ABSTRACT
Background: Infections with respiratory syncytial virus (RSV) cause significant morbidity and hospitalization in older adults. We studied the humoral, mucosal and B cell responses of an investigational adjuvanted RSV sF vaccine, MEDI7510, in older adults.
Methods: In a substudy of a randomized (1:1), double-blind, placebo-controlled study of MEDI7510 in adults ≥60 years of age, we collected blood and nasal secretions at days 0, 8, 29, 91 and 180 post-vaccination to measure F-specific IgG and IgA antibodies by ELISA, and plasmablasts and memory B cells by IgA/IgG dual-color fluorospot.
Results: The 27 vaccine- and 18 placebo-recipients had a mean age of 73 years and included 24 women. Among vaccinees, 93% had significant increases in F-specific plasma IgG 85% had increased plasma IgA; 74% had increased nasal IgG and 26% nasal IgA; 93% had IgG and 89% IgA plasmablasts on Day 8 post-immunization; and 82% had IgG and 7.4% IgA memory B cell responses to the vaccine. Vaccinees <70 years of age and women had the highest responses to the vaccine.
Conclusions: This adjuvanted vaccine generated robust humoral immune responses in older adults, including RSV F-specific systemic and mucosal antibodies and memory B cells. Nevertheless, age ≥70 years was associated with decreased immunogenicity of the adjuvanted vaccine.
Introduction
Respiratory syncytial virus (RSV) is recognized as an important cause of significant respiratory illness in older adults,Citation1-Citation3 particularly in those with underlying chronic cardiac and pulmonary disease.Citation4,Citation5 Efforts to develop an RSV vaccine are in progress.Citation6,Citation7 Although there are no established correlates of protection to direct vaccine development, both serum neutralizing antibodies and nasal IgA have been associated with a reduced likelihood of developing serious RSV infection in this population.Citation8-Citation13 Importantly, a protective role for serum antibodies has not been universally demonstrated. However, in some studies that failed to correlate serum neutralizing titers with protection, nasal IgA or IgG titers showed protective effects.Citation11,Citation14 In addition, T-cell mediated immunity (CMI) is thought to play an essential role in ending established RSV infections. This is suggested by animal models, studies in children and by the potential for severe or fatal RSV infection in patients with CMI defects.Citation15-Citation18
Developing an effective vaccine for older individuals can rely on boosting pre-existing RSV responses and, therefore, is not at risk of priming for enhanced disease. However, the difficulty arises from reduced magnitude and persistence of immune responses in proportion to increasing age in older individuals.Citation19-Citation23 To address this obstacle, some investigational RSV vaccines have included adjuvants to enhance immunogenicityCitation6. MEDI7510 contains soluble RSV F protein (F) and glycopyranosyl lipid A (GLA), a toll-like receptor 4 (TLR4) agonist in an oil-in-water stable emulsion (SE). This vaccine induced RSV neutralizing antibodies and interferon γ-producing T-cells in murine and non-human primate models with significant enhancement of immune responses resulting from the adjuvant inclusion.Citation24,Citation25 Phase 1 studies in individuals ≥60-year old demonstrated safety, anti-F IgG, RSV neutralizing antibodies, palivizumab-competing antibodies and RSV-specific CMI, and confirmed the added value of the adjuvant.Citation26,Citation27
A double-blind phase 2b placebo-controlled study of MEDI7510 in 1900 subjects ≥60-years old was undertaken to assess the efficacy of this vaccineCitation28. MEDI7510 again demonstrated robust humoral and CMI RSV-specific responses, but lacked efficacy.
In this report, we present a single-site substudy of the mucosal and systemic immune responses following immunization with MEDI7510.
Results
Demographic characteristics of the study population
The sub-study enrolled all the participants at the Denver study site, including 27 vaccine- and 18 placebo-recipients (). The two groups were balanced with respect to age and race, but there were 37% women in the vaccine group and 78% in the placebo.
Table 1. Demographic characteristics of the participants in the sub-study
F-specific systemic antibody responses to the vaccine
All subjects had pre-existing F-specific IgG antibodies detected at baseline, which did not appreciably differ between treatment groups. Vaccinees had statistically significant increases in systemic F-specific IgA and IgG antibody concentrations from baseline to day 29 post-vaccination (10.7- and 8.1-fold, respectively, p ≤ 0.001), whereas placebo-recipients did not ( and S11). Using a threshold of ≥3-fold increase to define sero-response, 85% of vaccinees had F-specific IgA responses and 93% had F-specific IgG responses, while placebo-recipients showed no responses (Table S1).
Figure 1. IgG and IgA systemic antibody responses to an adjuvanted investigational RSV sF vaccinee. Data were derived from 27 vaccinee and 18 placebo-recipients 60 to 88 years of age. The boxplots and whiskers represent the minimum, 1st quartile, median, 3rd quartile and maximum values. Panel A shows individual F-specific IgA plasma antibody responses. The dotted line represents the lower limit of quantitation (LLOQ) (68.6 ng/ml). Day 29 and 91 results were significantly higher than baseline in vaccinees (p ≤ 0.001), but not in placebo recipients. Panel B shows individual F-specific IgG responses in vaccine and placebo-recipients before (day 1) and after vaccination (day 29). The F-specific IgG concentrations were >LLOQ (0.66 Ab U/ml) for all subjects. Day 29 results were significantly higher than baseline in vaccinees (p ≤ 0.001), but not in placebo recipients
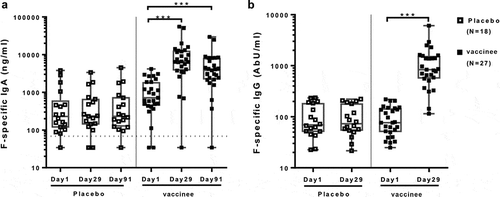
The F-specific IgG antibody response to the vaccine consisted of IgG1 and IgG3, which had 9.7- and 3.6-fold increases, respectively, from pre- to post-immunization (p ≤ 0.0001; ). F-specific IgG2 and IgG4 antibodies did not change with vaccination. F-specific IgG significantly correlated with F-specific IgG1 concentrations before and after vaccination (r ≥ 0.67; FDR-adjusted p ≤ 0.004; Table S2), but not with F-specific IgG3, indicating that IgG1 was the main component of the vaccine-induced IgG response.
