ABSTRACT
Since their widespread use, pneumococcal conjugate vaccines (PCVs) have proven effective at reducing both invasive and noninvasive pneumococcal diseases and nasopharyngeal carriage of Streptococcus pneumoniae (Spn). To establish this level of protection, a three-dose schedule with a single booster (3 + 1) was the immunization regime in the USA. Alternatively, WHO-approved schedules of 3 + 0 and 2 + 1 are now becoming adopted in many countries to reduce the cost of vaccination. Sustained protection from pneumococcal disease and carriage requires persisting levels of antibody and cellular immune memory. Although antibody responses to PCVs are well studied, less is known concerning the cellular response to the vaccine in young children. In this report, circulating PCV-13 serotype-specific B and T cell memory in paired blood samples from children before and after PCV13 dose 3 and booster immunizations was analyzed to determine changes in the adaptive immune response. No significant differences in memory B cell populations were detected comparing post dose 2 vs. post dose 3. However, the booster dose significantly increased the frequency of Spn-specific memory B cells compared to the pre-booster. Spn-specific memory T cells were not detected with the method used. These data suggest that booster vaccination increases Spn-specific memory B cells that may impact long-term protective antibody titers.
Introduction
It has been well demonstrated that the covalent attachment of an immunogenic protein to a polysaccharide antigen (i.e. conjugate vaccine) converts the polysaccharide antigen from a T-independent to a T-dependent antigen that induces immunologic memory.Citation1,Citation2 Studies with the first conjugate vaccine – Haemophilus influenzae b conjugate – showed that three primary doses plus a booster (3 + 1) resulted in protective antibody and cellular memory responses.Citation3,Citation4 Following this approach, pneumococcal conjugate vaccines (PCVs) have been proven to be effective among infants and young children for protection against invasive pneumococcal diseases (IPDs)Citation5,Citation6 and non-IPD diseases such as pneumonia, sinusitis, and otitis media.Citation7-9 Protection from the acquisition of nasopharyngeal (NP) carriage by vaccine serotypes of S. pneumoniae (Spn) also occurs.Citation5,Citation6,Citation10,Citation11 Generating and sustaining efficacious humoral immunity by PCV13 directed against pneumococcal antigens requires effector and memory B and CD4+ T cells.Citation12,Citation13 However, there are limited studies of PCV13 effectiveness in generating a cellular immune memory response, especially in blood samples collected from young children.
Different pneumococcal vaccination schedules are recommended across Europe and other countries after consideration of the epidemiology, disease burden, immunogenicity of the vaccine, its compatibility with other vaccines, and cost to purchase. With consideration to cost and a focus on IPD, the WHO recently updated its PCV policy to support the use of three doses as either “3 + 0” or “2 + 1” schedules.Citation14,Citation15 We lack a clear understanding of the full impact of reduced-dose schedules on IPD and non-IPD relative to 3 + 1. Most European countries have adopted the 2 + 1 schedule according to their routine infant immunization schedule.
Clinically relevant levels of functional antibodies against Spn are achieved by serial primary doses of PCV13, followed by an additional increase in antibody following booster vaccination in both the 2 + 1 and 3 + 1 schedules.Citation16,Citation17 The relative role of the third primary and booster vaccinations in reaching sustained protective antibody titers against the pneumococcal serotypes included in the vaccine is less clear.Citation14 In our recent work (Kaur, et al., Vaccine, provisionally accepted and under revision), we compared serum antibody levels from infants after dose 2 and dose 3 of PCV13 and pre- (3 + 0) versus post-booster (3 + 1) PCV13. We found that PCV13 dose 3 increased antibody titers to 5 of 13 Spn vaccine serotypes, and booster further increased antibody titers to all 13 vaccine serotypes. In a study comparing Dutch children who received PCV10 versus PCV13, both circulating antibody and memory B cell frequencies were higher for most vaccine serotypes after PCV10 or PCV13 booster vaccination.Citation18 However, a comparison of dose 3 and booster vaccinations was not done, and paired samples were not analyzed from the same children. Studies in adults have also shown production and replenishment of antibody-producing memory B cells after PCV13.Citation12,Citation13 To further study the value of PCV13 dose 3 and booster in generating cellular memory, we have compared B and T cell memory responses after 2, 3, and post-booster doses of PCV13 vaccine in children.
