ABSTRACT
Three phase II randomized trials evaluated the safety/immunogenicity of two formulations of live-attenuated tetravalent dengue virus (TDEN) vaccine in dengue-endemic (Puerto Rico, Thailand) and non-endemic (US) regions (NCT00350337/NCT00370682/NCT00468858). We describe cell-mediated immune (CMI) responses; safety and humoral responses were reported previously. Participants received two doses of vaccine or control (placebo or the precursor live-attenuated TDEN vaccine) 6 months apart. Selected US participants received a booster 5–12 months post-dose 2. Evaluated subsets of the per-protocol cohorts included 75 primarily dengue virus (DENV)-unprimed US adults, 69 primarily flavivirus-primed Thai adults, and 100 DENV-primed or DENV-unprimed Puerto Rican adults/adolescents/children. T-cell responses were quantified using intracellular cytokine staining (ICS; DENV-infected cell-lysate or DENV-1/DENV-2 peptide-pool stimulation) or IFN-γ ELISPOT (DENV-2 peptide-pool stimulation). Memory B-cell responses were quantified using B-cell ELISPOT. Across populations and age strata, DENV serotype-specific CD4+ T-cell responses were slightly to moderately increased (medians ≤0.18% [ICS]), DENV-2–biased, and variable for both formulations. Responses in unprimed subjects were primarily detected post-dose 1. Response magnitudes in primed subjects were similar between doses. Multifunctional CD8+ T-cell responses were detected after peptide-pool stimulation. T-cell responses were mostly directed to DENV nonstructural proteins 3 and 5. Memory B-cell responses were tetravalent, of low-to-moderate magnitudes (medians ≤0.25%), and mainly observed post-dose 2 in unprimed subjects and post-dose 1 in primed subjects. A third dose did not boost CMI responses. In conclusion, both formulations of the live-attenuated TDEN vaccine candidate were poorly to moderately immunogenic with respect to B-cell and T-cell responses, irrespective of the priming status of the participants.
Abbreviation
ATP: according-to-protocol; ICS: Intracellular Cytokine Staining; NS3: Nonstructural protein 3; ELISPOT: Enzyme-Linked ImmunoSpot; JEV: Japanese encephalitis virus; PBMC: peripheral blood mononuclear cells
Introduction
Dengue is endemic in over 125 countries in tropical and sub-tropical areas. There are an estimated 390 million dengue infections annually worldwide, and in recent years 50–100 million infections manifested clinically each year.Citation1-Citation3 The causative agents, the dengue viruses (DENVs), are single-stranded, positive-sense RNA viruses of the Flaviviridae family. Infection with any of the four antigenically distinct, but closely related serotypes (DENV-1, −2, −3 and −4) can manifest as a subclinical infection, dengue fever, dengue hemorrhagic fever, or dengue shock syndrome.Citation4
Correlates of risk or protective immunity have not been established for dengue.Citation5 Neutralizing antibody (nAb) responses (naturally induced) have been associated with protection from infection with DENV-1, −2 and −4, with DENV-2 potentially requiring higher titers.Citation6 The nAb responses have partial cross-reactivity between serotypes. Infection-induced homotypic immunity can confer long-term protection, potentially persisting for decades, while the induced heterotypic responses and their protective capacities are thought to wane after a few years.Citation7 It has been proposed that during a secondary infection, pre-existing heterotypic non-neutralizing antibody profiles could be associated with increased dengue immunopathology.Citation8 However no direct association between nAb response profiles (with respect to serotype specificity and neutralization titers) and protection or risk of disease was observed in efficacy trials evaluating a live-attenuated tetravalent dengue (TDEN) vaccine, at least in the available sample set.Citation9
Cell-mediated immune (CMI) endpoints, and their associations with protection or pathogenicity, have also been explored in multiple clinical vaccine trials. Though such associations were generally weaker as compared to those for humoral responses, the cell-mediated arm of the immune system is thought to contribute to protection against dengue. DENV serotype-specific memory B cells are important for the secondary humoral response. In addition, high-frequency, pre-existing T-helper (Th) and cytotoxic T cells (together with antibodies) are believed to mediate protection by neutralizing the virus, secreting inflammatory cytokines (notably IFN-γ) and killing infected cells.Citation10-Citation14 Studies in a transgenic murine model deficient in IFN-α/β and IFN-γ receptors support a protective role for T cells against primary infection (reviewed in ref.Citation15). Yet, while high-avidity, homologous, tetravalent and Th1-biased memory T-cell responses activated after secondary infection are considered beneficial, low-affinity, heterologous and TNF-α–biased memory T-cell responses might promote immunopathology.Citation16 Moreover, clinical outcomes may depend on the epitope(s) targeted by the DENV-specific T-cell response.Citation17,Citation18
One live-attenuated recombinant vaccine (Dengvaxia, Sanofi Pasteur) has been registered in several countries with an indication for vaccination in those aged 9 years and older, but its efficacy varies across the infecting serotype(s) and the recipients’ pre-vaccination dengue serostatus.Citation7 Furthermore, a safety signal (an increased risk of hospitalization for dengue disease) was observed in vaccine recipients 2–5 years of age.Citation19,Citation20 Therefore, a dengue vaccine that is efficacious for all ages and independent of serotype or prior exposures to DENV remains a critical medical need. Several other live-attenuated dengue vaccine candidates are in development.Citation21
The precursor of the TDEN vaccine candidate, F17/Pre, consisting of monovalent vaccines combined into a tetravalent preparation at administrationCitation22,Citation23 was evaluated in phase I/II pediatric studies in Thailand.Citation24,Citation25 Subsequent efforts to improve the vaccine’s quality led to two new formulations, F17 and F19. These formulations were prepared from re-derived, amplified F17/Pre master seeds and then lyophilized as a tetravalent vaccine.Citation26 F17 and F19 differed only in their DENV-4 concentration. These formulations were evaluated in populations in a region where DENV is not endemic (US), as well as in DENV-endemic regions (Thailand and Puerto Rico).Citation26-Citation28 The study populations consisted of primarily DENV-unprimed US adults, primarily flavivirus-primed Thai adults, and a mixed-age Puerto Rican population that was balanced in terms of DENV-primed and -unprimed subjects. When administered in two doses 6 months apart, the vaccines were well tolerated and immunogenic in terms of nAb responses in each population, without consistent immunogenicity differences between the formulations across the three studies.
It is well established that the magnitude of DENV-specific immune response to a live-attenuated TDEN vaccine may vary by the subject’s baseline priming status with respect to DENV or other flaviviruses.Citation7,Citation14,Citation29 While a majority of the primed subjects in the abovementioned trials mounted tetravalent nAb responses after a single dose, most of the unprimed subjects needed a second dose to attain this.Citation26-Citation28 Here, we report on the CMI responses observed in these trials. We characterized the DENV serotype-specific CD4+ and CD8+ T-cell and memory B-cell responses in subsets of the per-protocol cohorts, and investigated the role of DENV- and/or flavivirus-specific pre-existing immunity in the induced responses.
Results
Study populations
In the evaluated subsets of the ATP cohorts, 19%, 96%, and 61% of the US, Thai, and Puerto Rican subjects, respectively, were DENV- or flavivirus-positive in neutralization tests at baseline, reflecting the overall priming status of the full ATP cohorts (). In the Puerto Rican study 85% of the subjects in the Adult/Adolescent (≥12 years of age) subgroup were DENV-primed, while the distribution was more balanced in the Child (<12 years of age) subgroup in which 49% of the subjects were primed.