Figure 2. IgG subclass antibody responses to an adjuvanted investigational RSV sF vaccine. Data were derived from 27 vaccine and 18 placebo-recipients 60 to 88 years of age. The boxplots and whiskers represent the minimum, 1st quartile, median, 3rd quartile and maximum values. Dotted lines represent the LLOQ of 5250, 120, 75, and 20 ng/mL for F-specific IgG1, IgG2, IgG3 and IgG4 assays, respectively. Panel A: IgG1; Panel B: IgG2; Panel C: IgG3; Panel D: IgG4. IgG1 and IgG3 were significantly higher at day 29 compared with baseline in vaccinees (p ≤ 0.001), but not in placebo recipients
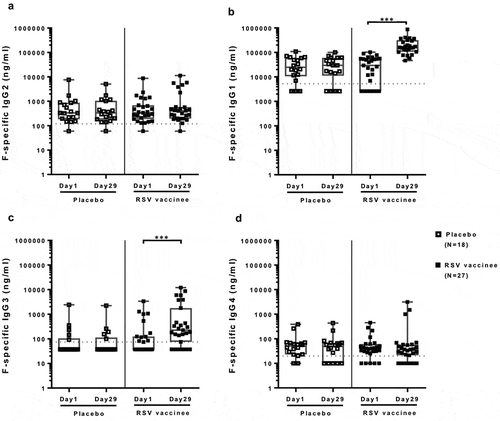
We investigated the effect of age and gender on vaccine-induced antibodies (Figures S1 and S2). Compared with younger (<70 years), older vaccinees had significantly lower F-specific IgA (p = 0.04), marginally lower F-specific IgG (p = 0.06) and lower F-specific IgA and IgG responder rates (100% IgA and IgG vs. 78% IgA and 89% IgG; Table S1). Women had higher F-specific IgG levels (p = 0.002) and responder rates (100% vs. 88%) than men, but similar F-specific IgA responses.
F-specific nasal antibody responses
Vaccine-recipients had 2.1-fold nasal F-specific IgA and 4.3-fold IgG increases from pre- to post-vaccination, while placebo-recipients did not (, S9 and S11). The nasal F-specific antibodies reached a maximum observed level at day 8, but levels were sustained through day 29 post-vaccination. Using a threshold of ≥3-fold increase over baseline in the first 29 days post-vaccination, 26% of vaccine-recipients had nasal F-specific IgA and 74% IgG responses (). Nasal F-specific IgG increased at the day 29 visit in one placebo-recipient who was diagnosed with RSV upper respiratory tract infection by PCR at the same visit.
Table 2. Frequency of participants with immune responses to the candidate vaccine
Figure 3. IgG and IgA nasal antibody responses to an adjuvanted investigational RSV sF vaccine. Data were derived from 27 vaccine and 18 placebo-recipients 60 to 88 years of age. The graphs show geometric means and 95% confidence intervals. The LLOQ to ULOQ range was 0.685–500 ng/mL for F-specific IgA, 4.1–3000 ng/mL for F-specific IgG, 13.7–10000 ng/mL for total IgA and 164–120000 ng/mL for total IgG, with values<LLOQ imputed at LLOQ/2 and values >LLOQ imputed at 2*ULOQ. Panel A: F-specific IgA; Panel B: F-specific IgG; Panel C: Total IgA; Panel D: Total IgG. F-specific IgG and IgA were significantly higher at days 8 and 29 compared with baseline in vaccinees (p ≤ 0.004), but not in placebo recipients. There were no changes in total nasal IgG or IgA after vaccination compared with baseline in either group
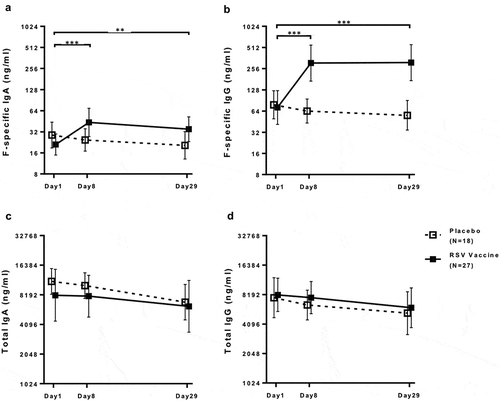
Nasal F-specific IgA and IgG levels significantly correlated pre-vaccination (r = 0.5; FDR p = 0.005; Table S2), but only marginally post-vaccination. Nasal F-specific IgA also correlated with with plasma F-specific IgA post-vaccination (r = 0.61; FDR p = 0.01). Nasal F-specific IgG correlated with serum F IgG only pre-vaccination (r = 0.51; FDR p < 0.003).
Compared with younger, older vaccinees had lower nasal F-specific IgA and IgG concentrations and responder rates (Figure S3; Table S1). There were no gender differences in the nasal F-specific antibody responses to the vaccine (Figure S4).
