ABSTRACT
Polysaccharides isolated from natural plants may represent a novel source of vaccine adjuvants. In this research, we focused on a natural plant polysaccharide, PCP-I, which is derived from Poria cocos, a Chinese traditional herbal medicine. We chose the anthrax protective antigen (PA) as a model to evaluate the adjuvant ability of PCP-I in enhancing the immunogenicity and protection of a PA-based anthrax vaccine. According to our results, PCP-I could significantly enhance anthrax specific anti-PA antibodies, toxin-neutralizing antibodies, anti-PA antibody affinity, as well as IgG1 and IgG2a levels. Besides, PCP-I increased the frequency of PA-specific memory B cells, increased the proliferation of PA-specific splenocytes, significantly stimulated the secretion of IL-4, and enhanced the activation of Dendritic cells (DCs) in vitro. The combination of PCP-I and CpG significantly enhanced the level of anti-PA antibodies and neutralizing antibodies, particularly PA-specific IgG2a, and shifted the Th2-bias to a Th1/Th2 balanced response. In addition, PCP-I with or without CpG could significantly improve the survival rate of immunized mice following challenge with the anthrax lethal toxin. These findings suggest that PCP-I may be a promising vaccine adjuvant that warrants further study.
Introduction
Prophylactic vaccines are one of the most effective tools for preventing infectious diseases. Currently, most novel vaccines are highly purified recombinant protein vaccines. Although these vaccines are typically safer compared to inactivated or live-attenuated vaccines, they are generally less immunogenic and thus require the addition of an adjuvant to generate an effective immune response.
Since the number of currently approved vaccine adjuvants remain very limited and they fail to meet the need to immunize against a wide range of different pathogens, the identification and development of new vaccine adjuvants is urgently required.Citation1,Citation2
Polysaccharides isolated from natural plants may represent a novel source of vaccine adjuvants. First, polysaccharides derived from plant species are nontoxic and biodegradable with a defined structure and composition. Second, such polysaccharides can be extracted from Chinese herbal medicine, the raw materials are abundant, and they are easy to isolate and purify,Citation3,Citation4 thus meeting the requirements for large-scale production. Furthermore, polysaccharides can be recognized by multiple receptors in vivo (e.g., toll-like receptors (TLRs), nod-like receptors (NLRs), and C-type lectins),Citation3 suggesting that polysaccharide-based adjuvants have the potential to activate the immune response through multiple signaling pathways, and are thus likely to induce a robust and comprehensive response against pathogens. Currently, the most successful natural plant polysaccharide-based adjuvant is Advax, which is derived from delta inulin.Citation5,Citation6 Advax performed well on vaccines against the West Nile virus and Japanese encephalitis virus, influenza, Listeria, and anthrax, among other pathogens, which highlights its broad efficacy across viral, bacterial and parasitic vaccines.Citation7
Anthrax is a zoonotic disease caused by the Gram-positive bacterium Bacillus anthracis (B. anthracis). It is estimated that 1.83 billion people live within regions of anthrax risk in the world.Citation8 Anthrax is also considered a serious biological threat because of its highly lethal effects of exposure via the inhalational route and the potential of weaponizing B. anthracis spores.Citation9,Citation10 The spore-forming B. anthracis is classified as Category A biothreat agent by the Centers for Disease Control and Prevention in the United States.Citation11 Vaccination is the most effective medical approach to anthrax prevention and control. The currently approved anthrax vaccines are Anthrax Vaccine Adsorbed (AVA, United States) and Anthrax Vaccine Precipitated (AVP, United Kingdom). The major functional immunogen of these vaccines is believed to be protective antigen (PA). However, the AVA and AVP still have some drawbacks, such as undefined composition, difficult quality control, and potential reactogenicity, and their efficacy can wane over time due to stability issues in storing alum-based vaccines.Citation12 The recombinant PA (rPA)-based vaccine is regarded as the second-generation anthrax vaccine candidate after AVA and AVP. This kind of vaccine has a clear antigenic component, is easy to prepare, and quality control can be implemented, which effectively reduces the potential safety hazards.
In this research, we focused on a natural plant polysaccharide, PCP-I, which is derived from Poria cocos, a Chinese traditional herb medicine. Poria cocos is a saprophytic fungus and its sclerotium is called “fu-ling” in China. The polysaccharides and derivatives of Poria cocos have been reported to exhibit beneficial biological activities, including anticancer, anti-inflammatory, antioxidant, and antiviral properties.Citation13 Here, we investigated the effects of PCP-I used as an adjuvant to enhance the immunogenicity and protection of an anthrax protective antigen-based vaccine. We also examined the synergistic effect of PCP-I combined with CpG as an adjuvant. Our findings indicate that PCP-I may be a promising vaccine adjuvant that warrants further development.
Materials and methods
Polysaccharide PCP-I preparation
PCP-I was prepared from Poria cocos as described previously.Citation14 Briefly, the sclerotium of Poria cocos was extracted with water, precipitated with ethanol, and then dialyzed and lyophilized to produce total polysaccharides (PCP-50). PCP-50 was passed through a DEAE-cellulose column to obtain two fractions (PCP-50-A and PCP-50-B). PCP-50-A was further isolated and purified using a Sephadex G-100 column and lyophilized to obtain a homogeneous active polysaccharide (PCP-I). PCP-I appeared as a white powder that dissolved readily in water. No endotoxin was found in PCP-I and its molecular weight was estimated as 30 kDa.