Table 1. Baseline immune status in the according-to-protocol cohorts and subsets.
CMI responses
T-cell responses measured by ICS assay
Different assays were performed (). DENV serotype-specific immune marker (CD40L/IL-2/TNF-α/IFN-γ)-expressing CD4+ and CD8+ T cells were first assessed using flow cytometry-based ICS assay upon in vitro stimulation with inactivated DENV-infected cell lysates. The gating strategy and representative scatterplots are presented in Figure S1.
Table 2. T- and B-cell assays.
Children
For both vaccines, frequencies of DENV-specific CD4+ T-cells expressing at least one marker were low (medians ≤0.03%), and primarily DENV-2–biased after each dose, expressing IL-2 as well as IFN-γ and TNF-α (). Between the doses, the DENV-2–specific responses tended to be higher following the first dose of F17 or F19 in primed subjects (peaking at months 3 and 6, respectively), and higher following the second dose (M7) of F17 in unprimed subjects (). The latter trend was less clearly seen in the F19 group. No T-cell or B-cell responses were observed after administration of placebo (data not shown).
Figure 1. DENV-specific CD4+ T-cell responses in Puerto Rican children. DENV-specific CD4+ T-cell responses in Puerto Rican children aged 1–12 years after immunization with the F17 or F19 vaccines are presented for the overall sample subset (a), and stratified by their DENV priming status at baseline (b). Blood samples were obtained prior to each vaccination (Day [D] 0 and month [M] 6), 3 months after the first dose (M3) and 1 month after the second dose (M7). Data are represented in box-and-whiskers plots as percentages of DENV-specific CD4+ T cells expressing (after in vitro stimulation) at least IL-2, IFN-γ or TNF-α among all CD4+ T cells, with medians, first and third quartiles, and minimum/maximum values presented.
![Figure 1. DENV-specific CD4+ T-cell responses in Puerto Rican children. DENV-specific CD4+ T-cell responses in Puerto Rican children aged 1–12 years after immunization with the F17 or F19 vaccines are presented for the overall sample subset (a), and stratified by their DENV priming status at baseline (b). Blood samples were obtained prior to each vaccination (Day [D] 0 and month [M] 6), 3 months after the first dose (M3) and 1 month after the second dose (M7). Data are represented in box-and-whiskers plots as percentages of DENV-specific CD4+ T cells expressing (after in vitro stimulation) at least IL-2, IFN-γ or TNF-α among all CD4+ T cells, with medians, first and third quartiles, and minimum/maximum values presented.](/cms/asset/a2e15c7d-578e-491f-a3b1-c9f9a7b87b81/khvi_a_1581536_f0001a_b.gif)
Adults and adolescents
Across the three studies, median frequencies of CD4+ T cells expressing at least two immune markers were increased (peak response ≤0.18%) when targeted to DENV-2, but very low (typically ≤0.04%) when targeted to the other serotypes (). No pre-vaccination responses were observed in the majority of US adults, consistent with their predominantly unprimed status. In these subjects, DENV-2–specific responses in the F17 and F19 groups were moderately increased after the first dose (month 1; medians ≤0.18%), with a relatively high inter-subject variability. These responses were decreased just before dose 2 (month 6), and marginally increased after dose 2 (month 7). No increased CD4+ T-cell responses were observed one month after the booster dose of F17 or F19 administered 5–12 months post-dose 2 (data not shown). However, it is noted that the number of subjects who received a third dose was low (N = 9 and 12 in the F17 and F19 groups, respectively). In addition, no consistent differences in responses were detected between the F17 or F19 vaccines on the one hand, and the precursor vaccine F17/Pre on the other hand (data not shown).
Figure 2. DENV-specific CD4+ T-cell responses in adults and/or adolescents in the US, Thailand and Puerto Rico. DENV-specific CD4+ T-cell responses after immunization with the F17 or F19 vaccines are presented for the overall sample subset of adults in the US and Thailand, and adolescents and adults in Puerto Rico (a), and for US subjects stratified by their DENV priming status at baseline, with numbers of evaluated subjects as indicated (b). Blood samples were obtained prior to each dose (Day [D] 0 and month [M] 6), 1 and 3 months after both the first dose (M1 and M3) and the second dose (M7 and M9). Data are presented in box-and-whiskers plots as percentages of DENV-specific CD4+ T cells expressing (after in vitro stimulation) at least two immune markers among IFN-γ, IL-2, TNF-α and CD40L of all CD4+ T cells, with medians, first and third quartiles, and minimum/maximum values presented.
![Figure 2. DENV-specific CD4+ T-cell responses in adults and/or adolescents in the US, Thailand and Puerto Rico. DENV-specific CD4+ T-cell responses after immunization with the F17 or F19 vaccines are presented for the overall sample subset of adults in the US and Thailand, and adolescents and adults in Puerto Rico (a), and for US subjects stratified by their DENV priming status at baseline, with numbers of evaluated subjects as indicated (b). Blood samples were obtained prior to each dose (Day [D] 0 and month [M] 6), 1 and 3 months after both the first dose (M1 and M3) and the second dose (M7 and M9). Data are presented in box-and-whiskers plots as percentages of DENV-specific CD4+ T cells expressing (after in vitro stimulation) at least two immune markers among IFN-γ, IL-2, TNF-α and CD40L of all CD4+ T cells, with medians, first and third quartiles, and minimum/maximum values presented.](/cms/asset/65c68eea-c12c-40fb-9882-adb04008ce2d/khvi_a_1581536_f0002a_b.gif)
The majority of Thai adults had a baseline multitypic response, of which only the DENV-2–specific responses were marginally increased (medians ≤0.06%) after the first, but not the second dose of either vaccine. Low baseline responses were also seen in the Puerto Rican adults and adolescents, of which the DENV-2–specific responses were slightly increased after the first dose (medians ≤0.11%), while no or minor increases were observed after the second dose of F17 or F19, respectively. Further analysis of the DENV-2–specific responses in this population revealed that the cells responding to F19 expressed mostly IL-2 and IFN-γ, suggestive of a Th0/Th1-biased phenotype, whereas no consistent pattern was observed for cells responding to F17 (Figure S2).
No CD4+ T-cell responses were detected after administration of placebo in all three studies (data not shown).
As expected given the subset’s composition, stratification of the responses in US subjects by their priming status revealed that in both vaccine groups, the kinetics of the response in unprimed subjects followed that observed for the entire subset (). In contrast, the response kinetics in primed subjects appeared similar to that seen in the Thai subjects, with no or only minor increases after each dose. Yet, no definitive conclusions could be drawn due to the low sample sizes of primed subjects (N = 3 or 4 vs N = 14 or 15 for unprimed subjects).
No CD8+ T-cell responses were detected in these ICS assays (data not shown). This may be a consequence of the nature of the stimulating antigen used in this assay (see ). Indeed, DENV-specific IFN-γ+ CD8+ T-cell responses, as well as IFN-γ+ CD4+ T-cell responses were detected 1 month post-dose 2 in the US study, using a modified ICS assay with DENV-1 and −2 peptide-pool stimulation (). Antigen-specific CD4+ and CD8+ T-cell responses were observed in 3 and 9 of the 13 vaccinated subjects tested, respectively () but not in the two tested placebo recipients (data not shown). A considerable proportion of the responding CD4+ or CD8+ T cells exhibited multifunctional expression profiles (i.e., expressing ≥2 immune markers, among CD40L [for CD4+ T cells], IL-2, TNF-α, IFN-γ, the degranulation marker CD107a, and [for CD8+ T cells], MIP-1β, a marker of inflammatory phagocytes). The responding CD8+ T cells were targeted against any of the four tested antigens, while the CD4+ T cells were mostly targeted against DENV-1 NS3.
