ABSTRACT
Antibodies are effective alternative tools to combat infections caused by Pseudomonas aeruginosa (PA), especially multi-drug-resistant PA. Thus, to solve the urgent need for an anti-PA antibody drug, we hypothesized that anti-PA intravenous immunoglobulins could be a practical attempt. Exotoxin A (ETA) is one of the most important factors for PA infection and is also a critical target for the development of immune interventions. In this study, a total of 320 sera were collected from healthy volunteers. The concentration of ETA-specific antibodies was determined by a Luminex-based assay and then purified by affinity chromatography. The purified IgGs were able to neutralize the cytotoxicity of ETA in vitro. We showed they had a prophylactic and therapeutic protective effect in PA pneumonia and ETA toxemia models. In addition, administration of nonspecific IgGs also provided partial protection. Collectively, our results provide additional evidence for IVIG-based treatment of infections caused by multi-drug-resistant PA and suggest that patients at high risk of PA pneumonia could be prophylactically treated with anti-ETA IgGs or even with nonspecific IgGs.
Introduction
Pseudomonas aeruginosa (PA) is a gram-negative, opportunistic pathogen responsible for infections in debilitated or immunocompromised patients, especially those who suffer from cystic fibrosis, mechanical ventilation, COPD and severe burns.Citation1,Citation2 Conventional treatment of PA infection is of inadequate effectiveness due to the increase of drug-resistance. Especially, the increasing prevalence of multi-drug-resistant PA (MDR-PA) and extensively drug resistance PA (XDR-PA) have urgently caused a need for alternative strategies in addition to novel antibiotics.Citation3,Citation4 Many antibody reagents, which target toxins or pathogenic factors of PA, were discovered to be effective in multiple animal models.Citation5 For example, MEDI3902, a bispecific human monoclonal antibody that binds to both the PcrV protein and the Psl exopolysaccharide of PA, has been tested in phase 1 clinical trial (NCT02255760).Citation6
Exotoxin A (ETA), encoded by the toxA gene and secreted via the type II secretion system, is the most potent toxin of PA. The LD50 of the exotoxin was reported to be 60–80 ng/mouse.Citation7 ETA has a single polypeptide chain consisting of 613 amino acids. Structurally and functionally ETA is composed of three domains, namely, domain I, domain II and domain III (from N to C). Domain I is responsible for binding to the α2-macroglobulin (α2MG) and low-density lipoprotein receptor-related protein 1 (LRP1) receptor on host cells. After translocation into host cells via domain II, the domain III of ETA catalyzes the transfer of the ADP-ribosyl moiety of NAD to elongation factor 2, which leads to the cessation of protein synthesis and then cell death. By this effect, injection of as low as 80 ng of ETA is able to cause necrosis; cellular swelling; and hemorrhaging in livers, lungs and kidneys.Citation7,Citation8
Because of its importance in the pathogenesis of PA infection, ETA has always been a critical target for the development of immune intervention. To date, active vaccination with ETA toxoid has provided as high as 93.8% protective efficacy in a mouse burn model.Citation9 In addition, a recombinant DNA vaccine encoding nontoxic ETA and PcrV also induced effective protection against a challenge of PA in mice airways.Citation10. Meanwhile, anti-ETA antibodies were able to neutralize the lethal effects of ETA and block the spread of PA in a burn wound infection mice model.Citation11
However, no ETA-specific antibody drug is available on the market so far. To address the difficult problem of drug-resistant PA infection, we hypothesized that a polyvalent ETA-specific intravenous immunoglobulin (IVIG) could be a rapidly developed and useful solution for the treatment of PA infections. Thus, we purified the ETA-specific IVIGs from healthy donors in this study. Moreover, their protective effects and mechanism were investigated in mouse pneumonia and toxemia models.
Results
Production and characterization of anti-eta iggs
As no ETA-based vaccine is available, we had to try to produce the ETA-specific IVIG from healthy donors. So far, 320 volunteers were enrolled, and their concentrations of anti-ETA antibodies were determined by a Luminex-based assay. The distribution of the concentrations of the donated sera are shown in , with an average concentration of 98.7 ng/ml. The highest level of the anti-ETA antibodies reached 918.24 ng/ml. These sera were classified into three groups according to the concentration of anti-ETA antibodies, namely, high (H), medium (M) and low (L) (). After affinity chromatography with protein A, the purity of IgGs from all three groups was no less than 95%, as determined by SDS-PAGE and non-reducing SDS-PAGE. Following that, the neutralizing activities of the purified anti-ETA IgGs were evaluated in vitro. As shown in , in the presence of ETA there was no significant difference in death between H IgG-treated cells and that of cells untreated with ETA. The neutralizing activities were reduced with a decrease in the level of anti-ETA IgGs, as observed in M- and L- IgGs treated cells. These data indicate that anti-ETA IgGs were able to block ETA cytotoxicity.