Animals and immunization
Female BALB/c mice (6–8 weeks old) were purchased from Beijing Vital River Co.Ltd. (Beijing, China). All animals were bred under specific pathogen-free conditions. All animal experiments were approved by the Animal Care and Use Committee of the Beijing Institute of Biotechnology.
For immunization experiments using PCP-I alone as adjuvants, groups of mice (BALB/c, n = 6) were immunized three times intramuscularly (i.m.) at two-week intervals with 0.5 μg of PA (PA was expressed and purified as described previouslyCitation15) unadjuvanted or adjuvanted with PCP-I (200 μg); mice from the control group were injected with PBS.
For immunization experiments using PCP-I combined with CpG (ODN 2006, Invivogen, Cat#tlrl-2006-5) as adjuvants, Groups of mice (BALB/c, n = 8) were immunized three times i.m. at two-week intervals with 0.5 μg of PA unadjuvanted or adjuvanted with PCP-I (200 μg) or PCP-I (200 μg) + CpG (25 μg) or CpG (25 μg); mice from the control group were injected with PBS. Four weeks after the last immunization, the mice were challenged with the anthrax lethal toxin. The anthrax lethal toxin (PA plus Lethal Factor (LF)), was prepared as described previously.Citation15
For the lymphocyte-mediated immunity experiment, groups of mice (BALB/c, n = 6) were immunized three times i.m. at two-week intervals with 5 μg of PA unadjuvanted or adjuvanted with PCP-I (200 μg). The mice from the control group were injected with PBS.
The mice were bled via tail bleeding two weeks after the third immunization, and splenocytes were also isolated from mice two weeks after the third immunization.
Serological tests
Enzyme-linked immunosorbent assay (ELISA)
The levels of PA-specific IgG, IgG1, and IgG2a were determined by ELISA as described previously, with minor modifications.Citation15 Serum samples were twofold serially diluted in 0.1% BSA/PBST (1:100 for total IgG or IgG1 antibodies and 1:50 for IgG2a) and incubated for 1 h at 37°C. After washing, horseradish peroxidase (HRP)-conjugated goat anti-mouse IgG (Santa Cruz, Cat#sc-2031), IgG1 (Santa Cruz, Cat#sc-2060), and IgG2a (Santa Cruz, Cat#sc-2061) were added and the samples incubated for 45 min at 37°C. The final OD was calculated as OD450 -OD630. The titers were presented as the highest sample dilution resulting in an OD greater than 2.1 times the mean of the OD of the nonimmune control sera.
Antibody avidity test
The antibody avidity assay was performed as an ELISA test with one additional step. NH4SCN (Sigma, Cat#V900169) at a concentration of 1.0 M was added to the ELISA plates after the incubation with the tested sera, and the mixture was incubated for 15 min at 37°C.Citation16 After washing with PBS containing 0.05% Tween 20, the ELISA procedure was continued as described above. The following calculation was used to derive the affinity constant A:
Affinity constant A = Log Titer (+NH4SCN)/Log Titer (-NH4SCN)
In vitro toxin neutralization assay (TNA)
Anthrax lethal toxin (50 ng/mL of PA plus 40 ng/mL of LF) was pre-incubated with a range of dilutions of mouse serum (from 1:50; duplicate wells were used for each dilution) in the same medium for 45 min at 37°C. Then, 100 μL of the sera-toxin mixture replaced the original medium in each well with the J774.1 cells, and the cells were incubated for 4 h at 37°C. Cell viability was analyzed using the average OD570-OD630 for each pair of wells treated with the MTT assay. The ED50 was calculated by four-parameter logistic curve fitting using SigmaPlot 12.0.
Lymphocyte proliferation assay
The assay was performed as described previously, with minor modifications.Citation17 Isolated splenocytes were plated at a density of 4 × 105 cells/well in 96-well round bottom cell culture plates. The cells were incubated with PA (10 μg/mL) at 37°C and 5% CO2 for 68 h, and then incubated with 10 μL CCK8 (Dojindo Laboratories, Cat#CK04) for 4 h at 37°C. Cells stimulated with ConA (Sigma, Cat#C5275) (1 μg/mL) or medium alone served as positive and negative controls, respectively. The proliferation index (PI) was calculated as OD PA/ODmedium.
Cytokine production assay
Isolated splenocytes were plated at a density of 4 × 105 cells/well in U-bottom 96-well cell culture plates. The cells were incubated with PA (10 μg/mL) at 37°C and 5% CO2 for three days. The supernatants were then harvested, and the cytokines were measured using MILLIPLEX multiplex assays (Millipore) and analyzed using MILLIPLEX Analyst 5.1 software (Millipore).
