ABSTRACT
In allergen-specific immunotherapy for asthma, antigens attached to dendritic cells increase the tryptophan metabolism in these cells and alter the Th17/Treg balance in the airways. Tryptophan metabolism has long been suggested to be relevant in the pathophysiology of allergic disorders, including asthma. Our study investigated whether tryptophan metabolites are responsible for the changes in Th17/Treg balance and decreases in airway hyperreactivity and inflammation seen during allergen-specific immunotherapy in an asthma model. Ovalbumin was injected intraperitoneally into mice to establish an asthma model, and then high dose ovalbumin allergen-specific immunotherapy was administered to induce immune tolerance. Airway hyperreactivity and serum ovalbumin-specific immunoglobulin E were measured to assess whether the animal model was successfully established. We then examined the influence of inhibition of tryptophan metabolism and the addition of tryptophan metabolites on allergen-specific immunotherapy-induced changes in the Th17/Treg balance and decreases in airway inflammation and inflammatory cytokines. Production of tryptophan metabolites was partly responsible for the allergen-specific immunotherapy-induced increase in Tregs, decrease in airway inflammation, and decrease in inflammatory cytokines. Ovalbumin-specific immunoglobulin E and airway hyperreactivity were not affected. In the context of asthma, an increase in tryptophan metabolites is one of the mechanisms by which allergen-specific immunotherapy achieves immune tolerance.
Introduction
Asthma is a chronic airway disease characterized by eosinophilic and neutrophilic airway inflammation, airway hyperreactivity (AHR), and an increase in serum allergen-specific immunoglobulin E (IgE).1,Citation2 In asthma, there is an imbalance in the lungs between inflammatory and immunosuppressive T cells. T helper (Th)2 cells and related inflammatory cytokines are increased, while Th1 cells decreased.Citation1,Citation2 In general, Th1 are effector inflammatory cells; in the context of asthma, they could be immunomodulatory. In a similar manner, Th17 cells and their inflammatory cytokines are increased in asthma and regulatory T (Treg) cells, which mediate immunosuppression, are decreased.Citation3
Asthma is related to allergy, and allergen-specific immunotherapy (AIT) is used to desensitize the patient and promote immune tolerance. AIT is thought to act by reversing the allergen-caused changes in the balance between inflammation-producing and inflammation-inhibiting T cells.Citation4
A key event in AIT is the skewing of the Treg/Th17 balance toward Treg cells. AIT induces Treg cells, and these cells can inhibit the Th2-related airway inflammation seen in asthma.Citation5,Citation6 Th17 cells, which secrete interleukin (IL)-17, inhibit the production of Treg cells and reverse the immune tolerance produced by AIT.Citation7 Treg and Th17 cells are derived from the same precursor cells,Citation2 and increasing the action of indoleamine 2,3 dioxygenase (IDO), a rate-limiting enzyme in the catabolism of tryptophan, can switch the differentiation of these precursor T cells into Treg cells rather than Th17 cells.Citation8
The action of IDO is thought to be due to the metabolites it produces.Citation8,Citation9 The presentation of antigens to pulmonary dendritic cells (DCs) increases the activity of the IDO contained in these cells. An increased number of tryptophan metabolites then enter the intercellular space and shift the maturation of precursor T cells toward the Treg, rather than the Th17, lineage.Citation10-Citation12 The modulation mechanism of Th17-Treg differentiation has been reported and indicates that tryptophan metabolites induce the coding for the foxp3 gene (Treg) and suppresses retineic-acid-receptor-related orphan nuclear receptor gamma (RORγ)-encoding (Th17). Therefore, the catabolite of tryptophan promotes generation of the Foxp3+ Treg cells and inhibition of differentiation in RoRγt+ Th17 cells.Citation13 Furthermore, tryptophan degradation (also known as the kynurenines [KYN] pathway) participates in the immune regulation of Treg cells, upregulating expression of the foxp3 transcript factor and increasing Treg cell differentiation, accompanied by a decreased expansion of Th17 cells.Citation14 Likewise, another study demonstrated that dichloroacetate induces Treg cell differentiation and suppresses Th17 differentiation by inhibiting the pyruvate dehydrogenase kinase (PDHK) activity of recombinant PDHK and the phosphorylation of pyruvate dehydrogenase.Citation15 Taken together, the skewing mechanism of Th17-Treg differentiation is regulated by tryptophan catabolite via upregulated AHR expression and then decreased generation of Th17.
Overall, IDO-1 is broadly expressed in different tumor cells, inflammatory/antigen-presenting cells, and stromal cells, and activates the Kynurenine/tryptophan signaling pathway to increase differentiation of Treg cells.Citation16 However, the transitional IDO immunomodulation pathway has been found to contribute to the control of allergic inflammation. The nature of 1-methyl tryptophan (1-MT) in the IDO1 signaling pathway is exhibited by its eliciting the MHC-restricted T cell mediated rejection of hemiallogeneic antigens.Citation16 1-MT is a selective inhibitor of enzymatic activity of the aryl hydrocarbon receptor (AHR), which is used in immunotherapy trials and in the treatment of cancer.Citation17 On the other hand, 1-MT participates in initiation of melatonin biosynthesis in human fibroblasts/melanoma cells and induces the tryptophan hydroxylase and hydroxyindole O-methyltransferase mRNA in fibroblasts and melanocytes.Citation18 Altogether, 1-MT may be involved in inhibiting IDO via blocking AHR activity and initiating melatonin biosynthesis in skin cells.
