ABSTRACT
TNF Receptor Superfamily (TNF-R-SF) signaling is a structurally well-defined event that requires proper receptor clustering and trimerization. While the TNF-SF ligands naturally exist as trivalent functional units, the receptors are usually separated on the cell surface. Critically, receptor assembly into functional trimeric signaling complexes occurs through binding of the natural ligand unit. TNF-R-SF members, including CD40, have been key immunotherapeutic targets for over 20 years. CD40, expressed by antigen-presenting cells, endothelial cells, and many tumors, plays a fundamental role in connecting innate and adaptive immunity. The multiple investigated strategies to induce CD40 signaling can be broadly grouped into antibody-based or CD40L-based approaches. Currently, seven different antibodies and one CD40L-based hexavalent fusion protein are in active clinical trials. In this review, we describe the biology and structural properties of CD40, requirements for agonistic signal transduction through CD40 and summarize current attempts to exploit the CD40 signaling pathway for the treatment of cancer.
Introduction
Cancer immunotherapy has become the most active field of research in third-generation cancer treatment. Most of the past therapeutic approaches, such as chemotherapy or radiotherapy, aimed at direct killing of tumor cells. Due to a lack of specificity, these therapies had frequent side effects on immune cells, often leading to a weakened immune status of the patient. The development of tumor-targeted therapeutics was a step into the direction of personalized medicine, led to an improvement in tumor specificity and restricted some side-effects, albeit at the frequent cost of overall response rates. In contrast, many clinical studies over the last few decades showed that targeting the tumor microenvironment (TME) and stimulating anti-tumor immunity results in robust and long-lasting anti-tumor responses. This high extent of specificity and adaptability is the foundation for immunotherapeutic approaches like vaccination against pathogens and cancer. Consequently, this led to the regulatory approval of immune-checkpoint inhibition (ICI) therapy targeting PD-1, CTLA-4 and PD-L1, for example, and this list may continue to expand with new immune checkpoints, such as TIM-3, LAG-3, and TIGIT, that are currently being investigated. The main goal of ICI therapy is to maintain previously established anti-tumor activity. In contrast, stimulatory immunotherapy targets, such as CD40, ICOS, CD27, GITR, OX40, and 4-1BB, are addressed with agonistic compounds and primarily focus on earlier phases of the immune response. The earliest acting of these molecules is most likely CD40, since it plays a critical role in antigen presentation and therefore, indirectly, T cell activation. An essential assignment of the immune system is to detect foreign antigens from viral, bacterial or parasitic infections, and discriminate those from self-antigens. Similarly, malignant cell transformation frequently generates neo-antigens, or “mutated-self” antigens, which are recognized by cytotoxic immune cells.Citation1,Citation2 In this review, we briefly describe the biological relevance of CD40, the specific requirements for agonistic signal transduction through CD40 and summarize current approaches to stimulate CD40 for the treatment of cancer.
Expression of CD40/CD40L
In order to achieve a strong and specific immune response, innate and adaptive branches need to be orchestrated across multiple interfaces. Many critically important phases of the immune response are mediated by the tumor necrosis factor (TNF) superfamily (SF) of ligands and their receptors, including CD40/CD40L.Citation3 Efficient antigen recognition by antigen-specific T cells critically depends on the presence and functionality of specialized antigen-presenting cells (APC), such as B cells and dendritic cells (DC).Citation2 These APC usually express the costimulatory surface receptor CD40 (TNFRSF5) on the cell surface. CD40 is a constitutively expressed 48-kDa type I transmembrane protein and a critical mediator of immune cell communication in bridging innate and adaptive immunity. CD40 is found on platelets, B cells, and myeloid cells, but also on non-hematopoietic cells like endothelial cells, fibroblasts, smooth muscle cells and even certain types of tumor cells. The cognate ligand for CD40 is CD154 (TNFSF5/CD40L), a 39-kDa type II transmembrane protein. Expression of CD40L is usually inducible and restricted to cells of the hematopoietic system, such as platelets, granulocytes, activated T cells, activated B cells and activated natural killer (NK) cells, but is also weakly expressed on endothelial and smooth muscle cells.
Biological relevance of CD40/CD40L
The pleiotropic functions of CD40 signaling in vivo have been previously reviewed.Citation3,Citation4 Briefly, CD40 expression on monocytes, and their progeny macrophages and DC, and B cells plays an important role in immune cell function. Monocytes are innate immune precursor cells with very high plasticity. They have the ability to differentiate into several cell types, such as macrophages, myeloid-derived suppressor cells (MDSC) and DC.Citation5,Citation6 CD40 signaling is an important trigger of the monocyte maturation process and mainly drives differentiation into macrophages of the M1 spectrum and DC. CD40 engagement on the surface of DC promotes cytokine and chemokine production, induces expression of costimulatory molecules, and facilitates the cross-presentation of antigens.Citation3 One of the main functions of CD40L is to enhance antigen-presentation to T cells by activating DC.Citation3 This step, called “licensing”, increases the interaction of DC with T cells by upregulation of surface proteins such as CD54 and CD86, and thus activates the latter.
B cells are likewise targets of CD40L activity. In the thymus, extensive crosstalk between T cells and B cells is required for maintenance of CD40-expressing B cells and enables negative selection of autoreactive T cells.Citation7 The direct interaction of B cells with CD4 + T cells induces expression of the CD40L by the T cells, which in turn protects B cells from undergoing apoptosis. In this function, CD40L provides a survival signal through activation of PI3K/Akt, which contributes to the observed longevity of activated B cells and their differentiation to plasma cells. Homeostatic proliferation and survival of antigen-specific B cells through CD40 are maintained by upregulation of anti-apoptotic members of the Bcl-2 family.Citation8 In fact, defects of the CD40/CD40L system are associated with deficiency in antibody class switching and somatic hyper-mutation in B cells (hyper-IgM syndrome).Citation9
Their interaction with activated CD40L-expressing T cells also increases the expression of MHC-II and costimulatory molecules like CD80 or CD86 and induces Ig-class switching in B cells.Citation3 Activated B cells migrate to lymphoid organs where they present antigen to T cells, and CD40-activated DC and B cells support the immune response by releasing immunostimulatory cytokines and chemokines like IL-6, IL-12p70, IFNγ, CXCL10, and TNFα. In addition, CD40-activated B cells are able to prime antigen-specific CD8 + T cells with enhanced secretion of cytokines including TNFα and IFNγ.Citation10,Citation11 Several studies provided evidence that ex vivo-activated CD40-expressing B cells are fully functional antigen-presenting B cells and subsequent adoptive cell transfer (ACT) therapy with these cells improved anti-tumor efficacy.Citation12-Citation14
CD40/CD40L relevance for cancer immunotherapy
The extraordinary ability of CD40/CD40L signaling to synchronize the innate, cellular and humoral branches of the immune response has inspired basic and clinical research for decades. Given the general expression profile and biological activities of CD40, many attempts have been made to understand the role signaling plays in anti-tumor immunity.