Methods
Study population
Samples were secured under an approved prospective longitudinal study of acute otitis media (AOM) protocol conducted in Rochester, NY (NIH NIDCD R01 08671), where permission had been granted for their use for other research purposes. Details of sample collection have been described previously in detail including parent consent and Institutional Review Board approval.Citation19-22 The study design included blood samples collected from children at 6, 9, 12, 15, 18, and 24 months of age. Child subjects were mostly from middle-class socioeconomic status families. All children who participated in the study received PCV13 vaccine at 2, 4, and 6 months of age and booster at ~15 months of age. As a consequence of the approved sampling time points for the study, samples analyzed after PCV13 dose 2, dose 3, and booster are from 2, 3, and 3 months post immunization, respectively. In all, 14 paired samples from 7 subjects were used for 2p versus 3p analysis, and 18 paired samples from 9 subjects were used for pre- versus post-booster analysis.
Polysaccharide labeling
Labeling of polysaccharides with fluorescent dye was performed as described by Khaskhely, et al.Citation23 with some modifications. Briefly, conjugation of all the polysaccharides to labeling molecule 5-(4,6-dichlorotriazinyl) aminofluorescein (DTAF) (Sigma-Aldrich) was performed by combining 1 mg of each polysaccharide. Pooled polysaccharides (13 mg/ml in 0.1 M borate buffer, pH 9) were incubated with 1 mg of 5-DTAF for 2.5 hours at 4ᵒC with gentle shaking. After the incubation, the above mixture was exchanged with Phosphate buffer saline (PBS) at 4°C using Centricon (MW cutoff = 3 kDa) by concentrating down to 1 ml and 4 changes of PBS to 50 ml. Approximately 10 µl of 5 M sodium cyanoborohydride was added to the exchanged sample and mixed for another 30 minutes in the dark at 4ᵒC. The samples were again exchanged in PBS and subjected to chromatography on a Sephadex G-25 column in the dark. Fractions containing labeled pooled polysaccharide complex with DTAF were pooled, aliquoted, and stored at −20°C. Labeled material was used within six months of labeling, and for each experiment, a small vial was thawed for use.
Peripheral blood mononuclear cell samples
Peripheral blood mononuclear cells (PBMCs) were isolated by differential centrifugation on Ficoll-Isopaque (Pharmacia), washed in PBS, suspended at a concentration of 107 cells/ml in cell recovery freezing media (Gibco), and frozen in liquid nitrogen for future use. 7–10 × 106 PBMCs per sample used for cellular analyses were thawed from liquid nitrogen and rested overnight in complete RPMI (10% heat-inactivated fetal bovine serum and pen/strep) in individual culture flasks. Cells were then harvested from flasks, washed in complete RPMI media with 10 mg/ml DNase I, and counted by Trypan blue exclusion. Any samples with less than 50% live cells were excluded from further analysis; most samples were >80% live. Cells were then split: 1 × 106 used for B cell flow cytometry and the remainder for T cell cultures. PBMCs for T cell analysis were added 1–2 × 106 cells/well to a 96-well round-bottom plate and incubated overnight in complete media. Three groups were media alone, 1 μg/ml Spn polysaccharide pool, or 10 ng/ml staphylococcal enterotoxin B (SEB). After 6 hours of incubation, all wells had 5 μg/ml brefeldin A added for the prevention of cytokine secretion. After overnight incubation, cells were harvested, washed in complete media, and stained for analysis by flow cytometry. For control, PBMCs from cord blood (negative control) and adult blood were used to standardize the labeling conditions and specificity.
Flow cytometry
B-cell assay
To enumerate total and PCV13 antigen-specific B cell subsets, total lymphocytes (1 × 106 cells per well) were incubated with Fc receptor blocking antibodies and then with DTAF-labeled PCV13 antigens (Spn-DTAF). For other surface staining, B cell panel included CD27-PE; CD24-PerCP/Cy5.5; CD21-PE/Cy7; IgD-APC; CD20-APC/Cy7; CD38-Pacific Blue; Live-Dead Aqua; and CD19-BV605 (BioLegend). Cells were run on an LSRII (BD) flow cytometer using proper unstained and fluorescence minus one (FMO) controls and then analyzed with FlowJo software (BD).