It has been reported that KYN can induce Treg cells to generate and differentiate.Citation16 Meanwhile, IDO has been suggested to contribute to the maintenance of peripheral immune tolerance in autoimmune diseases and it has been established that IDO is expressed on the ring wall of granulomas to maintain the tolerogenic milieu of infectious granulomas.Citation19 Furthermore, KYN also affects other metabolism pathways, which correspond to kynureninase and kynurenine 3-monooxygenase (3-OH KYN), to modulate immunology/allergy function.Citation19 The effects of the various metabolites of the IDO pathway contribute to tolerance induction in the Th2-dependent allergic pathway.
The question asked in the current study was: which of the effects of AIT treatment of allergic asthma are caused by an increase in tryptophan metabolites? To answer this question, we created a mouse model of ovalbumin (OVA)-induced allergic asthma and AIT, then examined the effect of 1) inhibiting tryptophan metabolism (using the specific inhibitor 1-methyl tryptophan [1-MT]) and 2) adding a tryptophan metabolite in this model to the Treg/Th17 balance and to the various physiological and cellular effects of AIT.
Methods
Animals
Female BALB/c mice (6–8 weeks old, body weight 16–20 g) were purchased from Beijing HFK Bioscience Co., Ltd. (Beijing, China). The study was approved by the Ethics Committee of Southwest Hospital of the Third Military Medical University.
Asthma/immunotherapy model
Mice were divided into five groups (n = 6 for each): healthy control group, asthma group, the OVA-AIT (immunotherapy) group, OVA-AIT-1-MT group (immunotherapy + IPO inhibitor), and OVA-AIT-1-MT-KYN group (immunotherapy + tryptophan metabolite). In this study, we established an OVA-induced asthma model as described in , which was modified based on previous studies.Citation20,Citation21 Saline was substituted for OVA in the control group in all procedures. On day 41, eosinophilic airway inflammation was found, with typical features reminiscent of human allergic asthma.Citation22 The following were used to validate the model: (a) AHR, (b) histological observation of lung inflammation; (c) bronchoalveolar lavage fluid (BALF) cell counts and concentrations of IL-5, IL-6, IL-10, IL-17, and interferon gamma (IFNγ), and (d) measurement of OVA-specific IgE in serum.
Figure 1. Schematic showing the study design and immunotherapy model. Mice were sensitized with ovalbumin (OVA) (10 μg) on day 7, then given 1-MT and kynurenine (KYN) intraperitoneal (i.p.) before a boost with OVA on the indicated day (1 μg). Mice were sacrificed on the day after the last challenge with 10% OVA.
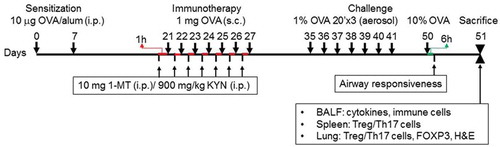
Establishment of asthma
Ten μg of OVA and 2.25 mg of Al(OH)3 were dissolved in 100 μl of aseptic normal saline. Sensitization in the asthma and OVA-AIT groups was performed by intraperitoneal (IP) injection of OVA at days 0 and 7.
Immunotherapy
Immunotherapy was given to the OVA-AIT group by subcutaneous injection of high-dose OVA on days 21 to 27. One mg of OVA and 2 mg Al(OH)3 in 200 µl of normal saline was used for these injections. This dose was selected after a pilot study showed that this amount did not cause a lethal allergic reaction.Citation20,Citation21
OVA challenge
OVA challenge was performed in the control, asthma, and OVA-AIT groups by inhalation of 1% OVA aerosol on days 35 to 41, and inhalation of 10% OVA aerosol on day 50.
OVA-AIT-1-MIT group
Mice were treated as in the OVA-AIT group except that, on days 21 to 27, they received an IP injection of 1-MT (10 mg/1 ml phosphate-buffered saline [PBS]),Citation20 an inhibitor of IDO-induced tryptophan metabolism, 1 h before each immunotherapy injection of OVA. In the current study, we focused on the immunomodulation function of the tryptophan metabolite kynurenine and we utilized the specific inhibitor 1-MT to validate the effects of tryptophan in the regulation of the Th17-Treg axis.
OVA-AIT-1-MT-KYN group
Mice were treated as in the OVA-AIT-1MT group except that, on days 21 to 27, they also received an IP injection of KYN (900 mg/kg),Citation20 a tryptophan metabolite, 1 h before each immunotherapy injection of OVA. In this study, 1-MT and KYN does not need to be administered continuously. Because we mainly observed the effect of allergen immunotherapy by giving the specific inhibitor 1-MT and/or KYN, further investigation may be needed of the effect of 1-MT and KYN during sensitization and/or challenge, to reflect clinical conditions.