Figure 1. Preparation of anti-ETA IgGs
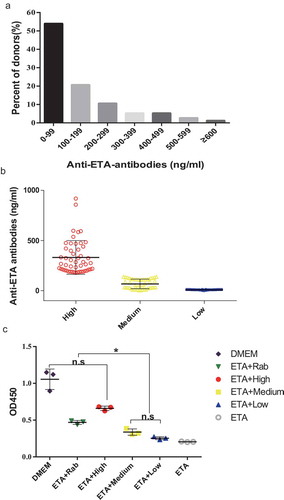
Prophylactic protection against PA infection of anti-eta iggs
First, an acute PA causing pneumonia was used to evaluate the prophylactic effects of ETA specific IgGs. shows the survival of mice given IgGs before being challenged with a lethal dose of PA (9.0 × 10Citation5/mouse). Clearly, the survival rates of mice in the PBS, Non-rab (non-specific IgGs), L (low) and Rab (rabbit anti-ETA IgGs) groups were significantly lower than that from M (medium) and H (high) groups. In particular, all mice in the PBS and Non-rab (non-specific IgGs) group died within as early as 36 and 60 hours after infection with PA, respectively (). In contrast, mice given the same amount of IgG containing high and medium levels of anti-ETA antibodies showed dramatic increases in survival compared to the other groups. However, no significant difference in survival was observed between these two groups (P > .05). In addition, mice from Rab group showed significantly better survival than that in PBS and Non-rab group, indicating a restricted specific protection of rabbit anti-ETA antibodies alone.
Figure 2. Prophylactic protection against PA infection of anti-ETA IgGs
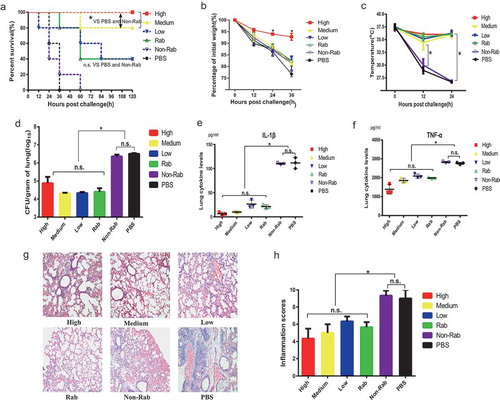
Next, mice injected with IgGs were challenged with a sublethal dose of PA to investigate the mechanism for ETA-specific IgG-mediated protection. Their body weight and body temperature were monitored for 36 and 24 hours. As expected, mice in the H group suffered less weight loss than mice in the other groups during the observation period of 36 hours (). Additionally, mice in the PBS and Non-Rab group and became hypothermic 12 hours after the challenge, and there were significant differences of body temperature between the two control group and the other groups ().
Furthermore, the number of bacteria in the lungs was determined, and the results showed that there was no significant difference among the H, M L and Rab groups (P > .05) and all of them were significantly lower than that in the PBS and Non-Rab group (). A similar trend was also observed when measuring the levels of inflammatory cytokines in the BALF. As shown in and , the levels of IL-1β and TNF-α in BALF of mice in the PBS and Non-Rab group were higher than those in the H, M, L and Rab groups; however, no significant difference was observed among the four groups.
To this end, histologic changes in the lung were also observed in the mice challenged with a sublethal dose of bacteria. Clearly, interstitial congestion and edematous morphological changes with inflammatory cell recruitment were observed in the lungs of mice treated with PBS, which indicates an acute severe inflammatory response. However modest inflammatory responses, which were characterized by reduced neutrophil infiltration and interstitial edema, were observed in mice given high, medium and even low levels of human IgGs and rabbit IgGs. Consistent with the microscopy observations, the inflammation scores of sections from the PBS and Non-Rab group were significantly higher than those of the other groups (), whereas no significant difference was observed among the groups that received anti-ETA IgGs purified from human and rabbits. Collectively, these data suggested that anti-ETA IgGs were protective against PA caused pneumonia in a concentration dependent manner.
Prophylactic protection against ETA toxemia of anti-ETA iggs
These findings led us to evaluate whether the anti-ETA IgGs were still protective in an ETA toxemia model. After challenge with 0.4 μg of ETA, all mice in the PBS and Non-Rab group died within 48 and 60 hours, respectively; however, the survival rate of the Rab, H, M and L groups were 100%, 100%, 80% and 20%, which indicates a protection against ETA (). These results were correlated with the changes in body weight and temperature when challenged with a half LD50 of ETA ( and ). Mice in the PBS group and Non-rab had a significant reduction of body weight loss and temperature. However, in the presence of high levels of anti-ETA antibodies, only small changes in body weight and temperature were observed compared with the mice given rabbit anti-ETA polyclonal antibodies.