T-cell assay
After overnight incubation and harvest, PBMCs were incubated with Fc receptor-blocking antibodies and then surface stained with CD4-AF488; CD3ϵ-PE/Dazzle 594; CD45RA-PerCP/Cy5.5; CD69-APC; and CD8α-V450 (all from BioLegend except CD8α antibody (BD)). Cells were washed and fixed with 4% paraformaldehyde and then maintained in Fix/Perm buffer (BD) for the remainder of staining procedure. After a wash in Fix/Perm, intracellular staining was performed with IL-4-PE; IFN-γ-PE/Cy7; IL-2-AF700; IL-17A-APC/Cy7; and TNF-α-BV605 (BioLegend). Cells were then washed and re-suspended in PBS for flow cytometry analysis with associated FMO controls on an LSRII (BD). Data analysis was performed with Flowjo (BD).
Antibody
Antibody titers to Spn serotypes were determined as previously described.Citation24 In brief, the ELISA included adsorption with pneumococcal cell wall polysaccharide mixture, CWPS Multi, a mixture of CWPS1 & CWPS2, which increases assay specificity when measuring pneumococcal serotype-specific total IgG antibodies. Pure polysaccharides used in the assay were purchased from the American Type Culture Collection (ATCC) and Statens Serum Institute (SSI).Citation25-27 Antibody concentrations were determined with the standard US reference pneumococcal serum lot G007, obtained from US FDA Center for Biologics Evaluation and Research, CBER that has an assigned value for each serotype (https://www.vaccine.uab.edu/007spBridging.pdf). In-house human control sera, low-, medium-, and high-positive titers, were established and run on each plate of samples as a control for assay variability. The assay cutoff values were 0.05–0.15 µg/ml for different serotypes.
Statistics
To compare data from clinic visits within the same patient, Wilcoxon matched-pairs signed-rank test was used to determine significance. Correlation analyses shown in were done using Spearman’s test. A p value <0.05 was considered significant. Data analysis was performed using Prism software (GraphPad).
Results
Patient cohort and identification of study samples
As a means of testing the cellular vaccine response to PCV13 in children, we utilized biorepository samples collected from children in Rochester, NY.Citation28 The demographics and composition of the cohort have been previously published (see Methods). Patient samples were chosen based on demographics, age, and availability of PBMCs from time points after PCV13 dose 2, dose 3, and pre- and post-booster vaccinations. We further screened for subjects that had low numbers of AOM events (fewer than three AOM episodes up to 36 months of age) since prior published work has suggested that a subset of otitis-prone children are low vaccine responders and have lower frequencies of circulating memory B cells.Citation29,Citation30 All children in the study received the full course of PCV13 vaccination at the prescribed ages. The paired samples used in these analyses are termed 2p and 3p (representing 2 months after dose 2 and 3 months after dose 3 of PCV13) and pre-B and post-B (representing pre-boost and 3 months after boost). The sampling visits were scheduled by age, thus effectively age matching the sample set across all patients studied.
Changes in circulating B cell memory during the PCV13 vaccination course
To address the impact of PCV13 vaccine on circulating B cell memory over the vaccine course, polysaccharides from the PCV13 serotypes were pooled and conjugated to DTAF (Spn-DTAF) as a means of detecting Spn-specific B cells by flow cytometry. A representative staining panel for B cells is shown in . In order to test Spn-DTAF reagent specificity, a small percentage of CD19+ B cells stained positive in child PBMCs but not in cord blood samples compared to FMO control (). In addition, a higher frequency of CD19+ CD27+ memory B cells stained with Spn-DTAF compared to total CD19+ cells (average of 2.38% Spn-DTAF+ among CD19+ cells, and 5.19% Spn-DTAF+ among CD19+ CD27+ cells, p < 0.001 by paired T-test).