B cell responses
F-specific IgA and IgG plasmablasts evaluated on fresh peripheral blood mononuclear cells (PBMC) were nearly undetectable before and significantly increased at Day 8 post-immunization in vaccinees (p < 0.0001 for both IgA and IgG; ), but not in controls. Using a 2.5-fold increase threshold, 90% of vaccinees had F-specific IgA and 93% IgG plasmablast responses, but controls did not (Table S1). The magnitude of F-specific IgA and IgG plasmablast responses did not correlate with F-specific IgA or IgG antibodies in the blood compartment (Table S2). Neither age nor gender had significant effects on the plasmablast response to the vaccine (Figures S5 and S6).
Figure 4. IgG and IgA plasmablast responses to an adjuvanted investigational RSV sF vaccine. Data were derived from 27 vaccine and 18 placebo-recipients 60 to 88 years of age. F-specific spot forming counts per million (SFC/106) cells were determined by subtracting the average counts in PBS wells from the average counts in RSV sF wells.The boxplots and whiskers represent the minimum, 1st quartile, median, 3rd quartile and maximum values. Dotted lines represent the LLOQ (3 SFC/106 cells for all assays). Values <LLOQ were imputed at LLOQ-1. Panel A: F-specific IgA; Panel B: F-specific IgG; Panel C: Total IgA; Panel D: Total IgG. F-specific IgA and IgG plasmablasts were significantly higher after immunization compared with baseline in vaccinees (p < 0.0001), but not in placebo recipients. There were no significant changes in total IgA or IgG plasmablasts after vaccination in either treatment group
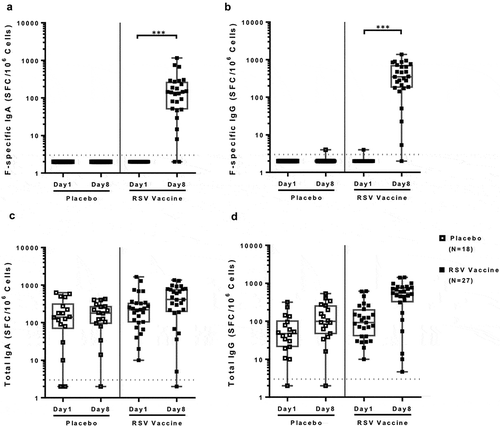
F-specific IgG memory B cells measured in cryopreserved PBMC were detected before and increased by 4.9-fold after immunization in vaccinees. At day 181 they remained significantly higher than baseline ( and S10). Twenty-two of 27 (82%) vaccinees and 1 of 18 (6%) controls were IgG-responders. F-specific IgA memory B cells did not significantly increase in either group. Two of 27 (7.4%) vaccine-recipients and no placebo-recipients had vaccine-induced F-specific IgA memory B cell responses defined as ≥4.5-fold rise over baseline (Table S1).
Figure 5. F-specific IgG and IgA memory B cell responses to an adjuvanted investigational RSV sF vaccine. Data were derived from 27 vaccine and 18 placebo-recipients 60 to 88 years of age. The graphs show geometric means and 95% confidence intervals. Dotted lines represent the LLOQ (2 SFC/106 cells for IgA; 14 SFC/106 cells for IgG). Values <LLOQ were imputed at LLOQ-1. Panel A: F-specific IgA; Panel B: F-specific IgG; Panel C: Total IgA; Panel D: Total IgG. F-specific IgG memory B cells were significantly higher at days 29, 91 and 181 compared with baseline in vaccinees (p ≤ 0.01), but not in placebo recipients. There were no significant changes in F-specific IgA memory B cells in either group
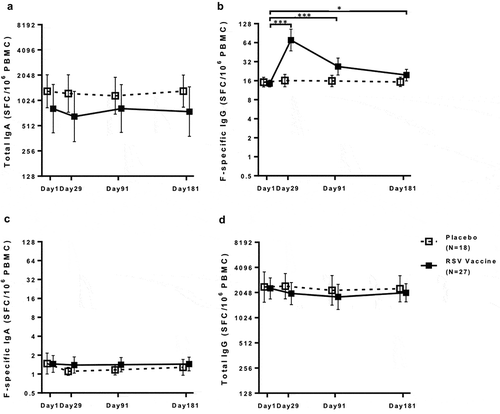
Only F-specific IgA memory B cells correlated with F-specific IgA plasma and nasal antibodies (r ≥ 0.54; FDR p ≤ 0.04). F-specific IgG memory B cells did not correlate with F-specific IgG antibodies in the blood or nose.
Older vaccinees had significantly lower vaccine-induced F-specific IgG memory B cells (p ≤ 0.04; Figure S7) and responder rates (72% vs. 100%) than younger vaccinees. F-specific IgA memory B cells were significantly lower at Day 29 in older vaccinees. Gender did not affect vaccine-induced F-specific IgA or IgG memory B cells (Figure S8).
Discussion
The goal of this study was to evaluate mucosal and systemic antibody and B cell responses generated by the investigational adjuvanted RSV sF vaccine, MEDI7510, in older adults. Limited human immunogenicity data consisting of microneutralizing, palivizumab-competitive and anti-F IgG antibodies and gamma interferon ELISPOT responses were generated during Phase 1 and Phase 2 studies, and the contribution of adjuvant to both cellular and humoral immunity had been demonstrated.Citation26,Citation27 Our data extends this information by demonstrating that the vaccine was highly immunogenic and generated systemic and nasal F-specific IgG and IgA responses, confirming the anticipated vaccine immunogenicity. Despite inclusion of the adjuvant, however, age had a significant effect on F-specific immunoglobulin responses in the blood and nasal mucosal compartments and on memory B-cell responses.
