ABSTRACT
Owing to their increased susceptibility to influenza infection, HIV+ individuals are recommended to receive annual influenza vaccination. However, influenza vaccination induced production of anti-influenza neutralization antibodies (Nab) is successful only in some viral-suppressed antiretroviral therapy (ART) treated HIV+ subjects. Additionally, the mechanism of antibody response induced by influenza vaccine in antiretroviral-treated HIV+ subjects is unclear. In this study, we conducted a cohort study which contains 40 HIV+ ART-treated individuals to whom one dose of seasonal influenza vaccine was administered. Blood samples were collected on day 0, 7, 14, and 28 post-vaccination, and serologic responses were characterized by ELISA and micro-neutralization to measure the total antibodies and Nab against influenza vaccines. Transcriptional profiling of peripheral blood mononuclear cells (PBMCs) and immunological assays was measured. Increased levels of proliferation of CD4+T cells and B cells with their corresponding subtypes were observed in HIV-infected subjects at day 7 (D7) following vaccination compared to pre-vaccination. Moreover, proliferation of CD4+T cells and B cells (D7) was correlated with influenza-specific H1N1 Nab at day 28 (D28). Our study could also demonstrate that apoptosis of CD4+T cells and B cells (D7) were inversely correlated with influenza-specific H1N1 Nab. Based on the Nab response after vaccination to each influenza subtypes (D28), HIV+ subjects were stratified as influenza vaccine responders and influenza vaccine non-responders (“responders” ≥ 4-fold increase from day 0; “non-responders” < 4-fold increase from day 0). A selected list of biological pathways (H1N1and H3N2: olfactory transduction, B: phagosome) enriched with transcripts were significantly altered in (ART) treated HIV+ subjects among Nab production responders. This study demonstrated a more detailed mechanism of immune regulation on influenza induced antibody response and revealed some knowledge regarding bioinformatics of vaccine responders and non-responder in influenza induced antibody production in ART-treated HIV patients.
Introduction
People with Human Immunodeficiency Virus (HIV) are highly susceptible to influenza-related morbidity and mortality, even though, the emergence of antiretroviral therapy (ART) has greatly reduced HIV-related morbidity and mortality.Citation1 HIV-positive people remain at increased risk of acquiring infectious diseases including influenza due to deficiencies in both humoral and cell-mediated immunity.Citation2 Seasonal influenza vaccination is the most effective method of preventing influenza infection and is highly recommended for all HIV-infected individuals.Citation1–3 Although, the gold standard for measuring antibody-based immunity for influenza viruses mainly depends on the hemagglutinin inhibition assay (HAI) and to a lesser extent the microneutralization assay (MN), neutralizing Ab is presumed to provide vaccine-induced protection and is important to resist the influenza infection. Previously study found broadly neutralizing antibodies induced by influenza vaccine can provide protection against lethal, heterologous virus challenge in vivo.Citation4–6 Passive injection of the anti-hemagglutinin (HA) neutralization antibody is proven to protect the mice against influenza virus infection.Citation7,Citation8 Such neutralization effect would stop the influenza virus infection, and eliciting neutralizing antibodies that recognize epitopes on the HA from different influenza subtypes would protect individuals from influenza and hence, prevent the spread of epidemic among populations.Citation9–11 However, the immune response to influenza vaccination is different in each individual. Understanding the variations in immune response following a vaccination is challenging but would be essential to give better protection to HIV + individuals.
As we know, with the help of follicular helper CD4+T (Tfh) cells, B cells in the germinal center undergo somatic hyper-mutation and affinity maturation. Consequently, memory B cells quickly shift from secreting low affinity neutralization antibodies to secreting antibodies of high affinity that can cross-react with the pandemic strain.Citation12,Citation13 Previous studies have shown that exposure to microbial elements induced innate immune activation might account for patients with poor CD4+T cell recovery under viral suppressive ART treatment.Citation14 Microbial translocation, such as Staphylococcus translocation, was been found to enhance germinal center response and induce autoantibody production.Citation15 Powell AM et al. found that HIV-infected individuals have impaired CD80 induction on memory B cells and CD40L induction on memory CD4+T cells in both responders and non-responders.Citation16 B cell function is correlated with measures of memory humoral immunity in response to seasonal influenza vaccination in healthy controls but not in ART-treated patients.Citation17 However, the immune responses to different influenza strains and the bioinformatics of responders and non-responder in influenza induced antibody production in ART-treated HIV patients are still unknown.