Airway hyperresponsiveness
Airway responsiveness was determined within 6 h after the final OVA challenge on day 50. Spontaneous respiration was measured by plethysmography. Mice received 3 min inhalations of methacholine at different concentrations (3.125, 12.5, 50 mg/mL). At 2 min after each inhalation, plethysmography was done for 5 min, and the mean “enhanced pause” (Pench) value obtained. The following equation was used for Pench: Pench = (peak expiratory flow/peak inspiratory flow)*pause. An airway responsiveness curve was constructed for each methacholine concentration.
Bronchoalveolar lavage fluid (BALF)
Twenty-four hours after the last OVA challenge, mice were anesthetized IP with 1.5% pentobarbital sodium, and orbital blood was collected and stored at −80ºC. Next, 1 ml PBS was infused into the trachea and then retrieved using a syringe, a process which was repeated 5 times. BALF was collected (80% of the fluid was retrieved), centrifuged at 4ºC for 10 min at 1500 r/min, the supernatant harvested and stored at −80ºC, and the cells re-suspended for cell counting. BALF leukocytes were counted using a hemocytometer.
Lung histology
After sacrifice, thoracotomy was performed and lung tissues were collected and fixed in 4% paraformaldehyde. After dehydration, lung tissues were embedded in paraffin, sectioned, and processed for hematoxylin and eosin staining. Sections were observed under a light microscope. The degree of inflammation in the bronchus, bronchioles, blood vessels, and alveoli was then assessed.
Enzyme-linked immunosorbent assay
Serum OVA-specific IgE and cytokines of BALF (IFNγ, IL-10, IL-17A, IL-5, and IL-6) were measured using enzyme-linked immunosorbent assay kits.
Detection of Treg and Th17 cells in the spleen and lung
The spleen was collected under aseptic conditions, ground, and filtered through a 40 µM mesh. Cells were harvested after washing twice with cold RPMI-1640. After the addition of erythrocyte lysis buffer, cells were centrifuged at 500 g for 2 min, and the supernatant removed. The cells were re-suspended with RPMI-1640 (Hyclone; GE Healthcare Life Sciences, Logan, UT, USA) and incubated at 37ºC in an environment with 5% CO2 for 12 h. Splenic and lung cells were incubated for 12 h in phorbol 12-myristate 13-acetate (PMA), based on a method previously reported.Citation23 Park et al. demonstrated that 14-hour incubation improved the recovery of lung cells and did not significantly affect the expression of the assayed markers on lung, mesenteric lymph nodes, spleen lymphocytes, and DCs.Citation23
Lung tissues were ground and digested in 3 mg/ml collagenase D at 37°C for 2 h. The resultant cells were re-suspended in RPMI-1640 and the cell suspension was filtered through a 70 μm mesh. After the addition of erythrocyte lysis buffer, the cell suspension was incubated at 37°C for 12 h in an environment with 5% CO2. The supernatant was removed and cells were re-suspended.
Cells were treated with ionomycin (500 mg/ml; Sigma-Aldrich Corp., St. Louis, MO, USA) in the presence of brefeldin A (10 µg/ml; Bioscience Inc., San Diego, CA, USA), and then with PMA (50 ng/ml, Sigma-Aldrich Corp., St. Louis, MO, USA) for 5 h. After centrifugation, the supernatant was removed and the cells were re-suspended with RPMI-1640 containing 10% PBS, followed by the addition of FITC-labeled anti-mouse CD4 antibody and FcR-Block (eBioscience Inc., San Diego, CA, USA). After incubation at 4ºC for 30 min, cells were washed in OBS, then fixed and permeabilized. Following the addition of Foxp3-PE (a Treg marker) antibody and PE-conjugated RORγt (a Th17 marker) antibody, incubation was done for 30 min at 4ºC, followed by flow cytometry according to the manufacturer’s instructions.
Detection of transcription factors in the lung by quantitative polymerase chain reaction (PCR)
Remaining lung tissues were processed for extraction of total RNA with the RNAzol® reagent. Reverse transcription was done with oligo (dT18) primers. Quantitative reverse transcription polymerase chain reaction (PCR) was performed with the ABI Step One Plus System (Applied Biosystems, Waltham, MA, USA) and QuantiFast SYBR Green PCR Kit (QIAGEN, Hilden, Germany). mRNA expression was determined with the 2−△△Ct method with β-actin as an internal control. The mRNA expression of target genes in treated mice was normalized to that in untreated mice (control group). The primer and probe sequences were: Foxp3 (forward, 5ʹ-GGCCCTTCTCCAGGACAGA-3ʹ; reverse, 5ʹ-GGC-ATGGGCATCCACAGT-3ʹ); RORγt (forward, 5ʹ-TGCGACTG-GAGGACCTTCTA-3ʹ; reverse, 5ʹ-TCACCTCCTCCCGTGA-AAAG −3ʹ); and β-actin (forward, 5ʹ-GGCTGTATTCCCCTCC-ATCG-3ʹ; reverse, 5ʹ-CCAGTTGGTAACAATGCCATGT-3ʹ). The PCR protocol consisted of maintaining the mixture at 95°C for 5 min, followed by 40 cycles of amplification at 95°C for 15 seconds and at 58°C for 1 min. In each run, the templates were assayed in triplicate; for the majority of samples, each run was repeated at least twice.
