ABSTRACT
With more demand for Sabin inactivated poliovirus vaccines (sIPVs) to support the global polio eradication effort worldwide, data regarding the potency characteristics of sIPV after exposure to freezing temperatures are urgently required. In the present study, the sIPVs were stored at −20°C for 24 h, 1 week, and 2 weeks in the freezer or in a vaccine carrier for 1 or 3 freeze-thaw cycle to evaluate the effect mediated by freezing temperatures that may be encountered during routine storage and transfer. The in vitro potency was then determined by a D-antigen enzyme-linked immunosorbent assay, and the in vivo potency was evaluated in Wistar rats. In the in vitro study for freezer storage groups, the D-antigen contents for all three types decreased and were lower than the release specifications after storing at −20°C for 2 weeks. After storing at −20°C for 1 week, the D-antigen contents for types I and III in combined group of a total of 45 vials, and for type II in the specific lot groups containing 15 vials decreased, but were within the release specifications. Moreover, no significant change in in vivo potency was observed. For vaccine carrier transfer groups, the D-antigen contents did not decrease after 1 freeze-thaw cycle; in contrast, it decreased, but no significant in vivo potency loss was observed after 3 freeze-thaw cycles. These results suggest that it may be possible to retain sufficient sIPV potency after short periods of freezing or freeze-thawing during transport.
Introduction
The use of the inactivated poliovirus vaccine (IPV) is recommended by the World Health Organization (WHO) Strategic Advisory Group of Experts in the polio end game strategy to eradicate all forms of polio.Citation1,Citation2 In May 2015, the 66th World Health Assembly endorsed the “Global Polio Eradication & Endgame Strategic Plan”, requiring the introduction of at least 1 dose of the IPV along with bOPV into routine immunization by October 2015 with a switch to the full use of the IPV by 2018 to complete the eradication of all wild, vaccine-related and Sabin polioviruses; the goal of this strategy is that no child will ever again suffer paralytic poliomyelitis.Citation3 However, ensuring a sufficient supply of the IPV is challenging for national health authorities and manufacturers. To produce IPV more safely, the WHO recommend that manufacturers develop IPV made from Sabin strains (sIPV) rather than the usual wild-type strains (wIPV). In 2015, the first sIPV made by the Institute of Medical Biology, Chinese Academy of Medical Sciences (IMBCAMS) was successfully licensed in China. Currently, IMBCAMS is preparing to achieve WHO prequalification of the sIPV, after which the vaccine will be supplied to developing countries via the United Nations International Children’s Emergency Fund (UNICEF).
The IPV is freeze-sensitive and should be stored at 2–8°C. According to WHO recommendations, the IPV should be distributed under cold chain conditions of refrigeration without freezing to maintain its potency.Citation4 However, during cold chain storage or transport, the vaccine may be exposed to nonoptimal or even extreme conditions, especially on the way to some remote peripheral vaccination sites in developing countries. Previous study analyzed 41 articles including up to 440 storage units and up to 103 shipments for vaccines have suggested that the percentage of vaccine exposing to freezing temperatures were 33.0% and 37.1% during storage, and were 38.0% to 19.3% during transportation in higher and lower income counties, respectively.Citation5 In some cases, 100% of the vaccine is exposed at least once to freezing temperatures,Citation5–Citation8 multiple freeze–thaw cycles in cold chains, or subzero temperatures for hundreds of hours during the distribution process.Citation9 Thus, manufacturers must clearly state on the package insert that vaccines incidentally exposed to freezing temperatures should be discarded. Unfortunately, only a few studies have evaluated the effects of freezing temperatures on the vaccines, especially for IPV.Citation10,Citation11 Recently, White et al. evaluated the system potency of wIPV after exposure to freezing temperatures, mimicking what a vaccine vial might encounter during transfer and storage.Citation12 As a new IPV with an important role in polio eradication worldwide, determining the potency of the sIPV after exposure to freezing temperatures is urgently required.
In the present study, for the first time, the sIPV was exposed to freezing temperatures to mimic the possible freezing conditions that occur during transportation and storage worldwide. Vaccine potency was tested to obtain primary data on freezing sensitivity based on a risk assessment, which will provide reference directions for future vaccine usage under the conditions of inadvertent accidents.