Figure 3. DENV-specific T-cell responses in US adults (ICS with peptide pool stimulation). (a) Representative flow plots of IFN-γ vs TNF-α production by CD4+ T cells or CD8+ T cells (left-hand and right-hand panels, respectively) after stimulation of peripheral blood mononuclear cells with negative control or the DENV-2 nonstructural (NS) 3 peptide pool are shown. Cells co-expressing other functions (CD107a, IL-2, CD40L or MIP-1β) are highlighted in color. Data are from one representative subject at 1 month after the second dose (M7). (b) Magnitudes of responses of CD4+ T cells and CD8+ T cells (left-hand and right-hand panels, respectively) producing at least IFN-γ after stimulation with DENV-1 envelope (E), DENV-2 E, DENV-1 NS3, or DENV-2 NS3 peptide pools are presented for 13 vaccinated subjects. PBMC were obtained pre-vaccination (D0) and 1 month after the second dose (M7). Data are background (negative control value)-subtracted. For the subjects with a D0 value available, a response was considered positive if it was ≥0.05%, and the background-subtracted value at M7 was at least 3 times higher than the subject-matched value at day 0.
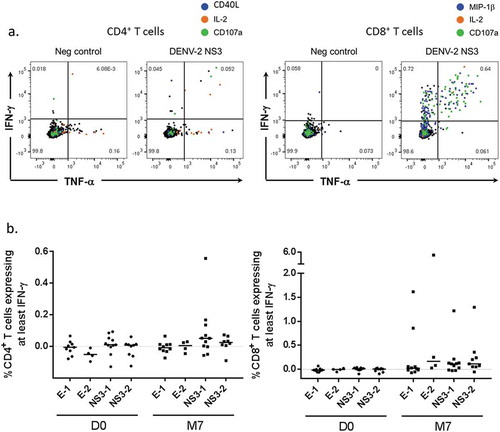
T-cell antigen specificity and cross-reactivity
Next, the specificity of the induced T-cell response was investigated at the antigen level. For a subset of the unprimed US subjects, T-cell specificity for eight individual DENV-2 antigens was assessed in IFN-γ ELISPOT (see ). No baseline responses were detected, consistent with the ICS results (data not shown). At 1 month post-dose 2, the majority of vaccine recipients mounted a response that was predominantly directed to NS3 and NS5 (detected in 82% and 73% of subjects, respectively), but the specificities were highly variable between subjects (). When the serotype cross-reactivity of the T cells was determined for eight subjects with a DENV-2 NS3-specific response, monovalent, bivalent, trivalent and tetravalent NS3-specific responses were observed in 3, 1, 1 and 3 subjects, respectively (), though the low sample size precluded any further conclusions.
Figure 4. Specificity of T-cell responses in DENV-unprimed subjects. The magnitudes and specificity of background (medium)-subtracted T-cell responses to different DENV antigens measured at 1 month after the second dose (Month 7) by IFN-γ ELISPOT are presented. Samples were collected from a subset of US subjects (with subject numbers indicated) who were DENV-unprimed at baseline. Each bar represents the response of one subject. A response was considered positive if it was ≥55 spot-forming units (SFU) per 106 PBMC, and exceeded response to the negative control by four-fold. (a) Magnitudes of T-cell responses to individual DENV-2 antigens measured after stimulation with peptide pools covering DENV-2 nonstructural (NS) 1, NS2, NS3, NS4, NS5, capsid (C), premembrane (PrM) and envelope (E) proteins are presented. (b) Relative proportions of the T-cell response to DENV-1, DENV-2, DENV-3 and DENV-4 NS3 peptide pools were determined for the subjects with DENV-2 NS3 specific response in the analysis shown in (a).
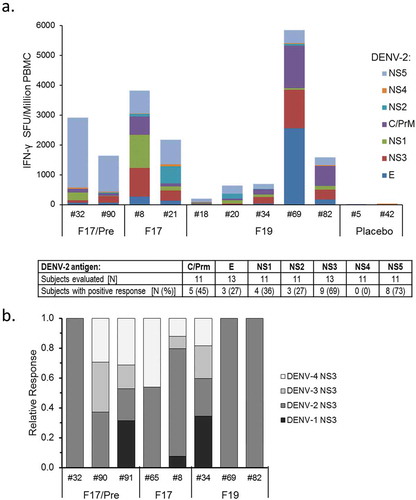
Memory B-cell responses
Memory B-cell responses were measured using the B-cell ELISPOT assay (Figure S3). Across the three studies, frequencies of DENV-specific memory B-cell responses in adults or adolescents were low to moderately high (medians ≤0.25%), balanced, and relatively variable between the subjects (), while no responses were detected after administration of placebo (data not shown). Indeed, both doses of either vaccine induced a response in a minority of the US subjects, which particularly in the F19 group appeared to be higher after dose 2. Aligned with the CD4+ T-cell responses, we observed in this study no memory B-cell responses after the booster vaccinations with F17 and F19, nor any differences between these formulations and the F17/Pre control. Among the Thai subjects, by contrast, the majority of subjects in both groups elicited a response after the first dose, with lower inter-subject variability and higher median responses in the F19 group, while only a minority of the subjects in either group demonstrated a boost after the second dose. By comparison, most of the Puerto Rican subjects mounted only responses to the F17 vaccine, which were of comparable magnitudes between the doses, though the variability was also relatively high. Similar results were obtained when these frequencies were corrected for the baseline response by subject (). Evaluation by priming status in the US study revealed a tendency for a higher increase in baseline responses in the primed subjects (), though sample sizes were low and unbalanced between the primed/unprimed subgroups.
Figure 5. DENV-specific B-cell responses to tetravalent live-attenuated DENV vaccines in different study populations. (a) Frequencies of DENV-specific B-cells for the overall sample subsets of study populations in the US and Thailand (adults) and Puerto Rico (adults and adolescents) after immunization with F17 or F19 vaccine are presented. Blood samples were obtained prior to each dose (Day [D] 0 and month [M] 6), at 1 and 3 months after both the first dose (M1 and M3) and the second dose (M7 and M9). Box-and-whiskers plots represent the percentages of responding B cells with medians, first and third quartiles, and the minimum/maximum values measured. (b) B-cell responses in the Puerto Rican subjects after subtraction of the pre-vaccination responses by subject are presented. (c) B-cell responses in the US subjects stratified by their DENV priming status at baseline are presented, with numbers of evaluated subjects indicated (numbers may vary by time-point and serotype).