In the present study, we tried to investigate anti-influenza neutralization antibody production on ART-treated HIV+ individuals. Therefore, we performed CD4+T cell and B cell functional assays. And a baseline PBMCs (pre-vaccination) and day 7 (post-vaccination) were performed using microarray analysis. Moreover, computational analyzes were used to identify the enriched gene expression modules that were related to the Nab, and determine how transcriptomic profiles were correlated with immune responses and Nab production in ART-treated HIV+ individuals. The main aim of this study is to characterize how immune response affects the production of neutralization antibody in ART-treated HIV+ individuals and try to find some genetic clues in different vaccine response groups (responders and non-responders).
Materials and methods
Study subjects
This study was approved by the Institutional Review Board at Tongji University. All participants provided written informed consents. In the present study, 40 HIV+ individuals were enrolled to receive the 2016–2017 seasonal influenza vaccine [A/California/7/2009 (H1N1); A/Hong Kong/4801/2014 (H3N2); B/Brisbane/60/2008 (influenza B virus)]. All participants had received influenza vaccination in the previous year, and had been on ART treatment for at least 2 years with undetectable viral load (plasma HIV RNA < 50 copies/mL). Blood samples were collected on day 0 (D0) before vaccination, day 7 (D7), day 14 (D14), and day 28 (D28) post-vaccination. The clinical characteristics of participants are shown in .
Table 1. Clinical characteristics of HIV+ individuals.
Flow cytometry
Whole blood was collected in EDTA-containing tubes for plasma isolation and stored at −80°C. PBMCs were isolated from whole blood over a Ficoll-Paque. The freshly isolated PBMCs were used for determining the percentage of apoptosis, and proliferation of CD4+T cells and B cells. For surface staining, antibodies were incubated with PBMCs at 4°C for 30 min. After surface staining, the cells were washed and analyzed by flow cytometry. The fluorochrome-labeled monoclonal antibodies used in this study included the following: anti-human CD3 (OKT3), anti-human CD4 (OKT4), anti-human CD8 (RPA-T8), anti-human CD19 (SJ25C1), anti-human CD27 (M-T271), anti-human CD38 (HIT2), anti-human CD45RA (HI100), anti-human CD138 (MI15), anti-Ki-67 (B56), annexin V (BD Pharmingen, San Jose, CA), and isotype control antibodies (BD Pharmingen). Cells were collected in a BD FACSVerse flow cytometer (BD Pharmingen), and data were analyzed by FlowJo software (Version 10.4.2).
Microneutralization assay
The influenza virus stocks of H1N1, H3N2, and influenza B virus which corresponded to the 2016–2017 seasonal influenza vaccine strains were prepared in Madin–Darby canine kidney (MDCK) cells (ATCC, Manassas, VA). Plasma was heat-inactivated at 56°C for 30 minutes, and was diluted from 1:10 with PBS followed by 2-fold serial dilution. Influenza virus solution that was standardized to 100x 50% tissue culture infective dose/ml (TCID50) was added to 96 well plates containing heat inactivated plasma to which 1 µg/ml tosyl-phenylalanyl-chloromethyl-ketone (TPCK) treated Trypsin (Sigma, St. Louis, MO) was added, and then the mixture was incubated at 37°C in 5% CO2 for 60 min. MDCK cells were added at 5x104cells/well and cultured for 18 h. An ELISA was then performed as previously described.Citation18
Microarray analyses
Isolation and purification of total RNA from PBMCs were performed using the RNeasy Plus Mini Kit (Qiagen, Hilden, Germany) in accordance with the manufacturer’s instructions. RNA was quantitated on a Nanodrop 1000 (NanoDrop Technologies, Wilmington, DE) and its integrity was determined using Agilent 2100 Bioanalyzer (Agilent Technologies, Santa Clara, CA). Affymetrix Human GeneChip U133 Plus 2.0 Array (Affymetrix, Santa Clara, CA) was used for the microarray hybridization according to the manufacturer’s protocol. All microarray data analyses and statistical analyses were performed by using the R language and environment (http://www.r-project.org/) for statistical computing.