Figure 3. Prophylactic protection against ETA toxemia of anti-ETA IgGs
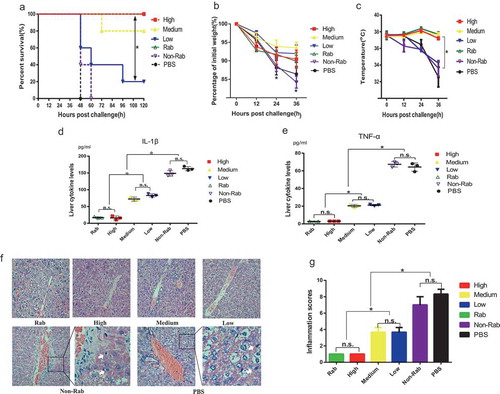
Next, the levels of IL-1β and TNF-α in the livers of mice were determined 24 hours after the challenge. As expected, the level of IL-1β in the H group was similar to that in the positive control group, indicating full protection by the high-level anti-ETA antibodies. However, the concentration of IL-1β in the liver increased with a reduction in the concentration of anti-ETA IgGs (). A similar trend of the change in TNF-α was observed, which also showed a pattern (). Additionally, HE staining of the liver from the PBS group showed hemorrhagic panlobular necrosis with infiltration of polymorphonuclear leukocytes and acidophilic (Councilman) bodies These pathologic changes were alleviated by an increase in anti-ETA IgGs (). Consistently, the results from semiquantitative analysis of these sections showed a similar trend, as observed by microscopy. The inflammation score of mice in the H group was as low as that in the positive control group. However, higher scores were observed in both the M and L groups, although both of them were lower than that of the PBS and Non-Rab group ().
Therapeutic protection against PA infection of anti-eta iggs
For the practical application of anti-ETA IgGs, we determined the therapeutic effects in an acute PA pneumonia model. In this setting, mice were intratracheally injected with a lethal dose of PA103. Three hours later, they were administered high-, medium- or low levels of anti-ETA IgGs. Ceftazidime and PBS were used as positive and negative controls, respectively. shows the survival of the mice in each group. Clearly, all mice administered PBS and non-specific rabbit IgGs died within 36 hours. However, 100% of the mice that received a high level of anti-ETA IgGs or caftazidime survived throughout the whole observation period. In addition, 20% of mice received low levels of anti-ETA IgGs and 40% of mice received rabbit anti-ETA IgGs survived for 120 hours ().
Figure 4. Therapeutic protection against PA infection of anti-ETA IgGs
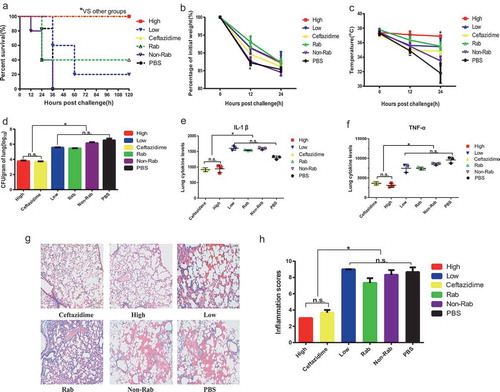
To further understand the mechanism of IgG-induced protection, mice were challenged with a sublethal dose of PA103 and treated with ETA-specific IgGs. The results from the observation of body weights and body temperatures showed that protection induced by high-level anti-ETA IgGs was as efficient as that induced by ceftazidime (), and partial protection was observed in mice treated with low level of anti-ETA IgGs or rabbit anti-ETA IgGs. Similarly, quantification of the bacteria load in the lung showed protective effects of high-level anti-ETA IgGs, as no significant difference in bacteria loads was observed between the high-level IgGs and ceftazidime. In addition, a similar pattern of inflammatory response was observed in the lungs (). Additionally, the levels of IL-1β and TNF-α from mice treated with high-ETA IgGs were as low as those treated with ceftazidime, which suggests an effective protection in the lung (). However, the concentrations of the two cytokines increased dramatically in PBS-treated mice (). The results from HE staining of lung sections showed that a restricted inflammatory response occurred in the lungs of high-ETA-treated mice, as was also observed in that from ceftazidime-treated mice (). Nevertheless, little changes in inflammatory response in the lungs of mice treated with low-ETA IgGs, rabbit anti-ETA IgGS and non-specific rabbit IgGs, were observed compared to that in mice treated with PBS. Taken together, these data indicate that high levels of anti-ETA IgGs were able to treat PA-caused pneumonia.