![Figure 5. DENV-specific B-cell responses to tetravalent live-attenuated DENV vaccines in different study populations. (a) Frequencies of DENV-specific B-cells for the overall sample subsets of study populations in the US and Thailand (adults) and Puerto Rico (adults and adolescents) after immunization with F17 or F19 vaccine are presented. Blood samples were obtained prior to each dose (Day [D] 0 and month [M] 6), at 1 and 3 months after both the first dose (M1 and M3) and the second dose (M7 and M9). Box-and-whiskers plots represent the percentages of responding B cells with medians, first and third quartiles, and the minimum/maximum values measured. (b) B-cell responses in the Puerto Rican subjects after subtraction of the pre-vaccination responses by subject are presented. (c) B-cell responses in the US subjects stratified by their DENV priming status at baseline are presented, with numbers of evaluated subjects indicated (numbers may vary by time-point and serotype).](/cms/asset/161b8633-3a24-4e7b-ad42-6ecdf7a6417b/khvi_a_1581536_f0005a_b.gif)
Among the Thai subjects, there was no apparent impact of the JEV priming status on the B-cell responses to either vaccine at baseline (), though again sample sizes were low and unbalanced. The B-cell response did however seem to correlate positively with the previously reportedCitation27 DENV-specific nAb responses at months 1 and 7 ().
Figure 6. Correlations between memory B-cell responses and neutralizing antibody responses in Thai adults. Memory B-cell responses and DENV-specific neutralizing antibody responses observed in Thai adults are presented at pre-vaccination (pre), by the subjects’ baseline Japanese encephalitis virus (JEV) priming status (a), and at pre-vaccination, 1 month after the first (P1(M1)) and the second (PII(M7)) dose, by DENV serotype (b).
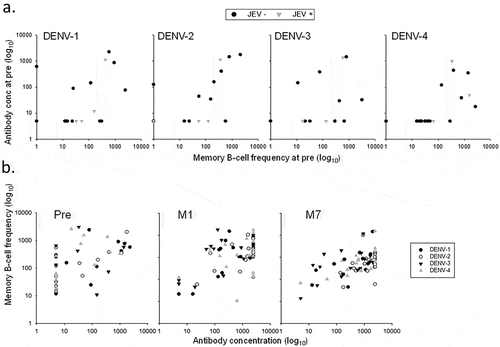
Discussion
We previously reported on the humoral immunogenicity of the F17/Pre, F17 and F19 formulations in different populations.Citation26-Citation28 As for other live-attenuated DENV vaccines,Citation14,Citation29,Citation30 we observed that the absolute magnitudes of nAb responses in primed individuals were generally greater than in unprimed subjects, although the vaccine-induced increases from pre-vaccination responses were lower in primed vs in unprimed subjects. In the US subjects, nAb responses to the first dose were biased to one serotype (most often DENV-2) and boosted and broadened to the other serotypes after the second dose,Citation26 whereas in the Thai subjects, the highest increase was observed after the first dose.Citation27 These differences may stem from variations in B-cell and T-cell memory from prior infection(s), resulting in different effector responses to vaccination. Here, we sought to investigate the impact of baseline priming on the T-cell and memory B-cell vaccine-induced responses underlying the nAb responses.
This investigation makes the following points: (1) Administration of the F17 or F19 vaccines induced variable and primarily DENV-2–biased CD4+ T-cell responses and low to moderately increased tetravalent memory B-cell responses in each population, without consistent differences between the formulations; (2) Aligned with the nAb response,Citation26 the third dose offered to the US adults did not boost the CMI response; (3) Upon evaluation in some individuals, the vaccines induced CD8+ T-cell responses with a multifunctional cytokine-expression profile; (4) While the trends in the kinetics of memory B-cell responses tended to mirror those seen in the nAb responses, no such parallel was apparent for the CD4+ T-cell responses.
Although most live-attenuated DENV vaccines are known to elicit mainly multitypic T-cell responses (reviewed in ref.Citation17), in the present populations the induced CD4+ T-cell responses failed to develop into balanced multitypic responses and were mainly targeted against DENV-2. It is unknown whether this serotype hierarchy is associated with higher replication levels of the DENV-2 vaccine strain and/or lower replication levels of the other three vaccine viruses. Alternatively or in addition, stimulation of PBMC with inactivated DENV-infected cell lysates instead of peptide-pools may have rendered the ICS assay less reproducible, as previously suggested,Citation31 and this could have prevented a consistent detection of multitypic responses.
In contrast to the nAb responses, the induced CD4+ T-cell responses in the unprimed (US) adults tended to be higher than in the primed adults/adolescents, and were increased mainly after the first dose. Possibly the absence of a pre-existing multitypic antibody response in these subjects allowed a more extensive replication of vaccine virus post-dose 1, inducing higher T-cell responses, while post-dose 2, replication was diminished by the immunity induced by the first dose. Similarly, in the Thai population, it is likely that the presence of pre-existing antibody responses limited viral replication, preventing a robust CMI response to vaccination. This was to some extent also the case in the Puerto Rican population, which was primed to a lower degree as compared with the Thai population. The lack of response to the third dose suggests that the long-term persisting humoral immunity sufficed to prevent vaccine virus replication after vaccination. Consistently, no vaccine-induced T-cell responses were detected in the majority of the Thai children who received a booster dose of F17/Pre or F17.Citation32 When we compared the kinetics of CD4+ T-cell responses between the Child and Adult/Adolescent groups in the present study by priming status, the observed patterns tended to match when comparing primed subjects, but were variable when comparing unprimed subjects. Again, conclusions may be hampered by the difficulties inherent to the antigenic preparation used in our main assay.
We presume that the absence of detectable CD8+ T cells after stimulation of PBMC using inactivated DENV-infected cell lysates was related to the assay rather than to the vaccines’ specific immunogenicity, since the protein format of the antigens may have been suboptimal for the detection of CD8+ T cells.Citation10,Citation33-Citation35 Indeed, we did detect CD8+ T-cell responses in the ICS assay with peptide-pool stimulation, similar to observations for other live-attenuated dengue or yellow fever vaccines using comparable methods.Citation36-Citation39 A possible explanation is that the inactivated viral antigen in the cell lysate is preferentially presented through the MHC-II pathway, while peptide pools of varying lengths may be presented by both MHC-I and MHC-II pathways, resulting in a different CD4+/CD8+ T-cell balance between the two stimulation methods.Citation33
CD8+ T-cell responses to infection or live-attenuated DENV vaccines are often biased toward the highly conserved NS proteins, particularly NS3 and NS5, while infection-induced CD4+ T-cell and B-cell responses mainly target the structural proteins E, PrM and C, as well as NS1.Citation36,Citation37,Citation39-Citation41 In the present study, the DENV-2–specific T-cell responses in the US adults were mainly directed to NS3 and NS5 (it is noted that the assay used for this analysis did not allow identification of the T-cell phenotypes). Furthermore, a proportion of the responding CD4+ and CD8+ T-cells tended to be Th0/Th1-biased and multifunctional, as seen for other live-attenuated dengue vaccines in primed and flavivirus-unprimed subjects.Citation17
Aligned with the nAb responses,Citation28 memory B-cell responses in the Thai adults were generally higher than in the US adults, and increases were mostly detected post-dose 1 rather than post-dose 2 as seen in the US adults, likely associated with the difference in priming status of these populations. The putative correlation between the memory B-cell and nAb responses to vaccination in the Thai subjects may be explained by differentiation of the memory B cells into antibody-secreting plasma cells upon antigenic (re-)stimulation, even though for other viral pathogens it has been suggested that these cell populations may not be governed by the same regulating mechanisms.Citation42
Since the vaccine-induced CMI responses were exploratory endpoints in these Phase II trials, the analyses were performed on randomly selected sample subsets of the per-protocol cohorts. The following limitations should therefore be noted in interpreting the current results. First, analysis of the difference in immunogenicity profiles between primed and unprimed participants was limited by the unbalanced distribution between these groups and relatively small sample sizes. Furthermore, due to these sample size limitations we were unable to stratify the primed subjects by their monovalent- or polyvalent-positive status at baseline, which may also have contributed to the substantial variability in CMI responses detected within each study population. For nAb responses, it has been observed that subjects with a baseline multitypic profile responded differently to live-attenuated TDEN vaccine administration as compared to seronegative or monotypic subjects,Citation43 and this may also apply to the vaccine-induced CMI responses. Last, as noted earlier, adequate assessment of the CD8+ T cell responses was hampered by the use of inactivated DENV-infected cell lysates in the main ICS assay, and by the limited sample size in the ICS using DENV peptide pool-based stimulation.
