ABSTRACT
Under the trend of antibiotic resistance of H. pylori leading to the decrease of eradication rate, the development of a vaccine is the best choice to fight against H. pylori. In this study, we attempted to reduce the amounts of required antigens by using three different parenteral routes of immunization and an adjuvant cGAMP (cyclic guanosine monophosphate-adenosine monophosphate) to enhance the immunogenicity of the vaccine candidate. The immune protection and post-challenge immune responses were assessed and compared in mice immunized with recombinant Helicobacter pylori urease A, urease B, and neutrophil-activating protein adjuvanted with cGAMP. The gastric mucosal colonization by H. pylori was significantly reduced in mice immunized by intranasal and, to a less degree, subcutaneous route, but not by intramuscular route. All immunized mice, regardless of the route of immunization, displayed significant, but comparable, increases in antigen-specific serum IgG and mucosal IgA responses 5 weeks post-challenge. The magnitude of the vaccine-induced protection appeared to be associated with the level of antigen-specific Th1 and particularly Th17 responses, as IL-17 responses were only detected in intranasally immunized mice. Taken together, we explored and confirmed the possibility of using a novel adjuvant (cGAMP) to induce significant protective immunity with 10% of oral vaccine antigen dosage through parenteral immunization, especially intranasal immunization. This may provide an alternative approach to oral immunization for the development of effective H. pylori vaccines.
Helicobacter pylori infects at least half of the world population and causes chronic active gastritis, duodenitis, peptic ulcer, and other extra-gastrointestinal disorders.Citation1-4 Moreover, H. pylori infection is a known, strong risk factor for the development of gastric carcinoma and gastric mucosa-associated lymphoid tissue (MALT) lymphoma.Citation3,Citation5 Thus, timely diagnosis and effective eradication treatment with antibiotics are critical for preventing the development of gastric cancer. However, the successful eradication efficacy has been hindered by the rapid emerging of antibiotic-resistant H. pylori strains. H. pylori is regarded as one of the highly antibiotic-resistant bacteria by WHO in 2017.Citation6 As such, there is a renaissance in developing novel or alternative prophylactic or therapeutic H. pylori vaccines and other modalities.Citation7-10
Despite tremendous research efforts in the last several decades and the identification of a number of promising H. pylori vaccine antigens [such as urease A (UreA), urease B (UreB), and neutrophil-activating protein (NAP), etc.],Citation8-10 the development of a safe and effective H. pylori vaccine remains a major challenge. Results from recent experimental and clinical studies have shown that oral vaccination with H. pylori protein antigens and mucosal adjuvants is effective against H. pylori infection,Citation11-14 although some important practical issues (such as the multiple immunizations and the short duration of the protective immunity) remain to be resolved. It was reported that at least 300 µg antigens were needed to induce an effective protection in mice against H. pylori infection by oral route of immunization,Citation11,Citation13 so the requirement of large amounts of antigens in the milligram scale may bring new challenges to the vaccine production and cost. Therefore, exploration of alternative vaccine formulation and immunization strategies to reduce the antigen doses is warranted.
It is well established that parenteral route of vaccination requires less amounts of antigens than oral immunization, particularly when formulated with an effective adjuvant. Indeed, we previously showed that intramuscular (i.m.) and subcutaneous (s.c.) immunization of mice with antigen doses that are at least 10× lower than those used in oral immunization induced comparable antigen-specific antibody and CD4 T cell responses, although the immunization often failed to elicit any Th17 responses, which are potentially critical for the vaccine-induced protection against H. pylori.Citation15,Citation16
In this study, we assessed and compared the protection and post-challenge immune responses in mice immunized by three parenteral routes with recombinant H. pylori UreA, UreB, and NAP adjuvanted with cGAMP in an attempt to delineate the potential protective immune response. cGAMP is a second messenger and has been demonstrated as a promising molecular adjuvant by regulating innate immune responses in mammals and enhancing the antigen-specific cellular immune responses, especially Th1 responses.Citation17-19 We also examined whether intranasal (i.n.) immunization induced better immune responses, especially Th17 responses, than i.m or s.c. immunization in mice.