The importance of mucosal antibodies in protection from respiratory illness, including RSV, has been previously reported.Citation12,Citation13 We demonstrated that the sF protein combined with the GLA adjuvant in the vaccine generated significant IgA (25.9% of recipients) and IgG (74.1% of recipients) antibodies in the nasal mucosa, which were detected as soon as 8 days post-immunization and persisted for ≥29 days. These findings are in agreement with a previous study which showed that the GLA adjuvant, but not R848, could enhance mucosal IgA production to coformulated HIV gp140 antigens given intranasally compared with antigen aloneCitation29. Both T-dependent and T-independent (TLR4-mediated) mechanisms of B cell class switching to IgA isotypes are likely with this adjuvant. While most IgA induction takes place in mucosal associated lymphoid tissue, IgA class switching can occur in other lymphoid tissue given the appropriate induced cytokine milieu, and circulating IgA plasmablasts expressing CCR10 can home to nasopharyngeal associated lymphoid tissues (NALT) where they could become sources of nasal IgACitation30. We observed a post-vaccination increase in both circulating IgA and IgG plasmablasts at day 8. Consistent with the intramuscular vaccine administration route, systemic IgG antibodies were boosted better than nasal IgG or IgA antibodies or systemic IgA by this vaccine. Systemic F-specific antibodies remained elevated for ≥90 days post-immunization, indicating the durability of the vaccine-induced response. A previous phase 1 trial had demonstrated that systemic F-specific IgG levels persisted at elevated levels for 1 yearCitation27.
Correlations between nasal and plasma F-specific IgA antibody concentrations were identified only after vaccination. This demonstrated that the vaccine was responsible for both local and systemic increases in F-specific IgA antibodies, since generally IgA antibodies do not transudate from the blood compartment into the mucosal secretions. The ratio of total IgA/IgG in nasal secretions was much higher than the ratio of total IgA/IgG1 in the blood, suggesting that IgA nasal secretions were not simply transudate as would be expected for IgG nasal secretions. It was surprising, however, that in our study nasal and systemic F-specific IgG antibody concentrations were not correlated. In contrast to the IgA antibodies, which were performed in the same laboratory, the nasal and systemic IgG antibodies were performed using different assay formats and in different laboratories, and technical differences may have contributed to the lack of correlation.
Despite all subjects presumably having been previously exposed to RSV, memory B cell responses were limited at baseline to IgG and did not include IgA. Failure to detect RSV-specific IgA memory B cells following RSV challenge has been previously reported, with the suggestion that this virus could specifically impair the generation of IgA memoryCitation11. Moreover, F-specific IgG memory B cells increased in all vaccinees 29 days after immunization and persisted for ≥181 days, but only 2 of 27 vaccinees demonstrated a significant increase in F-specific IgA memory B cells . Of note, total IgA memory B cells were detected in all subjects. Taken together, these data seem to support a generalized deficit in RSV-specific IgA B cell memory formationCitation11. However, the F-specific IgA memory B cells correlated with the nasal and systemic F-specific IgA concentrations after, but not before, vaccination, indicating that a quantifiable, albeit small, number of F-specific IgA memory B cells were generated or boosted by the vaccine.
The plasmablast and memory B cell responses to the vaccine did not correlate with each other. This was not unexpected given that these two types of responses are independently generatedCitation31. In contrast, the long-lived plasma cells, which are responsible for systemic antibody production, and the memory B cells are similarly regulatedCitation32. Nevertheless, the F-specific IgG memory B cells did not correlate with the systemic or nasal F-specific IgG.
Among older adults, individuals ≥70 years of age have lower responses to vaccines than those 60 to <70. This was clearly shown for the live attenuated zoster vaccine which has significantly lower immunogenicity and efficacy in individual 60 to <70 years of age, compared with 70 to <80.Citation33,Citation34 In contrast, a new adjuvanted recombinant zoster vaccine showed very similar immunogenicity and efficacy in these two age groups.Citation35-Citation37 In this study, adults >70 years of age had lower responses to the vaccine, including local and systemic F-specific antibodies and F-specific memory B cells. This identifies an area in which the vaccine could be improved and the importance of studying vaccine responses in the very elderly. Most of the antibody responses to sF were slightly higher in women compared with men. However, the plasmablast and memory B cell responses were similar. Comparisons of immunogenicity of other vaccines in males and females have generated conflicting results, but some authors found a negative effect of testosterone on responses to influenza vaccine.Citation38,Citation39
Limitations of this study included a relatively small sample size, particularly for subgroup analyses, and unequal randomization to placebo and vaccine groups at the study site, because overall randomization was not stratified by site. We had only one placebo recipient who had RSV endpoints at our site, we were unable to assess differences in responses between those who did and did not develop RSV disease. Other participants may have had asymptomatic infections among placebo and vaccine recipients that were not diagnosed by PCR. However, there were no asymptomatic seroconversions in the placebo group and no increases in antibody titers after day 7 post-vaccination in the vaccine group to suggest that asymptomatic infections may have confounded the data. In addition, because all subjects received adjuvanted vaccine, we were unable to demonstrate the contribution of the adjuvant to immune responses; however, the observed responses were consistent with a Th1-biased immune response that would be expected from the inclusion of a TLR-4 agonist such as GLA.Citation24,Citation25 The nasal antibody results were enriched by the use of absorbent paper for the collection of nasal fluid, which was simple and well-tolerated. This method, unlike nasal wash, does not result in sample dilution and does not require adjustments.Citation40,Citation41 In addition, we reported the results using human IgG and IgA quantitative standards, which will allow others to compare their results with ours in future studies.