Materials and methods
Test groups
Three lots of the sIPV (lot#20151019, 20151018, and 20151222) were randomly selected and subjected to different temperature conditions that mimic the cold chain distribution of the sIPV. The lots were divided into 6 groups (15 vials per lot were assigned to each group; 45 total vials were allocated per combined group): 5 test groups subjected to different treatments, and there was 1 control group. Of these groups, 3 were designed to mimic freezing during storage: group 1 was placed in the freezer at −20°C for 1 day, group 2 was placed at −20°C for 1 week, and group 3 was placed at −20°C for 2 weeks. For the remaining 2 groups, freezing during transportation was mimicked.Citation13 Vials in group 4 were placed in direct contact with unconditioned ice packs (ice packs frozen at −20°C for 24 h and then immediately placed in a vaccine carrier) in a vaccine carrier for 8 h at room temperature. The vaccines were removed after 8 h, visually inspected for evidence of freezing, and then stored at 2–8°C for 16 h (1 freeze-thaw cycle). Group 5 was treated with the same conditions as group 4 for a total of three times performed on three consecutive days (3 freezing-thaw cycles). Group 6 was stored at 2–8°C and served as the untreated control ().
Table 1. The treatments mimicking freezing conditions during storage and transport for lot 20151019, lot 20151018, and lot 20151222 in vitro study.
Based on the above in vitro results, 2 additional lots of the sIPV (lot#20160709 and 20160711) were used to determine the freezing points for evaluating in vivo potency by randomly dividing the samples into 4 groups. Group 7 was placed in a freezer at −20°C for 1 day, group 8 was placed at −20°C for 1 week, group 9 was treated the same as group 5 (3 freeze-thaw cycles), and group 10 was stored at 2–8°C, serving as the untreated control (). Each group contained 30 vials from the two lots. The samples in each group were completely mixed together (liquid mixing) after treatment, and the D-antigen content in vitro was evaluated, and in vivo potency tests were performed.
Table 2. Treatments mimicking freezing conditions during storage and transport for lot 20160709 and lot 20160711 in the in vivo study.
Vaccines
Five commercial lots (lot# 20151019, 20151018, 20151222, 20160709, and 20160711) of the sIPV produced by IMBCAMS were randomly selected. Briefly, Vero cells were cultured on a microcarrier in a 550-L bioreactor, and three types of poliovirus working seeds (type I: Sabin SO+3; type II: Sabin SO+3; type III: Pfizer.RSO3) were inoculated into the microcarrier and incubated at 33°C for 48–96 h in the bioreactors. The virus suspension was harvested, ultrafiltered, purified by chromatography, and inactivated by formalin. The product was formulated to contain 30, 32, and 45 D-antigen units (DU)/dose (0.5 mL) for types I, II, and III, respectively.Citation14
Freezing point determination
The cooling curve method was applied to measure the freezing point of the sIPV by using a LyoStar II freezer dryer (manufactured by SP Scientific, Warminster, PA, USA) according to the manufacturer’s protocol. Two lots of the sIPV were used to measure the freezing point before treatment by inserting the 4 temperature probes of the freezer dryer into 4 vials of each lot, which was followed by placing the samples in the lyophilizer with the shelf temperature preset to −30°C. The first inflection point of temperature observed during the increase in the cooling curve was defined as the freezing point based on the temperature recorded during the freezing process.
Visual inspection and temperature monitoring
Throughout the different treatments, the actual temperatures were monitored by electronic temperature recorders (). Each sample vial in the different groups was stored at 2–8°C after visual inspection to identify the freezing status.
D-antigen assay in vitro studies
The D-antigen assay was performed for each sample by enzyme-linked immunosorbent assay, followed by calculation of the D-antigen units with a four-parameter logistic equation relative to the in-house standard.Citation14 The release specifications for D-antigen content in the final product were ≥48/54/82 DU/mL for types I, II, and III, respectively, according to the quality control standards of IMBCAMS.