Figure 1. Flow cytometry gating scheme for identification of Spn-specific B cells
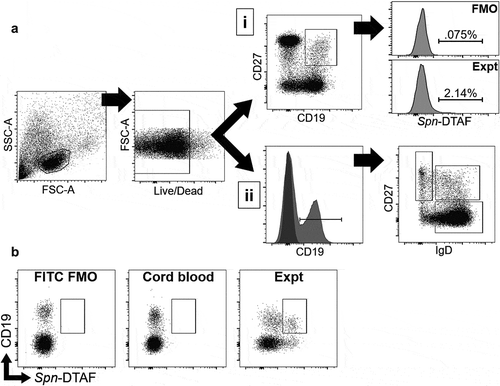
Patient PBMCs from 2p, 3p, pre-B, and post-B time points were thawed and rested overnight (see Methods). Memory B cells were identified as CD19+ CD27+ PBMCs and then further stained with Spn-DTAF to identify antigen-specific cells. There was a significant increase in the percentage of both overall (Supplemental Figure 1A) and Spn-DTAF+ memory B cells () in post-B samples compared to pre-B. No difference in memory B cell populations was identified comparing 2p and 3p PBMCs ( and Supplemental Figure 1A). The percentage frequency of Spn-DTAF+ cells was higher at 2p and 3p compared to pre-B and post-B, likely due to the larger overall memory B cell pool in older children. Published data have demonstrated that memory B cells can be further segregated by IgD staining: IgD+ memory B cells have memory characteristics but are not class switched, while IgD- memory B cells have increased co-stimulatory molecule expression, express IgA or IgG, and have increased activation-induced cytidine deaminase expression compared to IgD+ memory cells.Citation31 Therefore, memory B cells (CD19+ CD27+) were further segregated by IgD+ and IgD- and those populations analyzed across visit types. Interestingly, IgD- memory B cells were the only population to show a significant difference and only in the comparison of pre-B and post-B PBMCs (Supplemental Figure 1B–C). Taken together, Spn-specific memory B cells as well as total and IgD- memory B cells increase in frequency after booster vaccination, suggesting antigen exposure from booster vaccination increased these populations of circulating memory B cells.
Figure 2. Changes in circulating B cell populations during the PCV13 vaccination course
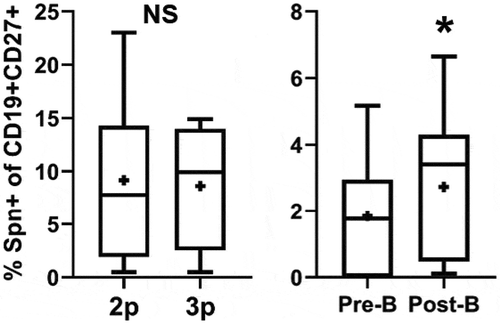
Some patient samples analyzed for PBMC responses were the same as those analyzed for serum antibodies to PCV13 in our previous analyses (Kaur, et al., Vaccine, provisionally accepted and under revision). Therefore, serum antibody and cellular B cell responses were compared to determine whether there was a correlation between circulating IgG and the frequency of memory B cells specific for Spn. shows that, for the patients in this overlapping data set, there was no correlation between serum IgG and memory B cell frequency.
Figure 3. Correlation analysis of circulating Spn-specific B cells and IgG
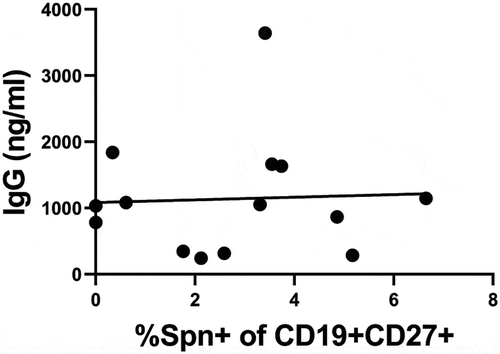
T cell response to vaccine antigens ex vivo
In order to restimulate PCV13-reactive T cells in PBMCs, we utilized an antigen pool of polysaccharides from all 13 PCV13 serotypes. This was chosen rather than PCV13 itself so as to avoid additionally detecting responses from the CRM197 protein used to conjugate the Spn polysaccharides due to its use in vaccine formulations other than PCV13. For flow cytometry analysis, CD4+ T cells responding in the restimulation culture were identified as CD3+ CD4+ CD69+ cells (representative staining panel in Supplemental Figure 2A). Compared to media control, Spn-stimulated cells were not detected above background in the assay. However, SEB stimulation resulted in an increase in CD69+ cell frequency across all samples and all time points (Supplemental Figure 2B). Using SEB-stimulated conditions, PBMCs were compared across the various visit types for potential differences. No statistically significant differences were observed in the frequency of CD69+ cells comparing 2p to 3p and pre-B to post-B (Supplemental Figure 2C). Data were also analyzed for CD8+ T cells and CD45RAlow populations with similar results (data not shown). Cytokines were analyzed by measuring the production of IL-2, IL-4, IFN-γ, TNF-α, and IL-17A in restimulation cultures. The group-averaged data set is reported in Supplemental Table 1. Similar to other measures, there were no significant differences in the frequency of cytokine-secreting cells between medium- and Spn-stimulated conditions. Cultures stimulated with SEB showed increased recovery of most cytokines as expected, but no differences comparing 2p vs. 3p or pre-B vs. post-B.