The F17 formulation was previously shown to have a marginally stronger immunogenicity profile than F19 with respect to humoral responses,Citation28 but this was not consistently seen for the CMI responses. Yet, in pediatric follow-up trials in Thailand, neither the first two doses of F17/Pre and F17, nor the booster vaccination with these vaccines administered 1 or 3.5 years later, induced durable multivalent nAb, B-cell or T-cell responses.Citation32 This is consistent with the waning of CMI responses over the 5–12 months post booster vaccination as observed here with the F17 or F19 vaccines. While persistent and balanced nAb and CMI responses that are stable after several years are presumably key features of a successful dengue vaccine, interpretation of vaccine-induced immunity data is hampered by the limited understanding of the specific immune profile required for protection.Citation5 This understanding is further complicated by the potential presence of asymptomatic dengue infections, as occasionally observed during vaccine trials,Citation25,Citation27 and by other issues which emerged from the efficacy trial data of the licensed dengue vaccine.Citation5,Citation7,Citation9 Therefore, in the absence of identified immune correlates of risk or protection, these obstacles in dengue vaccine development can currently only be overcome by performing clinical endpoint studies, possibly supported by studies using dengue human infection models.Citation44
Conclusion
The live-attenuated TDEN vaccine candidates were poorly to moderately immunogenic with respect to B-cell and T-cell responses in primed and unprimed subjects. Two vaccine doses elicited mostly variable CMI responses, which could not be boosted by a third dose. While the B-cell responses were mostly tetravalent, T-cell responses were imbalanced between the serotypes. Previously the vaccine was shown to be unable to induce durable multivalent DENV-specific nAb responses.
A tetravalent purified inactivated DENV vaccine formulated with aluminum hydroxide or an Adjuvant System (AS01E or AS03B) is currently in Phase 1 clinical trials (ref.Citation45 and NCT01702857). Several scientific and technological challenges still have to be met to ensure the required development of future vaccines against this important medical need.
For the benefit of healthcare professionals, a graphical summary contextualizing the results and relevance of this clinical research is presented in .
Materials & methods
Study designs
The placebo-controlled, randomized, phase II trials conducted in the US, Thailand and Puerto Rico (NCT00350337, NCT00370682 and NCT00468858, respectively; refs.Citation26-Citation28) evaluated the safety and immunogenicity of the F17 and F19 vaccines, with the precursor vaccine F17/Pre used as control in the US study. The studies were conducted in accordance with Good Clinical Practice guidelines, the 1996 Helsinki Declaration and respective state regulations.
Objectives
The objective of the present manuscript was to describe DENV serotype-specific CD4+/CD8+ T-cell and memory B-cell responses to the F17, F17/Pre and F19 vaccines following the two-dose primary vaccination, and in the US adults also following the booster vaccination, in subsets of each study cohort.
Participants
Healthy US, Thai, and Puerto Rican males and females were between 18 and 45, 20 and 25, and 1 and 50 years of age at enrollment, respectively. As described previously,Citation26-Citation28 US and Puerto Rican subjects were categorized at baseline as either DENV-primed (seropositive to at least one DENV serotype) or unprimed, and Thai subjects as either flavivirus-primed (seropositive to at least one DENV serotype or to JEV) or unprimed. The present evaluations were performed on sample subsets that were randomly selected from the ATPcohorts of each study (). For these evaluations, the subset of Puerto Rican subjects was stratified by age into the Child or Adult/Adolescent subgroups (ranges between 1 and 12 and ≥12 years of age, respectively).
Vaccinations
F17/Pre contained four live-attenuated DENV strains (one each serotype).Citation25,Citation46 The two newer formulations F17 and F19 were prepared from re-derived F17/Pre vaccine strains following a slightly adapted process. The process modifications included: (1) application of three additional passages in fetal rhesus lung cells, (2) use of a carbohydrate stabilizer instead of human serum albumin for formulation of monovalent bulks, and (3) lyophilization of the final vaccine as a tetravalent product.Citation26 F19 was identical to F17 except that its DENV-4 concentration was reduced by four-fold (US and Thai studies) or 50-fold (Puerto Rican study) relative to that in F17.Citation26-Citation28 Subjects received two doses of vaccine or cell-culture medium placebo 6 months apart. Nine and 12 of the US subjects of the F17 and F19 groups respectively received a third dose of the vaccine used for their primary immunization between 5 and 12 months after the second dose.
Immunogenicity evaluations
Blood samples for CMI evaluations were collected before each dose (day 0, month 6), 3 months after the first dose (month 3), 1 month after the second dose (month 7), and 1 or 6 months after the third dose (for US subjects only). In the US and Thai studies, samples were also collected 1 month after the first dose (month 1) and 3 months after the second dose (month 9). Testing was performed at GSK, Rixensart, Belgium unless specified otherwise. presents an overview of the CMI assays performed in each study.
T-cell responses
Intracellular cytokine staining
DENV serotype-specific CD4+/CD8+ T-cell responses in adults and adolescents were assessed using ICS and flow cytometry upon short-term in vitro stimulation of PBMC as described previously.Citation32,Citation47 For the Child subgroup in the Puerto Rican study, the ICS assay was performed using undiluted whole blood, based on a previously described assay.Citation48-Citation50 Briefly, PBMC or whole blood was stimulated for 2 h by a heat-inactivated (30 min, 56 °C) lysate of Vero cells infected with the respective DENV serotype (using the DENV-1–4 parent vaccine strains WP-74, S16803, CH53489, and 341750, respectively), or cultured with negative (medium) or positive (staphylococcal enterotoxin B) controls. Brefeldin A was then added for a subsequent 18-h incubation at 37°C. For whole blood samples, PBMC were isolated and fixed following the lysis of red blood cells and then frozen and stored at −80°C. The cells were subsequently stained and analyzed by flow cytometry, following a method that was similar to the one used for the PBMC samples.Citation32,Citation47 For both the PBMC-based and whole blood-based assays, results were expressed as percentages of antigen-specific CD4+/CD8+ T cells expressing combinations of the immune markers CD40L, IFN-γ, IL-2 and TNF-α. Background (the signals obtained in cells stimulated with medium only) was subtracted from all values. To evaluate DENV-specific CD4+/CD8+ T cells in adults and adolescents, ICS results were presented as background-subtracted percentages of CD4+ or CD8+ T-cells expressing at least two of the four immune markers. This parameter (instead of expression of a single marker) was selected to increase the specificity signal of the assay.