Potency assay in vivo studies
Immunogenicity was evaluated as described below. Adult Wistar rats of both sexes weighing 180–200 g were purchased from the Institute of Laboratory Animal Sciences, Chinese Academy of Medical Sciences (CAMS). Animals were housed at the CAMS in a Biohazard level one animal facility under specific pathogen-free conditions. All animal experiments were performed in compliance with relevant laws and institutional guidelines and in accordance with the ethical standards of the Declaration of Helsinki. The rats were randomly divided into 4 groups (10 rats in each group) and administered 3 doses of 0.5 mL of vaccine at days 0, 22, and 43 via injection into the bilateral hind limb muscles (0.25 mL in each limb). Blood samples were collected at days 0 (before injection), 21, 42, and 64 (post inoculation) for evaluation in a neutralization assay ().
The neutralizing antibody titers against all 3 types of poliovirus were measured by a standard microneutralization assay in 96-well plates according to WHO recommendations.Citation15,Citation16 An antibody titer of ≥1:4 was considered as seroconversion for serum-negative rats before immunization, whereas a 4-fold increase in the antibody level was considered as seroconversion for serum-positive rats before immunization.
Statistical analysis
Statistical analyzes were performed using the SPSS 22.0 statistical package (SPSS, Inc., Chicago, IL, USA). The D-antigen contents and antibody titers of the samples in different groups were compared by the analysis of variance test, which was followed by further multiple comparisons between the different test and control groups with the Dunnett method. A p-value <0.05 was considered to indicate statistical significance. Furthermore, analysis of covariance was performed. Different lots were treated as covariant factors, followed by further statistical analysis with multiple comparisons between the treatment and control groups via the Bonferroni correction method.
The seroprotection rate was defined as the percentage of each group with titers ≥1:4. Viral neutralizing antibody titers were transformed into log2 titers to calculate geometric mean titers and 95% confidence intervals (CIs), with an individual neutralizing antibody titer lower than the detection limit (i.e., 1:4) given an arbitrary value of 1:1.
Results
Three lots of the sIPVwere randomly selected and subjected to different temperature situations that mimic the cold chain distribution of the sIPV. The lots were divided into 6 groups (test groups 1–5 and control group 6), and the D-antigen assay was performed for each sample via enzyme-linked immunosorbent assay to determine the in vitro potency of the sIPV (). Evaluation of the in vitro potency demonstrated that the D-antigen contents of the sIPVs placed in the freezer at −20°C for 1 day and 1 week, mimicking freezing during storage, and for 3 freeze-thaw cycles, mimicking freezing during transport, were decreased but remained in compliance with the specification; subsequently, an additional 2 sIPV lots (lot# 20160709 and 20160711) were selected by randomly dividing them into 4 groups (test groups 7–9 and control group 10) to evaluate the in vivo sIPV potency in Wistar rats (). For limited samples in each group (15 vials/lot), a combined group, consisting of lots 20151019, 20151018, and 20151222 and totaling 45 vials, was analyzed for in vitro D antigen content detection. Similarly, a combined group, consisting of lots 20160709 and 20160711 and totaling 30 vials, was analyzed via an in vivo immunogenicity test.
sIPV freezing point
As shown in the temperature curves in , the average freezing points were −15.9°C for lot 20160709 and −12.5°C for lot 20160711.
Visual inspection and temperature monitoring in different treatment groups
The temperature was monitored for both in vitro and in vivo potency tests in all 10 groups. All samples were completely frozen in the groups stored at −20°C. Some vials stored in the vaccine carrier were partially frozen, whereas some were not frozen ().
Table 3. Temperature fluctuations and visual inspection of different treatments.
For group 4, the temperatures rapidly decreased to below 0°C and were maintained for 3.5–6 h ()). The lowest temperature reached −14.0°C, and the temperature was maintained at below 0°C for 8 h during each cycle in group 5 ()).
Figure 4. Temperature monitoring results in a vaccine carrier with ice packs.