The results of this sub-study and of the systemic antibody responses measured in the parent phase 2b study showed robust immunogenicity of MEDI7510, and do not explain the failure of the vaccine to protect older adults against RSV infectionCitation42. The failure of the vaccine did not correlate with the age of the vaccinees. Previous reports also showed T cell responses were generated by this vaccineCitation26. Additional data are being generated to determine characteristics of the antibodies generated by the vaccine, including binding site on the F protein and the ability to cross-neutralize circulating RSV strains. While both pre-fusion and post-fusion conformations of the RSV F protein can induce protective neutralizing antibodies in animal models, recent data suggest that the pre-fusion conformation may be a more potent immunogen in animal models and that most of the F-specific antibodies in unvaccinated adults are directed at epitopes displayed by the pre-fusion conformation.Citation43,Citation44 Further studies are warranted combining RSV F proteins with different amino acid sequences and/or conformations with GLA-SE, which showed excellent adjuvant characteristics.
Participants and methods
Vaccine
The investigational RSV vaccine MEDI7510 consisted of 120 µg of RSV F protein from the A2 strain in the postfusion configuration and 5 µg of GLA-SE in 0.5 mL total volume for intramuscular administrationCitation42.
Clinical trial
This was conducted under a University of Colorado site-specific amendment to the parent randomized, double-blind, phase 2b study of MEDI7510 (NCT02508194). The substudy was approved by the Colorado Multiple Instititutions Review Board. Subjects were independent-living, immune-competent and aged ≥60 years of age who provided written informed consent. Subjects received unadjuvanted, standard dose inactivated influenza vaccine in one arm and were randomized 1:1 to receive saline placebo or MEDI7510 vaccine in the other arm. Randomization was stratified by age (≤75 and >75 years). Clinical endpoints and testing for RSV using RT-PCR on mid-turbinate swabs have been previously reportedCitation42. This study used blood and nasal fluid obtained at before vaccination (day 1) and days 8, 29, 91 and 181.
Sample collection and processing
For nasal fluid, 8 mm x 40 mm strips of Whatman™ quantitative filter paper (Grade 42, #1442–5551) were inserted approximately one inch into each nostril and left in place for 5 minutes. Upon removal, both strips were placed in tubes with 3 mL cold 0.1M Tris pH 7.4 containing 0.3% bovine serum albumin (BSA), 0.01% sodium azide and 0.002% Tween. The tubes were centrifuged at 500xg for 10 minutes, and supernatants were frozen. Plasma obtained from heparinized whole blood was frozen at −80ºC prior to use. PBMC separated on Histopaque-1077 (Sigma, cat# H8889) were set aside in phosphate- buffered saline (PBS) for plasmablast fluorospots or cryopreserved in fetal bovine serum (FBS) containing 10% dimethylsulfoxide.
Nasal IgG and IgA ELISA
Immulon II HB microwells (Fisher, cat# 14-245-81) were coated overnight with RSV sF protein at 10 µg/mL in PBS, anti-IgG antibody (MabTech Human IgG ELISA cat# 3850-1AD) at 2 µg/mL or anti-IgA antibody (MabTech Human IgA ELISA cat# 3830-1AD) at 1 µg/mL. After blocking, nasal fluid diluted in incubation buffer (PBS containing 0.05% Tween and 0.1% BSA) at 1:10 for RSV F-specific IgG; 1:5 for F-specific IgA; 1:400 for total IgG; and 1:100 for total IgA, were incubated, along with human IgG and IgA standards included in the kit, for 2 hours at room temperature. After washing, IgG wells were incubated with anti-IgG-ALP (MabTech) followed by pNPP (ImmunoChemistry Technologies, cat# 6279). IgA wells were incubated with anti-IgA-biotin (MabTech, cat# 3840-4-250) followed by streptavidin-AP (MabTech, cat# 3310-8) and pNPP. Plates were read at 405 nm using a Thermo Multiskan FC reader (ThermoFisher Scientific). The concentration of antibodies bound to RSV F-protein was interpolated on the corresponding IgG or IgA nonlinear regression variable slope sigmoidal dose response using Prism 7.0 software (GraphPad Software Inc) and reported in ng/mL.
Plasma IgA ELISA
Immulon II HB microwells were coated overnight with RSV F-protein at 10 µg/mL in PBS or anti-IgA antibody (MabTech Human IgA ELISA cat#3860-1AD) at 2 µg/mL. After blocking, plasma diluted 1:100 in incubation buffer was added to the wells along with human IgA standards included in the kit and incubated for 2 hours at room temperature. After washing, wells were incubated with anti-IgA-ALP (MabTech) followed by pNPP. Plates were read and the amount of bound antibody was calculated as above.
Serum IgG
Serum F-specific IgG were determined using a multiplexed assay based on the Meso Scale Discovery platform as previously describedCitation45. F-specific antibody units per mL (Ab U/mL) are reported relative to a pooled human sera reference standard arbitrarily defined as 100 Ab U/mL.
Plasma IgG subclass ELISAs
Immulon II HB microwells were coated overnight with RSV F-protein at 10 µg/mL in PBS, along with IgG subclass calibrators and controls (CellSciences kit M1551). After blocking, wells were incubated with plasma diluted in incubation buffer (IgG1 1:1000,1:3000; IgG2 1:10; IgG3 1:50; IgG4 1:10) for one hour. After washing, wells were incubated with mouse-HRP (Thermo/Invitrogen) antibodies anti-IgG1 at 1:1000 and anti-IgG2, IgG3 and IgG4 at 1:500. After washing, wells were incubated with ABTS (Southern Biotech). Bound antibodies were read and quantitated as above.