Anti-ETA iggs mediated protections depends on ETA specific antibodies
To further confirm the specific protection of anti-ETA antibodies, the level of anti-PcrV antibodies in donated sera were determined simultaneously. However, no significant difference was observed among the H, M, and L groups, which was classified by the titer of anti-ETA antibodies (Figure S1). Then IgGs containing high level of anti-ETA were firstly incubated ETA coated beads to remove these antibodies. The protection of the IgGs after the removal of anti-ETA antibodies were evaluated. In the ETA toxemia model, the absorption of anti-ETA antibodies lead to complete loss of protection, as no significant difference of survival was observed between GST-ETA absorbed group (H-Abs group) and PBS group (). Whereas a mixture of the two kind of IgGs showed partial protection. Similar trends were also observed in the changes of bodyweights and body temperatures (&). Subsequently, the prophylactic and therapeutic protection of GST-ETA absorbed IgGs were evaluated in acute pneumonia models. Clearly, mice treated with GST-ETA absorbed IgGs showed significantly deceased survival, when compared to that with the unabsorbed IgGs (). However significant difference of survival was observed between mice treated with GST-ETA absorbed IgGs and PBS, which indicates a partial protection mediated by non-specific or other IgGs. Meanwhile, results from changes of bodyweights and body temperatures showed a similar trend (). To this end, the therapeutic effects of high-level anti-ETA IgGs was also significantly impaired after the removal of ETA specific antibodies (). In addition, a restricted therapeutic protection of GST-ETA absorbed IgGs could still be observed. Consistent with the survival observations, result from the bodyweights and body temperatures showed a similar trend of change of therapeutic protection (&).
Figure 5. Anti-ETA IgGs mediated protections depends on ETA specific antibodies
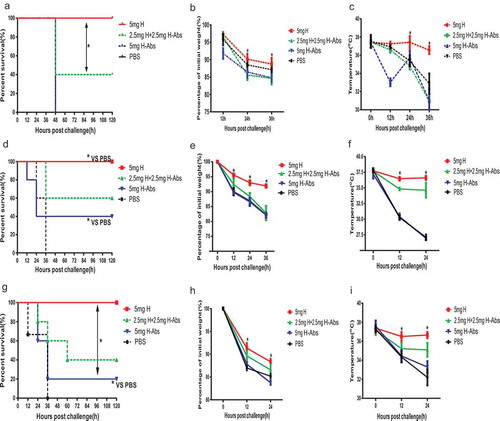
Discussion
Recently, the prevalence of multi-drug-resistant bacteria has promoted the urgent need for alternative treatment strategies. Antibody-based therapies have made remarkable progress. To date, obiltoxaximab for Bacillus anthracis has proceeded to clinical applications,Citation12 and bezlotoxumab is the first antibody available for secondary prevention of recurrence of Clostridioides difficile infection that has been approved by the regulatory agencies in US and Europe.Citation13 In cases of PA infection control, Teiji Sawa et al reported that a molecularly engineered humanized anti-PcrV IgG, KB001.Citation14 It had demonstrated its safety, a favorable pharmacokinetic profile, and promising potential as a nonantibiotic strategy to reduce airway inflammation and damage in PA pneumonia.Citation15 In addition, the phase 1 clinical trial of MEDI3902 is ongoing, which is a bispecific human monoclonal antibody (NCT02255760).Citation6
A sufficient amount of passively transferred antibodies could provide rapid protection against PA infections, especially combined with antibiotics. In fact, a monoclonal full human antibody is an ideal form for antibody drugs, but they require tremendous investments and years to develop. To fulfill the urgent need for antibody drugs against MDR-PA and other multi-drug-resistant pathogens, IVIG containing pathogen-specific antibodies could be an optimal choice. An IVIG containing a high concentration of anti-PcrV antibodies has been proven to be protective in mice.Citation16,Citation17 Here, we found that injection with ETA-specific IVIG was also able to confer protection, which provides additional evidence for IVIG-based treatment of infections caused by multi-drug-resistant bacteria.
One interesting finding is that the low levels anti-ETA IgGs showed limited prophylactic and even therapeutic protection, although no neutralization activity was observed. We deduced that there were two possible explanations. One is that these IgGs could contain other PA-specific antibodies, such as anti-PcrV antibodies, which have previously been proven to be protective.Citation16,Citation17 In addition, several other studies showed that a lower incidence of infectious episodes was observed after a prophylactic treatment of nonspecific intravenous IgG.Citation18 Thus, we deduced that the other explanation could be that immunoglobulin provides nonspecific protection against PA infection. Previous studies have shown that immunoglobulin is able to act as an immune activator by promoting lymphocyte development and improving lymphocyte function simultaneously.Citation19,Citation20
Previously studies have showed that the major virulence of cytotoxic PA103 in rat was quite dependent on ExoU toxin, but not ETA.Citation21 Despite its disability to cause lung epithelial injury, ETA was found to contribute to lung infection by reducing the constitutive IL-18 levels, and by weak induction of IL-1β, IL-6 and other proinflammatory cytokines.Citation22 In addition, a PA103 mutant, which did not produce ETA, showed reduced pulmonary colonization when compared with the wild-type PA103 strain.Citation23 Moreover, vaccination of a DNA vaccine encoding ETA alone showed reduced bacteria loads and lung injuries in an acute PA pneumonia model.Citation10 Thus, it is not surprising to note that anti-ETA antibodies conferred prophylactic and therapeutic protections against acute PA pneumonia in our study.
