ABSTRACT
Ricin toxin, a plant-derived, mannosylated glycoprotein, elicits an incapacitating and potentially lethal inflammatory response in the airways following inhalation. Uptake of ricin by alveolar macrophages (AM) and other pulmonary cell types occurs via two parallel pathways: one mediated by ricin’s B subunit (RTB), a galactose-specific lectin, and one mediated by the mannose receptor (MR;CD206). Ricin’s A subunit (RTA) is a ribosome-inactivating protein that triggers apoptosis in mammalian cells. It was recently reported that a single monoclonal antibody (MAb), PB10, directed against an immunodominant epitope on RTA and administered intravenously, was able to rescue Rhesus macaques from lethal aerosol dose of ricin. In this study, we now demonstrate in mice that the effectiveness PB10 is significantly improved when combined with a second MAb, SylH3, against RTB. Mice treated with PB10 alone survived lethal-dose intranasal ricin challenge, but experienced significant weight loss, moderate pulmonary inflammation (e.g., elevated IL-1 and IL-6 levels, PMN influx), and apoptosis of lung macrophages. In contrast, mice treated with the PB10/SylH3 cocktail were essentially impervious to pulmonary ricin toxin exposure, as evidenced by no weight loss, no change in local IL-1 and IL-6 levels, retention of lung macrophages, and a significant dampening of PMN recruitment into the bronchoalveolar lavage (BAL) fluids. The PB10/SylH3 cocktail only marginally reduced ricin binding to target cells in the BAL, suggesting that the antibody mixture neutralizes ricin by interfering with one or more steps in the RTB- and MR-dependent uptake pathways.
Keywords:
Introduction
Ricin toxin is a biological threat agent of concern to civilian and military personnel alike.Citation1 Ricin is readily isolated in large quantities from castor beans (Ricinus communis), which are cultivated worldwide for ornamental and industrial purposes. Ricin itself is a ~ 65 kDa glycoprotein consisting of two subunits, RTA and RTB, joined via a disulfide bond. RTB, a lectin specific for galactose and N-acetyl galactosamine, promotes ricin attachment to membrane-bound glycoproteins and glycolipids on mammalian cells. RTB is also involved in retrograde trafficking of ricin to the trans Golgi network (TGN) and endoplasmic reticulum (ER). In the ER, RTA is liberated from RTB and translocated into the cytoplasm via the Sec61 complex.Citation2 RTA is a ribosome-inactivating protein (RIP) that catalyzes the hydrolysis of a conserved residue within the sarcin/ricin loop of 28S rRNA.Citation3-Citation5 Ricin, which is mannosylated at three different positions, is also very efficiently taken up by cells that express the mannose receptor (MR; CD206), such as Kupffer cells (KCs) and alveolar macrophages (AM).Citation6 Ultimately, ricin-intoxicated cells undergo programmed cell death.
In rodents and nonhuman primates (NHPs), inhalation of ricin evokes the equivalent of acute respiratory distress syndrome (ARDS).Citation7,Citation8 Hallmarks of ricin exposure include neutrophilic infiltration, intra-alveolar edema, accumulation of pro-inflammatory cytokines like IL-1 and IL-6 in bronchoalveolar lavage (BAL) fluids, and fibrinous exudate. AM are notoriously sensitive to the effects of ricin, possibly due to their expression of the MR; they rapidly undergo apoptosis following ricin uptake and their numbers plummet in just a few hours.Citation9-Citation11 AMs are also likely a major source of the pro-inflammatory cytokines and chemokines observed in BAL fluids after toxin insult. The lethal dose 50 (LD50) of ricin by aerosol is ~4 μg/kg in mice and ~6 μg/kg in NHPs, with the mean time to death in both species being 36–48 h following ≥3 x LD50 toxin exposure.Citation12-Citation14
In the absence of FDA-approved measures to prevent or counteract the effects of ricin, the current guidance for post-exposure therapy is strictly supportive care. Efforts to produce small molecule inhibitors of ricin toxin have shown promise in vitro, but have not been successful when transferred to animal models because of issues related to limited bioavailability and/or insufficient potency.Citation15 At the same time, much effort has been invested in the development of a ricin toxin vaccine, especially for use by military personnel.Citation16,Citation17 The two recombinant RTA-based subunit vaccines that have been evaluated in Phase I clinical trials, RiVax and RVEc, were well tolerated and immunogenic, although the onset of toxin-neutralizing titers was highly variable and were often slow to appear, depending on the vaccine and dose regimen.Citation18-Citation20
For those reasons, there is a strong case to be made for investigating passive immunization strategies as a means to impart immediate protection against ricin toxin.Citation21,Citation22 Indeed, there are numerous studies demonstrating the potential of polyclonal and monoclonal (MAb) antibodies to function in pre- and post-exposure settings to protect mice from ricin toxin given by injection, ingestion or inhalation.Citation23-Citation28 Of particular significance was a report that a humanized IgG monoclonal antibody (MAb), called PB10, when given intravenously, was able to rescue Rhesus macaques from lethal dose of ricin toxin administered by aerosol.Citation29 In a follow-up study, an engineered variant of PB10 IgG with an extended serum half-life (“huPB10-LS”) was evaluated in non-human primates as a preexposure prophylactic. It was found that a single intravenous infusion of PB10-LS IgG (25 mg/kg) was sufficient to protect Rhesus macaques four weeks later from a ~ 3 x LD50 ricin toxin challenge (C. Roy, et al., manuscript submitted). However, preexisting PB10, even at relatively high doses in the BAL fluids, was not sufficient to fully suppress toxin-induced inflammation and pulmonary damage associated with ricin exposure. Even in mouse models, high dose PB10 treatment does not necessarily fully negate morbidity (e.g., weight loss, ruffled fur) associated with toxin insult, even though the animals invariably survive intoxication.Citation13,Citation14
We reasoned that combining PB10 with a second MAb might improve overall in vivo toxin-neutralizing activity, especially in light of other reports in the literature demonstrating additive and often synergistic benefits associated with MAb cocktails in vitro and in vivo.Citation22,Citation26,Citation30-Citation33 PB10 is directed against an immunodominant epitope near RTA’s active siteCitation34 and evidence indicates that PB10 neutralizes ricin by interfering with trafficking of the toxin from the plasma membrane to the TGN.Citation35 Therefore, coupling PB10 with a second MAb that affects ricin attachment to target cells might afford the greatest combined benefit. In this regard, SylH3 was the candidate of choice.
Among the dozen or more anti-RTB MAbs that have been described to date, we reported recently that only SylH3 is able to neutralize ricin in the context of the lung.Citation27 For example, eight out of 10 mice administered SylH3 (400 μg) by intraperitoneal injection survived a subsequent 10 x LD50 IN ricin. All other anti-RTB MAbs afforded far less protection (e.g., 2 out of 10 mice), as compared to SylH3. SylH3 was also the most effective MAb at blocking ricin attachment to galactose receptors in a solid phase binding assay.Citation27 Thus, in this report, we sought to examine the capacities of PB10 and SylH3, individually and in combination, administered intranasally to mice to protect the lungs and local macrophages from the effects of ricin toxin exposure.
Materials and methods
Chemicals and biological reagents
Ricin toxin (Ricinus communis agglutinin II) was purchased from Vector Laboratories (Burlingame, CA). Ricin was dialyzed against phosphate-buffered saline (PBS) at 4°C in 10,000 MW cutoff Slide-A-Lyzer dialysis cassettes (Pierce, Rockford, IL) prior to use. The relative potency of each lot of ricin toxin is determined in mouse LD50 studies upon receipt. Thereafter, potency is determined in Vero cell cytotoxicity assays. As a rule, a single lot of ricin toxin is used per study. As needed, lots of ricin toxin are validated by SDS-PAGE and probed with a panel of MAbs against known neutralizing and non-neutralizing epitopes.
The following anti-mouse primary antibodies were used for flow cytometry: F4/80 FITC, CD45 PE, CD11b PerCP-Cy5.5, Ly6G APC, CD19 APC-Fire (Cy7) (BioLegend, CA). Alexa Fluor 647 (F4/80+) (BD Biosciences, NJ). Murine MAbs against RTA (PB10) and RTB (SylH3) were purified by the Wadsworth Center’s Protein Expression core facility using ion-exchange and protein G chromatography as described previously.Citation27,Citation34 Unless noted otherwise, all other chemicals were obtained from Sigma-Aldrich (St. Louis, MO).
Mouse studies
Mouse studies were conducted under strict compliance with the Wadsworth Center’s Institutional Animal Care and Use Committee (IACUC). Female BALB/c mice (ages 8–10 weeks) were purchased from Taconic Biosciences (Rensselaer, NY). For acute exposures, PB10 (40 μg; 2 mg/kg), SylH3 (40 μg), or the combination (20 µg PB10 + 20 µg SylH3) were mixed with ricin (10xLD50; ~2 µg per mouse) in PBS and then administered in a final volume of 40 µl to mice by the intranasal (i.n.) route. This was designed time zero (t = 0). After 24 h, blood was collected via submandibular venipuncture method. The mice were then euthanized by carbon dioxide asphyxiation and the lungs were lavaged with 1 ml of ice-cold PBS. Bronchoalveolar lavage (BAL) fluids plus cells were centrifuged at 3000 rpm at 4ºC for 10 min, after which the supernatants are transfered to a fresh tubed and then followed by a second centrifugation at 13,200 rpm for 10 min. Supernatants were collected and stored at −20ºC until analysis. Cells were resuspended in 200 µl HBSS for flow cytometry analysis immediately.