Differential gene expression and pathway analysis
Robust Multichip Average (RMA) normalization was performed, which included background adjustment and quantile normalization. The patterns of differential gene expression between the study groups were evaluated by generating linear models and moderated t-statistic or ANOVA with the package Limma.Citation19 Empirical Bayes moderation and Benjamini and Hochberg false-discovery rate (FDR) corrections for multiple tests were employed for the differentially expressed probes. The selection criteria of differentially expressed genes were defined with fold-difference >1.2 and P-value < 0.05. Both the fold-difference and P-value cutoffs were set in order to avoid over-estimation of the differentially expressed probes or penalizing particular transcriptional profiles in pathway analyses. Gene function analysis was performed based on gene ontology (GO, http://geneontology.org/), whereas pathway enrichment analysis was based on Kyoto Encyclopedia of Genes and Genomes (KEGG, https://www.genome.jp/kegg/) and Reactome (https://reactome.org/). The protein-to-protein interactions of different gene expressions were based on STRING (https://string-db.org/).
Weighted gene co-expression network analysis
To identify co-expressed or co-regulated genes associated with the Nab production, the weighted gene coexpression network analysis (WGCNA) package was performed on an iMac with 64 G memory. We then used the 31,718 present genes and applied the R implementation of WGCNA. We performed WGCNA clustering using the ‘blockwiseModules’ function, and the soft threshold power was 7 (as established by scale-free topology network criteria), and minimum module size of 30. Associations of individual genes with the trait of different subtypes of Nab production after vaccination were quantified by determining the gene significance which was defined as the absolute value of the correlation between the gene and the trait. A list of identifiers was performed for gene function and pathway analysis.
Statistical analysis
Data analysis was performed using R or Prism 6.0 (GraphPad Software, La Jolla, CA). The differences between the groups were compared using one-way ANOVA, Mann–Whitney’s U test (unpaired), or Wilcoxon matched-pairs signed rank t-test as appropriate. To explore associations between pairs of continuous variables, Spearman’s correlation test was applied. P value of less than 0.05 was considered as statistically significant.
Results
Production of neutralization antibodies in HIV+ individuals after influenza vaccination
A total of 40 ART-treated HIV+ subjects were included in the current study. The clinical characteristics are shown in . Neutralizing antibodies were considered as protective against influenza viral infection. To determine the magnitude of Ab response to influenza vaccine, we measured influenza-specific Nabs using viral micro-neutralization assays. As compared to baseline, seasonal influenza vaccination significantly induced neutralizing antibodies against H1N1, B and H3N2 strains in the HIV+ individuals (P < .0001, P = .023, P < .0001, respectively, ). Nevertheless, Nab responses elicited by influenza showed substantial difference among influenza subtype strains, and only certain proportion of individuals (H1N1:40%, H3N2:22.5%, B:37.5%) showed increased level of Nab after receiving influenza vaccination ().