As an ETA-based vaccine was not available on the market, we had to prepare the ETA-specific IgGs from healthy volunteers who have just recovered from a natural infection of PA. As a result, the purified anti-ETA antibodies showed limited titers and subsequently restricted protection, as we observed in this study. Thus, to improve the protective effect, we suggest purifying high-titer ETA specific IgGs from ETA-vaccinated volunteers. Another reason for the limited protection could be the complicated pathogenesis of PA. To our knowledge, by its type I, II, III and VI secretion system, PA is able to deliver plenty of toxic effectors to cause infectious diseases.Citation24,Citation25 These pathogenic effects may not be affected by the anti-ETA IgGs alone, which resulted in the limited protection. Therefore, a combination of IgGs that neutralized other pathogenic factors could provide better protection.
There were two major limitations in our study. Firstly, the protection of anti-ETA IgGs was tested only in acute pneumonia and toxemia model by PA strain PA103. Thus, additional clinical PA isolates and other animal models were suggested to applied to confirm the anti-ETA IgGs mediated protection. Secondly, the mechanisms for IgGs with low anti-ETA titer were not investigated. To find the protective agents in this IgGs would benefit the discovery of novel drugs for the control of PA infection.
In conclusion, in this study we proved that anti-ETA IgGs could be purified from healthy donors and that these IgGs showed a prophylactic and therapeutic protection in PA pneumonia and ETA toxemia models. In addition, administration of nonspecific IgGs also provided partial protection. Collectively, our data suggest that patients at a high-risk of PA pneumonia could be prophylactically treated with anti-ETA IgGs or even nonspecific IgGs.
Materials and methods
Mice and strains
Six- to eight-week-old specific pathogen-free female BALB/c mice were purchased from Beijing HFK Bioscience Limited Company (Beijing, People’s Republic of China). Mice were maintained under barrier conditions in a biohazard animal room. PA strain PA103 was obtained from the ATCC (No. 29260). All experiments complied with ethical regulations and were approved by the Animal Ethical and Experimental Committee of the Third Military Medical University (N0. TMMU0157).
Purification of eta-specific immunoglobulin
From December 2013 to March 2016, a total of 320 sera were collected from healthy volunteers from Xinqiao Hospital in Chongqing, China. Written informed consent was obtained from all enrolled volunteers. The concentration of ETA-specific antibodies was determined by a Luminex-based assay. In brief, beads were coated with nontoxic purified recombinant ETA (ETA) at a concentration of 1.4 μg/million beads. After gradient dilutions, sera were incubated with the ETA-coated beads at 37°C for 45 minutes. These beads were then incubated with PE-labeled goat anti-human Fc antibodies at 37°C for 30 minutes after washing with PBST three times. Finally, these beads were loaded on the Luminex 200 platform to determine the intensity of fluorescence of each reaction. A human anti-ETA monoclonal antibody (2H10) was used as a control. Meanwhile, the concentrations of anti-PcrV antibodies of these sera were determined in the same way. The concentration and intensity of fluorescence of the mAb was used to generate a standard curve, and the concentration of anti-ETA antibodies in each serum sample was calculated.
According to the concentration of anti-ETA antibodies, the 320 sera classified into three groups, high, medium and low. And fifty sera were randomly selected from each group and mixed together, which were then loaded on a protein A Sepharose column in PBS buffer. The IgGs were eluted with citrate buffer solution (pH = 4.0) and then equilibrated with PBS. The concentration of antibodies was determined using the New Flash TM Protein any KD (Dakewe, Beijing). The anti-ETA IgGs were stored at −80°C until use.
Meanwhile, rabbit anti-ETA polyclonal antibodies were produced. Briefly, rabbits were immunized with 500 μg of denatured ETA-formulated Al(OH)3 adjuvant three times at 2-week intervals. One week after the last immunization the sera were collected, and the titers were tested by ELISA. The sera of unimmunized rabbit (non-specific IgGs) were harvested in the same way. Finally, the IgG in the two sera was purified by Protein A affinity chromatography as described for the human sera.
In vitro analysis of the neutralizing activities of anti-eta iggs
The neutralizing activities of the anti-ETA IgGs were determined by an L929 cell model.Citation11 In brief, 100 μl of cell suspension was dispensed in 96-well plates (5000 cells per well). Then, the plates were incubated at 37°C for 24 hours. Next, 0.66 μg of wild-type ETA mixed with or without 1.5 μg of anti-ETA IgG was added and incubated for an additional 60 hours. Cells treated with 10 μl of DMSO were used as a positive control. Finally, the death of the cells was monitored by a CCK-8 kit according to the instructions of the manufacturer (BBI Life Science). In short, 10 μl of CCK-8-solution was added to each well and incubated for 1 hour. The absorbance was measured at 450 nm. Each experimental condition was performed in triplicate.
