu C, Kim T-Y, Choo S-P, Trojan J, Welling TH, et al. Nivolumab in patients with advanced hepatocellular carcinoma (CheckMate 040): an open-label, non-comparative, phase 1/2 dose escalation and expansion trial. Lancet. 2017;389:2492. doi:10.1016/S0140-6736(17)31046-2.
- Sato K, Akamatsu H, Murakami E, Sasaki S, Kanai K, Hayata A, Tokudome N, Akamatsu K, Koh Y, Ueda H, et al. Correlation between immune-related adverse events and efficacy in non-small cell lung cancer treated with nivolumab. Lung Cancer. 2018;115:71–74. doi:10.1016/j.lungcan.2017.11.019.
- Goldkuhle M, Dimaki M, Gartlehner G, Monsef I, Skoetz N. Nivolumab for adults with Hodgkin’s lymphoma (a rapid review using the software RobotReviewer). Cochrane Database Syst Rev. 2018; doi:10.1002/14651858.CD012556.
- Hamid O, Robert C, Daud A, Hodi FS, Hwu WJ, Kefford R, Wolchok JD, Hersey P, Joseph RW, Weber JS, et al. Safety and tumor responses with lambrolizumab (Anti–PD-1) in melanoma. N Engl J Med. 2013;369:134–44. doi:10.1056/NEJMoa1305133.
- Robert C, Schachter J, Long GV, Arance A, Grob JJ, Mortier L, Daud A, Carlino MS, McNeil C, Lotem M, et al. Pembrolizumab versus ipilimumab in advanced melanoma. N Engl J Med. 2015;372:2521–32. doi:10.1056/NEJMoa1503093.
- Ribas A, Hamid O, Daud A, Hodi FS, Wolchok JD, Kefford R, Joshua AM, Patnaik A, Hwu W-J, Weber JS, et al. Association of pembrolizumab with tumor response and survival among patients with advanced melanoma. J Am Med Assoc. 2016;315:1600. doi:10.1001/jama.2016.4059.
- Garon EB, Rizvi NA, Hui R, Leighl N, Balmanoukian AS, Eder JP, Patnaik A, Aggarwal C, Gubens M, Horn L, et al. Pembrolizumab for the treatment of non-small-cell lung cancer. N Engl J Med. 2015;372:2018–28. doi:10.1056/NEJMoa1501824.
- Gallacher D, Armoiry X, Auguste P, Court R, Mantopoulos T, Patterson J, De Santis M, Cresswell J, Mistry H. Pembrolizumab for previously treated advanced or metastatic urothelial cancer: an evidence review group perspective of a NICE single technology appraisal. Pharmacoeconomics. 2019;37:19–27. doi:10.1007/s40273-018-0689-3.
- Moskowitz CH, Ribrag V, Michot JM. PD-1 blockade with the monoclonal antibody pembrolizumab (MK-3475) in patients with classical hodgkin lymphoma after brentuximab vedotin failure: preliminary results from a phase 1b study (KEYNOTE-013). Blood. 2014;124:290.
- Tahara M, Muro K, Hasegawa Y, Chung HC, Lin CC, Bhumsuk Keam, Takahashi K, Cheng JD, Bang YJ. Pembrolizumab in Asia‐Pacific patients with advanced head and neck squamous cell carcinoma: analyses from KEYNOTE‐012. Cancer Sci. 2018;109:771–6. doi:10.1111/cas.13480.
- Falchook GS, Leidner R, Stankevich E, Piening B, Bifulco C, Lowy I, Fury MG. Responses of metastatic basal cell and cutaneous squamous cell carcinomas to anti-PD1 monoclonal antibody REGN2810. J ImmunoTher Cancer. 2016;4:70. doi:10.1186/s40425-016-0176-3.
- Migden MR, Rischin D, Schmults CD, Guminski A, Hauschild A, Lewis KD, Chung CH, Hernandez-Aya L, Lim AM, Chang AL, Rabinowits G. PD-1 blockade with cemiplimab in advanced cutaneous squamous-cell carcinoma. N Engl J Med. 2018;379:341–51. doi:10.1056/NEJMoa1805131.