Statistical analysis
Continuous variables were presented as means and standard deviations (SDs). Comparisons between groups were performed using one-way ANOVA with the Bonferroni adjustment method for the post-hoc test. Comparisons between different doses of methacholine were performed by repeated measurement of analyses of the variable (ANOVA). Statistical analyses were performed using IBM SPSS statistical software version 22 for Windows (IBM Corp., Armonk, New York, USA). Two-tailed P < .05 indicated statistical significance.
Results
Tryptophan metabolites reduce OVA-specific IgE and inflammatory cytokines in an AIT-suppressed asthma model
Levels of the three pro-inflammatory mediators IL-5, IL-6, and IL-17A were more than doubled in the BALF of asthmatic mice and were significantly decreased, but not to control levels, in the immunotherapy group (). The anti-inflammatory mediator IL-10 was decreased in asthmatic mice, a decrease that was completely blocked by immunotherapy. However, immunotherapy had no effect on the increase in IFNγ seen in asthmatic mice (Supplementary Table 1). In addition, the increase in OVA-specific IgE seen in the serum of asthmatic mice was significantly, but not completely, blocked by immunotherapy ().
Table 1. Comparison of serum OVA-specific IgE and inflammatory cytokines in BALF between groups in high dose OVA AIT.
Furthermore, inhibition of tryptophan metabolism blocked the decrease in three inflammatory mediators (IL-5, IL-6, and IL-17) caused by immunotherapy (). The addition of the tryptophan metabolite KYN reversed this blockade (). Inhibition of tryptophan metabolism or addition of a tryptophan metabolite had no effect on the immunotherapy-induced increase in the anti-inflammatory mediator IL-10, or in the decrease in OVA-specific IgE ().
Tryptophan metabolites reduce immune cell count in an AIT-suppressed asthma model
Immunotherapy significantly decreased the asthma-induced elevated total cell, eosinophil, and lymphocyte numbers, but not to control levels. Neutrophil levels were slightly, but significantly, increased in asthmatic mice, but immunotherapy had no effect on these levels (). Blocking tryptophan metabolism significantly decreased the effect of immunotherapy on the total and eosinophil cell numbers. Adding the tryptophan metabolite KYN reversed the effect of blocking tryptophan metabolism. Blocking tryptophan metabolism or adding KYN had no effect on the immunotherapy-related decrease in lymphocytes. Neither immunotherapy nor changes in tryptophan metabolism altered the increased number of neutrophils seen in asthmatic mice ().
Table 2. Comparisons between groups in cell numbers of total, eosinophils, lymphocytes, and neutrophils in BALF.
Tryptophan metabolites regulate the Treg/Th17 balance in an AIT-suppressed asthma model
In both the spleen and lung, Treg cells were decreased and Th17 cells increased significantly in the asthma group compared to the control group. Immunotherapy partly, but significantly, reversed the asthma-induced changes (). In addition, levels of Treg mRNA were decreased and levels of Th17 mRNA were increased in asthmatic mice compared to controls, and these changes were also partly, but significantly, blocked by immunotherapy (). Furthermore, inhibition of tryptophan metabolism blocked, and the addition of KYN restored, the changes in Treg and Th17 cell number in the lung and spleen caused by immunotherapy ().
Table 3. Comparisons between groups in Treg cells and RORγt+ T cells of spleen and lung.
Table 4. Comparison between groups in mRNA-Foxp3/β-actin and mRNA-RORγt/β-actin of lung.
Tryptophan metabolites reduce lung inflammation and airway hyperreactivity in an AIT-suppressed asthma model
Asthma-induced lung inflammation was also decreased by immunotherapy (). After immunotherapy, the inflammation beside the bronchus and blood vessels was improved significantly. In asthmatic mice, eosinophils and neutrophils were found in the lung interstitium and alveolar space; also noted were mucus plug in the bronchial lumen, infiltration of inflammatory cells, congestion and edema of the vascular wall, and necrosis and shedding of epithelial cells in the bronchus ()). In addition, a mucus plug was seen in the bronchial lumen, inflammatory cells were noted in the bronchus, the vascular wall showed congestion and with edema, and necrosis and shedding of epithelial cells were observed. In the OVA-AIT group ()), inflammation was decreased compared to that seen in the asthma group. In the healthy control group, the bronchus, alveoli, and blood vessels were clear and no inflammation was present ()). In asthmatic mice with immune tolerance, the inflammation was attenuated significantly as compared to the asthma group, with much fewer infiltrated eosinophils and neutrophils ()). Inflammation was worse in the OVA-AIT-1-MT group than in the OVA-AIT group ()), but the inflammation in the OVA-AIT-1-MT-KYN group was markedly attenuated compared to the OVA-AIT group ()). Furthermore, asthma-induced AHR was significantly decreased by immunotherapy at all methacholine doses (). Changes in tryptophan metabolism had no effect on the immunotherapy-induced decrease in AHR ().