Enzyme-linked immunospot assay (ELISPOT)
To determine the number of PA-specific memory B cells, freshly collected splenocytes were cultured at a density of 1 × 106 cells/mL in complete medium with 1 μg/mL R848 (Invivogen, Cat#tlrl-r848-5) plus 10 ng/mL IL-2 (R&D, Cat#402-ML-100) at 37°C, 5% CO2 for four days. The cells were collected, washed with medium, and plated for ELISPOT analyzes on plates that were previously coated with 100 μL PBS containing 10 μg/mL of PA or 10 μg/mL of goat anti-mouse IgG Fc (Abcam, Cat#ab97261) overnight at 4°C, and then blocked for 2 h at 37°C with PBS containing 2% BSA. The plates were washed after an 18 h incubation with the cells, incubated with HRP-conjugated rabbit anti-mouse IgG (1:1000, Abcam, Cat#ab6728), and developed using the aminoethyl carbazole (AEC) substrate solution (BD Bioscience, Cat#551951). Spots corresponding to Ab-secreting cells were counted using the AT-Spot2100 ELISPOT plate Analyzer (AntaiYongxin Medical Technology).
The numbers of PA-specific cytokine-secreting cells were analyzed using a mouse IFN-γ/IL-4 precoated ELISPOT kit (Dakewei, Cat#2210005/Cat#2210402), in accordance with the manufacturer’s instructions. Briefly, freshly collected splenocytes were plated at a density of 4 × 105 cells/well in 96-well plates, incubated with PA (10 μg/mL) at 37°C and 5% CO2 for 48 h. Cells stimulated with ConA (1 μg/mL) or medium alone served as the positive and negative controls. Wells were washed, incubated with biotinylated anti-mouse IL-4 or IFN-γ monoclonal antibodies at 37°C for 1 h, and then washed again. Streptavidin-HRP was then added and incubated at 37°C for 1 h before washing and adding the AEC substrate solution. Spots corresponding to cytokine-secreting cells were counted using an AT-Spot2100 ELISPOT plate Analyzer.
Dendritic cell (DC) activation
Bone marrow-derived DCs (BMDCs) were generated by cultivating bone marrow cells in RPMI 1640 supplemented with 10% fetal bovine serum (FBS), 1% Penicillin/Streptomycin solution, 20 ng/mL mGM-CSF(R&D, Cat#415-ML-020) and 10 ng/mL mIL-4 (R&D, Cat#415-ML-020) for seven days. The cells were stimulated with PA 50 μg/mL + PCP-I 2 mg/mL, PA 50 μg/mL, LPS (Sigma, Cat#L4391) 2 μg/mL, or PBS for 24 h. The expression of CD11c (BD Pharmingen clone HL3, Cat#553801), MHC II (BD Pharmingen clone AF6-120.1, Cat#562823), and CD86 (BD Pharmingen clone GL1, Cat#560582) on DCs was evaluated by FACSVerse (BD).
Challenge experiment
For the lethal toxin challenge experiment, immunized BALB/c mice were challenged by an intravenous administration of anthrax lethal toxin (25 μg LF plus 50 μg PA in 0.1 mL of PBS per mouse) into the tail vein at four weeks after the last immunization. Survival was monitored every 8 h for seven days.
Statistical analysis
GraphPad Prism 5.0 (GraphPad Software, USA) was used to create the graphs and for statistical analysis. Significant differences between experimental and control groups were analyzed using a one-way or two-way ANOVA. Animal survival curves were created using the Kaplan-Meier method, and statistical analyses of survival curves were performed using a log-rank test.
Results
PCP-I enhances antibody responses against PA
The antibody response is the main indicator for measuring the efficacy of the anthrax vaccine. To evaluate the immune-enhancing ability of the polysaccharide adjuvant PCP-I derived from Poria cocos, the antibody response against PA was measured in BALB/c mice. Mice were bled two weeks after the last immunization and anti-PA IgG was determined by ELISA. Compared to the PA alone group, the addition of PCP-I to PA showed an 11.0-fold increase in PA-specific IgG titers (1.82 × 104 vs. 1.62 × 103) ()), indicating a strong immune enhancement effect of the polysaccharide adjuvant PCP-I.
Figure 1. PCP-I enhances antibody responses against PA. Groups of mice (BALB/c, n = 6) were immunized three times i.m. at two-week intervals with 0.5 μg of PA unadjuvanted or adjuvanted with PCP-I (200 μg). The mice of the control group were injected with PBS. Mice were bled 14 days following their last immunization for measurement of anti-PA antibodies (a), antibody avidity (b), and IgG subclass titers (c) by ELISA. The toxin-neutralizing antibody titers were measured by TNA (d). Results are presented as the mean ± SD (*p < .05, ***p < .001).
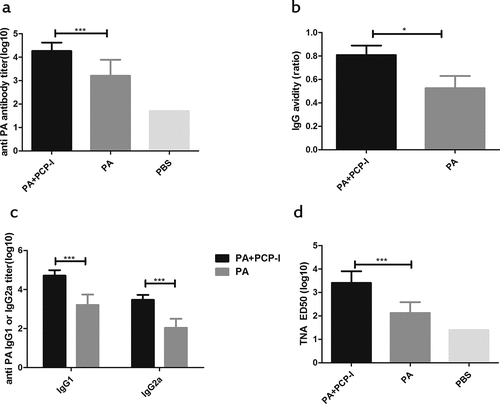
Avidity is one index of the quality of the antibody response that is influenced by the antibody valency and antibody-antigen binding affinity. We used an ELISA-based assay to assess avidity. The results showed that PA+PCP-I significantly induced a more enhanced high-affinity antibody response (Affinity constant A = 0.80) than that induced by PA alone (Affinity constant A = 0.52) ()). This result suggested that the polysaccharide adjuvant PCP-I significantly enhanced the binding affinity of PA-specific antibodies to PA in the serum of the immunized mice.