Cellular response after vaccination and its correlation with anti-influenza neutralization antibodies production in HIV+ individuals
To determine whether the cellular responses elicited by vaccination would affect the Nab production, we analyzed apoptosis and proliferation of B cells and CD4+T cells. We chose day 7 (D7) as the cellular response collection time point after vaccination, because the peak influenza-specific IgG+ antibody-secreting plasma cell (ASC) response were found at approximately day 7.Citation20 There was no difference between pre-vaccination (D0) and post-vaccination (D7) in the frequency of apoptotic B cells, CD4+T cells, the subsets of B cells, and the subsets of CD4+T cells in PBMCs (). The expression of Ki-67, a marker of proliferation in T cells and B cells, was elevated due to the cellular responses to influenza vaccine. After influenza vaccination, the percentage of Ki-67 positive total CD4+T cells and Ki-67 positive subtypes of CD4+T cells, which included central memory CD4+T cells (cmCD4+, CD45RA-CCR7+), Effector/Effector memory CD4+T cells (Eff/EM CD4+, CD45RA-CCR7-), and transitional memory CD4+T cells (tmCD4+, CD45RA+CCR7-), were increased significantly (), but not naïve CD4+T cells (nCD4+, CD45RA+CCR7+). The levels of Ki-67 positive total B cells, naïve B cells (CD3-CD19+CD27-), and memory B cells (CD3-CD19+CD27+) were increased significantly in HIV+ individuals ().
Figure 2. Cellular immune responses to vaccination are related to influenza Nab production in HIV+ individuals. (A) Dot plots from a representative donor show subsets of CD4+T cells, B subset (naïve, memory), Annexin V and Ki67 staining. (B) Frequencies of AnnexinV+ total CD4+T cells and before (D0) and after (D7) vaccination. (C) Frequencies of AnnexinV+ total B cells and subsets of B cells before (D0) and after (D7) vaccination. (D) Intracellular Ki-67 expression in total CD4+T cells and subsets of CD4+T cells before (D0) and after (D7) vaccination. (E) Intracellular Ki-67 expression in total B cells and subsets of B cells before (D0) and after (D7) vaccination. (F) Correlation analysis between cellular immune response on day 7 (D7) and Nab production on day 28 or Nab fold change (D28/D0) in HIV-infected subjects. (G) Correlation analysis between AnnexinV expression in CD4+T cells or B cells on D7 and the fold change (D28/D0) of Nab against influenza H1N1 subtype in HIV+ subjects. (H) Correlation analysis between Ki-67 expression in CD4+T cells or B cells on D7 and the Nab against influenza H1N1 subtype on D28 in HIV+ subjects. Wilcoxon matched-pairs signed rank t-test and Spearman’s correlation test.
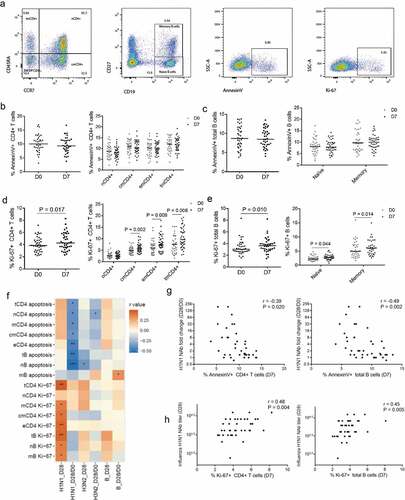
Next, we questioned whether the Nab production after vaccination would be related to the cellular immune response that we observed. Hence, we investigated the relationship between proliferation and apoptosis of B cells and CD4+T cells (D7) and plasma levels of each subtype of Nab (D28) after vaccination. Surprisingly, expression of Ki-67 in CD4+T and B cells (D7) was correlated with anti-H1N1 influenza Nab (D28) but not with anti-H3N2 or with anti-B of Nab after-vaccination (). Moreover, the percentages of apoptotic B cells, CD4+T cells, and CD4+T cell subtypes were inversely correlated with the fold change of anti-H1N1 influenza Nab after vaccination (). Again, we did not observe any relationship between apoptotic B cells or apoptotic CD4+T cells at D7 after vaccination with the fold change in anti-H3N2 or anti-B s influenza Nab ().
Transcriptional signatures in Nab responder compared with non-responder
To study the mechanism of neutralization antibody production in HIV+ individuals after influenza vaccination, we examined the gene expression of PBMCs from HIV+ subjects with neutralization antibody responders (≥4-fold change of Nab at D28/D0) and non-responders (<4-fold change of Nab at D28/D0). In many previous studies, the seroresponse was defined as a ≥ 4-fold increase in neutralization titer, Citation21–23 so we also used this stratification criterion. RNA was extracted from a freshly isolated PBMCs on D0 and D7, with a total of 24 samples tested, including neutralization antibody responders (against H1N1, n = 4; H3N2, n = 4; B, n = 3) and non-responders (against H1N1, n = 8; H3N2, n = 8; B, n = 9) on D0 and D7 post-vaccination. Subsequently, their transcriptomes were analyzed using Affymetrix arrays.