Discussion
Understanding the impact of PCV vaccine schedules on cellular immune memory is important because the maintenance of antibody-mediated protection against pneumococcal disease, NP carriage, and consequent herd immunity requires memory B and T cells. Unfortunately, there are limited data comparing the cellular immune response before and after various vaccine doses throughout the schedule in order to determine best practice. In this report, we attempt to address this for PCV13 vaccination by asking how dose 3 and booster vaccines impact cellular immunity. Taken together, our data suggest that dose 3 does not greatly impact the number of circulating Spn-specific memory B cells compared to dose 2; however, booster immunization significantly increases circulating Spn-specific memory B cells. Longer-term protection might be anticipated from such a result. Interestingly, we also observed increased frequencies of total and IgD- memory B cells after booster immunization. Since several booster immunizations are given around the same time as PCV, these data further suggest that this immunization schedule results in an overall increase in class-switched memory as a result of booster immunization.
Despite overnight restimulation with Spn antigens, it was difficult to obtain robust data for T cell responses in an antigen-specific fashion. A limitation of this study is that once samples were split for separate B and T cell analyses, there was limited sample for testing of multiple culture conditions. It is possible that a longer ex vivo stimulation period (to allow for proliferation of Spn-specific T cells as previously published by our group using heat-killed bacteriaCitation32) or addition of exogenous co-stimulationCitation33 could have increased the signal-to-noise in these cultures. Alternatively, the exclusion of CRM197 from the restimulation conditions may have eliminated the detection of some immunodominant T cell epitopes from the culture. Overall, it is unclear from this study whether PCV booster vaccination also impacts circulating Spn-specific memory T cells. Further work is needed to determine the impact of immunization on these cell populations.
For a subset of patients, both serum IgG and circulating memory B cells were compared, and they did not correlate within the study group. These data suggest that circulating memory cannot be inferred from relative serum IgG levels and that booster vaccine responses may be variable compared to responses observed to earlier primary vaccinations in the same patient. At face value, this result is not surprising since circulating memory B cells are not the primary source of class-switched antibody production. However, these data do suggest that some children who made a strong antibody response to primary vaccination did not make a strong cellular response to booster. On the other hand, some children who did not make a strong antibody response to primary vaccination did make a strong memory response. Since memory B cells are known to rapidly respond to a future encounter with the same pathogen and participate in boosting the plasma cell pool,Citation34 it is suggestive that children who made weak vaccine responses in the first year of life can ‘catch up’ by making robust cellular responses to booster.
One caveat to the comparison of responses to dose 2, dose 3, and booster is the amount of time between vaccination and sample collection since it varied from 2 to 3 months. Comparing the sets of data, there was more variability in results from 2p and 3p samples compared to pre-B and post-B. The most likely explanation for this is the timing of the vaccination course. Given that the second and third doses of PCV13 are given at 4 and 6 months of age, respectively, differences in maternal antibody levels and individual differences in vaccine response will have a greater impact. In contrast, the 15- and 18-month time points were compared for pre- and post-booster studies when children have received more PCV13 doses and maternal antibody has waned. Taking these differences into account, we only compared data between 2p and 3p and pre- and post-booster in our analyses.