Generation of recombinant ETA
The gene encoding ETA was synthesized by the Shenggong (Shanghai, China) company and cloned into the pGEX6p-1 vector to generate the plasmid pGEX6p-1-ETA. Escherichia coli harboring the pGEX6p-1-ETA vector were inoculated into LB broth and incubated at 37°C for 5.5 hours. The cells were then treated with IPTG (0.2 M) and incubated at 16°C for an additional 12 hours. Cells were collected by centrifugation at 5000 rpm for 20 min and resuspended in lysis buffer (20 mM Tris, 150 mM NaCl, pH = 8.0). After sonication, the supernatant was collected by centrifugation and incubated with GST resins (Best Chrom). The GST resins were then washed with PBST buffer, and PreScission proteinase was added to remove the GST tag. The eluted protein was then dialyzed against PBS and stored at −80°C before use. The purity of the protein was assessed by sodium dodecyl sulfate polyacrylamide gel electrophoresis (SDS-PAGE).
Evaluation of anti-eta antibodies in pneumonia mode
To test the prophylactic effects of anti-ETA IgGs, each mouse was intraperitoneally injected with 5 mg of antibodies. One hour later, the mice were intratracheally challenged with a lethal dose of PA103 (9.0 × 10Citation5 CFU/mouse). To further understand the mechanism for anti-ETA antibody-mediated protection, mice were also challenged with a sublethal dose of PA103 (4.5 × 10Citation5 CFU/mouse). The changes in body temperature and loss of weight after the challenges were recorded. In addition, the bacterial load in the lung was determined. Finally, the lungs were collected and homogenized in 1 ml of sterilized PBS buffer 24 hours postchallenge. The homogenates were than plated on LB plates at a 10-fold serial dilution and cultured at 37°C for 20 hours. The number of colonies on the plates was counted and used to calculate the number of bacteria in the lung.
To test the therapeutic effects of anti-ETA IgGs, each mouse was intraperitoneally injected with 5 mg of antibodies three hours after intratracheally challenged with a lethal dose of PA103 (9.0 × 10Citation5 CFU/mouse). To better understand the mechanism for anti-ETA antibody-mediated protection, mice were also challenged with a sublethal dose of PA103 (4.5 × 10Citation5 CFU/mouse). The changes in body temperature and loss of weight after the challenges were recorded. In addition, the bacterial load in the lung was determined as previously.
In addition, the inflammatory response in the lung was measured in two ways as described previously.Citation7 First, the pathologic changes in the lungs were observed. Lungs were collected and fixed in formaldehyde (10%) 24 hours after the mice were challenged with PA103. Then, the tissues were embedded in paraffin, sectioned, and stained with hematoxylin and eosin (HE). Hyperemia, edema, hemorrhage, and neutrophil infiltration were graded 0 (normal) to 3 (severe) by a single pathologist. The scores of each parameter were added together to obtain the inflammation score of each section. Four sections from each mouse were evaluated, and the inflammation scores were calculated to semiquantify the pathological changes. Second, the levels of inflammatory cytokines in bronchoalveolar lavage fluid (BALF) were determined. Briefly, BALF was collected four hours after the challenge. The concentrations of TNF-α and IL-1β were determined by ELISA kits (DAKEWE) following the manufacturer’s instructions.
Evaluation of anti-eta antibodies in a toxemia model
To test the protective effects of anti-ETA IgGs, 5 mg of antibodies was incubated with 0.4 μg of purified ETA at 37°C for 1 hour. Then, the mixture was intraperitoneally administered to each mouse, and the number of deaths were recorded every 12 hours. In addition, mice were challenged with a mixture containing 0.2 μg of ETA to investigate the mechanism for antibodies-mediated protection. The body temperature and weight were recorded every 12 hours for 36 hours. In addition, livers from challenged mice were collected and fixed in neutral 10% formalin and embedded in paraffin. Following sectioning, tissues were stained with HE and viewed at 400× by microscopy. The histological changes were scored according to the observation of steatosis, inflammation and liver injury as described before.Citation26 Moreover, the level of TNF-α in the liver was determined as described previously.