To further determine the effects of PCP-I on the PA-specific antibody response, the PA-specific IgG1 and IgG2a titers were examined. As shown in ), the PA-specific IgG1 and IgG2a antibody titers induced by the PA+ PCP-I group were significantly higher than that of the PA group (5.13 × 104 vs. 1.58 × 103; 2.95 × 103 vs. 1.09 × 102), with an approximately 32-fold or 27-fold increase in IgG1 or IgG2a, respectively. These results suggested that PCP-I significantly enhanced the titer level of the PA-specific IgG subclass.
The TNA assay measured the ability of the immunized mouse serum to inhibit the toxin-mediated killing of the macrophage cell line J774A.1. As show in ), PA + PCP-I induced a neutralizing antibody with an ED50 of 2.57 × 103, which was 19.5-fold significantly higher than that of the PA alone group (1.32 × 102). This indicated that the polysaccharide adjuvant PCP-I could increase the PA-specific neutralizing antibody and protect the J774A.1 cells from the toxin-mediated death.
PCP-I enhances immune memory against PA
Successful vaccination rests on immune memory.Citation18 An ELISPOT assay was used to determine the effect of PCP-I on the generation of PA-specific memory B-cell responses. Fourteen days following the last immunization, splenocytes from immunized mice were stimulated for six days to allow memory B cells to differentiate into antibody-secreting cells (ASCs). The frequency of PA-specific ASCs as a percentage of the total IgG-secreting cells was assessed. The PA + PCP-I group induced a greatly higher frequency of PA-specific memory B-cells (4.26%) than that in the PA alone group (0.87%) ()). This result suggested that PCP-I adjuvant significantly increased the number of PA-specific IgG memory B cells, and may help to maintain a persistent immunity against PA.
Figure 2. PCP-I enhances immune memory against PA. Groups of mice (BALB/c, n = 6) were immunized three times i.m. at two-week intervals with 5 μg of PA unadjuvanted or adjuvanted with PCP-I (200 μg). The control group of mice was injected with PBS. Spleens were collected 14 days after the last immunization to measure the frequency of PA-specific memory B cells by ELISPOT (a). PA-specific splenocyte proliferation was assessed by CCK8 (b). The number of PA-specific cytokine IL-4-secreting cells (c) and IFN-γ-secreting cells (d) was quantified by ELISPOT. PA-specific cytokine expression in the culture supernatant as assessed via MILLIPLEX Multiplex Assays (e). The results are presented as the mean ± SD (*p < .05; **p < .01; ***p < .001).
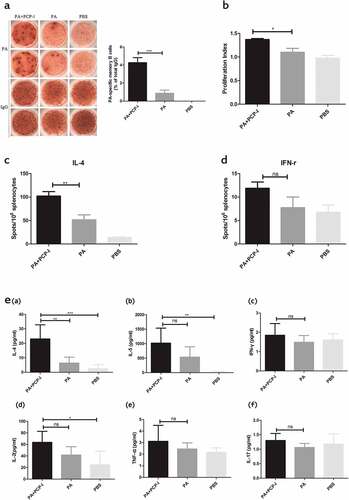
The effect of PCP-I on PA-specific T cell responses was measured by an in vitro splenocytes proliferation assay. As shown in ), significant PA-specific proliferation was obtained in the PA + PCP-I group (PI = 1.37), while the proliferation in mice immunized with PA alone or the PBS groups was only 1.10 and 0.98 (PI), respectively.
To further characterize the influence of PCP-I to cytokine production by PA-specific memory T cells, the frequency of T cells secreting Th1 and Th2 cytokines in response to the PA stimulation was measured using a cytokine ELISPOT assay (). IL-4 is the predominant cytokine secreted by Th2 cells. Consistent with the significant enhancement of serum IgG1 (a Th2 isotype) by PCP-I, this group exhibited a significantly higher frequency of IL-4-secreting T cells (102/106) compared to the other groups (PA, 51.5/106; PBS, 13.75/106) (p < .05). The frequencies of IFN-γ-secreting cells, a marker of Th1 cells, were very low, at approximately 11.88/106 (PA+PCP-I), 7.75/106(PA), and 6.75/106 (PBS). These results suggested that PCP-I could enhance PA-specific IL-4 (Th2) immune responses.
Cytokine levels in the culture supernatants were also determined using a MILLIPLEX Multiplex Assay (MMA). Splenocytes isolated from mice immunized with PA + PCP-I exhibited significantly increased expression of IL-4 (23.01pg/mL) compared with cells from the PA immunized alone group (6.34 pg/mL) by MMA, with no difference in the level of IL-5 (1020.78 vs. 534.78 pg/mL), IFN-γ (1.85 vs. 1.48 pg/mL), IL-2(63.52 vs. 41.73 pg/mL), TNF-α (3.11 vs. 2.45 pg/mL), and IL-17 (1.30 vs. 1.06 pg/mL) expression ()). The higher expression of IL-4 induced by PA+ PCP-I was also consistent with the significant enhancement of PA-specific IL-4 spots in the ELISPOT assay.