Because of this, CD40-activated DC can be used for vaccination therapies in the context of cancer and infectious diseases such as tuberculosis.Citation15,Citation16 Another interesting outcome of CD40 signaling is a phenotype shift from the “alternatively activated” M2 spectrum to the anti-tumorigenic or “classically activated” M1 spectrum. This M1/M2-spectrum designation is especially relevant in regard to tumor-associated macrophages (TAM) in the context of cancer immunotherapy. The “classically activated” macrophages of the so-called M1 spectrum arise in response to GM-CSF and stimuli like IFNγ and LPS, and are usually considered to confer a pro-inflammatory immune response and provide antigen cross-presentation. M1 spectrum macrophages are mostly associated with favorable anti-tumoral immune responses.Citation17 “Alternatively activated” or M2 spectrum macrophages are generated from exposure to M-CSF and cytokines, such as IL-4 and IL-10 and are involved in tissue repair and resolution of an inflammatory state.Citation5 The tumor-associated M2 spectrum is appreciated as a key driver of cancer progression and metastasis.Citation18 The tight interaction of tumor and infiltrating macrophages strongly shapes the tumor microenvironment in establishing inflammatory or pro-tumorigenic local conditions. It has been proposed that skewing the M2/M1/spectrum balance within the tumor should improve the anti-tumor immune response by favoring infiltration and activation of cytotoxic T cells.Citation19-Citation21 This has been described for multiple models, such as pancreatic cancer and a mouse xenograft model of glioblastoma.Citation22 The repolarization of macrophages is consistent with CD40 activation-induced downregulation of CD206 and increased expression of CD54, indicating a change in migratory capacity and efficient macrophage activation.Citation23-Citation26
Interestingly, despite the broad expression of CD40 in many tissues, tumor cells seem to be susceptible to CD40-induced killing by membrane-bound CD40L.Citation27 Membrane-bound CD40L expressed on DC can directly induce apoptosis in CD40-positive tumor cellsCitation28 and T cell-independent cytostatic activity was attributed to CD40 stimulated IFNγ primed macrophages in a murine model of B16 melanoma.Citation29 Part of this phenomenon might not be a direct effect of CD40 signaling, but of secondary upregulation of death receptors on susceptible cell types.Citation30 However, some clinical effect of the anti-CD40 antibodies lucatumumab and dacetuzumab has been shown against B cell malignancies, which could be attributed to antibody-mediated phagocytosis by macrophages and induction of antibody-dependent cytotoxicity (ADCC).Citation31 Following ligation of CD40, tumor cells show signs of DNA damage, secrete pro-angiogenic factors, like VEGF and IL-8, and generally adopt a state reflecting a senescence-associated secretory phenotype (SASP) due to CD40-induced NFκB activation.Citation32
CD40/CD40L structure, organization, and signal transduction pathways
TNF-R-SF signaling is a structurally well-defined event that requires proper three-dimensional receptor clustering and trimerization (). While the TNF-SF ligands naturally exist as trivalent functional units, the receptors are usually separated on the cell surface. TNF-SF ligands naturally assemble on the cell surface as homo-trimers containing three receptor-binding sites that are located at the three identical clefts between the neighboring monomers. Critically, receptor assembly into functional trimeric signaling complexes occurs through binding of the natural ligand unit. The interaction of these trimeric TNF-SF ligands with their corresponding receptors, expressed on the surface of other cells, leads to very precise receptor clustering. This extracellular receptor ordering by the ligands translates into close proximity of the intracellular domains of the receptor followed by ordered assembly of the intracellular signaling complex.Citation33-Citation36 The trimeric structure of the TNF-SF ligands and the resulting receptor clusters are prerequisites for productive signal transmission into the cell. Due to these specific requirements, monovalent and bivalent approaches have generally proven to be minimally effective in vivo.Citation34-Citation36
Figure 1. Requirements for TNF-R-SF signaling.
TNF-R-SF signaling is a structurally well-defined event that requires proper three-dimensional receptor clustering and trimerization. A. The TNF-SF ligands naturally exist as trivalent functional units and the receptors are usually separated on the cell surface. B. The assembled TNF-SF ligands contain three receptor-binding sites that are located at the three identical clefts between the neighboring monomers. C. Receptor assembly into functional trimeric signaling complexes occurs through binding of the natural ligand unit. D. The interaction of these trimeric TNF-SF ligands with their corresponding receptors, expressed on the surface of other cells, leads to very precise receptor clustering followed by intracellular signal transduction.
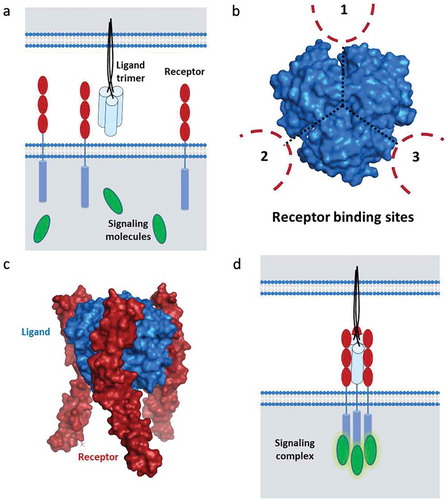
Like other members of the TNF-R-SF, CD40 is a membrane-bound molecule that can be activated by the membrane-bound ligand through direct cell-to-cell contact but also by the soluble ligand in solution. A diverse spectrum of downstream molecular and cellular processes has been shown to be regulated by CD40 engagement.Citation37 Ligation of the receptor by the cognate ligand induces the formation of spatially well-defined and trimeric signaling complexes, facilitating recruitment of TNF receptor-associated factors (TRAFs) and the NFκB activator-1 (Act1). The particular composition of the signaling complex, primarily depending on the cell type, triggers various pathways. For example, association with TRAF6 mainly activates the JAK/Stat3 pathway and TRAF1/2 induce MKK/p38/ERK1/2 signaling, Act1 has multiple functions in activation of the NFkB pathway, JNK, and PI3K signaling and in conjunction with TRAF3 amplifies MKK/p38/ERK1/2 signaling. Moreover, depending on the specific context, CD40 ligation can activate both the “classical” and non-canonical NFkB pathway.Citation38
Implications for development of CD40 agonists
Based on their unique receptor clustering patterns, generating productive downstream signals from TNF-R-SF receptors is dependent on agonistic compounds having a very precise structure and three-dimensional organization.Citation39 Due to the critical role that CD40 plays in anti-tumor immune responses, various strategies to induce CD40 signaling have been explored. They can be broadly grouped into agonistic antibody-based or CD40L-based approaches.Citation36 The latter group can be further divided into recombinant protein approaches, using CD40L mimetics, and gene therapy approaches, introducing the CD40L gene into target cells. The first CD40-targeting clinical trials were initiated over 20 years ago. These initial trials tested recombinant CD40L as well as CD40L-overexpressing cellular vaccines. The first agonistic antibody trials, using CP-870,893 and SGN-40, began approximately five years later. These early clinical trials showed some encouraging results; however, the clinical activity has been limited due to a number of potential factors.Citation40-Citation42 The next generation of CD40 agonists has been designed to overcome these limitations. Currently, seven antibodies () and four CD40L-based approaches are in active clinical trials (). In this section, we will discuss the main characteristics as well as the strengths and weaknesses of the three main approaches.
Table 1. Antibody-based CD40-targeting compounds in ongoing clinical trials.
Table 2. Gene therapy and recombinant CD40 agonists in ongoing clinical trials.
Antibodies
Monoclonal antibody technology, described by Köhler and Milstein in the 1970s, has revolutionized science and medicine. Antibodies are 150-kDa Y-shaped proteins produced by B cells. The tips of the two “Y” arms, located at the amino-terminal of the molecule, each contains an antigen-binding site, or paratope, that binds to a specific epitope on a given protein. The carboxy-terminal end is called the Fc region, or Fragment crystallizable region, and it contains the constant-region segments that define the isotype, or class, of the specific antibody. This region determines the specific immune response generated by an antibody by binding to specific Fc receptors expressed by many cell populations as well as complement proteins. Interestingly, although there are five isotypes in mammals, IgA, IgD, IgE, IgG, and IgM, all therapeutic antibodies use one of the four IgG subclasses.Citation43
The most important feature of antibodies is the high specificity for their cognate epitope. This specificity is determined by the complementarity determining regions (CDR) located in the antigen-binding site. Importantly, antibodies are bivalent regarding target binding, meaning that they have two epitope-binding sites per molecule. In contrast to the natural ligands, which bind their cognate receptor in a specific well-defined manner, antibodies can specifically bind to multiple unique epitopes, both linear and conformational, on a given protein. Importantly, the location of the epitope plays a critical role in the activity. This was originally demonstrated by showing that antibodies with distinct epitopes produce distinct CD40 signaling cascades following binding.Citation44-Citation46 This idea has been further refined more recently where the stimulatory activity of human anti-CD40 antibodies was shown to decrease as epitopes become closer to the cell membrane.Citation47
Interestingly, the authors speculate that the importance of the epitope location is actually related to the accessibility of the Fc domain. Specifically, while the Fc domain of antibodies that binds distal to the cell surface is accessible to Fcγ receptors (FcγR), the Fc domain of antibodies that binds closer to the membrane will not be able to optimally bind to FcγR. This highlights the importance that both the antigen-binding site and Fc domain play in activity. Because of their bivalent nature, anti-CD40 antibodies, unless crosslinked, do not provide the required clustering capacity for significant efficacy.Citation48 In fact, therapeutic antibodies, especially anti-CD40 antibodies, frequently rely on secondary crosslinking via Fcγ receptors (FcγR) for biological activity.Citation48,Citation49 These Fc/FcR interactions result in additional responses. For example, the Fc region mediates complement-dependent cytotoxicity (CDC) or ADCC of the target cell, which as mode-of-action is sometimes desirable and used for tumor targeting purposes. Indeed, ADCC is the underlying cause for the activity of the anti-CD40 antibody lucatumumab in treatment of chronic lymphocytic lymphoma as well as other antibodies used as cancer therapeutics such as trastuzumab (anti-HER2), rituximab (anti-CD20) and varlilumab (anti-CD27).Citation31,Citation50-Citation52 This means that both ends of the antibody play critical roles in the biological function. In terms of anti-CD40 antibodies, this also means that they have a mixed mode of action. This becomes especially important when discussing the therapeutic index of anti-CD40 antibodies, comparing efficacy and safety.