For survival experiments, mice were challenged with ricin and MAb mixtures as described above and then were monitored daily for 7 days for symptoms of ricin intoxication and weight loss. To assess the therapeutic potential of the MAbs, mice were challenged with ricin (2 µg/mouse) by the intranasal route and then treated with 40 µg of PB10 alone or in combination with SylH3 (20 µg PB10 +20µg SylH3) by the intranasal route at the indicated time points. Mice were monitored for 14 days following toxin challenge. To examine the capacity of the individual MAbs and the antibody cocktail to passively protect mice against systemic ricin toxin challenge, mice received a mixture of ricin (2 µg; 10 x LD50) and MAbs (0.5 µg, 1.5 µg or 5 µg) or antibody cocktail (1:1 ratio of PB10: SylH3) by intraperitoneal injection. Mice were monitored for 3 days following toxin challenge.
During the course of a study, mice were weighed once daily and visually inspected twice daily for signs of morbidity. Visual inspections were done using a grading sheet approved by the IACUC. We recorded and graded signs of hunching, mild to moderate weakness, ataxia, distended abdomen, diarrhea, solitary nesting, ruffled fur, severe weakness, tremors, circling, head tilt, seizures, and swollen eyes. Mice were euthanized when their clinical score or weight loss exceeded a predetermined threshold.
Flow cytometric analysis of cell received from BAL
After lavage, the population of cells from the BAL fluid was examined by flow cytometry. Cells from BAL were resuspended in 200 µl HBSS and seeded in a clear, U-bottom, 96-well plate (Krackeler Scientific). Controls included unstained cells and individually stained cells for compensation. Cells were pelleted by centrifugation (1500 rpm for 5 min) and red blood cells were lysed with 150 mM ammonium chloride buffer.Citation36 Cells were incubated on ice for 15 min in 100 µL blocking solution containing TruStain fcXTM (anti-mouse CD16/32) antibody (Biolegend, San Diego, CA, USA) and centrifuged at 1500 rpm for 5 min. Cells were incubated with 100 µL of conjugated primary antibodies diluted in sort buffer (20 mM HEPES, 1mM EDTA, 2%FBS) and incubated on ice for 45 min. Primary antibodies were removed by centrifuging at 1500 rpm for 5 min, and the cells were washed twice in 200 µL of sort buffer. Finally, the plate was spun at 1500 rpm for 5 min, and the cells were resuspended in 400 uL of sort buffer in 5 mL Falcon tubes (Krackeler). Cells were analyzed immediately with a BD FACS Aria II flow cytometer utilizing BD FACSDiva software (BD Biosciences, Franklin Lakes, NJ, USA).
Cytokine quantification by cytometric bead array (CBA)
IL-6 and IL-1 levels in BAL fluids were determined using the BD CBA mouse flex sets (Becton Dickinson, Franklin Lakes, NJ). Dilution series of mouse IL-6 and IL-1 standards, included in the kit and prepared according to the manufacturer’s instructions, were included in each run to enable quantification. Assays were performed according to the manufacturer’s instructions: 50 μl of assay beads, 50 μl of the studied sample or standard, and 50 μl of PE-labeled antibodies (Detection Reagent) were added consecutively to each sample tube and then incubated at room temperature in the dark for 2 h. Next, the samples were washed and centrifuged. After that, the pellet was resuspended in Wash Buffer and analyzed on the same day in a flow cytometer. Flow cytometry was performed using a BD FACSCalibur™ system utilizing BD CellQuest™ software.
Histopathology and IHC
The lungs were instilled intratracheally with 10% buffered formalin prior to removal from the thoracic cavity then immersed in formalin for 24 h. Tissues were subsequently transferred to 70% ethanol prior to processing and embedding. Tissue sections were stained with hematoxylin and eosin (H&E) for histopathological evaluation. Tissue sections (3–4 μm thick) were deparaffinized in CitriSolve (Decon Labs., King of Prussia, PA) and rehydrated by processing them through graded alcohols. Antigen retrieval for cleaved caspase-3 was performed by heating the slides to 95°C in Rodent Decloaker (Biocare Medical; Pacheco, CA) for 40 min using a rice steamer. Endogenous IgG and nonspecific background were blocked with Rodent Block M (Biocare Medical), followed by an alkaline phosphatase (AP) block (BLOXALL; Vector Laboratories, Burlingame, CA). The primary antibody (GTX22302; Gene Tex, Irvine, CA) was incubated on the tissue sections (1:100 dilution) for 1 h at room temperature. Subsequently, sections were sequentially incubated with a rabbit-on-rodent tissue AP-based polymer (Biocare Medical) and Fast Red (Warp Red; Biocare Medical). Sections were counterstained with Tacha’s hematoxylin (Biocare Medical) and mounted with EcoMount (Biocare Medical).
IHC-labeling of neutrophils and macrophages was achieved using primary antibodies against Ly6G (ab25377; Abcam, Cambridge, MA) and F4/80 (GTX26640; Gene Tex) at a 1:300 and 1:100 dilution, respectively. Prior to incubation with the primary antibodies, tissue sections were pretreated with Proteinase K (0.1 mg/mL) for 5 min and blocked, as described.Citation37 Detection was achieved using a rat-on-rodent tissue HRP-based polymer (Biocare Medical) with subsequent application of Vina Green chromogen (Biocare Medical). Sections were counterstained with Nuclear Fast Red (Vector Laboratories) and mounted using EcoMount.
Cell cytotoxicity assay
The Vero and MH-S cell lines were purchased from the American Type Culture Collection (Manassas, VA). Cell culture media were prepared by the Wadsworth Center Media Services facility. Cells were maintained in a humidified incubator at 37°C with 5% CO 2. To measure the ricin cytotoxicity in MH-S cells, cells were trypsinized, adjusted to ~5 × 104 cells per ml, and seeded (100 μl/well) into white 96-well plates (Corning Life Sciences, Corning, NY), and incubated for 24 hr. Cells were then treated with a twofold serial diluted ricin (start at 1µg/ml) or medium alone for 2 h. The cells were washed to remove non-internalized toxin and then incubated for an additional ~48 h. Cell viability was assessed using CellTiter-GLO reagent (Promega, Madison, WI). All treatments were performed in triplicate, and 100% viability was defined as the average value obtained from wells in which cells were treated with medium only. To measure the neutralizing activity of ricin-specific MAbs, Vero or MH-S cells were seeded at ~5 × 104 cells per ml in 96-well plates and treated with ricin (10 ng/ml), ricin + MAbs mixture or medium alone (negative control) for 2 h. After washing and then incubating for ~48 h, cell viability was assessed using CellTiter-GLO reagent. Cell viability was normalized to cells treated with medium only.
Ricin attachment to BAL cells
To assess the ability of MAbs to inhibit ricin attachment to lung alveolar macrophages, freshly isolated BAL from BALB/c mice were resuspended in 200 µl HBSS and seeded in a U-bottom, 96-well plates. After lysis of red blood cells with ammonium chloride, the remaining cells were stained with ricin-FITC (2 μg/ml) or ricin-FITC plus MAbs (30 µg/ml total) for 30 min on ice in the dark. The cell mixture was also treated with Alexa Fluor 647-labeled anti-F4/80+ (0.2 µg/ml) to mark alveolar macrophages. The cells were then washed with HBSS and subjected flow cytometry using FACS Calibur (BD Biosciences). A minimum of 5,000 cells were analyzed per sample.
Statistical analyses
One-way ANOVAs were performed to compare cytokine levels and the percentage of CD45+ cells between treatment groups. Two-way repeated measures ANOVAs with the Geisser-Greenhouse correction and Sidak’s multiple comparisons post hoc test were used to compare the effects of antibody intervention on weight loss between groups, while Dunnett’s multiple comparisons post hoc test was used to determine if the change in weight was significant compared to the starting weights. We considered p-values of ≤0.05 (two-tailed tests) to be statistically significant. Statistical analyses were performed using GraphPad Prism 7 (GraphPad Software, San Diego, CA).
Results
Intranasal (IN) administration of PB10-SylH3 MAb cocktail promotes survival following IN ricin challenge
We assessed the capacities of PB10 and SylH3, individually and in combination, to passively protect mice against lethal dose ricin challenge by the IN route. Groups of adult BALB/c mice were exposed intranasally to 10xLD50 ricin without or with MAb co-administration, as described in the Materials and Methods. Over the next 7 days, the mice were weighed daily and monitored for morbidity that included signs of lethargy, ruffled fur, hunching, ataxia, and reduced movement. The experiments were terminated on day 7. We chose a working dose of 40 µg MAb total per mouse, which is equivalent to ~2 mg/kg of body weight, since previous studies had indicated that this was the minimum required to confer passive protection against intranasal ricin challenge.Citation13,Citation14 In contrast, SylH3 was previously shown to be protective in the intranasal challenge model, but only at very high doses (400 µg; 20 mg/kg).Citation27
Control mice that received ricin toxin (without the addition of MAb) experienced rapid weight loss and died within 4 days (). Mice that received ricin plus SylH3 (40 µg; 2 mg/kg) also experienced a significant weight loss and the majority of animals died before the termination of the experiment (). Mice that received ricin plus PB10 (40 µg; 2 mg/kg) all survived to day 7 (), but experienced significant weight loss () and displayed overt signs of morbidity, including lethargy, ruffled fur, hunching, ataxia, and reduced movement (data not shown). In contrast, mice that received ricin plus the combination of PB10 and SylH3 (20 µg + 20 µg; 2 mg/kg) survived without any significant weight loss () or overt signs of morbidity (data not shown), suggesting that the MAb cocktail affords significant benefit over either PB10 or SylH3 alone.