Figure 2. Representative histological images of the indoleamine 2,3 dioxygenase (IDO) inhibitor, 1-methyl tryptophan (1-MT), and the tryptophan metabolite, kynurenine (KYN), on the allergen-specific immunotherapy (AIT). Representative lung sections stained with hematoxylin and eosin (H&E) from the indicated group: (a) control, (b) treated with ovalbumin (OVA) plus AIT, (c) asthma, (d) stimulated with OVA + AIT then 1-MT, (e) stimulated with OVA + AIT, then 1-MT + KYN. Data represent mean ± SEM of 2 independent experiments with n ≥ 3 mice per experiment.
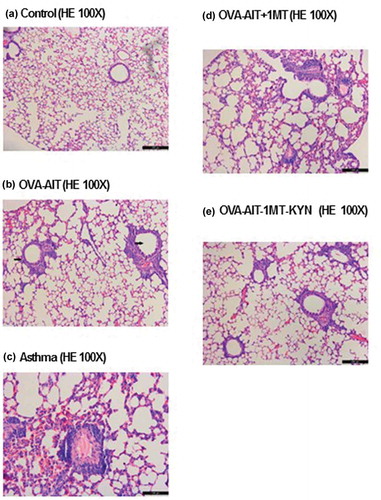
Discussion
In the current study, we found that tryptophan metabolism can reduce lung inflammation and attenuate airway hyperreactivity in an OVA-induced asthma mouse model. We demonstrated the main mechanism by which tryptophan metabolism affects immunomodulation function, by inducing Treg cell differentiation and reducing the expansion of Th17 cells through the KYN signal pathway. Meanwhile, Taber et al.Citation20 reported that inhibition of tryptophan metabolism during AIT reversed the AIT effect on eosinophilia and Th2 cytokines, but had no effect on AHR or OVA-specific IgE.Citation20 However, Taber did not examine the effect of tryptophan metabolites on AIT-induced changes in Treg/Th17 balance or on airway histology. In the present study, we clearly demonstrated that tryptophan metabolites generated by IPO during immunotherapy are crucial in the regulation of allergen-induced allergic airway Treg/Th17 balance in this mouse model. Our data appear to provide the first report connecting tryptophan metabolites to antigen-specific immunotherapy for asthma, providing direct evidence of a novel molecular mechanism of AIT through tryptophan metabolite-regulated Treg/Th17 balance.
On the other hand, the presentation of antigens to pulmonary dendritic cells (DC) increases the activity of the IDO contained in these cells.Citation11,Citation12 An increased number of tryptophan metabolites would then enter the intercellular space and shift the maturation of precursor T cells toward the Treg, rather than the Th17, lineage.Citation10-Citation12 Thus, our results imply that an allergen-DC might initiate an increase in IDO production of tryptophan metabolites as the mechanism partly responsible for the AIT-induced increase in Tregs, the decrease in airway inflammation, and the decrease in inflammatory mediators of Th2 and Th17. Although many studies have examined the IDO activation of Treg cells,Citation10-Citation12 most have not considered this issue in the context of allergen-specific immunotherapy for asthma.
The results leave questions, however, as to why IL-10, OVA-specific IgE, and AHR levels were not affected in the tryptophan metabolite-induced immunotherapy model and what mechanisms might underlie the AIT-induced decrease in AHR. In addition, tryptophan metabolites did not potentiate the reduction of IgE levels by means of suboptimal immunotherapy. These data demonstrate that immunotherapy differentially regulates the pathways leading to allergen-induced airway inflammation, increased serum IgE levels, and increases airway responsiveness, indicating the presence of multiple mechanisms. In addition, these data support earlier observations that allergen-specific IgE levels and the development of AHR can be dissociated from the induction of eosinophilic airway inflammation in mouse models.Citation24 Moreover, because blocking tryptophan metabolism inhibited the AIT-induced increase in Tregs, one would expect it also to inhibit the increase in the Treg-produced cytokine, IL-10, but it did not. However, IL-10 is produced by both activated Treg cells and activated regulatory B (Breg) cells during the induction of tolerance, and a Breg effect might mask any effect on Treg-produced IL-10. IgE release is inhibited by IL-10 combined with transforming growth factor beta (which we did not measure),Citation2 and this combined action may have obscured any tryptophan metabolite effect on IgE levels.