The H. pylori vaccine contains 10 μg of each UreA, UreB, and NAP (total 30 μg antigens) admixed with 5 μg cGAMP (InvivoGen, San Diego, CA) in a total volume of 26 μl for i.n. immunization or 100 μl for s.c. and i.m. immunization. UreA and UreB from H. pylori 26695 and NAP from H. pylori Sydney strain 1 (SS1), were expressed and produced in E. coli BL21 (DE3), with an N-terminal 6x His tag (Tiangen Biotech Co., Ltd, Beijing, China). The purity of the protein antigens was above 90% as detected by HPLC (1260 Infinity, Agilent Technologies Inc., Santa Clara, CA, USA). The amounts of endotoxin in antigens were <0.5 EU/µg protein as determined by kinetic turbidimetric limulus test.
Six-week-old, female BALB/c mice were purchased from Beijing Vital River Laboratory Animal Technology Co., Ltd (Beijing) and randomly divided into four groups (n = 6). This study was approved by the National Vaccines and Serum Institute Animal Ethics Committee (Approval #NVSI-RCD-JSDW-SER-2018011).
Mice were immunized at weeks 0 and 2 by one of the three parenteral routes (i.m., s.c. or i.n.). Intranasal immunization was performed under general anesthesia by intraperitoneal injection of 0.1 ml of 1% pentobarbital sodium and the vaccine was administered alternately from the two nostrils as we described previouslyCitation20 so that each nostril received about 13 µl. Two weeks after the last immunization, mice were challenged by gavage with 3 × 109 colony forming units (CFU) of freshly grown H. pylori in 0.2 ml Brucella broth. All mice were sacrificed 5 weeks after challenge for samples (blood, stomachs, and spleens) collection as described previously.Citation13,Citation15,Citation16 Half of the stomach was homogenized in 0.5 ml Brucella broth for 1 min at 60 Hz using Tissue Lyser (SCIENTZ-48, XinZhi, China) and centrifuged. The supernatant was collected and used for enzyme-linked immunosorbent assay (ELISA). The other half was similarly homogenized in Brucella broth and used for determination of the number of H. pylori.
Serum antigen-specific IgA, IgG, and IgG isotypes (IgG1 and IgG2a) as well as mucosal IgA responses against the three vaccine antigens used in mouse immunization were determined by indirect ELISA. Enzyme-linked immunospot assay (ELISpot) was used to determine the antigen-specific cellular immune responses (cytokines) as described elsewhere.Citation13,Citation15,Citation16
The GraphPad Prism 5 software (GraphPad Software Inc., San Diego, CA, USA) was used for statistical analysis. The data of multiple groups were compared by one-way ANOVA followed by Mann–Whitney U test. Differences were considered significant when P < .05.
We first compared the protective immunity against H. pylori colonization induced by different parenteral routes of immunization. Groups of immunized and unimmunized mice were challenged with H. pylori SS1 2 weeks after the last immunization, and the mice were sacrificed and the bacterial burdens in the stomach were determined 5 weeks post-challenge. As shown in , i.n. and, to a lesser degree, s.c. immunization induced a significant reduction of H. pylori colonization in the gastric mucosa as compared to unimmunized mice (P < .01 or P < .05, respectively), whereas i.m. immunization showed no overt protection against the challenge (). Moreover, the gastric H. pylori colonization was significantly lower in i.n. immunized mice than that in the s.c. or i.m. immunized mice.