For a subset of the US subjects (N = 15; ) an additional ICS assay was performed at the Walter Reed Army Institute of Research (WRAIR) laboratories (Silver Spring, MD, US). In this assay, stimulation was done with peptide pools covering the NS 3 and structural envelope (E) proteins of DENV-1 and DENV-2 serotypes. Briefly, cryopreserved PBMC were thawed and placed in RPMI 1640 medium supplemented with 10% FBS, L-glutamine, penicillin, and streptomycin (RPMI-10). Peptides (13 to 20-mer overlapping by 10–12 amino acids; BEI Resources) were resuspended in 100% dimethyl-sulfoxide (DMSO) at 200 µg/mL/peptide. PBMC were plated in 96-well plates at 1 × 106 cells/well in a total volume of 200 µL RPMI-10, and stimulated with either 1 µg/mL/peptide of the relevant peptide pool in 0.5% DMSO (1:200 dilution), or medium (RPMI-10 containing 0.5% DMSO; negative control), in the presence of anti-CD28, anti-CD49d, and fluorescein isothiocyanate-conjugated anti-CD107a antibodies. Cells were incubated at 37°C for 1 h prior to addition of Brefeldin A and monensin, and were left overnight for further incubation at 37°C. Cells were then washed and stained with a viability marker followed by surface antibodies against CD3, CD4, CD8, CD14, and CD19. After fixation in 4% formaldehyde, cells were permeabilized and stained with antibodies against IFN-γ, TNF-α, macrophage inflammatory protein 1β (MIP-1β), IL-2, and CD40L, and analyzed. Results were expressed as background-subtracted percentages of antigen-specific CD4+/CD8+ T cells expressing combinations of the immune markers CD40L, IFN-γ, IL-2 and TNF-α, CD107a and MIP-1β. A response was considered positive if it was ≥0.05%, and the background-subtracted value at month 7 was at least three times higher than the subject-matched value at day 0.
IFN-γ ELISPOT
For a second subset of the US subjects (N = 13; ) DENV-2–specific IFN-γ-secreting T cells were enumerated using IFN-γ ELISPOT as previously described.Citation51 Testing was performed at WRAIR laboratories. Briefly, PBMC were stimulated in vitro overnight with DENV-2 peptide pools covering NS1, NS2, NS3, NS4, NS5, capsid (C), premembrane (PrM) and E. Human cytomegalovirus/Epstein-Barr virus/influenza virus (CEF) peptide pools were used as a positive control. A response was considered positive if it was ≥55 spot-forming units (SFU)/106 PBMC and exceeded the response to the negative control (medium supplemented with 0.5% DMSO) by four-fold. Results were expressed as background-subtracted SFU/106 cells. Serotype cross-reactive responses were evaluated for subjects with a DENV-2 NS3-specific response in the preceding analysis (N = 8). The NS3-specific response magnitudes were determined for each serotype using DENV-1–4 NS3 peptide pools.
Memory B-cell responses
DENV serotype-specific memory B-cell responses were quantified by B-cell ELISPOT as described previouslyCitation32 (see Figure S3), and reported as frequencies of DENV antigen (recombinant truncated 80E protein)-specific memory B cells within the total memory B-cell population. No B-cell responses were assessed for the Child subgroup in the Puerto Rican study due to limited blood sample volumes.
Statistical analyses
ICS data were analyzed using FlowJo software (FlowJo LLC, Ashland, OR, US) and GraphPad Prism (GraphPad Software, Inc., San Diego, CA). Frequencies of DENV-specific memory B cells and DENV-specific CD4+ and CD8+ T cells were calculated, and descriptive statistical analyses (including the calculation of medians, interquartile ranges, minima and maxima) of these responses were performed using SAS v9.1 (SAS Institute Inc., Cary, NC, US).
Acknowledgments
The authors thank the participating volunteers, and acknowledge the contributions of the clinicians, nurses, QA/QC personnel, data management and cohort retention staff, pharmacists and laboratory technicians of the clinical research sites at the study sites in the US, Thailand and Puerto Rico. They also thank Elodie Tournay, Meng Shi, Murielle Carton, Sarah Charpentier, Stéphanie De Simoni, Dinis Fernandes Ferreira, Samira Hadiy, Caroline Hervé, Michel Janssens, Olivier Jauniaux, Pierre Libert, Michaël Mestré, Alexandre Smirnoff and Zineb Soussi from GSK, Coreen Beaumier, currently at the Baylor College of Medicine, Houston, TX, US, as co-investigator of the Puerto Rican study, and the teams at AFRIMS and WRAIR for their contributions to the reported studies. Finally, they thank Ellen Oe (GSK) and Sophie Timmery (XPE Pharma & Science on behalf of GSK) for providing scientific writing services in the manuscript’s development, and publication management, respectively.
Authors Contribution
KB, JC, KE, HF, RG, RJ, PM, SS, ST and VW contributed substantially to the conception and design of the studies. JC, IE, HF, RG, RJ, PM, SS, PS, ST and VW participated in the collection and analysis of the CMI data. KB, JC, KE, HF, BI, RJ, PM, SS, ST and VW contributed to the interpretation of the results. All authors had full access to the data and were involved in critical revision of the manuscript for important intellectual content. The corresponding author was responsible for manuscript submission.
Disclaimer
The current material has been reviewed by the Walter Reed Army Institute of Research, and the Institute confirms that there is no objection to its presentation and/or publication. The opinions or assertions contained herein are the private views of the authors, and are not to be construed as official, or as reflecting true views of the US Department of the Army or the US Department of Defense.
Disclosure of potential conflicts of interest
PM and BI are or were at the time of the studies employees of the GSK group of companies, and report holding shares in the GSK group of companies. RJ and ST received financial and other support without any personal financial benefit from the GSK group of companies as part of the CRADA between the U.S. Army and the GSK group of companies. KE has a patent for Tetravalent Live-Attenuated DENV vaccine issued to GSK. KB, JC, IE, HF, SS, PS, RG, VW have nothing to disclose.
Supplemental Material
Download MS Word (17.2 MB)Supplementary material
Supplemental data for this article can be accessed on the publisher’s website.
Additional information
Funding
References
- Stanaway JD, Shepard DS, Undurraga EA, Halasa YA, Coffeng LE, Brady OJ, Hay SI, Bedi N, Bensenor IM, Castaneda-Orjuela CA, et al. The global burden of dengue: an analysis from the Global Burden of Disease Study 2013. Lancet Infect Dis. 2016;16(6):712–23. doi:10.1016/S1473-3099(16)00026-8.
- Bhatt S, Gething PW, Brady OJ, Messina JP, Farlow AW, Moyes CL, Drake JM, Brownstein JS, Hoen AG, Sankoh O, et al. The global distribution and burden of dengue. Nature. 2013;496(7446):504–07. doi:10.1038/nature12060.
- Shepard DS, Undurraga EA, Halasa YA, Stanaway JD. The global economic burden of dengue: a systematic analysis. Lancet Infect Dis. 2016;16(8):935–41. doi:10.1016/S1473-3099(16)00146-8.
- WHO. Dengue haemorrhagic fever: diagnosis, treatment, prevention and control. 2nd ed. Switzerland: World Health Organization; 1997.
- Katzelnick LC, Harris E. Immune correlates of protection for dengue: state of the art and research agenda. Vaccine. 2017;35(36):4659–69. doi:10.1016/j.vaccine.2017.07.045.
- Buddhari D, Aldstadt J, Endy TP, Srikiatkhachorn A, Thaisomboonsuk B, Klungthong C, Nisalak A, Khuntirat B, Jarman RG, Fernandez S, et al. Dengue virus neutralizing antibody levels associated with protection from infection in Thai cluster studies. PLoS Negl Trop Dis. 2014;8(10):e3230. doi:10.1371/journal.pntd.0003230.