Plasmablast fluorospots
96-well Multiscreen HTS™ IPFL membrane plates (MabTech IgG/IgA FluoroSpot kit, Cat. FS-05R06G-10) were coated either with total anti-IgG and anti-IgA antibodies (MabTech) per the manufacturer’s instructions; RSV F-protein at 10 µg/mL; or PBS and incubated overnight at 4°C. After blocking the wells with B-cell medium consisting of RPMI1640 containing 10% FBS, 100 µg/mL penicillin/streptomycin and 10 mM HEPES buffer for ≥30 minutes, 100µL of PBMC in B-cell medium at 2.5 × 106 cells/mL were added to each well. Plates were incubated at 37°C for 20 hours and developed as per manufacturer instructions. Spots were counted with a Cellular Technology Limited (CTL) ImmunoSpot analyzer.
Memory B cell fluorospots
Cryopreserved PBMC were thawed, resuspended at 1 × 106 cells/mL in B-cell medium and stimulated with 1 µg/mL R848 (MabTech) and 100 ng/mL recombinant human interleukin-2 (MabTech) for 7 days at 37°C. Stimulated PBMC at 5 × 106 cells/mL in B-cell medium were added to plates prepared as above at 5 × 105 cells/well for PBS control and RSV F-specific IgG/IgA or 5 × 104 cells/well for the total IgG/IgA. After overnight incubation at 37°C, plates were developed and analyzed as above.
Statistical analysis
The geometric mean concentration of antibody or B cell levels and geometric fold-increases from baseline were used to summarize vaccine responses at each visit. A general linear model including pre-vaccination levels, gender and age group as covariates was applied to adjust for imbalances and potential confounding effects of these covariates on the outcome measures. Vaccine responder criteria were established considering assay variability in positive control samples and between placebo pre- and post-vaccination responses. Vaccine responder thresholds of 4.5-, 2.5- and 3-fold rise from baseline for memory B-cell F IgA; memory B-cell IgG and plasmablasts; and antibodies, respectively, achieved <5% false positive responder rates. Correlations were evaluated using Spearman correlation coefficient. P values were adjusted for multiple comparisons using the false discovery rate (FDR). Analyses used SAS 9.4 (SAS Institute).
Disclosure of potential conflicts of interest
SLL, LY, MTE, and JF are employees of MedImmune and may own stock or stock options in AstraZeneca, its parent company.
Supplemental Material
Download MS Word (11.8 MB)Acknowledgments
The authors thank Deirdre Ferrall and David Claypool for assistance in conducting the study. GLA-SE was licensed from Immune Design Corporation (Seattle, WA, USA) pursuant to an existing agreement.
Supplementary material
Supplemental data for this article can be accessed on the publisher’s website.
Additional information
Funding
References
- Falsey AR, Hennessey PA, Formica MA, Cox C, Walsh EE. Respiratory syncytial virus infection in elderly and high-risk adults. N Engl J Med. 2005;352:1749–59. doi:10.1056/NEJMoa043951.
- Thompson WW, Shay DK, Weintraub E, Brammer L, Cox N, Anderson LJ, Fukuda K. Mortality associated with influenza and respiratory syncytial virus in the United States. JAMA. 2003;289:179–86.
- Widmer K, Zhu Y, Williams JV, Griffin MR, Edwards KM, Talbot HK. Rates of hospitalizations for respiratory syncytial virus, human metapneumovirus, and influenza virus in older adults. J Infect Dis. 2012;206:56–62. doi:10.1093/infdis/jis309.
- Lee N, Lui GC, Wong KT, Li TC, Tse EC, Chan JY, Yu J, Wong SSM, Choi KW, Wong RYK, et al. High morbidity and mortality in adults hospitalized for respiratory syncytial virus infections. Clin Infect Dis. 2013;57:1069–77. doi:10.1093/cid/cit471.
- Walsh EE, Peterson DR, Falsey AR. Risk factors for severe respiratory syncytial virus infection in elderly persons. J Infect Dis. 2004;189:233–38. doi:10.1086/380907.
- Guvenel AK, Chiu C, Openshaw PJ. Current concepts and progress in RSV vaccine development. Expert Rev Vaccines. 2014;13:333–44. doi:10.1586/14760584.2014.878653.
- Higgins D, Trujillo C, Keech C. Advances in RSV vaccine research and development - a global agenda. Vaccine. 2016;34:2870–75. http://www.who.int/immunization/sage/meetings/2016/april/1_Advances_RSV_Vaccine_Research_Development_A_Global_Agenda.pdf.
- Falsey AR, Walsh EE. Relationship of serum antibody to risk of respiratory syncytial virus infection in elderly adults. J Infect Dis. 1998;177:463–66.
- Hall CB, Walsh EE, Long CE, Schnabel KC. Immunity to and frequency of reinfection with respiratory syncytial virus. J Infect Dis. 1991;163:693–98.
- Piedra PA, Jewell AM, Cron SG, Atmar RL, Glezen WP. Correlates of immunity to respiratory syncytial virus (RSV) associated-hospitalization: establishment of minimum protective threshold levels of serum neutralizing antibodies. Vaccine. 2003;21:3479–82.
- Habibi MS, Jozwik A, Makris S, Dunning J, Paras A, DeVincenzo JP, de Haan CAM, Wrammert J, Openshaw PJM, Chiu C. Impaired antibody-mediated protection and defective IgA B-cell memory in experimental infection of adults with respiratory syncytial virus. Am J Respir Crit Care Med. 2015;191:1040–49. doi:10.1164/rccm.201412-2256OC.