- Powles T, Eder JP, Fine GD, Braiteh FS, Loriot Y, Cruz C, Bellmunt J, Burris HA, Petrylak DP, Teng S-L, et al. MPDL3280A (anti-PD-L1) treatment leads to clinical activity in metastatic bladder cancer. Nature. 2014;515:558. doi:10.1038/nature13904.
- McDermott DF, Sosman JA, Sznol M, Massard C, Gordon MS, Hamid O, Powderly JD, Infante JR, Fassò M, Wang YV, et al. Atezolizumab, an anti-programmed death-ligand 1 antibody, in metastatic renal cell carcinoma: long-term safety, clinical activity, and immune correlates from a phase Ia study. J clin oncol. 2016;34:833–42. doi:10.1200/JCO.2015.63.7421.
- Bernard-Tessier A, Bonnet C, Lavaud P, Gizzi M, Loriot Y, Massard C. [Atezolizumab (Tecentriq®): activity, indication and modality of use in advanced or metastatic urinary bladder carcinoma]. Bull Cancer. 2018;105:140. doi:10.1016/j.bulcan.2017.10.030.
- American Association for Cancer Research. Atezolizumab extends survival for breast cancer. Cancer Discov. 2017; 7: OF10. doi:10.1158/2159-8290.CD-NB2017-053.
- D’Angelo SP, Russell J, Lebbe C, Chmielowski B, Gambichler T, Grob JJ, Kiecker F, Rabinowits G, Terheyden P, Zwiener I, Bajars M. Efficacy and safety of first-line avelumab treatment in patients with stage IV metastatic merkel cell carcinoma: a preplanned interim analysis of a clinical trial. JAMA oncol. 2018;4:e180077. doi:10.1001/jamaoncol.2017.4473.
- Gulley JL, Rajan A, Spigel DR, Iannotti N, Chandler J, Wong DJL, Leach J, Edenfield WJ, Wang D, Grote HJ, et al. Avelumab for patients with previously treated metastatic or recurrent non-small-cell lung cancer (JAVELIN solid tumor): dose-expansion cohort of a multicentre, open-label, phase 1b trial. Lancet Oncol. 2017;18:599–610. doi:10.1016/S1470-2045(17)30240-1.
- Siu LL, Even C, Mesia R, Remenar E, Daste A, Delord JP, Krauss J, Saba NF, Nabell L, Ready NE, et al. Safety and efficacy of durvalumab with or without tremelimumab in patients with PD-L1-low/negative recurrent or metastatic HNSCC: the phase 2 CONDOR randomized clinical trial. JAMA oncol. 2018. doi:10.1001/jamaoncol.2018.4628.
- Antonia SJ, Villegas A, Daniel D, Vicente D, Murakami S, Hui R, Kurata T, Chiappori A, Lee KH, de Wit M, et al. Overall survival with durvalumab after chemoradiotherapy in stage III NSCLC. N Engl J Med. 2018;379:2342–50. doi:10.1056/NEJMoa1809697.
- Bagir EK, Acikalin A, Avci A, Gumurdulu D, Paydas S. PD-1 and PD-L1 expression in thymic epithelial tumours and non-neoplastic thymus. J Clin Pathol. 2018. doi:10.1136/jclinpath-2017-204788.
- Hamanishi J, Mandai M, Iwasaki M, Okazaki T, Tanaka Y, Yamaguchi K, Higuchi T, Yagi H, Takakura K, Minato N, et al. Programmed cell death 1 ligand 1 and tumor-infiltrating CD8+ T lymphocytes are prognostic factors of human ovarian cancer. Proc Natl Acad Sci U S A. 2007;104:3360–65. doi:10.1073/pnas.0611533104.
- Hu K, Wang ZM, Li JN, Zhang S, Xiao ZF, Tao YM. CLEC1B expression and PD-L1 expression predict clinical outcome in hepatocellular carcinoma with tumor hemorrhage. Transl Oncol. 2018;11:552–58. doi:10.1016/j.tranon.2018.02.010.
- Rossille D, Gressier M, Damotte D, Maucort-Boulch D, Pangault C, Semana G, Le Gouill S, Haioun C, Tarte K, Lamy T, et al. High level of soluble programmed cell death ligand 1 in blood impacts overall survival in aggressive diffuse large B-Cell lymphoma: results from a French multicenter clinical trial. Leukemia. 2014;28:2367–75. doi:10.1038/leu.2014.137.