- Wichmann O, Vannice K, Asturias EJ, de Albuquerque Luna EJ, Longini I, Lopez AL, Smith PG, Tissera H, Yoon IK, Hombach J. Live-attenuated tetravalent dengue vaccines: the needs and challenges of post-licensure evaluation of vaccine safety and effectiveness. Vaccine. 2017;35(42):5535–42. doi:10.1016/j.vaccine.2017.08.066.
- Guzman MG, Alvarez M, Halstead SB. Secondary infection as a risk factor for dengue hemorrhagic fever/dengue shock syndrome: an historical perspective and role of antibody-dependent enhancement of infection. Arch Virol. 2013;158(7):1445–59. doi:10.1007/s00705-013-1645-3.
- Guy B, Briand O, Lang J, Saville M, Jackson N. Development of the Sanofi Pasteur tetravalent dengue vaccine: one more step forward. Vaccine. 2015;33(50):7100–11. doi:10.1016/j.vaccine.2015.09.108.
- Hatch S, Endy TP, Thomas S, Mathew A, Potts J, Pazoles P, Libraty DH, Gibbons R, Rothman AL. Intracellular cytokine production by dengue virus-specific T cells correlates with subclinical secondary infection. J Infect Dis. 2011;203(9):1282–91. doi:10.1093/infdis/jir012.
- Weiskopf D, Angelo MA, de Azeredo EL, Sidney J, Greenbaum JA, Fernando AN, Broadwater A, Kolla RV, De Silva AD, de Silva AM, et al. Comprehensive analysis of dengue virus-specific responses supports an HLA-linked protective role for CD8+ T cells. Proc Natl Acad Sci U S A. 2013;110(22):E2046–E2053. doi:10.1073/pnas.1305227110.
- Yauch LE, Prestwood TR, May MM, Morar MM, Zellweger RM, Peters B, Sette A, Shresta S. CD4+ T cells are not required for the induction of dengue virus-specific CD8+ T cell or antibody responses but contribute to protection after vaccination. J Immunol. 2010;185(9):5405–16. doi:10.4049/jimmunol.1001709.
- Rothman AL. DHIM supporting immunologic investigations and the identification of immune correlates of protection. J Infect Dis. 2014;209(Suppl 2):S61–S65. doi:10.1093/infdis/jiu111.
- Slifka MK. Vaccine-mediated immunity against dengue and the potential for long-term protection against disease. Front Immunol. 2014;5:195. doi:10.3389/fimmu.2014.00195.
- Zellweger RM, Shresta S. Mouse models to study dengue virus immunology and pathogenesis. Front Immunol. 2014;5:151. doi:10.3389/fimmu.2014.00151.
- Mathew A, Townsley E, Ennis FA. Elucidating the role of T cells in protection against and pathogenesis of dengue virus infections. Future Microbiol. 2014;9(3):411–25. doi:10.2217/fmb.13.171.
- Rothman AL, Medin CL, Friberg H, Currier JR. Immunopathogenesis versus protection in dengue virus infections. Curr Trop Med Rep. 2014;1(1):13–20. doi:10.1007/s40475-013-0009-0.
- Schmid MA, Diamond MS, Harris E. Dendritic cells in dengue virus infection: targets of virus replication and mediators of immunity. Front Immunol. 2014;5:647. doi:10.3389/fimmu.2014.00647.
- Hadinegoro SR, Arredondo-García JL, Capeding MR, Deseda C, Chotpitayasunondh T, Dietze R, Muhammad Ismail HI, Reynales H, Limkittikul K, Rivera-Medina DM, et al. Efficacy and long-term safety of a dengue vaccine in regions of endemic disease. N Engl J Med. 2015;373(13):1195–206. doi:10.1056/NEJMoa1506223.
- WHO. Addendum to report of the Global Advisory Committee on Vaccine Safety (GACVS), 10-11 June 2015(1). Safety of CYD-TDV dengue vaccine. Wkly Epidemiol Rec. 2015;90(34):421–23.
- Pang T, Mak TK, Gubler DJ. Prevention and control of dengue-the light at the end of the tunnel. Lancet Infect Dis. 2017;17(3):e79–e87. doi:10.1016/S1473-3099(16)30471-6.
- Edelman R, Wasserman SS, Bodison SA, Putnak RJ, Eckels KH, Tang D, Kanesa-Thasan N, Vaughn DW, Innis BL, Sun W. Phase I trial of 16 formulations of a tetravalent live-attenuated dengue vaccine. Am J Trop Med Hyg. 2003;69:48–60.
- Innis BL, Eckels KH. Progress in development of a live-attenuated, tetravalent dengue virus vaccine by the United States Army Medical Research and Materiel Command. Am J Trop Med Hyg. 2003;69:1–4.
- Simasathien S, Thomas SJ, Watanaveeradej V, Nisalak A, Barberousse C, Innis BL, Sun W, Putnak JR, Eckels KH, Hutagalung Y, et al. Safety and immunogenicity of a tetravalent live-attenuated dengue vaccine in flavivirus naive children. Am J Trop Med Hyg. 2008;78:426–33.
- Watanaveeradej V, Simasathien S, Nisalak A, Endy TP, Jarman RG, Innis BL, Thomas SJ, Gibbons RV, Hengprasert S, Samakoses R, et al. Safety and immunogenicity of a tetravalent live-attenuated dengue vaccine in flavivirus-naive infants. Am J Trop Med Hyg. 2011;85(2):341–51. doi:10.4269/ajtmh.2011.10-0501.
- Thomas SJ, Eckels KH, Carletti I, De la Barrera R, Dessy F, Fernandez S, Putnak R, Toussaint JF, Sun W, Bauer K, et al. A phase II, randomized, safety and immunogenicity study of a re-derived, live-attenuated dengue virus vaccine in healthy adults. Am J Trop Med Hyg. 2013;88(1):73–88. doi:10.4269/ajtmh.2012.12-0361.
- Watanaveeradej V, Gibbons RV, Simasathien S, Nisalak A, Jarman RG, Kerdpanich A, Tournay E, De La Barrerra R, Dessy F, Toussaint JF, et al. Safety and immunogenicity of a rederived, live-attenuated dengue virus vaccine in healthy adults living in Thailand: a randomized trial. Am J Trop Med Hyg. 2014;91(1):119–28. doi:10.4269/ajtmh.13-0452.
- Bauer K, Esquilin IO, Cornier AS, Thomas SJ, Quintero Del Rio AI, Bertran-Pasarell J, Morales Ramirez JO, Diaz C, Carlo S, Eckels KH, et al. A Phase II, randomized, safety and immunogenicity trial of a re-derived, live-attenuated dengue virus vaccine in healthy children and adults living in Puerto Rico. Am J Trop Med Hyg. 2015;93(3):441–53. doi:10.4269/ajtmh.14-0625.
- Anderson KB, Gibbons RV, Edelman R, Eckels KH, Putnak RJ, Innis BL, Sun W. Interference and facilitation between dengue serotypes in a tetravalent live dengue virus vaccine candidate. J Infect Dis. 2011;204(3):442–50. doi:10.1093/infdis/jir279.
- Halstead SB. Identifying protective dengue vaccines: guide to mastering an empirical process. Vaccine. 2013;31(41):4501–07. doi:10.1016/j.vaccine.2013.06.079.