Figure 1. Protection afforded by PB10, SylH3 and the PB10/SylH3 cocktail against intranasal ricin exposure in a mouse model. Groups of adult BALB/c mice were challenged IN with ricin (2 µg/mouse; equivalent to 10xLD50) or ricin plus anti-ricin MAbs (40 µg; 2 mg/kg). (a) Kaplan-Meier plot of mouse survival over period of 7 days for following challenge. Overlapping lines in the graph were nudged slightly to differentiate symbols that would otherwise be superimposed on each other. (b) Change in body weights over a 7 day period following ricin exposure. Linear regression analysis was used to examine the relationship between weight and treatment at times post-ricin challenge. Slopes were compared via ANOVA with Tukey’s multiple comparisons test. Different letters indicate statistically significant differences determined by the test. The data are from two independent experiments with three mice per group.
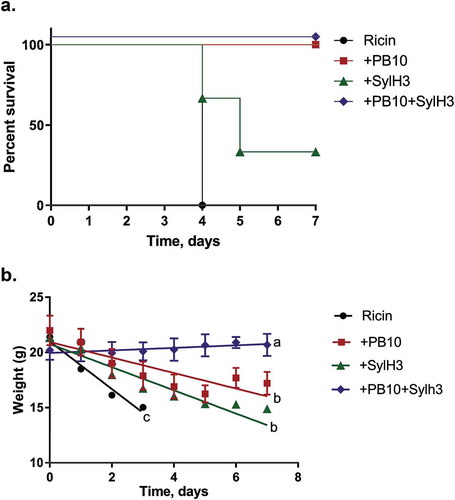
We next compared the benefit of PB10 to the PB10 + SylH3 cocktail in a larger cohort of mice. As shown in , while PB10 and the MAb cocktail were each sufficient to protect mice against 10xLD50 ricin, the PB10-treated mice experienced significant weight loss over the 7-day experiment, whereas the MAb cocktail-treated mice actually gained weight. Thus, the MAb cocktail clearly was superior to either PB10 or SylH3 alone in neutralizing the effects of ricin when delivered via the IN route.
Table 1. PB10/SylH3 MAb cocktail prevents weight loss following ricin toxin challenge.
To determine whether the MAb cocktail also affords a benefit over PB10 as a therapeutic, mice were challenged IN with ricin then followed by the PB10 or PB10/SylH3 cocktail treatment by the same route (IN) at 2 h intervals (2, 4, 6, 8 h). Animals that received PB10 or the PB10/SylH3 cocktail at the 2, 4 or 6 h time points survived ricin challenge (). However, mice that received the MAb cocktail lost significantly less weight than did their counterparts who only received PB10 (; Table S1). The benefit of the cocktail was also apparent when administered 8 h post-ricin exposure: 33% of the animals survived with PB10 treatment, while 83% survived with cocktail (data not shown). Thus, the MAb cocktail is more effective than PB10 at rescuing mice from IN ricin exposure.
Figure 2. Therapeutic potential of the PB10/SylH3 cocktail in a mouse model of intranasal ricin challenge. BALB/c mice (n = 3-6/group) were challenged IN with ricin (2 µg/mouse) and then given PB10 (40 µg; 2 mg/kg) or PB10/SylH3 (20 µg + 20 µg; 2 mg/kg) cocktail by the IN route at the indicated time points (i.e., +2, +4, +6 h) post ricin challenge. Animals were weighed daily for 11 days thereafter. Each datum point represents the mean percent weight change (with standard errors) for each group. A two-way repeated measures ANOVA was performed to examine the influence of antibody intervention and time post-challenge on weight loss, as detailed in the Materials and Methods. Asterisks indicate statistically significant differences between percent weight lost in the PB10-treated group of mice versus the PB10/SylH3 cocktail-treated groups of mice. See Table S1 for examination of the significant differences in weight loss for each group, as compared to pre-challenge.
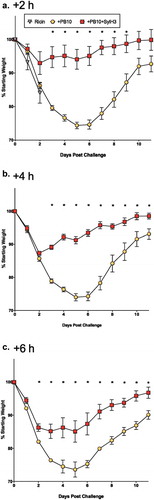
We next assessed the benefit of the PB10/SylH3 cocktail in a systemic model of ricin challenge. Specifically, ricin (2 µg; 10 x LD50) was mixed with serial dilutions of PB10, SylH3, or the PB10/SylH3 cocktail and then administered to groups of mice by intraperitoneal (IP) injection (Figure S1). Mice were monitored for 3 days following toxin challenge. SylH3 at these relatively low doses (0.5–5 µg; 0.25–1.25 mg/kg) afforded no appreciable protection against a 10 x LD50 ricin challenge, which agrees with published reports.Citation27,Citation38,Citation39 Mice that received 5 µg of PB10 (1.25 mg/kg) survived the systemic ricin challenge, whereas mice that received 1.5 (1.25 mg/kg) or 0.5 µg (0.25 mg/kg) PB10 did not (Figure S1; data not shown). Unexpectedly, mice that received ricin and the PB10/SylH3 cocktail fared no better than mice that received ricin and PB10 alone. For example, mice that received 1.5 µg (1.25 mg/kg) of the PB10 or PB10/SylH3 cocktail each died within 48 h. Similar results were obtained when the ricin-MAb mixtures were administered by the intravenous route (B. Mooney and N. Mantis, unpublished results). Moreover, the PB10/SylH3 cocktail did not demonstrate an improved IC50 in Vero cell assay as compared to either PB10 or SylH3 MAb alone (Figure S2A).
We also obtained and tested MH-S cells, a continuous mouse alveolar macrophage-like cell line first described in 1989.Citation40 Although the MH-S cells proved to be extremely sensitive to the effects of ricin (Figure S2B), the benefit of the MAb cocktail was not apparent, as compared to PB10 (Figure S2C). In other words, PB10 and the PB10 + SylH3 cocktail were equally effective at protecting the cells from ricin intoxication. It is unclear if the failure to recapitulate the effect of the MAb cocktail is a function of the MH-S cells themselves, which are known to “ … lack defining features of primary alveolar macrophages … ”,Citation41 or a factor that is specific to the lung environment and not recapitulated in cell culture. Nonetheless, these results demonstrate that the benefits of the PB10/SylH3 cocktail are specific to pulmonary ricin exposure.
The PB10/SylH3 MAb cocktail reduces ricin-induced lung pathology and apoptosis
To better define how the PB10/SylH3 cocktail fortifies the lung against ricin-induced damage, we performed histopathologic analysis of lung tissues from experimental animals. Groups of adult mice were challenged IN with saline, ricin, or ricin plus PB10, SylH3, or the PB10/SylH3 cocktail. The animals were euthanized 24 h later and lung tissue collected for H&E staining, alongside immunohistochemistry (IHC). Mice that received saline served as the baseline (). In animals that received ricin, pulmonary damage ranged from moderate to severe and consisted of multifocal-to-coalescing interstitial suppurative pneumonia with edema and necrosis (), consistent with other reports conducted with mice and NHPs.Citation42-Citation44 Of note was the number of neutrophils lining the endothelial surface of large and medium-size vessels, which is indicative of margination, and in the tunica media, which is a hallmark of emigration. Neutrophils were also infiltrating the perivascular space of large and medium-size blood vessels. Occasionally, we observed focal areas of endothelial damage and fibrinoid necrosis consistent with vasculitis. Perivascular neutrophilic infiltration was most prominent in (but not restricted to) the hilar region and characterized by numerous apoptotic bodies and karyorrhectic debris. Airways were spared from inflammation and tissue damage. Staining for activated (cleaved) caspase-3 revealed numerous inflammatory cells (~10 cells/high power field – HPF) undergoing apoptosis while migrating through the vessel walls, as well as in the perivascular and interstitial infiltrate (compare to ).