Our study has limitations. The blood draw sampling schedule to study antibody, B and T cell memory responses after PCV13 was not 1-month post vaccination as occurred in most prior studies. Our opportunistic collection of blood samples occurred 2–3 months post vaccination. Measurement of antibody and memory B and T cell responses 1 month after vaccination would almost certainly have been preferred as this is most common in immunogenicity studies. The study was not designed a priori for any immunogenicity outcomes, and the results are consequently mainly hypothesis generating.
Given the increased total number of childhood vaccinations and the transfer of vaccines to developing nations with different clinical infrastructures, vaccine schedules need to be limited to what is essential for effective protection. Overall, our data suggest that PCV13 booster vaccination stimulates an increase in cellular Spn-specific immunity following three primary immunizations. Given the limited effect of dose 3 compared to dose 2 on the observed cellular immune response, the suggestion from this study is that a 2 + 1 vaccine schedule may be more similar to a 3 + 1 schedule than the alternative 3 + 0 option. These findings have implications for determining efficient vaccine schedules that take into account both cellular and humoral immunity as aspects of protection.
Disclosure of potential conflicts of interest
No potential conflicts of interest were disclosed.
Supplemental Material
Download MS Power Point (697.9 KB)Acknowledgments
We thank all the nurses and staff of pediatric practices, Dr Janet Casey, the parents who consented, and the children who participated in study sample collections. We thank lab members Saleem Basha, Lukas Pfannenstiel, Jill Mangiafesto, Tom Geiger, and Jareth Wischmeyer for technical help.
Supplementary material
Supplemental data for this article can be accessed online at http://dx.doi.org/10.1080/21645515.2020.1753438.
Additional information
Funding
References
- Avery OT, Goebel WF. Chemo-immunological studies on conjugated carbohydrate-proteins: V. The immunological specificity of an antigen prepared by combining the capsular polysaccharide of type III pneumococcus with foreign protein. J Exp Med. 1931;54:437–47. doi:https://doi.org/10.1084/jem.54.3.437.
- Guttormsen HK, Sharpe AH, Chandraker AK, Brigtsen AK, Sayegh MH, Kasper DL. Cognate stimulatory B-cell-T-cell interactions are critical for T-cell help recruited by glycoconjugate vaccines. Infect Immun. 1999;67:6375–84. doi:https://doi.org/10.1128/IAI.67.12.6375-6384.1999.
- American Academy of Pediatrics Committee on Infectious Diseases: Haemophilus influenzae type b conjugate vaccines: recommendations for immunization of infants and children 2 months of age and older: update. Pediatrics. 1991;88:169–72.
- Madore DV, Johnson CL, Phipps DC, Popejoy LA, Eby R, Smith DH. Safety and immunologic response to Haemophilus influenzae type b oligosaccharide-CRM197 conjugate vaccine in 1- to 6-month-old infants. Pediatrics. 1990;85:331–37.
- Pilishvili T, Lexau C, Farley MM, Hadler J, Harrison LH, Bennett NM, Reingold A, Thomas A, Schaffner W, Craig A, et al. Sustained reductions in invasive pneumococcal disease in the era of conjugate vaccine. J Infect Dis. 2010;201:32–41. doi:https://doi.org/10.1086/648593.
- Whitney CG, Pilishvili T, Farley MM, Schaffner W, Craig AS, Lynfield R, Nyquist A-C, Gershman KA, Vazquez M, Bennett NM, et al. Effectiveness of seven-valent pneumococcal conjugate vaccine against invasive pneumococcal disease: a matched case-control study. Lancet (London, England). 2006;368:1495–502. doi:https://doi.org/10.1016/S0140-6736(06)69637-2.
- Eskola J, Kilpi T, Palmu A, Jokinen J, Haapakoski J, Herva E, Herva E, Takala A, Käyhty H, Karma P, et al. Efficacy of a pneumococcal conjugate vaccine against acute otitis media. N Engl J Med. 2001;344:403–09. doi:https://doi.org/10.1056/NEJM200102083440602.
- Black SB, Shinefield HR, Ling S, Hansen J, Fireman B, Spring D, Noyes J, Lewis E, Ray P, Lee J, et al. Effectiveness of heptavalent pneumococcal conjugate vaccine in children younger than five years of age for prevention of pneumonia. Pediatr Infect Dis J. 2002;21:810–15. doi:https://doi.org/10.1097/00006454-200209000-00005.