PCP-I enhances the activation of PA-specific DCs in vitro
DCs are considered to be the initiators of the adaptive T cell immune response, and play a central role in adjuvant-induced immune responses.Citation19 We used BMDCs to investigate the ability of the different preparations to activate PA-specific DCs in vitro. As shown in , PA + PCP-I could significantly up-regulate the expression of MHC-II (74.03%) and CD86 (79.70%) on the surface of DCs, compared to the PA (60.9% and 63.17%, respectively) and PBS (60.97% and 62.63%, respectively) stimulated groups. This suggested that PCP-I could significantly promote the maturation of DCs.
Figure 3. PCP-I enhances the activation of PA-specific DCs in vitro. Bone marrow-derived DCs were stimulated with 50 μg/mL PA +PCP-I 2 mg/mL, 50 μg/ml PA, 2 μg/mL LPS, or PBS for 24 h in vitro. The cells were stained and the expression of CD11c/MHCII (a), CD11c/CD86 (b) on DCs was analyzed by FACS. Results are representative of two separate experiments (***p < .001).
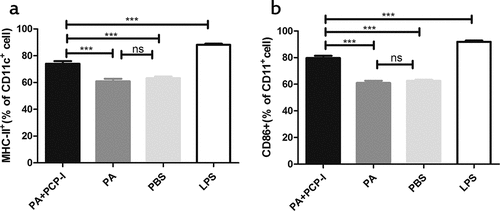
PCP-I combined with CpG cooperate to produce enhanced immune responses against PA and elicit a TH1/TH2 balanced response
Recently, the development of adjuvants composed of multiple types of immune agonists has shown promise.Citation20 It is believed to provide a more effective vaccine by an immune synergy. According to the above results, PCP-I tends to enhance the Th2 immune response. CpG is regarded to be a strong Th1-biased adjuvant, it can induce T cells to promote the development of CTL, and increase secretion of IgG2a antibodies.Citation21 Thus, we evaluated the adjuvant effect of PCP-I combined with CpG.
As shown in , PA + PCP-I + CpG induced a significantly higher anti-PA antibody titer (1.02 × 105) and neutralizing antibody ED50 (1.38 × 104) response compared with the PA + PCP-I group (1.82 × 104 and 2.57 × 103, respectively) and the PA +CpG group (3.55 × 103 and 1.62 × 103, respectively). As shown in ), the avidity of the PA+PCP-I+ CpG group (Affinity constant A = 0.73) was significantly higher than that of the PA+CpG group (Affinity constant A = 0.55); however, there was no significant difference compared to the PA+PCP-I (Affinity constant A = 0.81) group. The results of the PA-specific IgG1 and IgG2a antibody titers showed that the IgG1 titer induced by PA + PCP-I + CpG (7.94 × 104) was slightly higher than that of the PA+PCP-I group (5.13 × 104) (). The IgG2a induced by PA + PCP-I + CpG (5.13 × 104) was almost 17.4-fold higher compared to that of the PA+PCP-I group (2.95 × 103) (p < .001) (). Furthermore, we observed that the response to PA alone was a Th2-biased immune response (IgG1/IgG2a ratio, 1.60) (). Although the addition of PCP-I enhanced the production of PA-specific IgG1 and IgG2a, the response remained Th2-biased (IgG1/IgG2a ratio, 1.36). When PCP-I was combined with CpG, the immune response shifted significantly toward a Th1/Th2 balanced response (IgG1/IgG2a ratio, 1.05). These results indicated that PCP-I combined with CpG can significantly induce a greater magnitude of antibody responses to PA and is prone to elicit a Th1/Th2 balanced immunity.
Figure 4. PCP-I combined with CpG further enhances antibody responses against PA. Groups of mice (BALB/c, n = 8) were immunized three times i.m. at two-week intervals with 0.5 μg of PA unadjuvanted or adjuvanted with PCP-I (200 μg) or PCP-I (200 μg) + CpG (25 μg) or CpG (25 μg). The mice of the control group were injected with PBS. Mice were bled 14 days following their last immunization to measure anti-PA antibodies (a), antibody avidity (c), IgG subclass titers (d (a)), or IgG1/IgG2a ratio (d (b)) by ELISA. The toxin-neutralizing antibody titers were measured by TNA (b). Results are presented as the mean ± SD (*p < .05; **p < .01; ***p < .001).
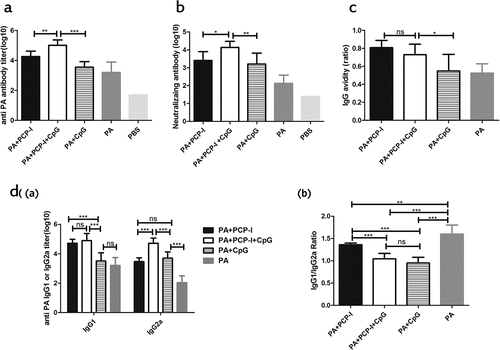
PCP-I with or without CpG improves the mouse survival rate against challenge with anthrax lethal toxin
To assess the ability of PCP-I or PCP-I+ CpG to enhance vaccine-specific protection, immunized BALB/c mice were challenged with anthrax lethal toxin via the tail vein four weeks after the third immunization. As shown in , all of the mice immunized with PBS or PA alone died within 72 h after challenge with anthrax lethal toxin. The PA +CpG group exhibited 50% protection against anthrax toxicity. The groups immunized with PA + PCP-I or PA + PCP-I + CpG, exhibited 75% (6/8) survival, which was significantly higher than the PA immunized group (p < .01) (). Although the anti-PA antibodies and neutralizing antibodies induced by PA + PCP-I + CpG were both higher than that of the PA + PCP-I group, there were no significant differences in survival rate following anthrax toxin challenge between these two groups.