Figure 3. Pathological evaluation of lung tissues following intranasal exposure to ricin. Histopathology (h&e; left two columns) and cleaved caspase-3 IHC (right column) in the lungs of animals inoculated with (a–c) saline, (d–f) ricin, (g–i) ricin + PB10, or (j–l) ricin plus the PB10/SylH3 cocktail. In panels a-c, alveoli are fully expanded, often with alveolar macrophages in the alveolar space (b, arrows), and no evidence of caspase-3 activity. Following ricin treatment (panels d–f); lungs were congested, edematous, and with evidence of various degrees of hemorrhage (d, bracket). Note damage of endothelial cells in blood vessels (e, box), with concomitant neutrophilic infiltration of the vessel wall and perivascular spaces. Prominent cleaved caspase-3 staining was evident in the alveoli, while inflammatory cells infiltrating the perivascular space and blood vessel wall were present (f, box). In mice that received PB10 and ricin (g–i) there was focally extensive alveolar obliteration and interstitial inflammation in the perihilar region (g, box). Neutrophils infiltrating the blood vessel wall, perivascular space, and alveoli walls (h). Caspase 3 activity was limited and restricted to the perivascular space infiltrate and alveoli walls (i). In mice that received PB10/SylH3 and ricin (panels j–l) there was perihilar congestion (j) and infiltration of the perivascular space and alveoli walls by macrophages and rare neutrophils (k). Cleaved caspase 3 was rarely detected in these tissues (L). Legend: a, alveoli; b, bronchi/bronchioles airways; v, lumen of blood vessels; p, perivascular space; w, blood vessel wall. Cells positive for cleaved caspase 3 (c, f, i, and l) appear crimson. Magnification of images a, d, g, and j is 5x (bar, 100 μm). Magnification of other images is 50x (bar, 10 μm).
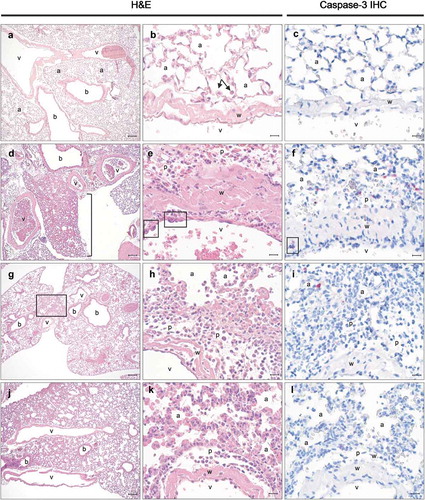
The histopathological changes observed in animals that received ricin in conjunction with either PB10 () or SylH3 (data not shown) were similar to those in animals challenged with ricin alone, albeit with a significant reduction in severity. Moreover, damage was almost entirely restricted to the hilar region. The neutrophilic infiltration extended from the perivascular space into the immediate interstitium, and in a few instances to the pleura. Focal necrosis in areas of inflammation was infrequent. IHC examination of cleaved caspase-3 revealed that the numbers of apoptotic cells in the lung were greatly reduced (<5 cells/HPF) when ricin was co-administered with either PB10 or SylH3, as compared to control animals.
In contrast, only minimal histopathological changes were evident in the lungs of mice that received ricin plus PB10/SylH3 cocktail (). While occasional blood vessels with scattered neutrophils marginating and migrating into the perivascular space were observed, inflammation of the immediate perivascular interstitium was rare and, when present, very minimal. Activated caspase-3 staining levels were essentially equivalent to saline-treated animals, indicating that the antibody cocktail prevented local toxin-induced cell death.
As additional follow-up, lung tissues from mice that survived IN ricin challenge due to co-administration of PB10 (data not shown) and PB10/SylH3 cocktail were collected on day 7 and subjected to histopathologic analysis (Figure S3). In tissues from mice that received ricin plus the PB10/SylH3 cocktail, analysis revealed evidence of focally extensive (perihilar) insult and ensuing inflammatory response and was confirmed by perihilar Type II hyperplasia (a sequela to Type I pneumocyte damage). Occasional signs of goblet cell metaplasia and lymphoid proliferation were also evident, suggesting that mice that received PB10/SylH3 cocktail did in fact experience some localized low-grade tissue damage due to ricin exposure.
The PB10/SylH3 MAb cocktail limits ricin-induced ablation of lung macrophages
Hallmarks of pulmonary ricin exposure include the ablation of lung macrophages and a concomitant influx of neutrophils into the BAL fluids and lung mucosa.Citation44,Citation45 To examine the effect of the PB10/SylH3 cocktail on the cellular composition of the lung, groups of animals were challenged IN with saline (vehicle control), ricin toxin alone or ricin plus PB10, SylH3 or the PB10/SylH3 cocktail. Twenty-four hours later, we collected BAL fluids and whole lung tissues and quantitated the number of macrophages (F4/80+) and neutrophils (Ly6G+) by flow cytometry () and IHC ().
Figure 4. Analysis of macrophage and neutrophils in BAL following intranasal ricin challenge. Mice were exposed IN to ricin (2 µg) or ricin plus PB10 (40 µg; 2 mg/kg), SylH3 (40 µg; 2 mg/kg), or PB10/SylH3 (20 µg + 20 µg; 2 mg/kg). Cells recovered from BAL fluids were collected at 24 h were analyzed by flow cytometry. Cells were pre-gated as single live CD45+ cells, and then subdivided into macrophages (CD11b−, F4/80+), neutrophils (CD11b+, Ly6G+), and B lymphocytes (CD19+). (a) Dot plots of macrophage and neutrophils in BAL from a representative mouse in each group. (b) Data expressed as mean (±SD) percentage of the various phenotypes in total CD45+ cells (n = 3/group). (c) The absolute number of cells were quantified for each phenotypic marker pair. In total, ~2x10Citation4 BAL cells were counted for each sample. One-way ANOVA followed by Tukey’s post-hoc comparisons tests were performed in the statistical analysis. Different letters indicate statistically significant differences determined by the test. Data shown are from one of the two independent experiments with three mice per group per experiment.
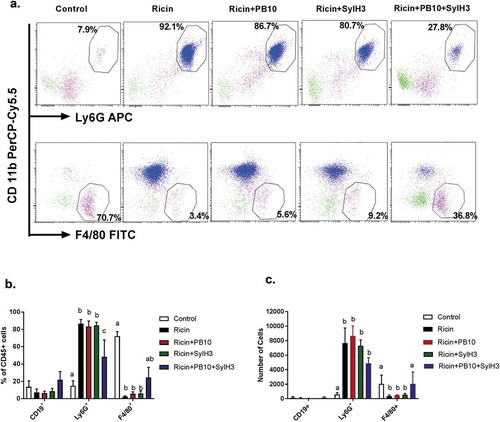
Figure 5. Immunohistochemical detection of macrophage and neutrophils following intranasal exposure to ricin. Mouse lung tissues were subject to IHC using antibodies to (a-c) F4/80 and (d-f) Ly6G to detect macrophages and PMNs, respectively. Tissues obtained from mice that had been exposed to (a, d) saline, (b, e) ricin, or (c, f) ricin plus the PB10/SylH3 (20 µg + 20 µg) cocktail. The perivascular space and alveoli in control animals (a, d) have scattered alveolar and interstitial macrophages (arrows) and a few neutrophils (arrowhead), all within normal limits. Following ricin exposure, there is marked macrophage depletion (b) and prominent neutrophilic margination and infiltration of the perivascular space and interstitium (e). Ricin plus PB10/SylH3 cocktail rescued macrophages (c) while having minimal impact on increasing the recruitment of neutrophils (f). (g, h) Quantitation of macrophage and neutrophil in tissue sections. The IHC positive cells were averages of ten 50x high power fields (HPF) in the perihilar region and 10 HPF distal to the perihilar region, at the bronchiole and respiratory bronchiole level. Cell counts were performed using an eyepiece micrometer. One-way ANOVA followed by Tukey’s post hoc comparisons tests were performed in the statistical analysis. Different letters indicate statistically significant differences determined by the test. Abbreviations: a, alveoli; v, lumen of blood vessel; p, perivascular space; w, blood vessel wall. Positive cells appear green (arrowheads). Magnification is 50x (bar = 10 µm).
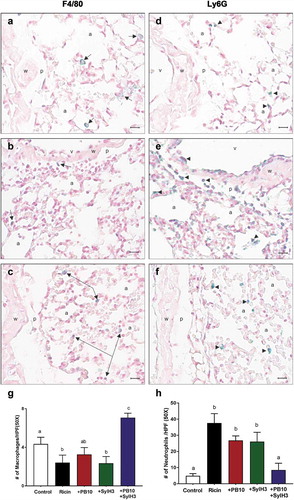
As expected, following ricin exposure there was a marked decline in F4/80+ cells and a corresponding increase in Ly6G+ cells in BAL, as compared to saline-treated controls (). As a percent of total leukocytes (CD45+), F4/80+ cells dropped from 80% to <5%, while PMNs increased from ~15% to more than 80%. A similar pattern was observed in tissue sections stained with anti-Ly6G and anti-F4/80 antibodies ( as compared to ). By IHC, there was a ~ twofold reduction in F4/80+ cells and nearly a 10-fold increase in Ly6G+ cells in tissue sections from ricin-treated mice, as compared to tissues from saline-treated animals.