The absence of significant clinical activity observed with early anti-CD40 antibodies has resulted in a number of modifications. Currently, seven unique anti-CD40 antibodies are in active clinical trials (), including CP-870,893, and some results have been recently published.Citation53-Citation55 These new studies have focused on investigating new epitopes, new Fc region design, and combination therapy in order to improve the limited clinical efficacy observed with previous compounds.
CD40L-based cellular and gene therapy
The earliest CD40-targeting approaches involved engineering the overexpression of CD40L in various cell populations. Currently, this can be further divided into two main approaches: CD40L-expressing cellular vaccines and CD40L-specific gene therapy using delivery systems such as oncolytic viruses.Citation56 Since the complexity of both of these topics is outside of the focus of this review, we would recommend a number of recent reviews.Citation57-Citation59 Both approaches are based on using the natural ligand (CD40L) to stimulate CD40 signaling, which we will cover in the next section. Alternatively, since DC are one of the main target cells for CD40L and cross-presentation of antigens, one way to improve efficacy and sensitivity of this cell type is transduction with CD40 and subsequent use as an improved vaccine vehicle. An autologous dendritic cell-based vaccine with in vivo activation of inducible CD40 is already in clinical trials for advanced prostate cancer.Citation16
The previous trials using these approaches have also been associated with limited clinical activity. They are also complicated, from a development standpoint, by the addition of cellular and gene therapy components. A number of recent developments, including active clinical trials and publications, have demonstrated the potential of these approaches. For example, CD40L-expressing Chimeric Antigen Receptor (CAR) T cells showed enhanced anti-tumor activity in preclinical models.Citation60 This approach combines the specificity of the CAR T cells target with CD40L-based costimulation, making it a “cellular bispecific”. Clearly, the results suggest that cellular vaccines are not the only adoptive cell therapy (ACT) that might benefit from targeting CD40. Additional developments in both the delivery method and the format of CD40L have also shown promise.Citation30,Citation56,Citation61-Citation63
CD40L-based recombinant proteins (natural ligand mimetics)
The last approach involves directly injecting soluble recombinant CD40L. These compounds are molecular mimics of the endogenous CD40L that specifically and exclusively interacts, via the receptor-binding domain, with CD40. This has been approached by using purified CD40L, stabilized trivalent/trimeric forms of CD40L and, more recently, hexavalent/hexameric forms of CD40L. As we have discussed earlier, CD40 needs to be correctly trimerized in order to transmit a productive signal. Although purified CD40L tends to trimerize in solution, the CD40L approaches have not been very successful. However, the stabilized trimeric forms, such as the isoleucine zipper CD40L formats, have shown some activity in combination with IL-6 blockade.Citation62
Recent results have shown that higher-order hexavalent approaches are superior for transmitting signals via the TNF-R-SF.Citation64-Citation67 The hexavalent agonists comprise a family of co-stimulatory TNF-SF ligands that specifically bind their cognate receptors on target cells and induce clustering of six receptor chains in a spatially well-defined manner. The specific compounds, such as HERA-CD40LCitation66 and MEDI5083 (NCT03089645), are fusion proteins composed of a trivalent but single-chain CD40L-receptor-binding domain (scCD40L-RBD), linked to a human IgG thereby generating a hexavalent molecule.
HERA-CD40L is designed with an Fc-silenced IgG1, which enables efficient receptor agonism on CD40-expressing cells in the absence of FcγR-mediated cross-linking. Strong activation of NFκB signaling was observed upon treatment of B cells with HERA-CD40L. Monocyte treatment with HERA-CD40L promoted differentiation toward the M1 spectrum and re-polarization of M2 spectrum macrophages toward the M1 spectrum phenotype. Treatment of in vitro co-cultures of T and B cells with HERA-CD40L triggered robust anti-tumor activation of T cells, which depended upon direct interaction with B cells. In vivo, a murine surrogate of HERA-CD40L stimulated clonal expansion of antigen-specific T cells and showed single-agent anti-tumor activity in the CD40-negative syngeneic MC38-CEA mouse model of colorectal cancer.Citation66
Although recombinant CD40L was among the first techniques used to target CD40, the promise of this approach is only recently being realized by combining the knowledge of the importance of trimerization and three-dimensional organization together with higher-order CD40L-based compounds. Since these compounds are true agonists that do not require FcγR-mediated crosslinking, they should have a superior safety profile compared to other approaches. New developments using trivalent, tetravalentCitation68 and hexavalent formats will continue to be investigated. As we will discuss in the next section, signaling through the TNF-R-SF needs to be carefully controlled. This is another example of the Goldilocks principle, where too little clustering results in no signal while too much clustering leads to overstimulation.
Comparison between anti-CD40 antibody- and CD40L-based approaches
While there have been no direct clinical comparisons between anti-CD40 antibody- and CD40L-based approaches, there have been many preclinical comparisons. One important caveat needs to be mentioned regarding the interpretation of mouse studies. The majority of mouse studies employ anti-CD40 antibodies with a xenogeneic origin. For example, clones FGK45 and 3/23 originate in rat and clones HM40-3 and 1C10 in hamster. The differences in the affinity of these isotypes to mouse Fc receptors as well as the development of anti-“drug” antibodies complicate the interpretation of the role of Fc receptor binding in activity. Studies using fully murine antibodies or human compounds in humanized mouse models, that express the human target as well as human Fc receptors, can help to clarify the results of mouse studies.Citation48
Induction of CD40 signaling (true agonistic activity)
It has been shown that antibodies with unique epitopes elicit unique biological activities following binding.Citation46,Citation47 In fact, antibodies that will be labeled agonistic or antagonistic, opposite extremes of the biological activity spectrum, differ in their epitopes.Citation46,Citation47,Citation69 Direct comparisons between bivalent antibodies, trivalent CD40L, and hexavalent CD40L showed induction of unique signaling complexes and biological activities.Citation46,Citation66,Citation70 Treatment with these CD40-targeting compounds also resulted in significantly different biological responses. For example, the effect of the hexavalent HERA-CD40L on the expression of activation, differentiation, and antigen-presentation markers was generally stronger than stimulation by trivalent CD40L or bivalent anti-CD40 antibodies.Citation66 Importantly, the weak activity displayed by the trivalent CD40L and antibodies was critically depended on crosslinking. Based on these findings it is becoming clearer that purified soluble bivalent antibodies are not suitable TNF-R-SF agonists. In contrast, the CD40L-based approaches, especially the soluble hexavalent CD40L molecules are able to provide a true agonistic signal via a defined single mode of action. The biological activity that accompanies treatment with anti-CD40 antibodies can be attributed to two modes of action. First, limited agonistic activity that is due to antibody aggregation or FcγR-mediated clustering. Second, agonist-independent activity that also depends on the specific epitope (epitope driven) and/or FcγR binding (Fc-domain driven). These unwanted activities result in significant disadvantages () compared to FcγR binding-deficient CD40L-based approaches.