Discussion
The adjuvant is an important part of a vaccine and influences its final efficacy. Polysaccharides belong to a class of natural polymers consisting of glycosidically linked carbohydrate monomers,Citation22 and are believed to have a great potential to be pattern recognition receptor (PRR) agonist adjuvants. Polysaccharides such as dextran, mannan, beta-glucans, and many others are found in the cell walls of bacteria or yeast.Citation23 They act as PRR agonists and have intrinsic targeting abilities to antigen-presenting cells (APCs), and have a natural capacity to enhance the immune response against the associated antigens. There are many approved vaccines using PRR agonists as adjuvants. For example, CpG is the adjuvant of the Hepatitis B vaccine HEPLISAV-B, and is also an agonist of TLR9. Compared to Engerix-B, in which aluminum hydroxide is used as an adjuvant, HEPLISAV-B could induce more rapid and durable antibody responses.Citation21
In previous studies, polysaccharides isolated from certain traditional Chinese herbs, such as the Isatis indigotica root and Poria cocos, were found to possess potential adjuvant activity.Citation2,Citation4,Citation14,4,Citation5–Citation27 Crude total polysaccharides or two purified polysaccharides, PCP-I and PCP-II from Poria cocos could enhance the humoral responses to the H1N1 influenza and HBsAg vaccines.Citation14,Citation24,Citation26 To better characterize the immune-enhancing ability of PCP-I, in the present study we used the PA of B. anthracis as a model antigen, and analyzed systematically the adjuvant capabilities of PCP-I to enhance the immunogenicity and protection of an anthrax protective antigen-based vaccine.
PCP-I is a white powder with a molecular weight of 30 kDa, comprising fucose, mannose, glucose, and galactose in a molar ratio of 1.00:1.81:0.27:7.27.Citation14 According to our results, as an adjuvant, PCP-I was able to increase anthrax-specific anti-PA antibodies, toxin-neutralizing antibodies, anti-PA antibody affinity, as well as the IgG1 and IgG2a levels. PCP-I improved the frequency of PA-specific memory B cells, increased PA-specific splenocyte proliferation, and significantly stimulated the secretion of IL-4. In addition, compared to the PA alone group, the PCP-I adjuvant could significant up-regulate the expression of MHC-II and CD86 on the surface of BMDCs. Mannose is reported to bind to C-type lectins expressed in APCs (DCs and macrophages). These C-type lectins are responsible for the recognition of different carbohydrates present in the cell wall of certain pathogens and lead to the endocytosis or phagocytosis of pathogens, eliciting complement activation and triggering inflammation.Citation22,Citation23 Furthermore, interactions with specific DC C-type lectins have been correlated with DC trafficking and induction of both humoral and cellular responses.Citation23,Citation28 Thus, we suspect that the immune-enhancing effect of PCP-I in vivo may be partially due to the mannose component of PCP-I binding to C-type lectins expressed on DCs. Such binding would serve as a signal, promote both the uptake and processing of PA and the maturation of DCs, accelerate T cell activation and polarization, and facilitate the occurrence of PA-specific acquired immunity. However, further studies are required to verify the precise receptor(s) and molecule(s) that mediate the adjuvant activity and to understand the more detailed mechanisms of action of PCP-I. Furthermore, in our other work, we found PCP-I may enhance the PA-specific immune response by increasing the germinal center response.Citation29
Multiple classes of pathogen-associated molecular patterns (PAMPs) have been successfully used as adjuvants in clinical trials, suggesting that these molecules have considerable potential as new adjuvants.Citation20 It is believed that multi-agonists could activate multi- immune signaling pathways, trigger immune synergies, and provide a more effective immune response. When we combined PCP-I with CpG, we obtained an enhanced anti-PA IgG titer and neutralizing antibody titer, as the IgG2a titer was almost 17.4-fold higher compared to the PCP-I adjuvant alone group, and elicited a Th1/Th2 balanced response. This promotion may result from the overlapping of the immune signaling pathways activated by polysaccharide adjuvant PCP-I and the TLR9 agonist CpG.
In a challenge experiment with the anthrax lethal toxin, the survival rates of mice in the PA+PCP-I and PA +PCP-I + CpG groups were all 75%. One potential reason for the absence of significant differences in the survival rate between the two groups is that the protection against toxin challenge is primarily dependent on toxin-neutralization antibodies, and the toxin challenge model in mice is not as sensitive as the cellular challenge model. Thus, the survival rate could not be entirely consistent with the results of cellular toxin neutralization assay. Besides, we also observed no significant differences in the survival rates of mice among PA+CpG group(50%) and those groups which received PCP-I (PA+PCP-I and PA +PCP-I + CpG,75%). The lack of a significant difference may be due to the adjuvant role of the CpG. Although the CpG adjuvant is a strong Th1-biased adjuvant, and the protective efficacy of anthrax vaccine is mainly mediated by Th2- biased immune response, it doesn’t mean CpG is no use for the ability to enhance the protective efficacy of the anthrax vaccine.