Figure 1. The number of H. pylori in the gastric tissue of immunized and unimmunized (control) mice as detected by quantitative bacteriology. The stock of H. pylori SS1 was cultured on goat blood agar plates for 72 h in a microaerophilic environment, and then cultured for another 18 h in Brucella broth supplemented with 10% fetal calf serum with shaking at 150 rpm. The optical density of H. pylori liquid culture was adjusted to 1.0 (~1.5 x 1010 CFU/ml), and used in mouse challenge experiments. Groups of immunized and unimmunized mice were orally challenged with 3 × 109 CFU of H. pylori SS1 2 weeks after the last immunization, and the mice were sacrificed 5 weeks after challenge. Serial dilutions of the stomach homogenates were plated on Helicobacter-selective plates. After 72 h of incubation at 37°C under microaerophilic conditions, visible colonies with typical H. pylori morphology were counted. Plates with 30 to 300 colonies were used for calculating the number of bacteria per stomach by multiplying by the appropriate dilution factor. The detection limit of the assays is log10 2.2/g stomach tissue. Data are presented as mean ± SEM and are representative of one of two independent experiments with similar results
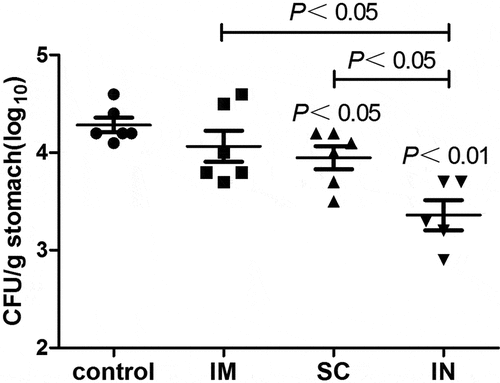
To get better knowledge on the effect of immunization route on the induced immune responses and protection, we compared the post-challenge serum antigen-specific IgG responses in unimmunized and immunized mice 5 weeks after H. pylori challenge. As shown in , the post-challenge IgG responses to all three antigens in mice immunized by different parenteral routes were significantly higher (approximately 1,000-fold for UreA, 500-fold for UreB, and 10,000-fold for NAP) than those in the unimmunized (control) mice (P < .01). In addition, the UreA-specific IgG was significantly higher in i.n. immunized mice than i.m. or s.c. immunization groups (P < .05 and 0.01, respectively). It was interesting to note that although the serum IgG responses in i.m. immunized mice were comparable to those in i.n. or s.c. immunized mice, the mice remained susceptible to the challenge (), suggesting the involvement of other immune components in the vaccine-induced protective immunity.
Figure 2. Serum antigen-specific IgG (A) and IgG1/IgG2a (B) responses in mice immunized with cGAMP adjuvanted H. pylori subunit vaccine by different parenteral routes. Groups of BALB/c mice (n = 6) were immunized with cGAMP adjuvanted H. pylori vaccine containing 10 µg of each UreA, UreB and NAP by the indicated route on days 0 and 14. The mice were orally challenged with 3 × 109 CFU of H. pylori SS1 at day 28, and sacrificed 5 weeks after challenge. The blood was collected and serum samples were prepared and assayed by ELISA. Serially diluted serum (10-fold) and gastric tissue homogenate supernatant (2-fold) were added to 96-well ELISA plates (100 µl/well), and incubated at 37°C for 1 h. After washing, horseradish peroxidase (HRP)-conjugated goat anti-mouse IgG, IgG1, or IgG2a (Southern Biotech, Birmingham, AL, USA) secondary antibody was added and incubated for 30 min. The wells were washed again before the addition of TMB substrate. The plates were incubated at 37°C for 15 min, the enzymatic reaction was stopped by adding 50-µl 2 M sulfuric acid, and the absorbance at 450 nm was then measured in a spectrophotometer (MultiskanMK3 plate reader, Thermo Scientific, Waltham, MA, USA). Data are presented as mean ± SEM and are representative of one of two independent experiments with similar results
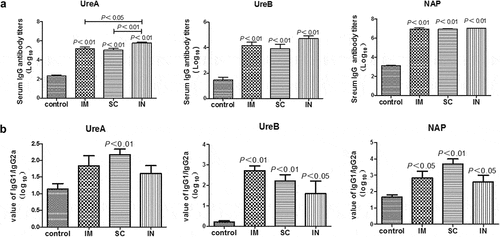
Analysis of the ratio of serum antigen-specific IgG1 and IgG2a showed that the parenteral immunization overall induced a high ratio of IgG1/IgG2a titer (), implying predominant Th2 immune responses. However, the IgG1/IgG2a ratios to all three vaccine antigens were generally lower in i.n. immunized mice than i.m. or s.c. immunized mice (), suggesting that the immune response induced by i.n. immunization tended to be more Th1 biased, or more Th1 and Th2 balanced, as compared to i.m. or s.c. route of vaccination.