- Esposito S, Principi N. Pneumococcal vaccines and the prevention of community-acquired pneumonia. Pulm Pharmacol Ther. 2015;32:124–29. doi:https://doi.org/10.1016/j.pupt.2014.02.003.
- O’Brien KL, Millar EV, Zell ER, Bronsdon M, Weatherholtz R, Reid R, Becenti J, Kvamme S, Whitney C, Santosham M. Effect of pneumococcal conjugate vaccine on nasopharyngeal colonization among immunized and unimmunized children in a community-randomized trial. J Infect Dis. 2007;196:1211–20. doi:https://doi.org/10.1086/521833.
- Käyhty H, Auranen K, Nohynek H, Dagan R, Mäkelä H. Nasopharyngeal colonization: a target for pneumococcal vaccination. Expert Rev Vaccines. 2006;5:651–67. doi:https://doi.org/10.1586/14760584.5.5.651.
- Papadatou I, Piperi C, Alexandraki K, Kattamis A, Theodoridou M, Spoulou V. Antigen-specific B-cell response to 13-valent pneumococcal conjugate vaccine in asplenic individuals with β-thalassemia previously immunized with 23-valent pneumococcal polysaccharide vaccine. Clin Infect Dis. 2014;59:862–65. doi:https://doi.org/10.1093/cid/ciu409.
- Stein KE. Thymus-independent and thymus-dependent responses to polysaccharide antigens. J Infect Dis. 1992;165(Suppl):S49–52. doi:https://doi.org/10.1093/infdis/165-supplement_1-s49.
- WHO Publication. Pneumococcal vaccines WHO position paper – 2012 – recommendations. Vaccine. 2012;30:4717–18. doi:https://doi.org/10.1016/j.vaccine.2012.04.093.
- Conklin L, Loo JD, Kirk J, Fleming-Dutra KE, Deloria Knoll M, Park DE, Goldblatt D, O’Brien KL, Whitney CG. Systematic review of the effect of pneumococcal conjugate vaccine dosing schedules on vaccine-type invasive pneumococcal disease among young children. Pediatr Infect Dis J. 2014;33(Suppl 2):S109–18. doi:https://doi.org/10.1097/INF.0000000000000078.
- Kieninger DM, Kueper K, Steul K, Juergens C, Ahlers N, Baker S, Jansen KU, Devlin C, Gruber WC, Emini EA, et al. Safety, tolerability, and immunologic noninferiority of a 13-valent pneumococcal conjugate vaccine compared to a 7-valent pneumococcal conjugate vaccine given with routine pediatric vaccinations in Germany. Vaccine. 2010;28:4192–203. doi:https://doi.org/10.1016/j.vaccine.2010.04.008.
- Esposito S, Tansey S, Thompson A, Razmpour A, Liang J, Jones TR, Ferrera G, Maida A, Bona G, Sabatini C, et al. Safety and immunogenicity of a 13-valent pneumococcal conjugate vaccine compared to those of a 7-valent pneumococcal conjugate vaccine given as a three-dose series with routine vaccines in healthy infants and toddlers. Clin Vaccine Immunol. 2010;17:1017–26. doi:https://doi.org/10.1128/CVI.00062-10.
- van Westen E, Wijmenga-Monsuur AJ, van Dijken HH, van Gaans-van den Brink JAM, Kuipers B, Knol MJ, Berbers GAM, Sanders EAM, Rots NY, van Els CA. Differential B-cell memory around the 11-month booster in children vaccinated with a 10- or 13-valent pneumococcal conjugate vaccine. Clin Infect Dis. 2015;61:342–49. doi:https://doi.org/10.1093/cid/civ274.
- Casey JR, Block S, Puthoor P, Hedrick J, Almudevar A, Pichichero ME. A simple scoring system to improve clinical assessment of acute otitis media. Clin Pediatr (Phila). 2011;50:623–29. doi:https://doi.org/10.1177/0009922811398391.
- Casey JR, Adlowitz DG, Pichichero ME. New patterns in the otopathogens causing acute otitis media six to eight years after introduction of pneumococcal conjugate vaccine. Pediatr Infect Dis J. 2010;29:304–09. doi:https://doi.org/10.1097/INF.0b013e3181c1bc48.