- Bagga B, Cehelsky JE, Vaishnaw A, Wilkinson T, Meyers R, Harrison LM, Roddam PL, Walsh EE, DeVincenzo JP. Effect of preexisting serum and mucosal antibody on experimental respiratory syncytial virus (RSV) challenge and infection of adults. J Infect Dis. 2015;212:1719–25. doi:10.1093/infdis/jiv281.
- Walsh EE, Falsey AR. Humoral and mucosal immunity in protection from natural respiratory syncytial virus infection in adults. J Infect Dis. 2004;190:373–78. doi:10.1086/421524.
- Vissers M, Ahout IM, de Jonge MI, Ferwerda G. Mucosal IgG levels correlate better with respiratory syncytial virus load and inflammation than plasma IgG levels. Clin Vaccine Immunol. 2015;23:243–45. doi:10.1128/CVI.00590-15.
- Geevarghese B, Weinberg A. Cell-mediated immune responses to respiratory syncytial virus infection: magnitude, kinetics, and correlates with morbidity and age. Hum Vaccin Immunother. 2014;10:1047–56.
- Munoz JL, McCarthy CA, Clark ME, Hall CB. Respiratory syncytial virus infection in C57BL/6 mice: clearance of virus from the lungs with virus-specific cytotoxic T cells. J Virol. 1991;65:4494–97.
- Hall CB, Powell KR, MacDonald NE, Gala CL, Menegus ME, Suffin SC, Cohen HJ. Respiratory syncytial viral infection in children with compromised immune function. N Engl J Med. 1986;315:77–81. doi:10.1056/NEJM198607103150201.
- Harrington RD, Hooton TM, Hackman RC, Storch GA, Osborne B, Gleaves CA, Benson A, Meyers JD. An outbreak of respiratory syncytial virus in a bone marrow transplant center. J Infect Dis. 1992;165:987–93.
- Ridda I, Macintyre CR, Lindley R, Gao Z, Sullivan JS, Yuan FF, McIntyre PB. Immunological responses to pneumococcal vaccine in frail older people. Vaccine. 2009;27:1628–36. doi:10.1016/j.vaccine.2008.11.098.
- Yao X, Hamilton RG, Weng NP, Xue QL, Bream JH, Li H, Tian J, Yeh S-H, Resnick B, Xu X, et al. Frailty is associated with impairment of vaccine-induced antibody response and increase in post-vaccination influenza infection in community-dwelling older adults. Vaccine. 2011;29:5015–21. doi:10.1016/j.vaccine.2011.04.077.
- Levin MJ, Oxman MN, Zhang JH, Johnson GR, Stanley H, Hayward AR, Caulfield MJ, Irwin MR, Smith JG, Clair J, et al. Varicella-zoster virus-specific immune responses in elderly recipients of a herpes zoster vaccine. J Infect Dis. 2008;197:825–35. doi:10.1086/528696.
- Shapiro ED, Berg AT, Austrian R, Schroeder D, Parcells V, Margolis A, Adair RK, Clemens JD. The protective efficacy of polyvalent pneumococcal polysaccharide vaccine. N Engl J Med. 1991;325:1453–60. doi:10.1056/NEJM199111213252101.
- Cherukuri A, Patton K, Gasser RA Jr., Zuo F, Woo J, Esser MT, Tang RS. Adults 65 years old and older have reduced numbers of functional memory T cells to respiratory syncytial virus fusion protein. Clin Vaccine Immunol. 2013;20:239–47. doi:10.1128/CVI.00580-12.
- Lambert SL, Aslam S, Stillman E, MacPhail M, Nelson C, Ro B, Sweetwood R, Lei YM, Woo JC, Tang RS, et al. A novel respiratory syncytial virus (RSV) F subunit vaccine adjuvanted with GLA-SE elicits robust protective TH1-type humoral and cellular immunity in rodent models. PLoS One. 2015;10:e0119509. doi:10.1371/journal.pone.0119509.
- Patton K, Aslam S, Shambaugh C, Lin R, Heeke D, Frantz C, Zuo F, Esser MT, Paliard X, Lambert SL. Enhanced immunogenicity of a respiratory syncytial virus (RSV) F subunit vaccine formulated with the adjuvant GLA-SE in cynomolgus macaques. Vaccine. 2015;33:4472–78. doi:10.1016/j.vaccine.2015.07.025.
- Falloon J, Ji F, Curtis C, Bart S, Sheldon E, Krieger D, Dubovsky F, Lambert S, Takas T, Villafana T, et al. A phase 1a, first-in-human, randomized study of a respiratory syncytial virus F protein vaccine with and without a toll-like receptor-4 agonist and stable emulsion adjuvant. Vaccine. 2016;34:2847–54. doi:10.1016/j.vaccine.2016.04.002.
- Falloon J, Talbot K, Curtis C, Ervin J, Krieger D, Dubovsky F, Takas T, Yu J, Yu L, Lambert SL, et al. Dose selection for an adjuvanted respiratory syncytial virus F protein vaccine for older adults based on humoral and cellular immune responses. Clin Vaccine Immunol. 2017;24. doi:10.1128/CVI.00157-17.
- Falloon J, Yu J, Esser MT, Villafana T, Yu L, Dubovsky F, Takas T, Levin MJ, Falsey AR. An adjuvanted, postfusion F protein-based vaccine did not prevent respiratory syncytial virus illness in older adults. J Infect Dis. 2017;216:1362–70. doi:10.1093/infdis/jix503.