Similarly, all immunized mice showed significantly higher UreA- and NAP-specific IgA responses (P < .01) in the stomach than unimmunized mice, but only i.n. immunization induced weak UreB-specific IgA responses (). In addition, there were large variations in the gastric UreB-specific IgA levels with some mice being strong responders and others weak responders. The reason for this was not clear. The potential of technical issue during the immunization was ruled out because the gastric IgA to UreA and NAP were much consistent between individual mice within the i.n. immunization group. The serum antigen-specific IgA responses were overall similar to the IgG responses in that all parenteral routes of immunization, especially the i.n. immunization, induced significant amounts of serum specific IgA responses (P < .05 and P < .01) in mice after H. pylori challenge (). In contrast to the UreB specific IgA responses in the stomach, which was induced only in i.n. immunized mice (), all routes of immunization induced significant amounts of serum UreB-specific IgA responses (). The finding that i.n. immunized mice showed the highest IgA levels in serum and stomach and best protection among the three immunized groups would suggest a potential role of IgA in anti-H. pylori immunity. In this regard, it was previously reportedCitation21,Citation22 that IgA played an important role to kill and eradicate H. pylori by its neutralization function although early workCitation9,Citation23 has questioned the contribution of IgA or even antibodies and B cells in the protective immunity in the mouse model of H. pylori infection. It was shown that prophylactic immunizaion of B cell knockout mice protected against H. pylori challenges at a comparable magnitude to those in wild type mice.Citation24 The controversy in the potential role of antibodies and B cells in the anti-H. pylori protective immunity can perhaps partially contribute to the difference in the vaccine antigens, immunization routes, and the adjuvants used among different studies.
Figure 3. Antigen-specific IgA responses in the stomach (A) and serum (B) of mice immunized with cGAMP adjuvanted H. pylori subunit vaccine by different parenteral routes. Groups of BALB/c mice (n = 6) were immunized with cGAMP adjuvanted H. pylori vaccine containing 10 μg of each UreA, UreB, and NAP by the indicated route on days 0 and 14. The mice were orally challenged with 3 × 109 CFU of H. pylori SS1 at day 28, and sacrificed 5 weeks after challenge. The stomachs and blood were collected and samples were prepared and assayed by ELISA as described in detail in legend, except that HRP-conjugated goat anti-mouse IgA (Southern Biotech, Birmingham, AL, USA) was used as secondary antibody. Data are presented as mean ± SEM and are representative of one of two independent experiments with similar results
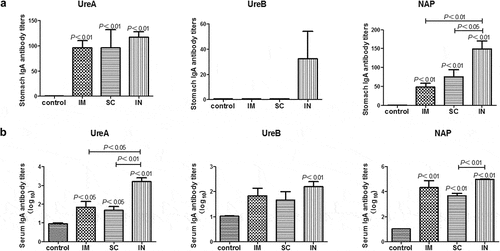
Recent studies suggested an important role of cell-mediated immunity (CMI), particularly antigen-specific CD4 + T cells and Th1/Th17 responses, in the vaccine-induced protective immunity against H. pylori infection.Citation23,Citation25 To better understand the potential effect of the immunization route on immune responses induced by our vaccine candidate, we compared the interferon (IFN)-γ, interleukin (IL)-17 and IL-4 responses in the culture supernatant of antigen-stimulated splenocytes from immunized and unimmunized mice. Results from ELISpot assays showed that all parenteral routes of immunization induced significant numbers of IFN-γ and IL-4 producing cells in the spleen (P < .01) (), but only i.n. immunization induced significant IL-17 responses (P < .01). In this regard, previous studies have shown that oral immunization with high antigen doses and mucosal adjuvant double-mutant heat-labile toxin induced significant Th17 responses in mice and protected