- Topalian SL, Taube JM, Anders RA, Pardoll DM. Mechanism-driven biomarkers to guide immune checkpoint blockade in cancer therapy. Nat Rev Cancer. 2016;16:275–87. doi:10.1038/nrc.2016.36.
- Wei SC, Levine JH, Cogdill AP, Zhao Y, Anang NAS, Andrews MC, Sharma P, Wang J, Wargo JA, Pe’er D, et al. Distinct cellular mechanisms underlie anti-CTLA-4 and Anti-PD-1 checkpoint blockade. Cell. 2017;170:1120–33 e17. doi:10.1016/j.cell.2017.07.024.
- Hellmann MD, Ciuleanu TE, Pluzanski A, Lee JS, Otterson GA, Audigier-Valette C, Minenza E, Linardou H, Burgers S, Salman P, Borghaei H. Nivolumab plus ipilimumab in lung cancer with a high tumor mutational burden. N Engl J Med. 2018;378:2093–104. doi:10.1056/NEJMoa1801946.
- Hellmann MD, Callahan MK, Awad MM, Calvo E, Ascierto PA, Atmaca A, Rizvi NA, Hirsch FR, Selvaggi G, Szustakowski JD, Sasson A. Tumor mutational burden and efficacy of nivolumab monotherapy and in combination with ipilimumab in small-cell lung cancer. Cancer Cell. 2018;33:853–61. e4. doi:10.1016/j.ccell.2018.04.001.
- Hellmann MD, Nathanson T, Rizvi H, Creelan BC, Sanchez-Vega F, Ahuja A, Ni A, Novik JB, Mangarin LMB, Abu-Akeel M, et al. Genomic features of response to combination immunotherapy in patients with advanced non-small-cell lung cancer. Cancer Cell. 2018;33. doi:10.1016/j.ccell.2018.03.018.
- Sakuishi K, Apetoh L, Sullivan JM, Blazar BR, Kuchroo VK, Anderson AC. Targeting Tim-3 and PD-1 pathways to reverse T cell exhaustion and restore anti-tumor immunity. J Exp Med. 2010;207:2187–94. doi:10.1084/jem.20100643.
- Hung AL, Maxwell R, Theodros D, Belcaid Z, Mathios D, Luksik AS, Kim E, Wu A, Xia Y, Garzon-Muvdi T, et al. TIGIT and PD-1 dual checkpoint blockade enhances antitumor immunity and survival in GBM. Oncoimmunology. 2018;e1466769. doi:10.1080/2162402X.2018.1466769.
- Le MI, Chen W, Lines JL, Day M, Li J, Sergent P, Noelle RJ, Wang L. VISTA regulates the development of protective antitumor immunity. Cancer Res. 2014;74:1933–44. doi:10.1158/0008-5472.CAN-13-1506.
- Curran MA, Kim M, Montalvo W, Al-Shamkhani A, Allison JP. Combination CTLA-4 blockade and 4-1BB activation enhances tumor rejection by increasing T-cell infiltration, proliferation, and cytokine production. PLoS One. 2011;6:e19499. doi:10.1371/journal.pone.0019499.
- Mazor R, King E, Pastan I. Anti-drug antibodies to LMB-100 are enhanced by mAbs targeting OX40 and CTLA4 but not by mAbs targeting PD1 or PDL-1. Cell Immunol. 2018;334:38–41. doi:10.1016/j.cellimm.2018.08.016.
- Postow MA, Chesney J, Pavlick AC, Robert C, Grossmann K, McDermott D, Linette GP, Meyer N, Giguere JK, Agarwala SS, et al. Nivolumab and ipilimumab versus ipilimumab in untreated melanoma. N Engl J Med. 2015;372:2006–17. doi:10.1056/NEJMoa1414428.
- Rizvi NA, Hellmann MD, Snyder A, Kvistborg P, Makarov V, Havel JJ, Lee W, Yuan J, Wong P, Ho TS, et al. Mutational landscape determines sensitivity to PD-1 blockade in non-small cell lung cancer. Science. 2015;348:124–28. doi:10.1126/science.aaa1348.