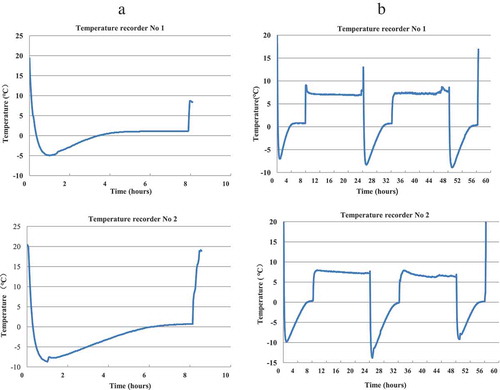
D-antigen content
D-antigen contents of four groups under conditions mimicking freezing during storage
The D-antigen contents differed for different types of poliovirus following different treatments when mimicking freezing storing at −20°C (). For all of the samples stored at −20°C for 2 weeks, the D-antigen contents for types I, II and III decreased in both the combined group and the groups consisting of each lot. Decreases of different types in different groups of samples stored at −20°C for 1 day and 1 week were also investigated. For type I, the D-antigen contents decreased after storing at −20°C for 1 week in the combined group and in the lot 20151222 and lot 20151018 groups ( and )) but did not decrease in the lot 20151019 group ()). After 1 day of freezing, the D-antigen contents decreased for type I only in the lot 20151019 group ()). For type II, no significant differences were observed in the combined groups after storing at −20°C for 1 day and 1 week ()), but the decreases were observed for different lots after either one day or one week of freezing ()). For type III, the D-antigen contents in all 3 lot groups were significantly reduced after storing at −20°C for 1 week. However, after 1 day of freezing treatment, the D-antigen contents decreased in the combined group and in the lot 20151019 and lot 20151018 groups (, and )) but not in the lot 20151222 group ()).
Figure 5. Changes in the D-antigen contents of the sIPV after different −20°C treatments.
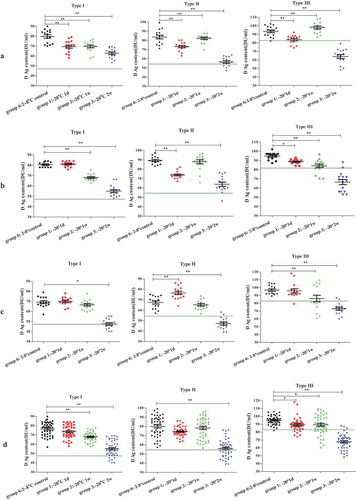
D-antigen contents for types I, II and III in the combined group and the 3 lot-specific groups after 1 day and 1 week of freezing were higher than the release specifications. However, when the results after 2 weeks of freezing were compared, the D-antigen content of type I was lower than 48 DU/mL, with an average mean vale of 47.5 DU/mL (95% CI: 44.8–50.3 DU/mL), in the lot 20151019 group, and the D-antigen content for type II was lower than 54 DU/mL, with average mean vale of 47.3 DU/mL (95% CI: 44.1–50.5 DU/mL), in the lot 20151019 group. For type III, the combined group and the lot 20151222, lot 20151018, and lot 20151019 groups showed decreases to below 82 DU/mL after freezing for 2 weeks, with values of 67.8 DU/mL (95% CI: 64.9–70.7 DU/mL), 63.9 DU/mL (95% CI: 59.1–68.8 DU/mL), 66.5 DU/mL (95% CI: 60.6–72.5 DU/mL), and 72.9 DU/mL (95% CI: 68.7–77.0 DU/mL), respectively.
D-antigen contents of 2 groups under conditions mimicking freezing during transportation
Significant losses in D-antigen contents for all 3 types were observed after 3 freeze-thaw cycles in the combined group and in each lot group except for type III in lot 20151019 (), while values decreased to below the release specifications only for type III in the lot 20151222 and lot 20151018 groups, with values of 76.5 DU/mL (95% CI: 73.6–76.9 DU/mL) and 79.3 DU/mL (95% CI: 76.0–82.5 DU/mL), respectively. For 1 freeze-thaw cycle, there were no decreases in the combined group for any of the 3 types and no decreases in any of the 3 lot groups for type II; however, the decreases were significant for types I and III in lot 20151222 and lot 20151018 but not in lot 20151019. However, all mean D-antigen contents were within the release specifications.
Immunogenicity assay
Based on the above in vitro potency test, two additional lots, 20160709 and 20160711, were randomly selected for immunogenicity assays and an in vivo potency test. The D-antigen contents in each group determined after storing at −20°C for 1 day and 1 week and after mimicking a vaccine carrier for 3 freeze-thaw cycles are shown in . The immunogenicity assay demonstrated that the neutralizing antibody titers for the 3 serotypes before immunization were all <1:4 and that the seroprotection rate reached 100% after 3 doses of immunization. There was no significant difference in the geometric mean titers among groups 8–9 compared to control group 10 ().