against H. pylori challenge.Citation13 On the other hand, s.c. or i.m. immunization of mice with H. pylori antigens adjuvanted with cGAMP induced strong antibody and Th1 immune responses, but failed to induce any Th17 responses.Citation15,Citation16 Since both Th1 and Th17 responses are implicated in the vaccine-induced protection against H. pylori infection by recruiting leukocytes, mainly neutrophils, to the site of infection and activating innate immunityCitation23,Citation25 and Th17 cells are abundant in the gastrointestinal tract, the induction of specific IL-17 responses in i.n. immunized mice perhaps contributed to their better protection against H. pylori challenge than the s.c. and i.m. immunization. In addition, the IFN-γ response in the i.n. immunized mice was significantly higher than that in mice immunized by i.m. or s.c. route (P < .01). On the other hand, i.n. immunization induced fewer IL-4-producing cells than i.m. or s.c. immunization (). Moreover, the IFN-γ and IL-4 responses in the restimulated splenocytes corroborated well with the serum IgG1 and IgG2a results, which showed a stronger Th1 and weaker Th2 responses in i.n. immunized mice as compared to i.m. or s.c. immunized mice.
Figure 4. Antigen-specific cytokine-producing cell frequencies in mice immunized with cGAMP adjuvanted H. pylori subunit vaccine by different parenteral routes. Single splenocyte suspensions were prepared in Dulbecco,s Modified Eagle Medium (DMEM, Gibco) supplemented with 10% heat-inactivated fetal calf serum (Gibco) and 50 μg/ml penicillin-streptomycin (Sigma), and seeded (1 × 106 cells per well) in 96-well PVDF plates that were pre-coated with different anti-cytokine antibodies. The cells were cultured at the presence or absence of H. pylori antigens (10 μg/ml) for 40 h at 37°C and 5% CO2. The positive spots were developed following the ELISpot kits operation guide and counted by an Elispot Reader (S5VERSA, CTL, Cleveland, OH, USA). The number of ELISpot positive splenic lymphocytes was numerated and presented, respectively, for IFN-γ (A), IL-17 (B) and IL-4 (C). Data are representative of one of two independent experiments giving similar results. The bars represent mean ± SEM of the positive cells to the respective cytokines
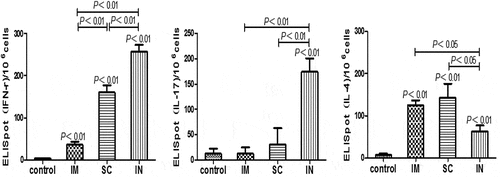
In this study, all three parenteral routes of immunization significantly increased the number of IL-4 and IFN-γ secreting splenocytes in the immunized mice, as compared to unimmunized mice. Moreover, the i.n. immunized mice showed higher frequency of IL-17 and IFN-γ secreting cells and lower frequency of IL-4 secreting cells than the i.m. or s.c. immunized mice, suggesting that this route of immunization could induce substantial Th1 and Th17 immune responses, especially Th17 responses. On the other hand, there were no overt IL-17 responses induced in i.m. and s.c. immunization groups, but their IFN-γ responses, especially in the s.c. group, were significantly higher than unimmunized group. A substantial reduction in the gastric H. pylori colonization was also observed in the s.c. immunization group of mice. These results supported the notion that antigen-specific Th1 responses play an important role in inducing protective immunity against H. pylori infection in mice and that Th17 responses may contribute further to the protection. Although i.m. immunization induced obvious IFN-γ responses, the number of H. pylori colonization in stomach was not different from the unimmunized mice. This raises the possibility that the IFN-γ response alone was not sufficient for the induction of a significant protection. Our study also suggests that significant decrease of gastric H. pylori colonization appears associated with strong antigen-specific IgG and IgA responses as well as T cells responses (including Th1 and Th17), as demonstrated in i.n. immunized mice.