- Le DT, Durham JN, Smith KN, Wang H, Bartlett BR, Aulakh LK, Lu S, Kemberling H, Wilt C, Luber BS, et al. Mismatch repair deficiency predicts response of solid tumors to PD-1 blockade. Science. 2017;357:409–13. doi:10.1126/science.aan6733.
- Schumacher TN, Schreiber RD. Neoantigens in cancer immunotherapy. Science. 2015;348:69–74. doi:10.1126/science.aaa4971.
- Sahin U, Tã¼Reci Ã. Personalized vaccines for cancer immunotherapy. Science. 2018;359:1355–60. doi:10.1126/science.aar7112.
- Ott PA, Hu Z, Keskin DB, Shukla SA, Sun J, Bozym DJ, Zhang W, Luoma A, Giobbie-Hurder A, Peter L, Chen C. An immunogenic personal neoantigen vaccine for patients with melanoma. Nature. 2017;547:217. doi:10.1038/nature22968.
- Lim CJ, Lee YH, Pan L, Lai L, Chua C, Wasser M, Lim TKH, Yeong J, Toh HC, Lee SY, et al. Multidimensional analyses reveal distinct immune microenvironment in hepatitis B virus-related hepatocellular carcinoma. Gut. 2018. doi:10.1136/gutjnl-2018-316510.
- Matson V, Fessler J, Bao R, Chongsuwat T, Zha Y, Alegre ML, Luke JJ, Gajewski TF. The commensal microbiome is associated with anti-PD-1 efficacy in metastatic melanoma patients. Science. 2018;359:104. doi:10.1126/science.aao3290.
- Routy B, Le CE, Derosa L, Cpm D, Alou MT, Daillã¨Re R, Fluckiger A, Messaoudene M, Rauber C, Roberti MP, Fidelle M. Gut microbiome influences efficacy of PD-1-based immunotherapy against epithelial tumors. Science. 2017;359:91. doi:10.1126/science.aan3706.
- Gopalakrishnan V, Spencer CN, Nezi L, Reuben A, Andrews MC, Karpinets TV, Prieto PA, Vicente D, Hoffman K, Wei SC, et al. Gut microbiome modulates response to anti-PD-1 immunotherapy in melanoma patients. Science. 2018;359:97–103. doi:10.1126/science.aan4236.
- Mathew M, Enzler T, Shu CA, Rizvi NA. Combining chemotherapy with PD-1 blockade in NSCLC. Pharmacol Ther. 2018;186:130–37. doi:10.1016/j.pharmthera.2018.01.003.
- Victor C, Rech AJ, Maity A, Rengan R, Pauken KE, Stelekati E, Benci JL, Xu B, Dada H, Odorizzi PM, et al. Radiation and dual checkpoint blockade activate non-redundant immune mechanisms in cancer. Nature. 2015;520:373–77. doi:10.1038/nature14292.
- Simeone E, Grimaldi AM, Festino L, Giannarelli D, Vanella V, Palla M, Curvietto M, Esposito A, Palmieri G, Mozzillo N, et al. Correlation between previous treatment with BRAF inhibitors and clinical response to pembrolizumab in patients with advanced melanoma. Oncoimmunology. 2017;6:e1283462. doi:10.1080/2162402X.2017.1283462.
- Winograd R, Byrne KT, Evans RA, Odorizzi PM, Meyer AR, Bajor DL, Clendenin C, Stanger BZ, Furth EE, Wherry EJ, et al. Induction of T-cell immunity overcomes complete resistance to PD-1 and CTLA-4 blockade and improves survival in pancreatic carcinoma. Cancer Immunol Res. 2015;3:399–411. doi:10.1158/2326-6066.CIR-14-0215.
- Brown ZJ, Yu SJ, Heinrich B, Ma C, Fu Q, Sandhu M, Agdashian D, Zhang Q, Korangy F, Greten TF. Indoleamine 2,3-dioxygenase provides adaptive resistance to immune checkpoint inhibitors in hepatocellular carcinoma. Cancer Immunol Immunother. 2018;67:1305–15. doi:10.1007/s00262-018-2190-4.
- Muller AJ, Manfredi MG, Zakharia Y, Prendergast GC. 2018. Inhibiting IDO pathways to treat cancer: lessons from the ECHO-301 trial and beyond. Semin Immunopathol. 2019;41:41–8. doi:10.1007/s00281-018-0702-0.