Co-delivery of ricin with either PB10 or SylH3 individually did not prevent macrophage (F4/80+) depletion or a dampen the neutrophilic (Ly6G+) influx, as compared to ricin-only treated mice (). For example, in BAL fluids, the percent reduction in F4/80+ cells and concomitant percent increase in Ly6G+ cells were identical between ricin-treated and ricin plus PB10-treated groups of mice, even though the ricin-treated mice were destined to die as a result of toxin exposure, whereas the ricin plus PB10-treated mice would have survived. By contrast, co-administration of ricin with the PB10/SylH3 cocktail by the IN route resulted in a significant reduction of lung macrophage death (and even an increase in total macrophages compared to saline controls) and a fewer PMNs in BAL fluids and lung tissues (, ). These results demonstrate that the PB10/SylH3 cocktail shields lung macrophages from ricin-induced cell death. Moreover, the fact that PB10/SylH3 + ricin treatment resulted in slightly increased numbers of macrophages recovered from the BAL fluids argues that the antibody-toxin immune complexes may even be stimulatory and recruit leukocyte populations.Citation46,Citation47
To further characterize the impact of the PB10/SylH3 cocktail on ricin-induced inflammation, we examined IL-1 and IL-6 cytokine levels in serum and BAL fluids at three different time points (6, 16, and 24 h) following IN ricin challenge. IL-6 and IL-1 are associated with ricin-induced ARDS in miceCitation48 and non-human primates.Citation29 In confirmation of previous reports, we found that IL-6 levels increased significantly in the sera (~10 fold) and BAL fluids (~100 fold) of mice after IN ricin challenge (Figure S4). Mice that received PB10 or SylH3 intranasally with ricin toxin did not experience an uptick in serum IL-6 and had suppressed IL-6 levels in BAL fluids, as compared to ricin-treated control animals (). Mice that received PB10 by injection 6 h before ricin challenge also had significantly lower levels of IL-6 in BAL and serum, even though PB10 did not affect the loss of macrophages or influx of PMNs (Figure S5).
Figure 6. Cytokines in sera and BAL fluids of mice following ricin challenge. Groups of mice were challenged IN with ricin (2 µg) or ricin plus MAbs (40 µg). (a) Sera and (b,c) BAL fluids were collected ~24 h after and subjected to CBA to measure IL-6 and Il-1, as indicated. Concentrations are expressed as the mean ± SD (n = 3/group). One-way ANOVA followed by Tukey’s post hoc comparisons tests were performed in the statistical analysis. Different letters indicate statistically significant differences determined by the test. The results presented are from one of the two independent experiments.
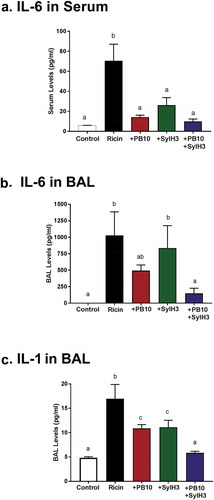
However, the PB10/SylH3 MAb cocktail was significantly more effective than either of the individual MAbs at suppressing IL-6 production in response to ricin. In fact, IL-6 levels in sera () and BAL fluids () were essentially at baseline in mice that received the PB10/SylH3 cocktail. A similar pattern was observed when IL-1 levels were examined (). These results demonstrate that the PB10/SylH3 cocktail suppresses the onset of ricin-induced pro-inflammatory cytokines, which are drivers of ricin-induced ARDS.
Effect of the PB10/SylH3 cocktail on ricin attachment to cells in the BAL
How does the PB10/SylH3 cocktail protect the lung from ricin toxin? We have reported that bispecific camelid antibodies that simultaneously target epitopes on RTA and RTB are effective at inhibiting ricin attachment to a monocyte cell line.Citation49 We postulated that the PB10/SylH3 cocktail might also interfere with ricin attachment, thereby accounting for the cocktail’s potent in vivo toxin-neutralizing activity. To address this question, freshly isolated cells from mouse BAL fluid were pulsed with FITC-labeled ricin (16 nM; 3 µg/ml) in the presence of 12-fold molar excess of SylH3 (30 µg/ml), PB10 (30 µg/ml), or the PB10/SylH3 cocktail (15 + 15 µg/ml). We then examined ricin-FITC attachment to the F4/80+ cell subset, specifically. Ricin-FITC bound to F4/80+ cells from the BAL with a geometric mean fluorescence (GMF) of ~2500 (). The addition of SylH3 reduced the amount of cell-associated ricin-FITC by ~40% (~1500 GMF; p < .05), while the addition of PB10 had no effect on ricin attachment (~2250 GMF). The PB10/SylH3 cocktail-reduced ricin-FITC attachment by only 15–20%, clearly indicating that the cocktail did not have increased inhibitory activity as compared to either of the MAbs alone. In fact, the cocktail was only half as effective as SylH3 alone at interfering with ricin binding to F4/80+ cells, which corresponds to there being only half the amount of SylH3 in the cocktail (15 µg/ml), as compared to the experiments with SylH3 alone (30 µg/ml). These results are consistent with the PB10/SylH3 cocktail interfering with ricin endocytosis or intracellular transport in lung macrophages, not blocking ricin attachment to cell surfaces.
Figure 7. Effect of PB10, SylH3 and PB10/SylH3 cocktail on ricin attachment to mouse lung macrophages. Cells from the BAL of naïve BALB/c mice were collected and stained with AlexaFluor 647 (F4/80+) coupled to FITC-labeled ricin (3 μg/ml), or pre-incubated mixture of FITC-ricin plus indicated MAbs (30 μg/ml) for 30 min on ice. The cells were washed and then subjected to flow cytometry. Cells were pre-gated as macrophages (F4/80+), and then were analyzed FITC as a measure of cell surface-bound ricin. Cells were stained with Alexa Fluor 647 in the absence of ricin-FITC as the control. (a) A representative histogram of ricin-fluorescence in F4/80+ cells. (b) Data were expressed as mean (±SD) of ricin-fluorescence in F4/80+ cell (n = 5/group). One-way ANOVA followed by Bonferroni’s post hoc comparisons tests were performed in the statistical analysis. Different letters indicate statistically significant differences determined by the test.
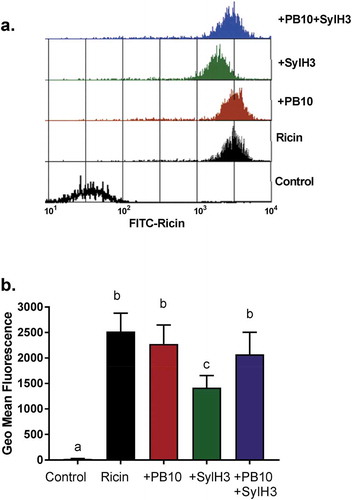
Discussion
It is well established that inhalation of even relatively small amounts of ricin toxin provokes widespread apoptosis of alveolar macrophages, intra-alveolar edema, neutrophilic infiltration, accumulation of pro-inflammatory cytokines, and fibrinous exudate.Citation7-Citation9,Citation25,Citation50-Citation52 Alveolar macrophages and IL-1 secretion, in particular, have been implicated in driving pro-inflammatory cytokine and chemokine production, microvascular permeability, and PMN influx.Citation10 Ricin also triggers cell death of airway epithelial cells, an activity that is enhanced in the presence of inflammatory cytokines like TNF-α and TRAIL.Citation53,Citation54
In this report, we demonstrated in mice that IN delivery of a bipartite MAb cocktail, one MAb against RTA (PB10) and one against RTB (SylH3), affords near complete protection against ricin toxin-induced pulmonary damage. Mice that received PB10/SylH3 concurrently with ricin toxin did not experience weight loss or have any significant lung inflammation, as measured by histopathology and pro-inflammatory cytokine production (IL-1, IL-6). The PB10/SylH3 mixture was also highly effective at shielding alveolar macrophages from ricin-induced killing, suggesting the antibody cocktail neutralizes ricin during the earliest steps in pulmonary toxicity.Citation10,Citation55-Citation58 The PB10/SylH3 cocktail also had activity as a post-exposure therapeutic. Overall, the results of this study demonstrate the utility of simultaneously targeting RTA and RTB as a strategy to more effectively counteract ricin’s effects on the lung.
Considering the extent of damage that ricin causes within the lung, it is somewhat remarkable that PB10, when given systemically to mice or Rhesus macaques before or even after ricin exposure, was able to rescue animals from lethality of intoxication. In macaques, PB10 intervention within 4 h resulted in a significant dampening of the pro-inflammatory response in the BAL, an observation that we now confirmed in mice. However, PB10’s capacity to annul the effects of ricin is finite, as evidenced by the fact that mice still experienced significant weight loss, a reduction in alveolar macrophages, and elevated levels of IL-1 and IL-6. By all accounts, combining PB10 with SylH3 resulted in essentially complete toxin neutralization. In fact, the benefit of PB10/SylH3 exceeded what was achieved through vaccination, where mice survived ricin aerosol exposure but lost ~25% body weight over the course of 3–4 days.Citation50
SylH3 is one of more than a dozen well-characterized anti-RTB MAbs reported in the literature.Citation26,Citation27,Citation38,Citation59-Citation61 Overall, its in vitro profile is similar to many other anti-RTB MAbs that have been described, with binding affinity (KD) for ricin of ~3 x 10−11 (M) and an IC50 value of 12 nM in a Vero cell-based cytotoxicity assay.Citation27 However, SylH3 stands out because when given by injection to mice it afforded partial protection from the effects of IN ricin challenge. Also, SylH3 remains the most effective MAb in our collection at blocking ricin-receptor interactions, as determined using solid-phase and cell-based attachment assays. For those reasons SylH3 was chosen as a pair with PB10 in the current study. Nonetheless, it remains to be determined whether SylH3 is unique in its capacity to synergize with PB10 or whether other anti-RTB MAbs function similarly. Two obvious candidates to consider are MH3 and 8A1. Although MH3 and 8A1 provided little or no protection to mice in the face of IN ricin challenge, the two MAbs have binding affinities (KD) and in vitro toxin-neutralizing activities equivalent to or greater than SylH3.Citation27 Other groups have reported anti-RTB MAbs with good in vitro and in vivo toxin-neutralizing profiles, including the ability to protect mice against IN ricin challenge.Citation26
We speculate that the PB10/SylH3 cocktail might neutralize ricin by different mechanisms within the context of the lung, depending on which cell type is being targeted. In the case of epithelial cells, ricin is internalized following attachment of RTB to terminal Gal/GalNAc residues on surface glycoproteins and glycolipids.Citation62 The toxin is then trafficked retrograde to the TGN and ER, where RTA is then liberated from RTB and translocated into the cytosol. At least in vitro, both PB10 and SylH3 by themselves have been shown to protect human airway epithelial cell lines from ricin intoxication, albeit by different mechanisms.Citation54 SylH3 blocks RTB attachment to galactosyl receptors on cell surfaces, while PB10 interferes with ricin trafficking from the plasma membrane to the trans-Golgi network.Citation35,Citation63 Thus, when combined, SylH3 and PB10 can theoretically affect both ricin uptake and intracellular trafficking.