The mechanisms by which IDO-mediated tryptophan metabolites regulate the immunotherapy-induced suppression of eosinophilia and TH2 cytokines are not yet known. Because IL-10 levels were not changed after combined treatment with immunotherapy and IDO inhibitors, a shift from a TH2 to a TH1 response is probably not at play. Therefore, one or more subsets of regulatory T cells and Th17 cells are implicated, because we here demonstrated a role for IDO in the generation of these two cell types. Moreover, human studies clearly demonstrated that allergen immunotherapy is associated with an increased number of cytokine–producing Treg cells.Citation25-Citation27 However, the IDO-dependent effect of immunotherapy is selective for allergic inflammatory responses, but not for IgE and AHR, which suggests the presence of multiple regulatory mechanisms. Another explanation for the differential regulation of asthma manifestations by immunotherapy might be that each requires a different level of immunosuppression or is differentially dependent on a memory T-cell response.
The effect of IL-17 during induction of OVA-related asthma differs from its effect during AIT for this condition. The loss of Th17 cells blocks the development of AHR, but not that of airway inflammation.Citation28 Injecting an antibody to IL-17 during AIT blocks both the AIT-induced decrease in AHR and the AIT-induced decrease in eosinophilia.Citation7 IL-17 also has a direct effect on airway smooth muscle and increases its contractility by increasing myosin light chain kinase phosphorylation.Citation7,Citation29,Citation30 Of the other two mediators we examined whose influence on the AIT-induced amelioration of AHR should be studied further, IgE levels are known are to be correlated with AHR, airway eosinophilia, and airway inflammation in asthma. However, IgE is not required for these changes in AHR- or inflammation-related parameters, because these changes still occur after allergen challenge in IgE-deficient mice.Citation24 IL-10 is an essential part of the AIT pathway to decrease AHR, but mice treated with an IL-10 receptor blocker during AIT show no AIT-related decrease in AHR, and no decrease in IgE, eosinophils, or IL-5.Citation31
The fact that patients with allergic airway disease often demonstrate increased IgE, Th2-type cytokines, and eosinophilic inflammation makes it difficult to evaluate the interrelationship or importance of each factor in the induction of airway inflammation and AHR. Previous studies reported that pooling of data was possible for methacholine PC20 for three studies which showed a symptom (nonspecific AHR) score of 0.74 (95% CI 0.17, 1.66), showing no clear evidence of benefit for AIT.Citation28 However, subcutaneous immunotherapy, but not sublingual immunotherapy, improved quality of life and decreased allergen-specific AHR.Citation32 According to these studies and our data, we suggest that IPO-dependent AIT for allergic asthma may has a major effect in allergen-specific AHR, but not in nonspecific AHR. Certainly, whether tryptophan metabolites regulate allergen-specific AHR merits further investigation.
Despite the general success of currently available asthma drugs, there are several reasons to pursue new ones. However, to date, there is no effective preventive strategy for asthma or a known cure.Citation33,Citation34 Unfortunately, the effects of inhaled corticosteroids on asthma rapidly disappear when the drug is discontinued.Citation24 Moreover, current asthma drugs generally do not reverse or slow down most of the long-term remodeling changes that occur in various cell types in the airway.Citation35 Treg and Th17 cells correlated with the degree of asthmatic airway remodeling.Citation36 According to these findings, we suggest that tryptophan metabolites regulated Treg/Th17 balance may provide the clue in the treated-strategy of asthma patients in future.
In this study, we investigated the relationship of tryptophan metabolites and 1-MT/KYN in allergen immunotherapy. Future studies may need to further investigate the immunomodulation effects on 1-MT/KYN in sensitization or challenge stage in an OVA-induced asthma model or in asthma patients, prior to establishing relevant clinical trials.
Conclusion
Our results show AIT regulates the Treg/Th17 balance and decreases airway inflammation in mice with OVA-induced allergic asthma. Further, tryptophan metabolites regulating the Treg/Th17 balance is one of novel mechanisms involved in tolerance induction during AIT-suppressed airway inflammation. Moreover, these novel mechanisms could provide the basis for the development of new therapies in the treatment of asthma patients.
Disclosure of potential conflicts of interest
No potential conflicts of interest were disclosed.
Supplemental Material
Download MS Word (24.3 KB)Supplementary material
Supplemental data for this article can be accessed online at here.
Additional information
Funding
References
- Choy DF, Hart KM, Borthwick LA, Shikotra A, Nagarkar DR, Siddiqui S, Jia G, Ohri CM, Doran E, Vannella KA, et al. TH2 and TH17 inflammatory pathways are reciprocally regulated in asthma. Sci Trans Med. 2015;7:301ra129. doi:10.1126/scitranslmed.aab3142.
- Martin-Orozco E, Norte-Munoz M, Martinez-Garcia J. Regulatory T cells in allergy and asthma. Front Pediat. 2017;5:117. doi:10.3389/fped.2017.00117.
- Jiang H, Wu X, Zhu H, Xie Y, Tang S, Jiang Y. FOXP3(+)Treg/Th17 cell imbalance in lung tissues of mice with asthma. Int J Clin Exp Med. 2015;8:4158–63.
- Akdis CA, Akdis M. Mechanisms of allergen-specific immunotherapy ad immune tolerance to allergens. World Allergy Organ J. 2015;8:1–12. doi:10.1186/s40413-015-0063-2.