Figure 2. Side effects of CD40 agonistic antibodies versus fusion molecules.
The orientation of the target epitope of CD40 agonistic antibodies within the CD40 molecule defines epitope-driven effects. In case the antibody prevents binding of the natural CD40L it inevitably inhibits favorable natural CD40 signaling. Contrarily, cross-linking receptor complexes that have been trimerized by the natural ligand can lead to hyper-clustering and uncontrolled overstimulation with increased risk of toxic side effects and exhaustion of immune cells. Fc-domain driven effects derive from the interaction of the antibody´s Fc domain with Fc receptors on different immune cells leading to unwanted (back-)signaling. Upon Fc receptor engagement these immune cells exert diverse effector functions such as ADCC thereby not only depleting the target cell population but also contributing to unspecific immune system activation. In contrast, agonistic fusion proteins with a hexavalent/Fc-silent structure preclude the aforementioned characteristics, possibly generating a more physiologic activity.
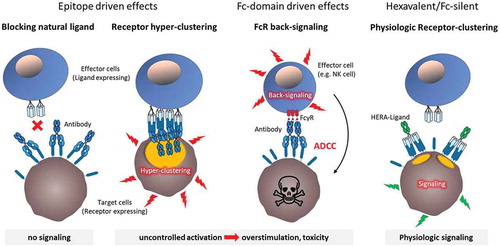
Side effects (independent of agonistic activity)
Unfortunately, agonist anti-CD40 antibody treatment is generally associated with toxicity in the clinic.Citation41,Citation71 A common set of adverse events is observed at higher doses in humans and mice.Citation41,Citation48,Citation71,Citation72 For example, cytokine release syndrome (CRS) can be observed within minutes to hours after treatment and hepatotoxicity occur within 24 h after treatment and can persist for weeks.Citation42,Citation73 Due to these effects, an MTD has been determined for systemically administered anti-CD40 antibodies used in the clinic, which ranges from 0.06 to 0.2 mg/kg.Citation74 These effects have been hypothesized to be related to multiple factors, including the expression of CD40 (and FcγR) by platelets and endothelial cells as well as antibody-specific characteristics (see ).Citation73 More recently, the clinical discussion around anti-CD40 antibodies has shifted from toxicity to therapeutic index (TI). The TI is a relative quantitative measurement of drug safety that compares the amount of a drug that causes a therapeutic effect to the amount that causes toxicity. Due to dose-limiting toxicities, the anti-CD40 antibodies in development may never reach an optimal therapeutic dose.Citation71 One alternative approach to reducing systemic side effects, local intratumoral administration of anti-CD40 antibodies, has shown promise in a number of preclinical models.Citation61,Citation71,Citation75 However, comparing intraveneous to intratumoral anti-CD40 antibody injection in a Phase I clinical trial revealed no significant overall differences in the TI; however, intratumoral administration at certain tumor locations was associated with a more favorable TI when compared to other locations.Citation54 This route of treatment needs to be further investigated and may only be applicable to certain types of tumors.
While the relationship between epitope location and function remains to be determined, it is clear that some antibodies block binding of the natural ligand, some enhance binding of the natural ligand and other have no influence on binding (). These epitope-driven side effects can prevent the activity of natural ligand, thus interfering with the anti-tumor immune response. Other antibodies can enhance ligand binding, thereby favoring receptor hyper clustering and overstimulation of the immune response, with increasing risk of toxic side effects and tumor-specific T cell exhaustion.Citation76
Back-signaling in FcγR-expressing cells, including phagocytic cells, granulocytes, as well as some lymphocyte populations, is responsible for Fc-domain-driven side effects (). Interestingly, many of these cell populations express both CD40 and FcγR. One of the most critical outcomes of FcγR-mediated back-signaling is depletion of CD40-expressing immune cells by ADCC. This potentially results in unwanted depletion of important cell populations, including monocytes, DC and B cells, which can compromise an efficient anti-tumor response. Activation of FcγR-expressing cell populations can also lead to over-stimulation of the immune response and could be responsible for the CRS seen following anti-CD40 treatment. In fact, hepatotoxicity is associated with macrophage-dependent cytokine production and in mouse models, anti-CD40 antibody-mediated toxicity is abrogated by depletion of FcγR-expressing Kupffer cells within liver sinusoids.Citation71,Citation72
Furthermore, lethal hepatotoxicity of CD40 agonistic antibody occurred when given prior to chemotherapy, but this could be circumvented by reversing the order of application.Citation72 These findings suggest that antibody-based approaches could have trouble as part of combinations with conventional anti-tumor treatments. In addition, due to the toxicities observed with anti-CD40 antibody therapy, many groups are experimenting with alternative administration routes, including intra-tumoral injections, which could also complicate combination therapy.Citation71,Citation77
Bispecific approaches targeting CD40
Bispecific CD40/targeting concepts comprise a number of different approaches, such as the generation of enhanced CAR-T cells (“cellular bispecifics”) expressing the CAR in combination with additional factors like CD40L,Citation60 antibodies recognizing two different epitopes either in cis or trans and even so-called bifunctional molecules with one targeting domain and one functional domain combined in one molecule. The development of bispecific CD40 agonists is challenging due to the ambiguous expression profile of CD40, nevertheless attempts have been made to target CD40-expressing tumors using CD40/mesothelin-bispecific constructs (ABBV-428, NCT02955251, currently recruiting), CD40/HER2-bispecific constructsCitation78 and so-called duokines.Citation79 Additional CD40 bispecifics are on the way, including CEA and others as tumor-targeting antigen.Citation80
Combination treatment with other therapies
Given the general expression profile and biological activities of CD40, it is obvious that the combination of CD40 agonists with other therapeutic options has been investigated in preclinical models. The use of CD40-targeted therapies with other immunomodulators or checkpoint inhibitors has shown great potential in various cancer models. For example, preclinical studies using agonistic anti-CD40 antibodies in combination with chemotherapyCitation81 or kinase inhibitorsCitation82 have shown promising results.
Radiotherapy (RT) is one of the pillars in cancer therapy and up to 70% of all cancer patients receive RT throughout their treatment regimen. One intended effect of RT is to release tumor antigens and establish a pro-inflammatory local TME. Consequently, application of CD40 agonists to enhance an RT-induced vaccination effect has been studied intenselyCitation83-Citation85 and demonstrated efficacy in multiple preclinical models. Eventually, modulation of the TME aims at activating/priming anti-tumoral cytotoxic T cells, which can be further supported using checkpoint inhibition blockade via PD-1, PD-L1 or CTLA-4. These treatments have demonstrated their ability to enhance remodeling of the macrophage compartment of non-immunogenic tumor models.Citation86,Citation87 Several combinations of CD40 antibodies with checkpoint inhibitors, in particular anti-PD-L1 and CTLA-4 antibodies, have been described in preclinical modelsCitation86,Citation88-Citation90 and recently showed some evidence of clinical activity in a non-controlled Phase I trial.Citation55
Additionally, CD40 ligation has proven therapeutic potential in combination with tumor vaccination strategies in mouse models of glioma and other indicationsCitation91 and improved the efficacy of adoptive cell transfer (ACT) therapy in a murine B16 melanoma model.Citation92 Finally, in addition to the CD40L-expressing CAR-T cells mentioned earlier, there is some evidence that combination treatment with CAR-T cells and anti-CD40 agonists could improve the anti-tumor immune response in a mouse model of pancreatic cancer.Citation93 Although these preliminary results are encouraging, properly controlled and randomized clinical studies will have to be performed before the true potential of CD40-targeting combination therapy will be understood.