Table 4. D-antigen contents for types I, II and III in each group.
Discussion
With the progress of global polio eradication, the sIPV is required to meet the complete immunization programs needs for children both in China and around the world, especially in developing countries. During cold chain storage or transport, inadvertent freezing and freeze-thaw cycles are likely to occur. To evaluate the potential influence of these conditions on the sIPV potency, the present study considers, for the first time, sIPV vial samples that were treated with different freeze-exposure conditions that may occur during vaccine storage and transportation worldwide.
The results showed that the D-antigen contents were decreased from 2.1% to 11.0% for the 3 types of sIPV after storage at −20°C for 1 day and 1 week. In addition, there were more obvious decreases (approximately 30%) in the D-antigen contents in all samples stored at −20°C for 2 weeks, with some decreasing to below the release specifications. Both the combined group and lot-specific groups showed decreases for type III, while some lot groups showed decreases for either type I or II. In a recent collaborative study to establish the first international standard for the sIPV, unstable D-antigen contents in vials placed at −20°C were observed in two potential candidate sIPV international standard samples.Citation13 After being stored at −20°C overnight, the D-antigen contents of 17/234 candidate samples were decreased by 3.5% to 7.6% and were decreased by 29.9% to 60.2% after being stored at −20°C for 2 weeks.Citation17,Citation18
These results indicate that the stability of the sIPV at freezing is similar to that of the wIPV, featuring the same sensitivity of inactivated polio characteristics with a significant decrease in D-antigens.Citation12 As observed with the potency of the wIPV, which remained qualified after exposure at −20°C for ≤7 days,Citation12 sIPVs exposed to freezing conditions at −20°C for 1 day or 1 week exhibited no significant reduction in immunogenicity in the in vivo analysis in the present study.
For the test groups exposed to unconditioned ice packs in the vaccine carrier to mimic the transportation conditions, the significant decrease in the sIPV D-antigen content was unstable after 3 freeze-thaw cycles. Similar to the freezing storage results, the in vivo test showed no significant decrease in antibody titers. These findings are similar to those obtained in a study of the wIPV, which confirmed that both IPOL and VeroPol exposed to freezing conditions in vaccine carriers during transportation had no decrease in in vivo potency.Citation12 Thus, the evaluation of both freezing storage conditions and conditions mimicking those of transport suggested that the potency of sIPV manufactured by IMBCAMS would remain qualified after freezing at −20°C for no more than 1 week and after maintenance in the vaccine carrier for 3 freeze-thaw cycles.
In this study, limitations in the paragraph for clarity to the reader, only 3 lots of the sIPV and 15 vial samples per each test group were used for the D-antigen assay, which may have led to variations in statistical analysis. For example, in the samples stored at −20°C for one day, the D-antigen content was significantly decreased in the type I lot 20151019, whereas there was no significant difference was found for either lot 20151018 or 20151222.
These results suggest that the sIPV may retain sufficient potency after short periods of freezing or freeze-thawing during transport. Clinical studies are needed to further investigate the effects of these storage conditions. Moreover, a larger number of sIPV lots and more vial samples per lot should be evaluated to confirm the sIPV freezing stability and to compare the consistency of freezing stability among lots.
Disclosure of potential conflicts of interest
No potential conflicts of interest are disclosed.
Acknowledgments
The authors would like to thank the Program for Appropriate Technology in Health (PATH) for providing suggestions on the treatment design in the early stages of this study and for providing the vaccine carrier (WHO-approved, model # AVC-46) with ice packs (WHO-approved 0.6-L packs).
Additional information
Funding
References
- WHO. tOPV with bOPV – briefing note February; 2015.
- Liu Y, Wang J, Liu S, Du J, Wang L, Gu W, Xu Y, Zuo S, Xu E, An Z, et al. Introduction of inactivated poliovirus vaccine leading into the polio eradication endgame strategic plan; Hangzhou, China, 2010–2014. Vaccine. 2017;35:1281–86. doi:10.1016/j.vaccine.2017.01.034.
- Nicoletta PRHT, Tallis G, Jafari HS. World Health Organization guidelines for containment of poliovirus following type-specific polio eradication — worldwide. MMWR Morb Mortal Wkly Rep 2015; 2015.