- Böhm L, Maxeiner J, Meyer-Martin H, Reuter S, Finotto S, Klein M, Schild H, Schmitt E, Bopp T, Taube C. Il-10 and regulatory T cells cooperate in allergen-specific immunotherapy to ameliorate allergic asthma. J Immunol. 2015;194:887–97. doi:10.4049/jimmunol.1401612.
- Hrusch CA, Tjota M, Sperling AI. The role of dendritic cells and monocytes in the maintenance and loss of respiratory tolerance. Curr Allergy Asthma Rep. 2015;15:494. doi:10.1007/s11882-014-0494-9.
- Kawakami H, Koya T, Kagamu H, Kimura Y, Sakamoto H, Yamabayashi C, Furukawa T, Sakagami T, Miyabayashi T, Hasegawa T, et al. IL-17 eliminates therapeutic effects of oral tolerance in murine airway inflammation. Clin Exp Allergy. 2012;42:946–57. doi:10.1111/j.1365-2222.2012.04006.x.
- Yan Y, Zhang GX, Gran B, Fallarino F, Yu S, Li H, Cullimore ML, Rostami A, Xu H. IDO upregulated regulatory T cells via tryptophan catabolite and suppresses encephalogenic T cell responses in experimental autoimmune encephalomyelitis. J Immunol. 2010;15:5953–61. doi:10.4049/jimmunol.1001628.
- Odemuyiwa SO, Ebeling C, Duta V, Abel M, Puttagunta L, Cravetchi O, Majaesic C, Vliagoftis H, Moqbel R. Tryptophan metabolites regulate mucosal sensitization to ovalbumin in respiratory airways. Allergy. 2009;64:488–92. doi:10.1111/j.1398-9995.2008.01809.x.
- Koch S, Mousset S, Graser A, Reppert S, Ubel C, Reinhardt C, Zimmermann T, Rieker R, Lehr HA, Finotto S. IL-6 activated BATF/IRF4 functions in lymphocytes are T-bet-independent and reversed by subcutaneous immunotherapy. Sci Rep. 2013;3:1754. doi:10.1038/srep01754.
- Sharma MD, Baban B, Chandler P, Hou DY, Singh N, Yagita H, Azuma M, Blazar BR, Mellor AL, Munn DH. Plasmacytoid dendritic cells from mouse tumor-draining lymph nodes directly activate mature Tregs via indoleamine. J Clin Invest. 2007;117:2570–82. doi:10.1172/JCI31911.
- Huang L, Baban B, Johnson BA, Mellor AL. Dendritic cells, indoleamine 2.3. dioxygenase and acquired immune privilege. Int Rev Immunol. 2010;29:133–55. doi:10.3109/08830180903349669.
- Romani L, Zelante T, De Luca A, Fallarino F, Puccetti P. IL-17 and therapeutic kynurenines in pathogenic inflammation to fungi. J Immunol. 2008;180(8):5157–62. doi:10.4049/jimmunol.180.8.5157.
- de Araújo EF, Feriotti C, Galdino NAL, Preite NW, Calich VLG, Loures FV. The IDO–ahR axis controls Th17/Treg immunity in a pulmonary model of fungal infection. Front Immunol. 2017;24:880. doi:10.3389/fimmu.2017.00880.
- Makita N, Ishiguro J, Suzuki K, Nara F. Dichloroacetate induces regulatory T-cell differentiation and suppresses Th17-cell differentiation by pyruvate dehydrogenase kinase-independent mechanism. J Pharm Pharmacol. 2017;69(1):43–51. doi:10.1111/jphp.2017.69.issue-1.
- Prendergast GC, Malachowski WP, DuHadaway JB, Muller AJ. Discovery of IDO1 inhibitors: from bench to bedside. Cancer Res. 2017;77(24):6795–811. doi:10.1158/0008-5472.CAN-17-2285.
- Lewis HC, Chinnadurai R, Bosinger SE, Galipeau J. The IDO inhibitor 1-methyl tryptophan activates the aryl hydrocarbon receptor response in mesenchymal stromal cells. Oncotarget. 2017;8(54):91914–27. doi:10.18632/oncotarget.20166.
- Moreno AC, Clara RO, Coimbra JB, Júlio AR, Albuquerque RC, Oliveira EM, Maria-Engler SS, Campa A. The expanding roles of 1-methyl-tryptophan (1-MT): in addition to inhibiting kynurenine production, 1-MT activates the synthesis of melatonin in skin cells. Febs J. 2013;280(19):4782–92. doi:10.1111/febs.12444.
- von Bubnoff D, Bieber T. The indoleamine 2,3-dioxygenase (IDO) pathway controls allergy. Allergy. 2012;67(6):718–25. doi:10.1111/j.1398-9995.2012.02830.x.
- Taher YA, Piavaux BJ, Gras R, van Esch BC, Hofman GA, Bloksma N, Henricks PA, van Oosterhout AJ. Indoleamine 2,3-dioxygenase-dependent metabolites contribute to tolerance induction during allergen immunotherapy in a mouse model. J Allergy Clin Immunol. 2008;121:983–91. doi:10.1016/j.jaci.2007.11.021.