Conclusion and outlook
Strategies to boost anti-tumor immune responses are among the most promising new developments in oncology and TNF-R-SF members, such as CD40, are important targets. Due to the unique requirements for generating productive TNF-R-SF signaling, agonistic compounds must generate very precise receptor structure and three-dimensional organization. Although various strategies to induce CD40 signaling have been explored, two decades of limited clinical success suggest that new approaches need to be explored. The profiling of bivalent anti-CD40 antibodies, recombinant trivalent CD40L, and recombinant hexavalent CD40L-based fusion proteins, has revealed significant differences in CD40-ligation induced signaling. The single-chain TNF-SF-RBD mimetics induce optimal trimeric assembly of the TNF-R-SF receptors, thereby enabling new drug concepts: If exploited as “2-fold-3”, or pairs of trimers, hexavalent fusion proteins, their agonistic activity is independent of additional crosslinking via FcγR. The hexavalent approach to target additional TNF-R-SF members with dedicated agonist molecules is underway and warrants further preclinical and clinical studies. Due to the unifying architectural features of the TNF-R-SF, this concept has been demonstrated for multiple family members.Citation65-Citation67
Despite the undoubted importance of CD40/CD40L as an initiator of anti-tumor immune response, the true power of this signaling pathway has not yet been fully unleashed by the agonists in clinical development. The limited clinical efficacy of current CD40 agonists results from structural and functional characteristics of antibodies, including the presence of only two target-binding sites per molecule, that are unsuitable for stimulating the TNF-R-SF. Thanks to the wide range of possibilities to target this signaling pathway also in combination with other drugs and therapies, systemically or locally, the near future will hopefully yield more clinical data, improve therapeutic efficacy and broaden the array of treatment options for many cancer patients.
Disclosure of potential conflicts of interest
All Authors are employees of Apogenix AG.
References
- Coulie PG, Van den Eynde BJ, van der Bruggen P, Boon T. Tumour antigens recognized by T lymphocytes: at the core of cancer immunotherapy. Nat Rev Cancer. 2014;14(2):135–46. doi:10.1038/nrc3670.
- Suek N, Campesato LF, Merghoub T, Khalil DN. Targeted APC activation in cancer immunotherapy to enhance the abscopal effect. Front Immunol. 2019;10:604. doi:10.3389/fimmu.2019.00604.
- Quezada SA, Jarvinen LZ, Lind EF, Noelle RJ. CD40/CD154 interactions at the interface of tolerance and immunity. Annu Rev Immunol. 2004;22:307–28. doi:10.1146/annurev.immunol.22.012703.104533.
- Korniluk A, Kemona H, Dymicka-Piekarska V. Multifunctional CD40L: pro- and anti-neoplastic activity. Tumour Biol. 2014;35(10):9447–57. doi:10.1007/s13277-014-2407-x.
- Hamilton TA, Zhao C, Pavicic PG Jr., Datta S. Myeloid colony-stimulating factors as regulators of macrophage polarization. Front Immunol. 2014;5:554. doi:10.3389/fimmu.2014.00554.
- Mia S, Warnecke A, Zhang XM, Malmstrom V, Harris RA. An optimized protocol for human M2 macrophages using M-CSF and IL-4/IL-10/TGF-beta yields a dominant immunosuppressive phenotype. Scand J Immunol. 2014;79(5):305–14. doi:10.1111/sji.12162.
- Fujihara C, Williams JA, Watanabe M, Jeon H, Sharrow SO, Hodes RJ. T cell-B cell thymic cross-talk: maintenance and function of thymic B cells requires cognate CD40-CD40 ligand interaction. J Immunol. 2014;193(11):5534–44. doi:10.4049/jimmunol.1401655.
- Koncz G, Hueber AO. The Fas/CD95 receptor regulates the death of autoreactive B cells and the selection of antigen-specific B cells. Front Immunol. 2012;3:207. doi:10.3389/fimmu.2012.00207.
- van Zelm MC, Bartol SJ, Driessen GJ, Mascart F, Reisli I, Franco JL, Wolska-Kusnierz B, Kanegane H, Boon L, van Dongen JJ, et al. Human CD19 and CD40L deficiencies impair antibody selection and differentially affect somatic hypermutation. J Allergy Clin Immunol. 2014;134(1):135–44. doi:10.1016/j.jaci.2013.11.015.
- Coler RN, Hudson T, Hughes S, Huang PW, Beebe EA, Orr MT. Vaccination produces CD4 T cells with a novel CD154-CD40-dependent cytolytic mechanism. J Immunol. 2015;195(7):3190–97. doi:10.4049/jimmunol.1501118.
- Mathieu M, Cotta-Grand N, Daudelin JF, Boulet S, Lapointe R, Labrecque N. CD40-activated B cells can efficiently prime antigen-specific naive CD8+ T cells to generate effector but not memory T cells. PLoS One. 2012;7(1):e30139. doi:10.1371/journal.pone.0030139.
- Kondo E, Gryschok L, Klein-Gonzalez N, Rademacher S, Weihrauch MR, Liebig T, Shimabukuro-Vornhagen A, Kochanek M, Draube A, von Bergwelt-Baildon MS. CD40-activated B cells can be generated in high number and purity in cancer patients: analysis of immunogenicity and homing potential. Clin Exp Immunol. 2009;155(2):249–56. doi:10.1111/j.1365-2249.2008.03820.x.
- Liebig TM, Fiedler A, Zoghi S, Shimabukuro-Vornhagen A, von Bergwelt-Baildon MS. Generation of human CD40-activated B cells. J Vis Exp. 2009;(32). doi:10.3791/1373.
- Wennhold K, Weber TM, Klein-Gonzalez N, Thelen M, Garcia-Marquez M, Chakupurakal G, Fiedler A, Schlosser HA, Fischer R, Theurich S, et al. CD40-activated B cells induce anti-tumor immunity in vivo. Oncotarget. 2017;8(17):27740–53. doi:10.18632/oncotarget.7720.
- Griffiths KL, Ahmed M, Das S, Gopal R, Horne W, Connell TD, Moynihan KD, Kolls JK, Irvine DJ, Artyomov MN, et al. Targeting dendritic cells to accelerate T-cell activation overcomes a bottleneck in tuberculosis vaccine efficacy. Nat Commun. 2016;7:13894. doi:10.1038/ncomms13894.
- Sonpavde G, McMannis JD, Bai Y, Seethammagari MR, Bull JMC, Hawkins V, Dancsak TK, Lapteva N, Levitt JM, Moseley A, et al. Phase I trial of antigen-targeted autologous dendritic cell-based vaccine with in vivo activation of inducible CD40 for advanced prostate cancer. Cancer Immunol Immunother. 2017;66(10):1345–57. doi:10.1007/s00262-017-2027-6.
- Luheshi N, Davies G, Legg J. Understanding the influence of the tumor microenvironment on macrophage responses to CD40 agonists. Oncoimmunology. 2014;3(2):e27615. doi:10.4161/onci.27615.
- Nielsen SR, Schmid MC. Macrophages as key drivers of cancer progression and metastasis. Mediators Inflamm. 2017;2017:1–11. doi:10.1155/2017/9624760.
- Heusinkveld M, de Vos van Steenwijk PJ, Goedemans R, Ramwadhdoebe TH, Gorter A, Welters MJ, van Hall T, van der Burg SH. M2 macrophages induced by prostaglandin E2 and IL-6 from cervical carcinoma are switched to activated M1 macrophages by CD4+ Th1 cells. J Immunol. 2011;187(3):1157–65. doi:10.4049/jimmunol.1100889.
- Luheshi N, Davies G, Poon E, Wiggins K, McCourt M, Legg J. Th1 cytokines are more effective than Th2 cytokines at licensing anti-tumour functions in CD40-activated human macrophages in vitro. Eur J Immunol. 2014;44(1):162–72. doi:10.1002/eji.201343351.