In this work, we investigated the efficacy of PCP-I used as an adjuvant. We proved that PCP-I has a good immune enhancement ability against PA, but the corresponding immune enhancement mechanism of PCP-I remains to be elucidated. The ability of adjuvants to broaden an immune response profile could be crucial to the success of vaccines, and the broadening effect of adjuvants may be mediated via expansion of B cell diversity, not merely through increased titers.Citation2 In the future work, on the one hand we need to detail the B cell subpopulation profiles caused by PCP-I, and on the other hand, we need to adopt new technologies including covalent chemistries, nanoparticulate systems, and so forth to upgrade the study of the PCP-I adjuvant, in order to provide desired antigen release kinetics and immune activation profiles.Citation20
In recent years, the density, age distribution, and travel habits of the global population have undergone fundamental changes, and the changing climate has promoted the emergence of new and old pathogens.Citation30 The outbreak of SARS, MERS, Ebola, Zika, and other epidemics has continuously challenged the global public health response capability. This has rendered urgent and essential the development of new vaccines and new adjuvants. As our understanding of the immune mechanisms increases, and with the advances in the development of reverse vaccinology technology, systemic vaccinology technology, vaccine targeting technology as well as the improvement of adjuvants, delivery technology, and preclinical models, we will be able to face the challenges posed by the emerging infectious diseases.
Disclosure of potential conflicts of interest
No potential conflicts of interest were disclosed.
Acknowledgments
We gratefully thank Yujie Li for technical supports and helpful discussions.
Additional information
Funding
References
- Lee S, Nguyen MT. Recent advances of vaccine adjuvants for infectious diseases. Immune Netw. 2015;15:51–57. doi:10.4110/in.2015.15.2.51.
- Reed SG, Orr MT, Fox CB. Key roles of adjuvants in modern vaccines. Nat Med. 2013;19:1597–608. doi:10.1038/nm.3409.
- Petrovsky N, Cooper PD. Carbohydrate-based immune adjuvants. Expert Rev Vaccines. 2011;10:523–37. doi:10.1586/erv.11.30.
- Rosales-Mendoza S, Salazar-Gonzalez JA, Decker EL, Reski R. Implications of plant glycans in the development of innovative vaccines. Expert Rev Vaccines. 2016;15:915–25. doi:10.1586/14760584.2016.1155987.
- Honda-Okubo Y, Saade F, Petrovsky N. Advax, a polysaccharide adjuvant derived from delta inulin, provides improved influenza vaccine protection through broad-based enhancement of adaptive immune responses. Vaccine. 2012;30:5373–81. doi:10.1016/j.vaccine.2012.06.021.
- Cooper PD, Barclay TG, Ginic-Markovic M, Petrovsky N. The polysaccharide inulin is characterized by an extensive series of periodic isoforms with varying biological actions. Glycobiology. 2013;23:1164–74. doi:10.1093/glycob/cwt053.
- Bruno de Paula Oliveira Santos B, Trentini MM, Machado RB, Rúbia Nunes Celes M, Kipnis A, Petrovsky N, Junqueira-Kipnis AP. Advax4 delta inulin combination adjuvant together with ECMX, a fusion construct of four protective mTB antigens, induces a potent Th1 immune response and protects mice against Mycobacterium tuberculosis infection. Hum Vaccin Immunother. 2017;13:2967–76. doi:10.1080/21645515.2017.1368598.
- Carlson CJ, Kracalik IT, Ross N, Alexander KA, Hugh-Jones ME, Fegan M, Elkin BT, Epp T, Shury TK, Zhang W, et al. The global distribution of Bacillus anthracis and associated anthrax risk to humans, livestock and wildlife. Nat Microbiol. 2019;4:1337–43. doi:10.1038/s41564-019-0435-4.
- Savransky V, Shearer JD, Gainey MR, Sanford DC, Sivko GS, Stark GV, Li N, Ionin B, Lacy MJ, Skiadopoulos MH. Correlation between anthrax lethal toxin neutralizing antibody levels and survival in guinea pigs and nonhuman primates vaccinated with the AV7909 anthrax vaccine candidate. Vaccine. 2017;35:4952–59. doi:10.1016/j.vaccine.2017.07.076.
- Verma A, Burns DL. Improving the stability of recombinant anthrax protective antigen vaccine. Vaccine. 2018;36:6379–82. doi:10.1016/j.vaccine.2018.09.012.
- Majumder S, Das S, Somani VK, Makam SS, Kingston JJ, Bhatnagar R. A bivalent protein r-PAbxpB comprising PA domain IV and exosporium protein BxpB confers protection against B. Anthracis spores and toxin. Front Immunol. 2019;10:498. doi:10.3389/fimmu.2019.00498.