In summary, we compared the post-challenge immune responses and protective efficacies among the three parenteral routes (s.c., i.m., and i.n.) of immunization and determined whether i.n. immunization with low amounts of H. pylori antigens adjuvanted with cGAMP can induce protective anti-H. pylori immunity in mice. Our results showed that i.n. and, to a lesser extent s.c., but not i.m., immunization induced significant reduction in the gastric H. pylori colonization in mice. Comparison of the post-challenge immune responses suggests that the protection was associated with the enhanced gastric antigen-specific IgA responses and the induction of Th1/Th17 responses. Mucosal immunization appears to be essential for this vaccine to induce Th17 responses which may contribute to the robust protection against H. pylori infection.
Despite 30 y of research efforts, the field of H. pylori vaccine development has not progressed significantly. The exact reason for this remains unknown. As in many other vaccines against microbial pathogens, it is likely to be multifaceted and associated with the ability of H. pylori to evade the attack of host immune responses, the lack of identified correlate of protection, and the requirement of protective immunity in addition to antibody responses. Although a phase III clinical trial with an oral subunit H. pylori vaccine (rLTB-UreB fusion protein) was successful in China in 2015 and the vaccine was subsequently approved by the regulatory authority, 12 the requirement of large amounts of antigens (15 mg/dose) for oral immunization hampers the vaccine to be successfully marketed. The vaccine was subsequently approved by the regulatory authority,Citation12 but the requirement of large amounts of antigens (15 mg/dose) for oral immunization hampers the vaccine to be successfully marketed. In this study, we attempted to reduce the amounts of required antigens by using parenteral route of immunization and an adjuvant to enhance the immunogenicity of the vaccine candidate. We did not detect the pre-challenge immune responses in immunized mice because most of the H. pylori vaccine studies have focused on the pre-challenge immune responses. The immune protection and post-challenge immune responses were assessed and compared side by side in mice, and the results provided an encouraging alternative approach to develop mucosal H. pylori vaccine via parenteral (i.n.) administration with low antigen dose.
Although our results demonstrated the feasibility to induce effective protective immunity against H. pylori infection by intranasal immunization with low antigen dosage, it remains to be determined if the quality and duration of the protective immunity induced by i.n. immunization is comparable or even superior to that induced by oral immunization. In addition, there were some concerns on the safety of i.n. route of immunization. However, this was perhaps more an issue when live attenuated vaccines or live vaccine vectors are used. Indeed, an i.n. flu vaccine (Flumist®) has been approved for eligible children and adults ages 2–49. Further, detailed studies to determine the optimal vaccine dose, dosage form, the immunization schedule, the biodistribution, and safety will be needed.
Disclosure of potential conflicts of interest
No potential conflicts of interest were disclosed.
Additional information
Funding
References
- Matsumoto H, Shiotani A, Graham DY. Current and future treatment of helicobacter pylori infections. Adv Exp Med Biol. 2019;1149:211–25. doi:10.1007/5584_2019_367.
- de Brito BB, da Silva FAF, Soares AS, Pereira VA, Santos MLC, Sampaio MM, Neves PHM, de Melo FF. Pathogenesis and clinical management of Helicobacter pylori gastric infection. World J Gastroenterol. 2019;25(37):5578–89. doi:10.3748/wjg.v25.i37.5578.