- Xue N, Zhou Q, Ji M, Jin J, Lai F, Chen J, Zhang M, Jia J, Yang H, Zhang J, et al. Chlorogenic acid inhibits glioblastoma growth through repolarizating macrophage from M2 to M1 phenotype. Sci Rep. 2017;7:39011. doi:10.1038/srep39011.
- Byrne KT, Vonderheide RH. CD40 stimulation obviates innate sensors and drives T cell immunity in cancer. Cell Rep. 2016;15(12):2719–32. doi:10.1016/j.celrep.2016.05.058.
- Novosad J, Holicka M, Novosadova M, Krejsek J, Krcmova I. Rapid onset of ICAM-1 expression is a marker of effective macrophages activation during infection of Francisella tularensis LVS in vitro. Folia Microbiol (Praha). 2011;56(2):149–54. doi:10.1007/s12223-011-0028-y.
- Roszer T. Understanding the mysterious M2 macrophage through activation markers and effector mechanisms. Mediators Inflamm. 2015;2015:816460. doi:10.1155/2015/816460.
- Hoves S, Ooi CH, Wolter C, Sade H, Bissinger S, Schmittnaegel M, Ast O, Giusti AM, Wartha K, Runza V, et al. Rapid activation of tumor-associated macrophages boosts preexisting tumor immunity. J Exp Med. 2018;215(3):859–76. doi:10.1084/jem.20171440.
- Wiehagen KR, Girgis NM, Yamada DH, Smith AA, Chan SR, Grewal IS, Quigley M, Verona RI. Combination of CD40 agonism and CSF-1R blockade reconditions tumor-associated macrophages and drives potent antitumor immunity. Cancer Immunol Res. 2017;5(12):1109–21. doi:10.1158/2326-6066.CIR-17-0258.
- Bugajska U, Georgopoulos NT, Southgate J, Johnson PW, Graber P, Gordon J, Selby PJ, Trejdosiewicz LK. The effects of malignant transformation on susceptibility of human urothelial cells to CD40-mediated apoptosis. J Natl Cancer Inst. 2002;94(18):1381–95. doi:10.1093/jnci/94.18.1381.
- Hill KS, Errington F, Steele LP, Merrick A, Morgan R, Selby PJ, Georgopoulos NT, O’Donnell DM, Melcher AA. OK432-activated human dendritic cells kill tumor cells via CD40/CD40 ligand interactions. J Immunol. 2008;181(5):3108–15. doi:10.4049/jimmunol.181.5.3108.
- Buhtoiarov IN, Lum H, Berke G, Paulnock DM, Sondel PM, Rakhmilevich AL. CD40 ligation activates murine macrophages via an IFN-gamma-dependent mechanism resulting in tumor cell destruction in vitro. J Immunol. 2005;174(10):6013–22. doi:10.4049/jimmunol.174.10.6013.
- Schiza A, Wenthe J, Mangsbo S, Eriksson E, Nilsson A, Totterman TH, Loskog A, Ullenhag G. Adenovirus-mediated CD40L gene transfer increases Teffector/Tregulatory cell ratio and upregulates death receptors in metastatic melanoma patients. J Transl Med. 2017;15(1):79. doi:10.1186/s12967-017-1182-z.
- Byrd JC, Kipps TJ, Flinn IW, Cooper M, Odenike O, Bendiske J, Rediske J, Bilic S, Dey J, Baeck J, et al. Phase I study of the anti-CD40 humanized monoclonal antibody lucatumumab (HCD122) in relapsed chronic lymphocytic leukemia. Leuk Lymphoma. 2012;53(11):2136–42. doi:10.3109/10428194.2012.681655.
- Xu W, Li Y, Yuan WW, Yin Y, Song WW, Wang Y, Huang QQ, Zhao WH, Wu JQ. Membrane-bound CD40L promotes senescence and initiates senescence-associated secretory phenotype via NF-kappaB activation in lung adenocarcinoma. Cell Physiol Biochem. 2018;48(4):1793–803. doi:10.1159/000492352.
- Watts TH. TNF/TNFR family members in costimulation of T cell responses. Annu Rev Immunol. 2005;23:23–68. doi:10.1146/annurev.immunol.23.021704.115839.
- Wyzgol A, Muller N, Fick A, Munkel S, Grigoleit GU, Pfizenmaier K, Wajant H. Trimer stabilization, oligomerization, and antibody-mediated cell surface immobilization improve the activity of soluble trimers of CD27L, CD40L, 41BBL, and glucocorticoid-induced TNF receptor ligand. J Immunol. 2009;183(3):1851–61. doi:10.4049/jimmunol.0802597.
- Wajant H. Principles of antibody-mediated TNF receptor activation. Cell Death Differ. 2015;22(11):1727–41. doi:10.1038/cdd.2015.109.
- Mayes PA, Hance KW, Hoos A. The promise and challenges of immune agonist antibody development in cancer. Nat Rev Drug Discov. 2018;17(7):509–27. doi:10.1038/nrd.2018.75.
- Elgueta R, Benson MJ, de Vries VC, Wasiuk A, Guo Y, Noelle RJ. Molecular mechanism and function of CD40/CD40L engagement in the immune system. Immunol Rev. 2009;229(1):152–72. doi:10.1111/j.1600-065X.2009.00782.x.
- Seigner J, Basilio J, Resch U, de Martin R. CD40L and TNF both activate the classical NF-kappaB pathway, which is not required for the CD40L induced alternative pathway in endothelial cells. Biochem Biophys Res Commun. 2018;495(1):1389–94. doi:10.1016/j.bbrc.2017.11.160.
- An HJ, Kim YJ, Song DH, Park BS, Kim HM, Lee JD, Paik SG, Lee JO, Lee H. Crystallographic and mutational analysis of the CD40-CD154 complex and its implications for receptor activation. J Biol Chem. 2011;286(13):11226–35. doi:10.1074/jbc.M110.208215.
- Vonderheide RH. The immune revolution: a case for priming, not checkpoint. Cancer Cell. 2018;33(4):563–69. doi:10.1016/j.ccell.2018.03.008.
- Vonderheide RH, Glennie MJ. Agonistic CD40 antibodies and cancer therapy. Clin Cancer Res. 2013;19(5):1035–43. doi:10.1158/1078-0432.CCR-12-2064.
- Beatty GL, Li Y, Long KB. Cancer immunotherapy: activating innate and adaptive immunity through CD40 agonists. Expert Rev Anticancer Ther. 2017;17(2):175–86. doi:10.1080/14737140.2017.1270208.
- Wold ED, Smider VV, Felding BH. Antibody Therapeutics in Oncology. Immunotherapy (Los Angel). 2016;2(1). doi:10.4172/2471-9552.1000108.
- Heath AW, Wu WW, Howard MC. Monoclonal antibodies to murine CD40 define two distinct functional epitopes. Eur J Immunol. 1994;24(8):1828–34. doi:10.1002/eji.1830240816.
- Bjorck P, Paulie S. CD40 antibodies defining distinct epitopes display qualitative differences in their induction of B-cell differentiation. Immunology. 1996;87(2):291–95. doi:10.1046/j.1365-2567.1996.428508.x.
- Bankert KC, Oxley KL, Smith SM, Graham JP, de Boer M, Thewissen M, Simons PJ, Bishop GA. Induction of an altered CD40 signaling complex by an antagonistic human monoclonal antibody to CD40. J Immunol. 2015;194(9):4319–27. doi:10.4049/jimmunol.1402903.
- Yu X, Chan HTC, Orr CM, Dadas O, Booth SG, Dahal LN, Penfold CA, O’Brien L, Mockridge CI, French RR, et al. Complex interplay between epitope specificity and isotype dictates the biological activity of anti-human CD40 antibodies. Cancer Cell. 2018;33(4):664–675.e4. doi:10.1016/j.ccell.2018.02.009.
- Dahan R, Barnhart BC, Li F, Yamniuk AP, Korman AJ, Ravetch JV. Therapeutic activity of agonistic, human anti-CD40 monoclonal antibodies requires selective FcgammaR engagement. Cancer Cell. 2016;29(6):820–31. doi:10.1016/j.ccell.2016.05.001.