- Hellings PW, Kasran A, Liu Z, Vandekerckhove P, Wuyts A, Overbergh L, Mathieu C, Ceuppens JL. Interleukin 17 orchestrates the granulocyte influx into airways after allergen inhalation in a mouse model of allergic asthma. Am J Resp Cell Molec Biol. 2003;28:42–50. doi:10.1165/rcmb.4832.
- Hellings PW, Hessel EM, Van Den Oord JJ, Kasran A, Van Hecke P, Ceuppens JL. Eosinophilic rhinitis accompanies the development of lower airway inflammation and hyper-reactivity in sensitized mice exposed to aerosolized allergen. Clin Exp Allergy. 2001;31(5):782–90. doi:10.1046/j.1365-2222.2001.01081.x.
- Park SY, Jing X, Gupta D, Dziarski R. Peptidoglycan recognition protein 1 enhances experimental asthma by promoting Th2 and Th17 and limiting regulatory T cell and plasmacytoid dendritic cell responses. J Immunol. 2013;190(7):3480–92. doi:10.4049/jimmunol.1202675.
- Mehlhop PD, van de Rijn M, Goldberg AB, Brewer JP, Kurup VP, Martin TR, Oettgen HC. Allergen-induced bronchial hyperreactivity and eosinophilic inflammation occur in the absence of IgE in a mouse model of asthma. Proc Natl Acad Sci U S A. 1997;94:1344–49. doi:10.1073/pnas.94.4.1344.
- Akdis CA, Blesken T, Akdis M, Wüthrich B, Blaser K. Role of interleukin 10 in specific immunotherapy. J Clin Invest. 1998;102:98–106. doi:10.1172/JCI2250.
- Francis JN, Till SJ, Durham SR. Induction of IL-101CD41CD251 T cells by grass pollen immunotherapy. J Allergy Clin Immunol. 2003;111:1255–61. doi:10.1067/mai.2003.1570.
- Jutel M, Akdis M, Budak F, Aebischer-Casaulta C, Wrzyszcz M, Blaser K, Akdis CA. IL-10 and TGF-beta cooperate in the regulatory T cell response to mucosal allergens in normal immunity and specific immunotherapy. Eur J Immunol. 2003;33:1205–14. doi:10.1002/eji.200322919.
- Pelaia G, Vatrella A, Busceti MT, Gallelli L, Calabrese C, Terracciano R, Maselli R. Cellular mechanisms underlying eosinophilic and neutrophilic airway inflammation in asthma. Mediatore Inflam. 2015;2015:879–783.
- Kudo M, Melton AC, Chen C, Engler MB, Huang KE, Ren X, Wang Y, Bernstein X, Li JT, Atabai K, et al. IL-17A produced by αβ T cells drives airway hyper-responsiveness in mice and enhances mouse and human airway smooth muscle contraction. Nat Med. 2012;18:546–54. doi:10.1038/nm.2684.
- Hirashara K, Nakayama T. CD4+T-cell subsets in inflammatory diseases: beyond the Th1/Th2 paradigm. Int Immunol. 2016;28:163–71. doi:10.1093/intimm/dxw006.
- Vissers JL, van Esch BC, Hofman GA, Kapsenberg ML, Weller FR, van Oosterhout AJ. Allergen immunotherapy induces a suppressive memory response mediated by IL-10 in a mouse asthma model. J Allergy Clin Immunol. 2004;1113:1204–10. doi:10.1016/j.jaci.2004.02.041.
- Dhami S, Kakourou A, Asamoah F, Agache I, Lau S, Jutel M, Muraro A, Roberts G, Akdis CA, Bonini M, et al. Allergen immunotherapy for allergic asthma: A systematic review and meta-analysis. Allergy. 2017;72(12):1825–48. doi:10.1111/all.2017.72.issue-12.
- Martinez FD. New insights into the natural history of asthma: primary prevention on the horizon. J Allergy Clin Immunol. 2011;128:939–45. doi:10.1016/j.jaci.2011.09.020.
- Guilbert TW, Morgan WJ, Zeiger RS, Mauger DT, Boehmer SJ, Szefler SJ, Bacharier LB, Lemanske RF, Strunk RC, Allen DB, et al. Long-term inhaled corticosteroids in preschool children at high risk for asthma. N Engl J Med. 2006;354:1985–97. doi:10.1056/NEJMoa051378.
- Warner SM, Knight DA. Airway modeling and remodeling in the pathogenesis of asthma. Curr Opin Allergy Clin Immunol. 2008;8:44–48. doi:10.1097/ACI.0b013e3282f3b5cb.
- Lou CY, Li M, Li L. Dynamic changes in percentages of CD4⁺ CD25⁺ regulatory T cells and Th17 cells in process of airway remodeling in mouse model of asthma. Zhongguo Dang Dai Er Ke Za Zhi. 2015;17:994–1000.