Confirmation of the specificity of anti-eta antibodies in a different models
E.coli harboring the pGEX6p-1-ETA vector was grown as described before. Cells were collected by centrifugation and resuspended in lysis buffer (20 mM Tris, 150 mM NaCl, pH = 8.0). After sonication, the supernatant was collected and incubated with GST resins (Best Chrom). After washing, The GST-ETA on resins were co-incubated with purified anti-hu ETA-IgGs for 24 hours. Then the IgGs were collected and stored at −80°C before use. The specificity of anti-ETA IgGs was tested in three experimental settings: 5 mg of high-level anti-ETA IgG(H), 2.5 mg of anti-hu ETA-IgG mixed with 2.5 mg of absorbed IgGs and 5 mg of absorbed IgGs (H-Abs). Their protective effects were evaluated in toxemia model and PA pneumonia model as described previously.
Statistics analysis
Data are presented as the mean ±SE or median. The significance of the differences was determined by unpaired parametric tests (one-way ANOVA), which was followed by the Tukey’s HSD test for multiple comparisons. Graphs were plotted and analyzed using Graph Prism v. 6.01. Bacterial burden was analyzed by the nonparametric Mann-Whitney test. The Kaplan-Meier test was used for analysis of the survival rate. Differences were considered as significant when the P value was lower than 0.05. All experiments except the animal challenge assays were conducted a minimum of three times.
Author contributions
QZ, XW and JG designed, coordinated and supervised the research. JZ and WC performed the major experiments. BY provided the serum samples. FY developed the pneumonia model. CG and XC collected clinical samples. LZ and HG assisted the Luminex measurement. JZ and JG wrote the manuscript. All authors revised the manuscript and contributed to improving the paper; all authors read and approved the final manuscript.
Disclosure of potential conflicts of interest
No conflict of interest exits in the submission of this manuscript.
Supplemental Material
Download PDF (58.9 KB)Supplemental data
Supplemental data for this paper can be accessed on the publisher’s website.
Additional information
Funding
References
- Nguyen L, Garcia J, Gruenberg K, MacDougall C. Multidrug-resistant pseudomonas infections: hard to treat, but hope on the horizon? Curr Infect Dis Rep. 2018;20:23. doi:10.1007/s11908-018-0629-6.
- Wieland K, Chhatwal P, Vonberg RP. Nosocomial outbreaks caused by Acinetobacter baumannii and Pseudomonas aeruginosa: results of a systematic review. Am J Infect Control. 2018;46:643–48. doi:10.1016/j.ajic.2017.12.014.
- Zhang J, Zhao C, Chen H, Li H, Wang Q, Wang Z, Zhang F, Wang H. A multicenter epidemiology study on the risk factors and clinical outcomes of nosocomial intra-abdominal infections in China: results from the Chinese Antimicrobial Resistance Surveillance of Nosocomial Infections (CARES) 2007-2016. Infect Drug Resist. 2018;11:2311–19. doi:10.2147/IDR.S182180.
- Bassetti M, Righi E, Vena A, Graziano E, Russo A, Peghin M. Risk stratification and treatment of ICU-acquired pneumonia caused by multidrug- resistant/extensively drug-resistant/pandrug-resistant bacteria. Curr Opin Crit Care. 2018;24:385–93. doi:10.1097/MCC.0000000000000534.
- Francois B, Luyt CE, Stover CK, Brubaker JO, Chastre J, Jafri HS. New strategies targeting virulence factors of staphylococcus aureus and Pseudomonas aeruginosa. Semin Respir Crit Care Med. 2017;38:346–58. doi:10.1055/s-0037-1602715.
- Ali SO, Yu XQ, Robbie GJ, Wu Y, Shoemaker K, Yu L, DiGiandomenico A, Keller AE, Anude C, Hernandez-Illas M, et al. Phase 1 study of MEDI3902, an investigational anti-Pseudomonas aeruginosa PcrV and Psl bispecific human monoclonal antibody, in healthy adults. Clin Microbiol Infect. 2019;25:629.e621-629.e626. doi:10.1016/j.cmi.2018.08.004.
- Armstrong S, Yates SP, Merrill AR. Insight into the catalytic mechanism of Pseudomonas aeruginosa exotoxin A. Studies of toxin interaction with eukaryotic elongation factor-2. J Biol Chem. 2002;277:46669–75. doi:10.1074/jbc.M206916200.
- Wretlind B, Pavlovskis OR. The role of proteases and exotoxin A in the pathogenicity of Pseudomonas aeruginosa infections. Scand J Infect Dis Suppl. 1981;29:13–19.
- Manafi A, Kohanteb J, Mehrabani D, Japoni A, Amini M, Naghmachi M, Zaghi AH, Khalili N. Active immunization using exotoxin A confers protection against Pseudomonas aeruginosa infection in a mouse burn model. BMC Microbiol. 2009;9:23. doi:10.1186/1471-2180-9-23.
- Jiang M, Yao J, Feng G. Protective effect of DNA vaccine encoding pseudomonas exotoxin A and PcrV against acute pulmonary P. aeruginosa Infection. PLoS One. 2014;9:e96609. doi:10.1371/journal.pone.0096609.