- Chmiela M, Karwowska Z, Gonciarz W, Allushi B, Staczek P. Host pathogen interactions in Helicobacter pylori related gastric cancer. World J Gastroenterol. 2017;23(9):1521–40. doi:10.3748/wjg.v23.i9.1521.
- Johnson KS, Ottemann KM. Colonization, localization, and inflammation: the roles of H. pylori chemotaxis in vivo. Curr Opin Microbiol. 2018;41:51–57. S1369-5274(17)30153-4 [pii]. doi:10.1016/j.mib.2017.11.019.
- Kanglesiji DYU, Lin Y, Xiaohong MA. Helicobacter pylori infection in the plateau region of China and its relationship with the pathogenesis of gastric cancer. Oncol Prog. 2017;15(5):584–6,603. doi:10.11877/j.1672-1535.2017.15.05.31.
- Tacconelli E, Carrara E, Savoldi A, Harbarth S, Mendelson M, Monnet DL, Pulcini C, Kahlmeter G, Kluytmans J, Carmeli Y, et al. Discovery, research, and development of new antibiotics: the WHO priority list of antibiotic-resistant bacteria and tuberculosis. Lancet Infect Dis. 2018;18(3):318–27. S1473-3099(17)30753-3 [pii]. doi:10.1016/S1473-3099(17)30753-3.
- Mirzaei N, Poursina F, Moghim S, Rashidi N, Ghasemian Safaei H. The study of H. pylori putative candidate factors for single- and multi-component vaccine development. Crit Rev Microbiol. 2017;43(5):631–50. doi:10.1080/1040841X.2017.1291578.
- Guo L, Yang H, Tang F, Yin R, Liu H, Gong X, Wei J, Zhang Y, Xu G, Liu K. Oral immunization with a multivalent epitope-based vaccine, based on NAP, urease, HSP60, and HpaA, provides therapeutic effect on H. pylori infection in mongolian gerbils. Front Cell Infect Microbiol. 2017;7:349. doi:10.3389/fcimb.2017.00349.
- Li B, Yuan H, Chen L, Sun H, Hu J, Wei S, Zhao Z, Zou Q, Wu C. The influence of adjuvant on UreB protection against Helicobacter pylori through the diversity of CD4+ T-cell epitope repertoire. Oncotarget. 2017;8(40):68138–52. doi:10.18632/oncotarget.19248.
- Yang WC, ChenL, Li HB, Li B, Hu J, Zhang JY, Yang SM, Zou QM, Guo H, Wu C. Identification of two novel immunodominant UreB CD4(+) T cell epitopes in Helicobacter pylori infected subjects. Vaccine. 2013;31(8):1204–09. doi:10.1016/j.vaccine.2012.12.058S0264-410X(12)01845-2.
- Sjokvist Ottsjo L, Jeverstam F, Yrlid L, Wenzel AU, Walduck AK, Raghavan S. Induction of mucosal immune responses against Helicobacter pylori infection after sublingual and intragastric route of immunization. Immunology. 2017;150(2):172–83. doi:10.1111/imm.12676.
- Zeng M, Mao XH, Li JX, Tong WD, Wang B, Zhang YJ, Guo G, Zhao ZJ, Li L, Wu DL, et al. Efficacy, safety, and immunogenicity of an oral recombinant Helicobacter pylori vaccine in children in China: a randomised, double-blind, placebo-controlled, phase 3 trial. Lancet. 2015;386(10002):1457–64. doi:10.1016/S0140-6736(15)60310-5S0140-6736(15)60310-5.
- Zhong YX, Chen J, Liu Y, Tang CF, Wei B, Liu MY. Oral immunization of BALB/c mice with Helicobacter pylori lysate combined with dmLT adjuvant for the induction of mucosal and system immune response. Chin J Microbiol Immunol. 2019;39(4):298–304. doi:10.3760/cma.j.0254-5101.2019.04.009.