The rules are different in the context of alveolar and other tissue macrophages where ricin is taken up by two different pathways: one involving RTB-mediated attachment to surface glycoproteins, as described above, and the other involving the mannose receptor (MR:CD205). Both RTA and RTB’s high mannose side chains serve as ligands for the MR. In bone marrow-derived macrophages (BDMCs), intoxication is 10–100 times more efficient via the MR than by RTB-mediated uptake and does not appear to involve retrograde transport to the ER.Citation6 Defining the pathway by which the MR mediates ricin uptake and delivery of RTA into the cytoplasm of macrophages and other cell types is a neglected area of research, but one that is experimentally tractable considering the advances in biochemical and microscopy-based tools that enable tracking of molecules at the single-cell level.Citation64,Citation65
But the question still remains: how does the PB10/SylH3 cocktail protect macrophages from ricin? We demonstrated in this study that the MAb mixture had no notable effect on toxin attachment to alveolar macrophages, indicating that the antibodies must interfere with either endocytosis or translocation of RTA into the cytoplasm. In a companion study, we present evidence in mouse Kupffer cells that PB10 and SylH3 interfere with a mannose-sensitive uptake pathway, rather than the lactose-sensitive pathway.Citation66 It is possible, therefore, that the MAbs prevent translocation of RTA from the endosome to the cytoplasm, which could be addressed using a combination of immunofluorescence microscopy and immunoprecipitation studies with different cell fractions (e.g., membrane, endosomal, cytoplasmic). Alternatively, decoration of ricin with one or more MAbs could promote FcR-mediated uptake and (presumably) degradation of ricin. Ultimately, deciphering how the PB10/SylH3 cocktail shields macrophages from intoxication awaits a better understanding of how ricin actually exploits the MR to deliver RTA into the cytosol. Toward this end, we have recently obtained the MR knockout mice first described by Lee and colleagues.Citation67 While we previously characterized the sensitivity of these mice to ricin exposure by injection,Citation68 we did not study them in the context of pulmonary toxicity or investigate the role the MR may play in sensitizing alveolar macrophages to the effects of ricin. These studies are now ongoing.
Another consideration is the possible impact of antibodies on the diffusion of ricin through airway mucus. In a recent study, for example, it was demonstrated that diffusion of Ebola pseudoviruses through human airway mucus is significantly retarded by the addition of ZMapp, a tripartite MAb cocktail.Citation69 The effect of ZMapp was attributed to the polyvalent interactions of IgG with mucins, rather than pseudovirus agglutination. In fact, even weakly neutralizing MAbs were able to replicate the effect of “muco-trapping”. It is possible, therefore, that the PB10/SylH3 cocktail affects spatial distribution and diffusion kinetics of ricin within the airway and thereby limits the amount of toxin that gains access to target cells in the first place. Virtually nothing is known about the distribution kinetics of ricin following inhalation, although recent results from the Rhesus macaque model indicate that a proportion of the toxin remains accessible to PB10 for at least 4 h.Citation29 It is therefore of great importance to define how and where antibodies given locally or systemically engage and inactivate their targets in the lung. We are particularly interested in the possible application of proximity ligation assays (PLA) as a means to localize toxin-antibody complexes in situ and then follow their fate over time.Citation70
There is a clear path forward in terms of pre-clinical evaluation of the PB10/SylH3 cocktail. As noted in the Introduction, PB10 has already been fully humanized and tested in a non-human primate model.Citation29 Very recently, humanized PB10 IgG was additionally engineered to include the so-called LS modification (M428L, N434S) within the Fc region to enhance serum half-life. Indeed, a single infusion of the huPB10-LS variant (25 mg/kg) was sufficient to protect Rhesus macaques from ricin aerosol challenge 28 days later (Roy et al., manuscript submitted). We have also fully humanized SylH3 and shown in preliminary studies that the huPB10/huSylH3 cocktail displays the same capacity to protect mice against ricin challenge as the mouse MAb cocktail (Y. Rong, M. Pauly, L. Zeitlin, and N. Mantis, unpublished results). There is reason to be optimistic that the beneficial effects of the PB10/SylH3 cocktail observed in mice will carry over to Rhesus macaques. For example, two humanized anti-pertussis toxin MAbs (hu1B7, hu11E6) that displayed synergy in a cell-based assay were shown to be significantly more effective than either of the individual MAbs alone when tested in a mouse and baboon model of whooping cough.Citation31 Similarly, a cocktail of humanized anti-TcdA and TcdB MAbs has shown marked degrees of protection in animal models of Clostridium difficile infection.Citation71,Citation72 The mouse and Rhesus macaque models of ricin intoxication are of the utmost importance, considering that the licensure of drug products for use in humans will fall under the Food and Drug Administration’s (FDA) Animal Rule since clinical trials with ricin toxin are unethical.
There are several limitations of this study that will need to be addressed in future investigations. Of particular importance is more fully evaluating the PB10/SylH3 cocktail’s therapeutic potential across a range of doses in the mouse model of intoxication. Our current study examined only a 1:1 ratio of the two MAbs and at a single dose of 2 mg/kg. We have not performed antibody titrations or explored different stoichiometries. Nor have we explored the effect of IgG subclass on biological activity, which in the case of anthrax toxin had a profound impact on MAb efficacy in vivo due to the contribution of Fc receptors in clearing toxin-Ab complexes.Citation73,Citation74 Finally, the actual mechanism(s) of action of PB10 and SylH3 remains poorly defined at the cellular and organismal level. Without that information, rational design of more effective interventions is not possible.
Author contributions
YR, FJTV, JD, and RS performed the experiments; YR, DE, FJTV and NJM analyzed results and prepared figures; YR, FJTV and NJM wrote the manuscript; project administration, NJM; funding acquisition, NJM.
Disclosure of potential conflicts of interest
No potential conflicts of interest were disclosed.
Acknowledgments
We are grateful to Helen Johnson in the Wadsworth Center’s mouse histopathology core and the immunology core for extensive assistance with flow cytometry. We gratefully acknowledge Beth Cavosie for administrative support. This work was supported by Contract No. HHSN272201400021C to NJM. The content is solely the responsibility of the authors and does not necessarily represent the official views of the National Institutes of Health. The funders had no role in study design, data collection, and analysis, decision to publish, or preparation of the manuscript.
References
- Cieslak TJ, Kortepeter MG, Wojtyk RJ, Jansen HJ, Reyes RA, Smith JO. Beyond the dirty dozen: a proposed methodology for assessing future bioweapon threats. Mil Med. 2018;183:e59–e65. doi:10.1093/milmed/usx004.
- Spooner RA, Lord JM. How ricin and shiga toxin reach the cytosol of target cells: retrotranslocation from the endoplasmic reticulum. Curr Top Microbiol Immunol. 2012;357:19–40. doi:10.1007/82_2011_154.
- Endo Y, Mitsui K, Motizuki M, Tsurugi K. The mechanism of action of ricin and related toxic lectins on eukaryotic ribosomes. The site and the characteristics of the modification in 28 S ribosomal RNA caused by the toxins. J Biol Chem. 1987;262:5908–12.
- Endo Y, Tsurugi K. RNA N-glycosidase activity of ricin A-chain. Mechanism of action of the toxic lectin ricin on eukaryotic ribosomes. J Biol Chem. 1987;262:8128–30.
- Tesh VL. The induction of apoptosis by Shiga toxins and ricin. Curr Top Microbiol Immunol. 2012;357:137–78. doi:10.1007/82_2011_155.
- Simmons BM, Stahl PD, Russell JH. Mannose receptor-mediated uptake of ricin toxin and ricin A chain by macrophages. Multiple intracellular pathways for a chain translocation. J Biol Chem. 1986;261:7912–20.
- Gal Y, Mazor O, Falach R, Sapoznikov A, Kronman C, Sabo T. Treatments for Pulmonary Ricin Intoxication: current Aspects and Future Prospects. Toxins (Basel). 2017;9:311. doi:10.3390/toxins9100311.