- Li F, Ravetch JV. Inhibitory Fcgamma receptor engagement drives adjuvant and anti-tumor activities of agonistic CD40 antibodies. Science. 2011;333(6045):1030–34. doi:10.1126/science.1206954.
- Collins DM, O’Donovan N, McGowan PM, O’Sullivan F, Duffy MJ, Crown J. Trastuzumab induces antibody-dependent cell-mediated cytotoxicity (ADCC) in HER-2-non-amplified breast cancer cell lines. Ann Oncol. 2012;23(7):1788–95. doi:10.1093/annonc/mdr484.
- Burris HA, Infante JR, Ansell SM, Nemunaitis JJ, Weiss GR, Villalobos VM, Sikic BI, Taylor MH, Northfelt DW, Carson WE 3rd, et al. Safety and activity of varlilumab, a novel and first-in-class agonist anti-CD27 antibody, in patients with advanced solid tumors. J Clin Oncol. 2017;35(18):2028–36. doi:10.1200/JCO.2016.70.1508.
- Maloney DG, Grillo-Lopez AJ, White CA, Bodkin D, Schilder RJ, Neidhart JA, Janakiraman N, Foon KA, Liles TM, Dallaire BK, et al. IDEC-C2B8 (Rituximab) anti-CD20 monoclonal antibody therapy in patients with relapsed low-grade non-Hodgkin’s lymphoma. Blood. 1997;90:2188–95.
- Vitale LA, Thomas LJ, He LZ, O’Neill T, Widger J, Crocker A, Sundarapandiyan K, Storey JR, Forsberg EM, Weidlick J, et al. Development of CDX-1140, an agonist CD40 antibody for cancer immunotherapy. Cancer Immunol Immunother. 2019;68(2):233–45. doi:10.1007/s00262-018-2267-0.
- Irenaeus SMM, Nielsen D, Ellmark P, Yachnin J, Deronic A, Nilsson A, Norlen P, Veitonmaki N, Wennersten CS, Ullenhag GJ. First-in-human study with intratumoral administration of a CD40 agonistic antibody, ADC-1013, in advanced solid malignancies. Int J Cancer. 2019;145:1189–99. doi:10.1002/ijc.32141.
- Bajor DL, Mick R, Riese MJ, Huang AC, Sullivan B, Richman LP, Torigian DA, George SM, Stelekati E, Chen F, et al. Long-term outcomes of a phase I study of agonist CD40 antibody and CTLA-4 blockade in patients with metastatic melanoma. Oncoimmunology. 2018;7(10):e1468956. doi:10.1080/2162402X.2018.1468956.
- Zafar S, Parviainen S, Siurala M, Hemminki O, Havunen R, Tahtinen S, Bramante S, Vassilev L, Wang H, Lieber A, et al. Intravenously usable fully serotype 3 oncolytic adenovirus coding for CD40L as an enabler of dendritic cell therapy. Oncoimmunology. 2017;6(2):e1265717. doi:10.1080/2162402X.2016.1265717.
- Saxena M, Bhardwaj N. Turbocharging vaccines: emerging adjuvants for dendritic cell based therapeutic cancer vaccines. Curr Opin Immunol. 2017;47:35–43. doi:10.1016/j.coi.2017.06.003.
- Hu Z, Ott PA, Wu CJ. Towards personalized, tumour-specific, therapeutic vaccines for cancer. Nat Rev Immunol. 2018;18(3):168–82. doi:10.1038/nri.2017.131.
- Dunbar CE, High KA, Joung JK, Kohn DB, Ozawa K, Sadelain M. Gene therapy comes of age. Science. 2018;359(6372). doi:10.1126/science.aan4672.
- Kuhn NF, Purdon TJ, van Leeuwen DG, Lopez AV, Curran KJ, Daniyan AF, Brentjens RJ. CD40 ligand-modified chimeric antigen receptor T cells enhance antitumor function by eliciting an endogenous antitumor response. Cancer Cell. 2019;35(3):473–488.e6. doi:10.1016/j.ccell.2019.02.006.
- Singh M, Vianden C, Cantwell MJ, Dai Z, Xiao Z, Sharma M, Khong H, Jaiswal AR, Faak F, Hailemichael Y, et al. Intratumoral CD40 activation and checkpoint blockade induces T cell-mediated eradication of melanoma in the brain. Nat Commun. 2017;8(1):1447. doi:10.1038/s41467-017-01572-7.
- Eriksson E, Milenova I, Wenthe J, Moreno R, Alemany R, Loskog A. IL-6 signaling blockade during CD40-mediated immune activation favors antitumor factors by reducing TGF-beta, collagen type I, and PD-L1/PD-1. J Immunol. 2019;202(3):787–98. doi:10.4049/jimmunol.1800717.
- Irenaeus S, Schiza A, Mangsbo SM, Wenthe J, Eriksson E, Krause J, Sundin A, Ahlstrom H, Totterman TH, Loskog A, et al. Local irradiation does not enhance the effect of immunostimulatory AdCD40L gene therapy combined with low dose cyclophosphamide in melanoma patients. Oncotarget. 2017;8(45):78573–87. doi:10.18632/oncotarget.19750.
- Richards DM, Marschall V, Billian-Frey K, Heinonen K, Merz C, Redondo Muller M, Sefrin JP, Schroder M, Sykora J, Fricke H, et al. HERA-GITRL activates T cells and promotes anti-tumor efficacy independent of FcgammaR-binding functionality. J Immunother Cancer. 2019;7(1):191. doi:10.1186/s40425-019-0671-4.
- Gieffers C, Kluge M, Merz C, Sykora J, Thiemann M, Schaal R, Fischer C, Branschadel M, Abhari BA, Hohenberger P, et al. APG350 induces superior clustering of TRAIL receptors and shows therapeutic antitumor efficacy independent of cross-linking via Fcgamma receptors. Mol Cancer Ther. 2013;12(12):2735–47. doi:10.1158/1535-7163.MCT-13-0323.
- Merz C, Sykora J, Marschall V, Richards DM, Heinonen K, Redondo Muller M, Thiemann M, Schnyder T, Fricke H, Hill O, et al. The hexavalent CD40 agonist HERA-CD40L induces T-cell-mediated antitumor immune response through activation of antigen-presenting cells. J Immunother. 2018;41(9):385–98. doi:10.1097/CJI.0000000000000246.
- Thiemann M, Richards DM, Heinonen K, Kluge M, Marschall V, Merz C, Redondo Muller M, Schnyder T, Sefrin JP, Sykora J, et al. A single-chain-based hexavalent CD27 agonist enhances T cell activation and induces anti-tumor immunity. Front Oncol. 2018;8:387. doi:10.3389/fonc.2018.00387.
- Lai N, Min Q, Xiong E, Liu J, Zhang L, Yasuda S, Wang JY. A tetrameric form of CD40 ligand with potent biological activities in both mouse and human primary B cells. Mol Immunol. 2019;105:173–80. doi:10.1016/j.molimm.2018.11.018.
- Yamniuk AP, Suri A, Krystek SR, Tamura J, Ramamurthy V, Kuhn R, Carroll K, Fleener C, Ryseck R, Cheng L, et al. Functional antagonism of human CD40 achieved by targeting a unique species-specific epitope. J Mol Biol. 2016;428(14):2860–79. doi:10.1016/j.jmb.2016.05.014.
- Baccam M, Bishop GA. Membrane-bound CD154, but not CD40-specific antibody, mediates NF-kappaB-independent IL-6 production in B cells. Eur J Immunol. 1999;29(12):3855–66. doi:10.1002/(SICI)1521-4141(199912)29:12<3855::AID-IMMU3855>3.0.CO;2-S.
- Knorr DA, Dahan R, Ravetch JV. Toxicity of an Fc-engineered anti-CD40 antibody is abrogated by intratumoral injection and results in durable antitumor immunity. Proc Natl Acad Sci U S A. 2018;115(43):11048–53. doi:10.1073/pnas.1810566115.