- Arias MA, Van Roey GA, Tregoning JS, Moutaftsi M, Coler RN, Windish HP, Reed SG, Carter D, Shattock RJ, Le Grand R. Glucopyranosyl lipid adjuvant (GLA), a synthetic TLR4 agonist, promotes potent systemic and mucosal responses to intranasal immunization with HIVgp140. PLoS One. 2012;7:e41144. doi:10.1371/journal.pone.0041144.
- Macpherson AJ, McCoy KD, Johansen FE, Brandtzaeg P. The immune geography of IgA induction and function. Mucosal Immunology. 2008;1:11–22. doi:10.1038/mi.2007.6.
- Tangye SG, Tarlinton DM. Memory B cells: effectors of long-lived immune responses. Eur J Immunol. 2009;39:2065–75. doi:10.1002/eji.200939531.
- De Silva NS, Klein U. Dynamics of B cells in germinal centres. Nat Rev Immunol. 2015;15:137–48. doi:10.1038/nri3804.
- Levin MJ, Schmader KE, Pang L, Williams-Diaz A, Zerbe G, Canniff J, Johnson MJ, Caldas Y, Cho A, Lang N, et al. Cellular and humoral responses to a second dose of herpes zoster vaccine administered 10 years after the first dose among older adults. J Infect Dis. 2016;213:14–22. doi:10.1093/infdis/jiv480.
- Oxman MN, Levin MJ, Johnson GR, Schmader KE, Straus SE, Gelb LD, Arbeit RD, Simberkoff MS, Gershon AA, Davis LE, et al. A vaccine to prevent herpes zoster and postherpetic neuralgia in older adults. N Engl J Med. 2005;352:2271–84. doi:10.1056/NEJMoa051016.
- Cunningham AL, Heineman TC, Lal H, Godeaux O, Chlibek R, Hwang SJ, McElhaney JE, Vesikari T, Andrews C, Choi WS, et al. Immune responses to a recombinant glycoprotein E herpes zoster vaccine in adults aged 50 years or older. J Infect Dis. 2018;217:1750–60. doi:10.1093/infdis/jiy095.
- Cunningham AL, Lal H, Kovac M, Chlibek R, Hwang SJ, Diez-Domingo J, Godeaux O, Levin MJ, McElhaney JE, Puig-Barberà J, et al. Efficacy of the herpes zoster subunit vaccine in adults 70 years of age or older. N Engl J Med. 2016;375:1019–32. doi:10.1056/NEJMoa1603800.
- Lal H, Cunningham AL, Godeaux O, Chlibek R, Diez-Domingo J, Hwang SJ, Levin MJ, McElhaney JE, Poder A, Puig-Barberà J, et al. Efficacy of an adjuvanted herpes zoster subunit vaccine in older adults. N Engl J Med. 2015;372:2087–96. doi:10.1056/NEJMoa1501184.
- Furman D, Hejblum BP, Simon N, Jojic V, Dekker CL, Thiebaut R, Tibshirani RJ, Davis MM. Systems analysis of sex differences reveals an immunosuppressive role for testosterone in the response to influenza vaccination. Proc Natl Acad Sci U S A. 2014;111:869–74. doi:10.1073/pnas.1321060111.
- Goldblatt D, Southern J, Andrews N, Ashton L, Burbidge P, Woodgate S, Pebody R, Miller E. The immunogenicity of 7-valent pneumococcal conjugate vaccine versus 23-valent polysaccharide vaccine in adults aged 50–80 years. Clin Infect Dis. 2009;49:1318–25. doi:10.1086/606046.
- Folsgaard NV, Chawes BL, Rasmussen MA, Bischoff AL, Carson CG, Stokholm J, Pedersen L, Hansel TT, Bønnelykke K, Brix S, et al. Neonatal cytokine profile in the airway mucosal lining fluid is skewed by maternal atopy. Am J Respir Crit Care Med. 2012;185:275–80. doi:10.1164/rccm.201108-1471OC.
- Nicholson GC, Kariyawasam HH, Tan AJ, Hohlfeld JM, Quinn D, Walker C, Rodman D, Westwick J, Jurcevic S, Kon OM, et al. The effects of an anti-IL-13 mAb on cytokine levels and nasal symptoms following nasal allergen challenge. J Allergy Clin Immunol. 2011;128:800–7 e9. doi:10.1016/j.jaci.2011.05.013.
- Falloon J, Yu J, Esser M, Villfana T, Dubovsky F, Takas T, Levin MJ, Falsey AR. An adjuvanted, post-fusion F protein-based vaccine did not prevent respiratory syncytial virus illness in older adults. J Infect Dis 2017; 216: 1362–1370. PMID: 29029260.
- Liang B, Surman S, Amaro-Carambot E, Kabatova B, Mackow N, Lingemann M, Yang L, McLellan JS, Graham BS, Kwong PD, et al. Enhanced neutralizing antibody response induced by respiratory syncytial virus prefusion f protein expressed by a vaccine candidate. J Virol. 2015;89:9499–510. doi:10.1128/JVI.01373-15.
- Ngwuta JO, Chen M, Modjarrad K, Joyce MG, Kanekiyo M, Kumar A, Yassine HM, Moin SM, Killikelly AMChuang G-Y, et al., Sci Transl Med. 2015 October 14; 7(309): 309ra162. doi: 10.1126/scitranslmed.aac4241
- Maifeld SV, Ro B, Mok H, Chu M, Yu L, Yamagata R, Leonardson T, Chio V, Parhy B, Park S, et al. Development of electrochemiluminescent serology assays to measure the humoral response to antigens of respiratory syncytial virus. PLoS One. 2016;11:e0153019. doi:10.1371/journal.pone.0153019.