- Pincus SH, Bhaskaran M, Brey RN 3rd, Didier PJ, Doyle-Meyers LA, Roy CJ. Clinical and pathological findings associated with aerosol exposure of macaques to ricin toxin. Toxins (Basel). 2015;7:2121–33. doi:10.3390/toxins7062121.
- Brown RF, White DE. Ultrastructure of rat lung following inhalation of ricin aerosol. Int J Exp Pathol. 1997;78:267–76. doi:10.1046/j.1365-2613.1997.300363.x.
- Lindauer ML, Wong J, Iwakura Y, Magun BE. Pulmonary inflammation triggered by ricin toxin requires macrophages and IL-1 signaling. J Immunol. 2009;183:1419–26. doi:10.4049/jimmunol.0901119.
- Wong J, Korcheva V, Jacoby DB, Magun B. Intrapulmonary delivery of ricin at high dosage triggers a systemic inflammatory response and glomerular damage. Am J Pathol. 2007;170:1497–510. doi:10.2353/ajpath.2007.060703.
- Roy CJ, Song K, Sivasubramani SK, Gardner DJ, Pincus SH. Animal models of ricin toxicosis. Curr Top Microbiol Immunol. 2012;357:243–57.
- Sully EK, Whaley KJ, Bohorova N, Bohorov O, Goodman C, Kim DH, Pauly MH, Velasco J, Hiatt E, Morton J, et al. Chimeric plantibody passively protects mice against aerosolized ricin challenge. Clin Vaccine Immunol. 2014;21:777–82. doi:10.1128/CVI.00003-14.
- Van Slyke G, Sully EK, Bohorova N, Bohorov O, Kim D, Pauly MH, Whaley KJ, Zeitlin L, Mantis NJ. Humanized monoclonal antibody that passively protects mice against systemic and intranasal ricin toxin challenge. Clin Vaccine Immunol. 2016;23:795–99. doi:10.1128/CVI.00088-16.
- Wahome PG, Robertus JD, Mantis NJ. Small-molecule inhibitors of ricin and Shiga toxins. Curr Top Microbiol Immunol. 2012;357:179–207. doi:10.1007/82_2011_177.
- Reisler RB, Smith LA. The need for continued development of ricin countermeasures. Adv Prev Med. 2012;2012:149737. doi:10.1155/2012/149737.
- Wolfe DN, Florence W, Bryant P. Current biodefense vaccine programs and challenges. Hum Vaccin Immunother. 2013;9:1591–97. doi:10.4161/hv.24063.
- Pittman PR, Reisler RB, Lindsey CY, Guerena F, Rivard R, Clizbe DP, Chambers M, Norris S, Smith LA. Safety and immunogenicity of ricin vaccine, RVEc, in a Phase 1 clinical trial. Vaccine. 2015;33:7299–306. doi:10.1016/j.vaccine.2015.10.094.
- Vance DJ, Mantis NJ. Progress and challenges associated with the development of ricin toxin subunit vaccines. Expert Rev Vaccines. 2016;15:1213–22. doi:10.1586/14760584.2016.1168701.
- Vitetta ES, Smallshaw JE, Schindler J. Pilot phase IB clinical trial of an alhydrogel-adsorbed recombinant ricin vaccine. Clin Vaccine Immunol. 2012;19:1697–99. doi:10.1128/CVI.00381-12.
- Froude JW, Stiles B, Pelat T, Thullier P. Antibodies for biodefense. MAbs. 2011;3:517–27. doi:10.4161/mabs.3.6.17621.
- Wagner EK, Maynard JA. Engineering therapeutic antibodies to combat infectious diseases. Curr Opin Chem Eng. 2018;19:131–41. doi:10.1016/j.coche.2018.01.007.
- Neal LM, O’Hara J, Brey RN 3rd, Mantis NJ. A monoclonal immunoglobulin G antibody directed against an immunodominant linear epitope on the ricin A chain confers systemic and mucosal immunity to ricin. Infect Immun. 2010;78:552–61. doi:10.1128/IAI.00796-09.
- Noy-Porat T, Alcalay R, Epstein E, Sabo T, Kronman C, Mazor O. Extended therapeutic window for post-exposure treatment of ricin intoxication conferred by the use of high-affinity antibodies. Toxicon. 2017;127:100–05. doi:10.1016/j.toxicon.2017.01.009.
- Poli MA, Rivera VR, Pitt ML, Vogel P. Aerosolized specific antibody protects mice from lung injury associated with aerosolized ricin exposure. Toxicon. 1996;34:1037–44. doi:10.1016/0041-0101(96)00047-5.
- Prigent J, Panigai L, Lamourette P, Sauvaire D, Devilliers K, Plaisance M, Volland H, Créminon C, Simon S, Sozzani S. Neutralising antibodies against ricin toxin. PLoS One. 2011;6:e20166. doi:10.1371/journal.pone.0020166.
- Rong Y, Van Slyke G, Vance DJ, Westfall J, Ehrbar D, Mantis NJ. Spatial location of neutralizing and non-neutralizing B cell epitopes on domain 1 of ricin toxin’s binding subunit. PLoS One. 2017;12:e0180999. doi:10.1371/journal.pone.0180999.
- Van Slyke G, Angalakurthi SK, Toth R, Vance DJ, Rong Y, Ehrbar D, Shi Y, Middaugh CR, Volkin DB, Weis DD, et al. Fine-specificity epitope analysis identifies contact points on ricin toxin recognized by protective monoclonal antibodies. Immunohorizons. 2018;2:262–73. doi:10.4049/immunohorizons.1800042.
- Roy CJ, Ehrbar DJ, Bohorova N, Bohorov O, Kim D, Pauly M, Davis JL, Dong B, Sun C, Page P, et al. Rescue of rhesus macaques from the lethality of aerosolized ricin toxin. JCI Insight. 2019;4. doi:10.1172/jci.insight.122686.
- Acquaye-Seedah E, Huang Y, Sutherland JN, DiVenere AM, Maynard JA. Humanised monoclonal antibodies neutralise pertussis toxin by receptor blockade and reduced retrograde trafficking. Cell Microbiol. 2018;20:e12948. doi:10.1111/cmi.12948.
- Nguyen AW, Wagner EK, Laber JR, Goodfield LL, Smallridge WE, Harvill ET, Papin JF, Wolf RF, Padlan EA, Bristol A, Kaleko M. A. A cocktail of humanized anti-pertussis toxin antibodies limits disease in murine and baboon models of whooping cough. Sci Transl Med. 2015;7:316ra195. doi:10.1126/scitranslmed.aad3106.
- Nowakowski A, Wang C, Powers DB, Amersdorfer P, Smith TJ, Montgomery VA, Sheridan R, Blake R, Smith LA, Marks JD. Potent neutralization of botulinum neurotoxin by recombinant oligoclonal antibody. Proc Natl Acad Sci U S A. 2002;99:11346–50. doi:10.1073/pnas.172229899.
- Pohl MA, Rivera J, Nakouzi A, Chow SK, Casadevall A. Combinations of monoclonal antibodies to anthrax toxin manifest new properties in neutralization assays. Infect Immun. 2013;81:1880–88. doi:10.1128/IAI.01328-12.
- Toth, RT IV, Angalakurthi SK, Van Slyke G, Vance DJ, Hickey JM, Joshi SB, Middaugh CR, Volkin DB, Weis DD, Mantis NJ. High-definition mapping of four spatially distinct neutralizing epitope clusters on rivax, a candidate ricin toxin subunit vaccine. Clin Vaccine Immunol. 2017;24. pii: e00237-17. doi:10.1128/CVI.00237-17.
- Yermakova A, Klokk TI, O’Hara JM, Cole R, Sandvig K, Mantis NJ. Neutralizing monoclonal antibodies against disparate epitopes on ricin toxin’s enzymatic subunit interfere with intracellular toxin transport. Sci Rep. 2016;6:22721. doi:10.1038/srep22721.
- Coligan J. Short protocols in immunology. Hoboken (NJ): John Wiley & Sons, Inc.; 2005.
- Ramos-Vara JA, Beissenherz ME. Optimization of immunohistochemical methods using two different antigen retrieval methods on formalin-fixed paraffin-embedded tissues: experience with 63 markers. J Vet Diagn Invest. 2000;12:307–11. doi:10.1177/104063870001200402.
- Yermakova A, Mantis NJ. Protective immunity to ricin toxin conferred by antibodies against the toxin’s binding subunit (RTB). Vaccine. 2011;29:7925–35. doi:10.1016/j.vaccine.2011.08.075.
- Yermakova A, Mantis NJ. Neutralizing activity and protective immunity to ricin toxin conferred by B subunit (RTB)-specific Fab fragments. Toxicon. 2013;72:29–34. doi:10.1016/j.toxicon.2013.04.005.
- Mbawuike IN, Herscowitz HB. MH-S, a murine alveolar macrophage cell line: morphological, cytochemical, and functional characteristics. J Leukoc Biol. 1989;46:119–27. doi:10.1002/jlb.46.2.119.
- Brenner TA, Rice TA, Anderson ED, Percopo CM, Rosenberg HF. Immortalized MH-S cells lack defining features of primary alveolar macrophages and do not support mouse pneumovirus replication. Immunol Lett. 2016;172:106–12. doi:10.1016/j.imlet.2016.02.012.