- Byrne KT, Leisenring NH, Bajor DL, Vonderheide RH. CSF-1R-dependent lethal hepatotoxicity when agonistic CD40 antibody is given before but not after chemotherapy. J Immunol. 2016;197(1):179–87. doi:10.4049/jimmunol.1600146.
- Medina-Echeverz J, Ma C, Duffy AG, Eggert T, Hawk N, Kleiner DE, Korangy F, Greten TF. Systemic agonistic anti-CD40 treatment of tumor-bearing mice modulates hepatic myeloid-suppressive cells and causes immune-mediated liver damage. Cancer Immunol Res. 2015;3(5):557–66. doi:10.1158/2326-6066.CIR-14-0182.
- Vonderheide RH, Flaherty KT, Khalil M, Stumacher MS, Bajor DL, Hutnick NA, Sullivan P, Mahany JJ, Gallagher M, Kramer A, et al. Clinical activity and immune modulation in cancer patients treated with CP-870,893, a novel CD40 agonist monoclonal antibody. J Clin Oncol. 2007;25(7):876–83. doi:10.1200/JCO.2006.08.3311.
- Fransen MF, Sluijter M, Morreau H, Arens R, Melief CJ. Local activation of CD8 T cells and systemic tumor eradication without toxicity via slow release and local delivery of agonistic CD40 antibody. Clin Cancer Res. 2011;17(8):2270–80. doi:10.1158/1078-0432.CCR-10-2888.
- Kedl RM, Jordan M, Potter T, Kappler J, Marrack P, Dow S. CD40 stimulation accelerates deletion of tumor-specific CD8(+) T cells in the absence of tumor-antigen vaccination. Proc Natl Acad Sci U S A. 2001;98(19):10811–16. doi:10.1073/pnas.191371898.
- Ishihara J, Ishihara A, Potin L, Hosseinchi P, Fukunaga K, Damo M, Gajewski TF, Swartz MA, Hubbell JA. Improving efficacy and safety of agonistic anti-CD40 antibody through extracellular matrix affinity. Mol Cancer Ther. 2018;17(11):2399–411. doi:10.1158/1535-7163.MCT-18-0091.
- Lu L, Liu N, Fan K, Zhang G, Li C, Yan Y, Liu T, Fu WH. A tetravalent single chain diabody (CD40/HER2) efficiently inhibits tumor proliferation through recruitment of T cells and anti-HER2 functions. Mol Immunol. 2019;109:149–56. doi:10.1016/j.molimm.2019.03.001.
- Fellermeier-Kopf S, Gieseke F, Sahin U, Muller D, Pfizenmaier K, Kontermann RE. Duokines: a novel class of dual-acting co-stimulatory molecules acting in cis or trans. Oncoimmunology. 2018;7(9):e1471442. doi:10.1080/2162402X.2018.1471442.
- Dahlen E, Veitonmaki N, Norlen P. Bispecific antibodies in cancer immunotherapy. Ther Adv Vaccines Immunother. 2018;6(1):3–17. doi:10.1177/2515135518763280.
- Qu X, Felder MA, Perez Horta Z, Sondel PM, Rakhmilevich AL. Antitumor effects of anti-CD40/CpG immunotherapy combined with gemcitabine or 5-fluorouracil chemotherapy in the B16 melanoma model. Int Immunopharmacol. 2013;17(4):1141–47. doi:10.1016/j.intimp.2013.10.019.
- Zhang JQ, Zeng S, Vitiello GA, Seifert AM, Medina BD, Beckman MJ, Loo JK, Santamaria-Barria J, Maltbaek JH, Param NJ, et al. Macrophages and CD8(+) T cells mediate the antitumor efficacy of combined CD40 ligation and imatinib therapy in gastrointestinal stromal tumors. Cancer Immunol Res. 2018;6(4):434–47. doi:10.1158/2326-6066.CIR-17-0345.
- Rech AJ, Dada H, Kotzin JJ, Henao-Mejia J, Minn AJ, Twyman-Saint Victor C, Vonderheide RH. Radiotherapy and CD40 activation separately augment immunity to checkpoint blockade in cancer. Cancer Res. 2018;78(15):4282–91. doi:10.1158/0008-5472.CAN-17-3821.
- Yasmin-Karim S, Bruck PT, Moreau M, Kunjachan S, Chen GZ, Kumar R, Grabow S, Dougan SK, Ngwa W. Radiation and local anti-CD40 generate an effective in situ vaccine in preclinical models of pancreatic cancer. Front Immunol. 2018;9:2030. doi:10.3389/fimmu.2018.02030.
- Ward-Kavanagh LK, Kokolus KM, Cooper TK, Lukacher AE, Schell TD. Combined sublethal irradiation and agonist anti-CD40 enhance donor T cell accumulation and control of autochthonous murine pancreatic tumors. Cancer Immunol Immunother. 2018;67(4):639–52. doi:10.1007/s00262-018-2115-2.
- Ma HS, Poudel B, Torres ER, Sidhom JW, Robinson TM, Christmas B, Scott B, Cruz K, Woolman S, Wall VZ, et al. A CD40 agonist and PD-1 antagonist antibody reprogram the microenvironment of nonimmunogenic tumors to allow T-cell-mediated anticancer activity. Cancer Immunol Res. 2019;7:428–42. doi:10.1158/2326-6066.CIR-18-0061.
- Xiong H, Mittman S, Rodriguez R, Moskalenko M, Pacheco-Sanchez P, Yang Y, Nickles D, Cubas R. Anti-PD-L1 treatment results in functional remodeling of the macrophage compartment. Cancer Res. 2019;79:1493–506. doi:10.1158/0008-5472.CAN-18-3208.
- Luheshi NM, Coates-Ulrichsen J, Harper J, Mullins S, Sulikowski MG, Martin P, Brown L, Lewis A, Davies G, Morrow M, et al. Transformation of the tumour microenvironment by a CD40 agonist antibody correlates with improved responses to PD-L1 blockade in a mouse orthotopic pancreatic tumour model. Oncotarget. 2016;7(14):18508–20. doi:10.18632/oncotarget.7610.
- Ngiow SF, Young A, Blake SJ, Hill GR, Yagita H, Teng MW, Korman AJ, Smyth MJ. Agonistic CD40 mAb-driven IL12 reverses resistance to anti-PD1 in a T-cell-rich tumor. Cancer Res. 2016;76(21):6266–77. doi:10.1158/0008-5472.CAN-16-2141.
- Sorensen MR, Holst PJ, Steffensen MA, Christensen JP, Thomsen AR. Adenoviral vaccination combined with CD40 stimulation and CTLA-4 blockage can lead to complete tumor regression in a murine melanoma model. Vaccine. 2010;28(41):6757–64. doi:10.1016/j.vaccine.2010.07.066.
- Chonan M, Saito R, Shoji T, Shibahara I, Kanamori M, Sonoda Y, Watanabe M, Kikuchi T, Ishii N, Tominaga T. CD40/CD40L expression correlates with the survival of patients with glioblastomas and an augmentation in CD40 signaling enhances the efficacy of vaccinations against glioma models. Neuro Oncol. 2015;17(11):1453–62. doi:10.1093/neuonc/nov090.
- Liu C, Lewis CM, Lou Y, Xu C, Peng W, Yang Y, Gelbard AH, Lizee G, Zhou D, Overwijk WW, et al. Agonistic antibody to CD40 boosts the antitumor activity of adoptively transferred T cells in vivo. J Immunother. 2012;35(3):276–82. doi:10.1097/CJI.0b013e31824e7f43.
- Stromnes IM, Burrack AL, Hulbert A, Bonson P, Black C, Brockenbrough JS, Raynor JF, Spartz EJ, Pierce RH, Greenberg PD, et al. Differential effects of depleting versus programming tumor-associated macrophages on engineered T cells in pancreatic ductal adenocarcinoma. Cancer Immunol Res. 2019;7:977–89. doi:10.1158/2326-6066.CIR-18-0448.