- Kaur R, Adlowitz DG, Casey JR, Zeng M, Pichichero ME. Simultaneous assay for four bacterial species including Alloiococcus otitidis using multiplex-PCR in children with culture negative acute otitis media. Pediatr Infect Dis J. 2010;29:741–45. doi:https://doi.org/10.1097/INF.0b013e3181d9e639.
- Kaur R, Casey JR, Pichichero ME. Serum antibody response to three non-typeable Haemophilus influenzae outer membrane proteins during acute otitis media and nasopharyngeal colonization in otitis prone and non-otitis prone children. Vaccine. 2011;29:1023–28. doi:https://doi.org/10.1016/j.vaccine.2010.11.055.
- Khaskhely N, Mosakowski J, Thompson RS, Khuder S, Smithson SL, Westerink MAJ. Phenotypic analysis of pneumococcal polysaccharide-specific B cells. J Immunol. 2012;188:2455–63. doi:https://doi.org/10.4049/jimmunol.1102809.
- Pichichero ME, Casey JR, Almudevar A, Basha S, Surendran N, Kaur R, Morris M, Livingstone AM, Mosmann TR. Functional immune cell differences associated with low vaccine responses in infants. J Infect Dis. 2016;213:2014–19. doi:https://doi.org/10.1093/infdis/jiw053.
- Henckaerts I, Goldblatt D, Ashton L, Poolman J. Critical differences between pneumococcal polysaccharide enzyme-linked immunosorbent assays with and without 22F inhibition at low antibody concentrations in pediatric sera. Clin Vaccine Immunol. 2006;13:356–60. doi:https://doi.org/10.1128/CVI.13.3.356-360.2006.
- Concepcion NF, Frasch CE. Pneumococcal type 22f polysaccharide absorption improves the specificity of a pneumococcal-polysaccharide enzyme-linked immunosorbent assay. Clin Diagn Lab Immunol. 2001;8:266–72. doi:https://doi.org/10.1128/CDLI.8.2.266-272.2001.
- Wernette CM, Frasch CE, Madore D, Carlone G, Goldblatt D, Plikaytis B, Benjamin W, Quataert SA, Hildreth S, Sikkema DJ, et al. Enzyme-linked immunosorbent assay for quantitation of human antibodies to pneumococcal polysaccharides. Clin Diagn Lab Immunol. 2003;10:514–19. doi:https://doi.org/10.1128/cdli.10.4.514-519.2003.
- Pichichero ME. Ten-year study of acute otitis media in Rochester, NY. Pediatr Infect Dis J. 2016;35:1027–32. doi:https://doi.org/10.1097/INF.0000000000001216.
- Sharma SK, Casey JR, Pichichero ME. Reduced serum IgG responses to pneumococcal antigens in otitis-prone children may be due to poor memory B-cell generation. J Infect Dis. 2012;205:1225–29. doi:https://doi.org/10.1093/infdis/jis179.
- Pichichero ME, Casey JR, Almudevar A. Nonprotective responses to pediatric vaccines occur in children who are otitis prone. Pediatr Infect Dis J. 2013;32:1163–68. doi:https://doi.org/10.1097/INF.0b013e31829e887e.
- Shi Y, Agematsu K, Ochs HD, Sugane K. Functional analysis of human memory B-cell subpopulations: IgD+CD27+ B cells are crucial in secondary immune response by producing high affinity IgM. Clin Immunol. 2003;108:128–37. doi:https://doi.org/10.1016/s1521-6616(03)00092-5.
- Basha S, Kaur R, Mosmann TR, Pichichero ME. Reduced T-helper 17 responses to Streptococcus pneumoniae in infection-prone children can be rescued by addition of innate cytokines. J Infect Dis. 2017;215:1321–30. doi:https://doi.org/10.1093/infdis/jix090.
- Waldrop SL, Davis KA, Maino VC, Picker LJ. Normal human CD4+ memory T cells display broad heterogeneity in their activation threshold for cytokine synthesis. J Immunol. 1998;161:5284–95.
- Lanzavecchia A, Sallusto F. Human B cell memory. Curr Opin Immunol. 2009;21:298–304. doi:https://doi.org/10.1016/j.coi.2009.05.019.