- Fogle MR, Griswold JA, Oliver JW, Hamood AN. Anti-ETA IgG neutralizes the effects of Pseudomonas aeruginosa exotoxin A. J Surg Res. 2002;106:86–98.
- Head BM, Rubinstein E, Meyers AF. Alternative pre-approved and novel therapies for the treatment of anthrax. BMC Infect Dis. 2016;16:621. doi:10.1186/s12879-016-1987-z.
- Navalkele BD, Chopra T. Bezlotoxumab: an emerging monoclonal antibody therapy for prevention of recurrent Clostridium difficile infection. Biologics. 2018;12:11–21. doi:10.2147/BTT.S127099.
- Sawa T, Ito E, Nguyen VH, Haight M. Anti-PcrV antibody strategies against virulent Pseudomonas aeruginosa. Hum Vaccin Immunother. 2014;10:2843–52. doi:10.4161/21645515.2014.971641.
- Milla CE, Chmiel JF, Accurso FJ, VanDevanter DR, Konstan MW, Yarranton G, Geller DE. Anti-PcrV antibody in cystic fibrosis: a novel approach targeting Pseudomonas aeruginosa airway infection. Pediatr Pulmonol. 2014;49:650–58. doi:10.1002/ppul.22890.
- Katoh H, Yasumoto H, Shimizu M, Hamaoka S, Kinoshita M, Akiyama K, Sawa T. IV immunoglobulin for acute lung injury and Bacteremia in Pseudomonas aeruginosa Pneumonia. Crit Care Med. 2016;44:e12–24. doi:10.1097/CCM.0000000000001271.
- Kinoshita M, Kato H, Yasumoto H, Shimizu M, Hamaoka S, Naito Y, Akiyama K, Moriyama K, Sawa T. The prophylactic effects of human IgG derived from sera containing high anti-PcrV titers against pneumonia-causing Pseudomonas aeruginosa. Hum Vaccin Immunother. 2016;12:2833–46. doi:10.1080/21645515.2016.1209280.
- Molica S, Musto P, Chiurazzi F, Specchia G, Brugiatelli M, Cicoira L, Levato D, Nobile F, Carotenuto M, Liso V, et al. Prophylaxis against infections with low-dose intravenous immunoglobulins (IVIG) in chronic lymphocytic leukemia. Results of a crossover study. Haematologica. 1996;81:121–26.
- Joao C, Ogle BM, Geyer S. Immunoglobulin promotes the diversity and the function of T cells. Eur J Immunol. 2006;36:1718–28. doi:10.1002/eji.200635908.
- Pires AE, Afonso AF, Queiros A, Cabral MS, Porrata L, Markovic SN, Kaveri SV, Da Silva MG, João C. Treatment with polyclonal immunoglobulin during T-cell reconstitution promotes naive T-cell proliferation. J Immunother. 2010;33:618–25. doi:10.1097/CJI.0b013e3181d3cb19.
- Finck-Barbancon V, Goranson J, Zhu L, Sawa T, Wiener-Kronish JP, Fleiszig SM, Wu C, Mende-Mueller L, Frank DW. ExoU expression by Pseudomonas aeruginosa correlates with acute cytotoxicity and epithelial injury. Mol Microbiol. 1997;25:547–57.
- Wieland CW, Siegmund B, Senaldi G, Vasil ML, Dinarello CA, Fantuzzi G. Pulmonary inflammation induced by Pseudomonas aeruginosa lipopolysaccharide, phospholipase C, and exotoxin A: role of interferon regulatory factor 1. Infection and Immunity. 2002;70:1352–58. doi:10.1128/iai.70.3.1352-1358.2002.
- Schultz MJ, Rijneveld AW, Florquin S, Speelman P, Van Deventer SJ, van der Poll T. Impairment of host defence by exotoxin A in Pseudomonas aeruginosa pneumonia in mice. J Med Microbiol. 2001;50:822–27. doi:10.1099/0022-1317-50-9-822.
- Hauser AR. The type III secretion system of Pseudomonas aeruginosa: infection by injection. Nat Rev Microbiol. 2009;7:654–65. doi:10.1038/nrmicro2199.
- Hood RD, Singh P, Hsu F, Guvener T, Carl MA, Trinidad RR, Silverman JM, Ohlson BB, Hicks KG, Plemel RL, et al. A type VI secretion system of Pseudomonas aeruginosa targets a toxin to bacteria. Cell Host Microbe. 2010;7:25–37. doi:10.1016/j.chom.2009.12.007.
- Kleiner DE, Brunt EM, Van Natta M, Behling C, Contos MJ, Cummings OW, Ferrell LD, Liu Y-C, Torbenson MS, Unalp-Arida A, et al. Design and validation of a histological scoring system for nonalcoholic fatty liver disease. Hepatology. 2005;41:1313–21. doi:10.1002/hep.20701.