- Li J, Lee RK, Chen W, Yan H. 2′-Fluoro-c-di-GMP as an oral vaccine adjuvant. RSC Adv. 2019;9(71):41481–89. doi:10.1039/c9ra08310c.
- Chen J, Zhong YX, Tang CF, Liu Y, Wang XW, Zhang YB, Wang P, Liu MY, Wei B. Cyclic guanosine monophosphate-adenosine monophosphate (cGAMP) enhances the immune responses against Helicobacter pylori in BALB/c mice. Chin J Microbiol Immunol. 2018;38(12):914–21. doi:10.3760/cma.j.0254-5101.2018.12.007.
- Chen J, Zhong YX, Liu Y, Tang CF, Zhang YB, Wei B, Liu MY. The preliminary analysis of immune response in BALB/c mice immunized with Cyclic guanosine monophosphate-adenosine monophosphate (cGAMP) and protein antigens from Helicobacter pylori by intramuscular injection. Chin J Biol. 2019;32(10):1074–79. doi:10.13200/j.cnki.cjb.002827.
- Kato K, Omura H, Ishitani R, Nureki O. Cyclic GMP-AMP as an endogenous second messenger in innate immune signaling by cytosolic DNA. Annu Rev Biochem. 2017;86:541–66. doi:10.1146/annurev-biochem-061516-044813.
- Yan Q, Wu X, Zhang Z, Guo F, Yang Q, Yang G. Cyclic guanosine monophosphate-adenosine monophosphate (cGAMP): a novel vaccine adjuvant. Chin J Microbiol Immunol. 2017;37(10):790–94. doi:10.3760/cma.j.0254-5101.2017.10.012.
- Chen J, Liu MY, Tang CF, Liu Y, Zhong YX, Tang F, Ma ZJ, Hou JW, Wei B. Cyclic guanosine monophosphate-adenosine monophosphate (cGAMP) enhances humoral immune responses against norovirus (GⅡ. 4) virus-like particles. Chin J Microbiol Immunol. 2017;37(10):761–65. doi:10.3760/cma.j.0254-5101.2017.10.007.
- Patel GBZH, Ponce A, Chen W. Mucosal and systemic immune responses by intranasal immunization using archaeal lipid-adjuvanted vaccines. Vaccine. 2007;25(51):8622–36. doi:10.1016/j.vaccine.2007.09.042.
- Wang G, Yu S, Yu X. Progress of immune mechanism on Helicobacter pylori vaccine. Chin J Cell MoI Immunol. 2017;33(1):128–32. doi:10.13423/j.cnki.cjcmi.008060.
- Fan HY, Wu XB, Yu F, Bai Y, Long BG. Oral immunization with recombinant Lactobacillus acidophilus expressing the adhesin Hp0410 of Helicobacter pylori induces mucosal and systemic immune responses. Clin Vaccine Immunol. 2014;21(2):126–32. doi:10.1128/CVI.00434-13CVI.00434-13.
- Sun H, Yuan H, Tan R, Li B, Guo G, Zhang J, Jing H, Qin Y, Zhao Z, Zou Q, et al. Immunodominant antigens that induce Th1 and Th17 responses protect mice against Helicobacter pylori infection. Oncotarget. 2018;9(15):12050–63. doi:10.18632/oncotarget.23927.
- Akhiani AA. The role of type-specific antibodies in colonization and infection by Helicobacter pylori. Curr Opin Infect Dis2005;18(3):223–27. doi:10.1097/01.qco.0000168382.86024.14.
- Bagheri N, Salimzadeh L, Shirzad H. The role of T helper 1-cell response in Helicobacter pylori-infection. Microb Pathog. 2018;123:1–8. doi:10.1016/j.micpath.2018.06.033.