- Bhaskaran M, Didier PJ, Sivasubramani SK, Doyle LA, Holley J, Roy CJ. Pathology of lethal and sublethal doses of aerosolized ricin in rhesus macaques. Toxicol Pathol. 2014;42:573–81. doi:10.1177/0192623313492248.
- Sabo T, Gal Y, Elhanany E, Sapoznikov A, Falach R, Mazor O, Kronman C. Antibody treatment against pulmonary exposure to abrin confers significantly higher levels of protection than treatment against ricin intoxication. Toxicol Lett. 2015;237:72–78. doi:10.1016/j.toxlet.2015.06.003.
- Sapoznikov A, Falach R, Mazor O, Alcalay R, Gal Y, Seliger N, Sabo T, Kronman C. Diverse profiles of ricin-cell interactions in the lung following intranasal exposure to ricin. Toxins (Basel). 2015;7:4817–31. doi:10.3390/toxins7114817.
- DaSilva L, Cote D, Roy C, Martinez M, Duniho S, Pitt ML, Downey T, Dertzbaugh M. Pulmonary gene expression profiling of inhaled ricin. Toxicon. 2003;41:813–22. doi:10.1016/s0041-0101(03)00035-7.
- Bhatia M, Zemans RL, Jeyaseelan S. Role of chemokines in the pathogenesis of acute lung injury. Am J Respir Cell Mol Biol. 2012;46:566–72. doi:10.1165/rcmb.2011-0392TR.
- Shushakova N, Skokowa J, Schulman J, Baumann U, Zwirner J, Schmidt RE, Gessner JE. C5a anaphylatoxin is a major regulator of activating versus inhibitory FcgammaRs in immune complex-induced lung disease. J Clin Invest. 2002;110:1823–30. doi:10.1172/JCI16577.
- Korcheva V, Wong J, Lindauer M, Jacoby DB, Iordanov MS, Magun B. Role of apoptotic signaling pathways in regulation of inflammatory responses to ricin in primary murine macrophages. Mol Immunol. 2007;44:2761–71. doi:10.1016/j.molimm.2006.10.025.
- Herrera C, Tremblay JM, Shoemaker CB, Mantis NJ. Mechanisms of ricin toxin neutralization revealed through engineered homodimeric and heterodimeric camelid antibodies. J Biol Chem. 2015;290:27880–89. doi:10.1074/jbc.M115.658070.
- Smallshaw JE, Richardson JA, Vitetta ES. RiVax, a recombinant ricin subunit vaccine, protects mice against ricin delivered by gavage or aerosol. Vaccine. 2007;25:7459–69. doi:10.1016/j.vaccine.2007.08.018.
- Wilhelmsen CL, Pitt ML. Lesions of acute inhaled lethal ricin intoxication in rhesus monkeys. Vet Pathol. 1996;33:296–302. doi:10.1177/030098589603300306.
- Wong J, Magun BE, Wood LJ. Lung inflammation caused by inhaled toxicants: a review. Int J Chron Obstruct Pulmon Dis. 2016;11:1391–401. doi:10.2147/COPD.S106009.
- Hodges AL, Kempen CG, McCaig WD, Parker CA, Mantis NJ, LaRocca TJ. TNF family cytokines induce distinct cell death modalities in the a549 human lung epithelial cell line when administered in combination with ricin toxin. Toxins (Basel). 2019;11:450. doi:10.3390/toxins11080450.
- Rong Y, Westfall J, Ehrbar D, LaRocca T, Mantis NJTRAIL. (CD253) sensitizes human airway epithelial cells to toxin-induced cell death. mSphere. 2018;3. doi:10.1128/mSphere.00399-18.
- Cook DL, David J, Griffiths GD. Retrospective identification of ricin in animal tissues following administration by pulmonary and oral routes. Toxicology. 2006;223:61–70. doi:10.1016/j.tox.2006.03.010.
- Doebler JA, Wiltshire ND, Mayer TW, Estep JE, Moeller RB, Traub RK, Broomfield CA, Calamaio CA, Thompson WL, Pitt ML. The distribution of [125I]ricin in mice following aerosol inhalation exposure. Toxicology. 1995;98:137–49. doi:10.1016/0300-483x(94)02978-4.
- Falach R, Sapoznikov A, Gal Y, Israeli O, Leitner M, Seliger N, Ehrlich S, Kronman C, Sabo T. Quantitative profiling of the in vivo enzymatic activity of ricin reveals disparate depurination of different pulmonary cell types. Toxicol Lett. 2016;258:11–19. doi:10.1016/j.toxlet.2016.06.003.
- Wong J, Korcheva V, Jacoby DB, Magun BE. Proinflammatory responses of human airway cells to ricin involve stress-activated protein kinases and NF-kappaB. Am J Physiol Lung Cell Mol Physiol. 2007;293:L1385–94. doi:10.1152/ajplung.00207.2007.
- McGuinness CR, Mantis NJ. Characterization of a novel high-affinity monoclonal immunoglobulin G antibody against the ricin B subunit. Infect Immun. 2006;74:3463–70. doi:10.1128/IAI.00324-06.
- Maddaloni M, Cooke C, Wilkinson R, Stout AV, Eng L, Pincus SH. Immunological characteristics associated with the protective efficacy of antibodies to ricin. J Immunol. 2004;172:6221–28. doi:10.4049/jimmunol.172.10.6221.
- Yermakova A, Vance DJ, Mantis NJ. Sub-domains of ricin’s b subunit as targets of toxin neutralizing and non-neutralizing monoclonal antibodies. PLoS One. 2012;7:e44317. doi:10.1371/journal.pone.0044317.
- Sandvig K, Olsnes S, Pihl A. Kinetics of binding of the toxic lectins abrin and ricin to surface receptors of human cells. J Biol Chem. 1976;251:3977–84.
- Yermakova A, Klokk TI, Cole R, Sandvig K, Mantis NJ. Antibody-mediated inhibition of ricin toxin retrograde transport. MBio. 2014;5:e00995. doi:10.1128/mBio.00995-13.
- Herrera C, Mantis NJ, Cole R. Applications in stimulated emission depletion microscopy: localization of a protein toxin in the endoplasmic reticulum following retrograde transport. Microsc Microanal. 2016;22:1113–19. doi:10.1017/S1431927616011879.
- Sowa-Rogozinska N, Sominka H, Nowakowska-Golacka J, Sandvig K, Slominska-Wojewodzka M. Intracellular transport and cytotoxicity of the protein toxin ricin. Toxins (Basel). 2019;11:350. doi:10.3390/toxins11060350.
- Mooney B, Torres-Velez FJ, Doering J, Ehrbar DJ, Mantis NJ. Sensitivity of Kupffer cells and liver sinusoidal endothelial cells to ricin toxin and ricin toxin-Ab complexes. J Leukoc Biol. 2019. doi:10.1002/JLB.4A0419-123R.
- Lee SJ, Evers S, Roeder D, Parlow AF, Risteli J, Risteli L, Lee YC, Feizi T, Langen H, Nussenzweig MC. Mannose receptor-mediated regulation of serum glycoprotein homeostasis. Science. 2002;295:1898–901. doi:10.1126/science.1069540.
- Gage E, Hernandez MO, O’Hara JM, McCarthy EA, Mantis NJ. Role of the mannose receptor (CD206) in immunity to ricin. Toxins (Basel). 2011;3(9):1131–45. doi:10.3390/toxins3091131.
- Yang B, Schaefer A, Wang YY, McCallen J, Lee P, Newby JM, Arora H, Kumar PA, Zeitlin L, Whaley KJ, et al. ZMapp reinforces the airway mucosal barrier against ebola virus. J Infect Dis. 2018;218:901–10. doi:10.1093/infdis/jiy230.
- Bagchi S, Fredriksson R, Wallen-Mackenzie A. In situ Proximity Ligation Assay (PLA). Methods Mol Biol. 2015;1318:149–59. doi:10.1007/978-1-4939-2742-5_15.
- Anosova NG, Cole LE, Li L, Zhang J, Brown AM, Mundle S, Zhang J, Ray S, Ma F, Garrone P, et al. A combination of three fully human toxin a- and toxin b-specific monoclonal antibodies protects against challenge with highly virulent epidemic strains of clostridium difficile in the hamster model. Clin Vaccine Immunol. 2015;22:711–25. doi:10.1128/CVI.00763-14.
- Cole LE, Li L, Jetley U, Zhang J, Pacheco K, Ma F, Zhang J, Mundle S, Yan Y, Barone L, et al. Deciphering the domain specificity of C. difficile toxin neutralizing antibodies. Vaccine. 2019;37:3892–901. doi:10.1016/j.vaccine.2019.05.040.
- Abboud N, Chow SK, Saylor C, Janda A, Ravetch JV, Scharff MD, Casadevall A. A requirement for FcgammaR in antibody-mediated bacterial toxin neutralization. J Exp Med. 2010;207:2395–405. doi:10.1084/jem.20100995.
- Chow SK, Casadevall A. Monoclonal antibodies and toxins–a perspective on function and isotype. Toxins (Basel). 2012;4:430–54. doi:10.3390/toxins4060430.