- WHO. Polio vaccines: WHO position paper; 2016:145–68. doi:10.1293/tox.24.223e.
- Hanson CM, George AM, Sawadogo A, Schreiber B. Is freezing in the vaccine cold chain an ongoing issue? A literature review. Vaccine. 2017;35:2127–33. doi:10.1016/j.vaccine.2016.09.070.
- Matthias DM, Robertson J, Garrison MM, Newland S, Nelson C. Freezing temperatures in the vaccine cold chain: a systematic literature review. Vaccine. 2007;25:3980–86. doi:10.1016/j.vaccine.2007.02.052.
- Poy A, van den Ent M, Sosler S, AR H, Brown S, Sodha S, Ehlman DC, Wallace AS, Mihigo R. Monitoring results in routine immunization: development of routine immunization dashboard in selected African Countries in the context of the polio eradication endgame strategic plan. J Infect Dis. 2017;216:S226–S36. doi:10.1093/infdis/jiw635.
- Das MK, Arora NK, Mathew T, Vyas B, Sindhu M, Yadav A. Temperature integrity and exposure to freezing temperature during vaccine transfer under the universal immunization program in Three States of India. Indian J Public Health. 2019;63:139–42. doi:10.4103/ijph.IJPH_123_18.
- Yakum MN, Ateudjieu J, Pelagie FR, Walter EA, Watcho P. Factors associated with the exposure of vaccines to adverse temperature conditions: the case of North West region, Cameroon. BMC Res Notes. 2015;8:277. doi:10.1186/s13104-015-1257-y.
- Fortpied J, Wauters F, Rochart C, Hermand P, Hoet B, Moniotte N, Vojtek I. Stability of an aluminum salt-adjuvanted protein D-conjugated pneumococcal vaccine after exposure to subzero temperatures. Hum Vaccin Immunother. 2018;14:1243–50. doi:10.1080/21645515.2017.1421878.
- Chen D, Tyagi A, Carpenter J, Perkins S, Sylvester D, Guy M, Kristensen DD, Jones Braun L. Characterization of the freeze sensitivity of a hepatitis B vaccine. Hum Vaccin. 2009;5:26–32. doi:10.4161/hv.5.1.6494.
- White JA, Estrada M, Weldon WC, Chumakov K, Kouiavskaia D, Fournier-Caruana J, Stevens E, Gary HE, Maes EF, Oberste MS, et al. Assessing the potency and immunogenicity of inactivated poliovirus vaccine after exposure to freezing temperatures. Biol J Int Assoc Biol Stand. 2018;53:30–38. doi:10.1016/j.biologicals.2018.03.002.
- WHO. Module 2: The vaccine cold chain; 2015.
- Liao G, Li R, Li C, Sun M, Li Y, Chu J, Jiang S, Li Q. Safety and immunogenicity of inactivated poliovirus vaccine made from Sabin strains: a phase II, randomized, positive-controlled trial. J Infect Dis. 2012;205:237–43. doi:10.1093/infdis/jir723.
- Lu Z. [Determination of polio-neutralizing antibody by the micro-tissue culture technic]. Zhonghua Liu Xing Bing Xue Za Zhi. 1985;6:356–58.
- Adeniji AJ, Onoja AB, Adewumi MO. Polio virus neutralizing antibody dynamics among children in a north-central and South-Western Nigeria state. J Immunoassay Immunochem. 2015;36:45–53. doi:10.1080/15321819.2014.893889.
- Crawt L, Atkinson E, Tedcastle A, Pegg E, Minor P, Cooper G, Rigsby P, Martin J. Report on the WHO collaborative study to establish the 1st International Standard for Sabin inactivated polio vaccine (sIPV). Expert Committee On Biological Standardization. Geneva, Switzerland; 2018.
- Crawt L, Atkinson E, Tedcastle A, Pegg E, Minor P, Cooper G, Lei S, Ling P, Li C, Zheng J, et al. Differences in antigenic structure of inactivated poliovaccines made from Sabin live-attenuated and wild-type poliovirus strains: impact on vaccine potency assays. J Infect Dis. 2019. doi:10.1093/infdis/jiz076.