- Weir GM, MacDonald LD, Rajagopalan R, Sivko GS, Valderas MW, Rayner J, Berger BJ, Sammatur L, Stanford MM. Single dose of DPX-rPA, an enhanced-delivery anthrax vaccine formulation, protects against a lethal Bacillus anthracis spore inhalation challenge. NPJ Vaccines. 2019;4:6. doi:10.1038/s41541-019-0102-z.
- Sun Y. Biological activities and potential health benefits of polysaccharides from Poria cocos and their derivatives. Int J Biol Macromol. 2014;68:131–34. doi:10.1016/j.ijbiomac.2014.04.010.
- Wu Y, Li S, Li H, Ma H, Zhao X, Liu K, Wu J, Liu Y, Shan J, Wang Y. Adjuvant effect of a polysaccharide PCP1-Ⅰ from Poria cocos on HBsAg antigen in mice. J Int Pharm Res. 2016;43:307–13.
- Yin Y, Zhang S, Cai C, Zhang J, Dong D, Guo Q, Fu L, Xu J, Chen W. Deletion modification enhances anthrax specific immunity and protective efficacy of a hepatitis B core particle-based anthrax epitope vaccine. Immunobiology. 2014;219:97–103. doi:10.1016/j.imbio.2013.08.008.
- Marcus H, Danieli R, Epstein E, Velan B, Shafferman A, Reuveny S. Contribution of immunological memory to protective immunity conferred by a Bacillus anthracis protective antigen-based vaccine. Infect Immun. 2004;72:3471–77. doi:10.1128/IAI.72.6.3471-3477.2004.
- Liu Y, Yin Y, Wang L, Zhang W, Chen X, Yang X, Xu J, Ma G. Engineering biomaterial-associated complement activation to improve vaccine efficacy. Biomacromolecules. 2013;14:3321–28. doi:10.1021/bm400930k.
- Tarlinton D, Good-Jacobson K. Diversity among memory B cells: origin, consequences, and utility. Science. 2013;341:1205–11. doi:10.1126/science.1241146.
- Lewis KL, Reizis B. Dendritic cells: arbiters of immunity and immunological tolerance. Cold Spring Harb Perspect Biol. 2012;4:a007401. doi:10.1101/cshperspect.a007401.
- Tom JK, Albin TJ, Manna S, Moser BA, Steinhardt RC, Esser-Kahn AP. Applications of immunomodulatory immune synergies to adjuvant discovery and vaccine development. Trends Biotechnol. 2019;37:373–88. doi:10.1016/j.tibtech.2018.10.004.
- Shi S, Zhu H, Xia X, Liang Z, Ma X, Sun B. Vaccine adjuvants: understanding the structure and mechanism of adjuvanticity. Vaccine. 2019;37:3167–78. doi:10.1016/j.vaccine.2019.04.055.
- Sun B, Yu S, Zhao D, Guo S, Wang X, Zhao K. Polysaccharides as vaccine adjuvants. Vaccine. 2018;36:5226–34. doi:10.1016/j.vaccine.2018.07.040.
- Cordeiro AS, Alonso MJ, de la Fuente M. Nanoengineering of vaccines using natural polysaccharides. Biotechnol Adv. 2015;33:1279–93. doi:10.1016/j.biotechadv.2015.05.010.
- Zhang W, Zheng X, Cheng N, Gai W, Xue X, Wang Y, Gao Y, Shan J, Yang S, Xia X. Isatis indigotica root polysaccharides as adjuvants for an inactivated rabies virus vaccine. Int J Biol Macromol. 2016;87:7–15. doi:10.1016/j.ijbiomac.2016.02.023.
- Wu Y, Li S, Li H, Zhao C, Ma H, Zhao X, Wu J, Liu K, Shan J, Wang Y. Effect of a polysaccharide from Poria cocos on humoral response in mice immunized by H1N1 influenza and HBsAg vaccines. Int J Biol Macromol. 2016;91:248–57. doi:10.1016/j.ijbiomac.2016.05.046.
- Li S, Wang Y, Li H, Jia P, Liu K, Jia Q, Shan J, Ma B. Adjuvant effect of total polysaccharides from Poria cocos on H1N1 influenza and HBsAg antigens. Chin J Pharmacol Toxicol. 2015;29:61–64..
- Shan J, Sun G, Ren J, Zhu T, Jia P, Qu W, Li Q, Wu J, Ma H, Wen S, et al. An α-glucan isolated from root of Isatis Indigotica, its structure and adjuvant activity. Glycoconj J. 2014;31:317–26. doi:10.1007/s10719-014-9525-y.
- Apostolopoulos V, Thalhammer T, Tzakos AG, Stojanovska L. Targeting antigens to dendritic cell receptors for vaccine development. J Drug Deliv. 2013;2013:869718. doi:10.1155/2013/869718.
- Liu K, Yin Y, Zhang J, Dong D, Liu J, LI R, Ma H, Shan J, Xu J. Mechanism of a polysaccharides PCP-I from Poria cocos on enhancing antigen-specific humoral immune response. Lett Biotechnol. 2017;28:249–55.
- Rauch S, Jasny E, Schmidt KE, Petsch B. New vaccine technologies to combat outbreak situations. Front Immunol. 2018;9:1963. doi:10.3389/fimmu.2018.01963.