- Rothman AL, Currier JR, Friberg HL, Mathew A. Analysis of cell-mediated immune responses in support of dengue vaccine development efforts. Vaccine. 2015;33(50):7083–90. doi:10.1016/j.vaccine.2015.09.104.
- Watanaveeradej V, Simasathien S, Mammen MP Jr., Nisalak A, Tournay E, Kerdpanich P, Samakoses R, Putnak RJ, Gibbons RV, Yoon IK, et al. Long-term safety and immunogenicity of a tetravalent live-attenuated dengue vaccine and evaluation of a booster dose administered to healthy Thai children. Am J Trop Med Hyg. 2016;94(6):1348–58. doi:10.4269/ajtmh.15-0659.
- Maecker HT, Dunn HS, Suni MA, Khatamzas E, Pitcher CJ, Bunde T, Persaud N, Trigona W, Fu TM, Sinclair E, et al. Use of overlapping peptide mixtures as antigens for cytokine flow cytometry. J Immunol Methods. 2001;255:27–40.
- Lindow JC, Borochoff-Porte N, Durbin AP, Whitehead SS, Fimlaid KA, Bunn JY, Kirkpatrick BD. Primary vaccination with low dose live dengue 1 virus generates a proinflammatory, multifunctional T cell response in humans. PLoS Negl Trop Dis. 2012;6(7):e1742. doi:10.1371/journal.pntd.0001742.
- Mangada MM, Ennis FA, Rothman AL. Quantitation of dengue virus specific CD4+ T cells by intracellular cytokine staining. J Immunol Methods. 2004;284:89–97.
- Weiskopf D, Angelo MA, Bangs DJ, Sidney J, Paul S, Peters B, De Silva AD, Lindow JC, Diehl SA, Whitehead S, et al. The human CD8+ T cell responses induced by a live attenuated tetravalent dengue vaccine are directed against highly conserved epitopes. J Virol. 2015;89(1):120–28. doi:10.1128/JVI.02129-14.
- Guy B, Nougarede N, Begue S, Sanchez V, Souag N, Carre M, Chambonneau L, Morrisson DN, Shaw D, Qiao M, et al. Cell-mediated immunity induced by chimeric tetravalent dengue vaccine in naive or flavivirus-primed subjects. Vaccine. 2008;26(45):5712–21. doi:10.1016/j.vaccine.2008.08.019.
- Akondy RS, Monson ND, Miller JD, Edupuganti S, Teuwen D, Wu H, Quyyumi F, Garg S, Altman JD, Del Rio C, et al. The yellow fever virus vaccine induces a broad and polyfunctional human memory CD8+ T cell response. J Immunol. 2009;183(12):7919–30. doi:10.4049/jimmunol.0803903.
- Chu H, George SL, Stinchcomb DT, Osorio JE, Partidos CD. CD8+ T-cell responses in flavivirus-naive individuals following immunization with a live-attenuated tetravalent dengue vaccine candidate. J Infect Dis. 2015;212(10):1618–28. doi:10.1093/infdis/jiv258.
- Duangchinda T, Dejnirattisai W, Vasanawathana S, Limpitikul W, Tangthawornchaikul N, Malasit P, Mongkolsapaya J, Screaton G. Immunodominant T-cell responses to dengue virus NS3 are associated with DHF. Proc Natl Acad Sci U S A. 2010;107(39):16922–27. doi:10.1073/pnas.1010867107.
- Rivino L, Kumaran EA, Jovanovic V, Nadua K, Teo EW, Pang SW, Teo GH, Gan VC, Lye DC, Leo YS, et al. Differential targeting of viral components by CD4+ versus CD8+ T lymphocytes in dengue virus infection. J Virol. 2013;87(5):2693–706. doi:10.1128/JVI.02675-12.
- Amanna IJ, Carlson NE, Slifka MK. Duration of humoral immunity to common viral and vaccine antigens. N Engl J Med. 2007;357(19):1903–15. doi:10.1056/NEJMoa066092.
- Dorigatti I, Aguas R, Donnelly CA, Guy B, Coudeville L, Jackson N, Saville M, Ferguson NM. Modelling the immunological response to a tetravalent dengue vaccine from multiple phase-2 trials in Latin America and South East Asia. Vaccine. 2015;33(31):3746–51. doi:10.1016/j.vaccine.2015.05.059.
- Thomas SJ. Dengue human infection model: re-establishing a tool for understanding dengue immunology and advancing vaccine development. Hum Vaccin Immunother. 2013;9(7):1587–90. doi:10.4161/hv.24188.
- Schmidt AC, Lin L, Martinez LJ, Ruck RC, Eckels KH, Collard A, De la Barrera R, Paolino KM, Toussaint JF, Lepine E, et al. Phase 1 randomized study of a tetravalent dengue purified inactivated vaccine in healthy adults in the United States. Am J Trop Med Hyg. 2017;96(6):1325–37. doi:10.4269/ajtmh.16-0634.
- Eckels KH, Dubois DR, Putnak R, Vaughn DW, Innis BL, Henchal EA, Hoke CH Jr. Modification of dengue virus strains by passage in primary dog kidney cells: preparation of candidate vaccines and immunization of monkeys. Am J Trop Med Hyg. 2003;69:12–16.
- Moris P, van der Most R, Leroux-Roels I, Clement F, Dramé M, Hanon E, Leroux-Roels GG, Van Mechelen M. H5N1 influenza vaccine formulated with AS03A induces strong cross-reactive and polyfunctional CD4 T-cell responses. J Clin Immunol. 2011;31(3):443–54. doi:10.1007/s10875-010-9490-6.
- Díez-Domingo J, Garcés-Sanchez M, Baldó JM, Planelles MV, Ubeda I, JuBert A, Marés J, Moris P, Garcia-Corbeira P, Dramé M, et al. Immunogenicity and safety of H5N1 A/Vietnam/1194/2004 (Clade 1) AS03-adjuvanted prepandemic candidate influenza vaccines in children aged 3 to 9 years: a phase II, randomized, open, controlled study. Pediatr Infect Dis J. 2010;29(6):e35–e46. doi:10.1097/INF.0b013e3181daf921.
- Idoko OT, Owolabi OA, Owiafe PK, Moris P, Odutola A, Bollaerts A, Ogundare E, Jongert E, Demoitié MA, Ofori-Anyinam O, et al. Safety and immunogenicity of the M72/AS01 candidate tuberculosis vaccine when given as a booster to BCG in Gambian infants: an open-label randomized controlled trial. Tuberculosis (Edinb). 2014;94(6):564–78. doi:10.1016/j.tube.2014.07.001.
- Garcia-Sicilia J, Arístegui J, Omeñaca F, Carmona A, Tejedor JC, Merino JM, García-Corbeira P, Walravens K, Bambure V, Moris P, et al. Safety and persistence of the humoral and cellular immune responses induced by 2 doses of an AS03-adjuvanted A(H1N1)pdm09 pandemic influenza vaccine administered to infants, children and adolescents: two open, uncontrolled studies. Hum Vaccin Immunother. 2015;11(10):2359–69. doi:10.1080/21645515.2015.1063754.
- Precopio ML, Butterfield TR, Casazza JP, Little SJ, Richman DD, Koup RA, Roederer M. Optimizing peptide matrices for identifying T-cell antigens. Cytometry Part A. 2008;73A(11):1071–78. doi:10.1002/cyto.a.20646.