In the next step, we compared the number of differential expression genes (DEGs) (D7 compare to D0, P < .05) from neutralization antibody responders with the DEGs from non-responders, in which extensive transcriptional changes were induced by influenza vaccination. A total of 633 genes were identified as significantly up- (374 genes) or downregulated (259 genes) in the responders where influenza vaccine successfully induced anti-H1N1subtype influenza Nab (H1N1 responders); and 742 genes were significantly up- (392 genes) or downregulated (350 genes) in the responders on whom influenza vaccine successfully induced anti-H3N2 subtype influenza Nab (H3N2 responders); whereas 421 genes were significantly up- (274 genes) or downregulated (147 genes) in the responders where influenza vaccine successfully induced anti-B influenza Nab (B responders). In contrast, much fewer DEGs were observed in the non-responders. In the non-responders, influenza vaccine failed to induce Nab against the following different influenza antigens; H1N1 subtype influenza antigens, H3N2 subtype influenza antigens and B influenza antigens that showed either up-regulation or down-regulation (172 genes, 133 genes and 175 genes, correspondingly). These findings indicated that much more genes were activated in the Nab responders as opposed to the Nab non-responders.
To better assess the DEGs variations of Nab among HIV+ individuals, we performed pathway analyses using KEGG (Kyoto Encyclopedia of Genes and Genomes) biological pathways to annotate and characterize genes expressed differently in the responders and non-responders. Vaccine-induced high or low Nab production against different subtype strains was associated with differentially expressed genes. A selected list of biological pathways enriched with transcripts were significantly altered in HIV+ subjects with neutralization antibody responder (). In H1N1 responders group, the most altered pathways following vaccination included the olfactory transduction (P = 2.5e-6), retrograde endocannabinoid signaling (P = .002), and serotonergic synapse (P = .003). In H3N2 responders group, the pathways with greatest change included the olfactory transduction (P = 1.5e-6) and cytosolic DNA-sensing pathway (P = .01). In B responders group, the pathways with greatest alteration included the phagosome (P = .006) and pancreatic secretion (P = .02). The hematopoietic cell lineage response gene pathway was enriched in the non-responders of influenza H1N1 and H3N2 ().
Table 2. The identified gene expression pathways from Kyoto Encyclopedia of Genes and Genomes (KEGG).
Transcriptomic modules recovery of the expression of the Nab related gene
Although comparing the DEGs at single gene level was good for the discovery of critical regulatory genes, a large number of comparisons would generate noise and might lack the power to detect the gene networks at transcriptional profiling levels. In order to investigate the genes that were associated with Nab production after vaccination, we applied the weighted gene co-expression network analysis (WGCNA) to find clusters (modules) of highly correlated genes algorithm. Here, the transcriptional clusters were used to determine specific gene expression patterns associated with particular immunological responses. The overall degree of correlation between the Nab and the clustered gene module was calculated of which nine modules showed correlation with Nab production after vaccination or the fold change of Nab induced by the vaccine (). To each type of Nab, the highest correlation modules were the MEslateblue module with influenza H3N2 Nab (D28) (r = 0.83, p = 8e-4) and MEoranged module with influenza B Nab fold change after vaccination (D28/D0) (r = −0.83, p = 8e-4), respectively. Importantly, MEoranged module was inversely correlated with influenza H1N1 and H3N2 Nab production after vaccination (r = −0.59, P = .05; r = −0.6, P = .01, respectively).
Figure 3. Gene co-expression modules in blood transcriptional profiles related to Nab production. (A) Gene set enrichment analysis of gene modules identified with WGCNA. Several significant module-trait associations are shown. (B) Gene ontolog gene function clustering analysis of each significant module.
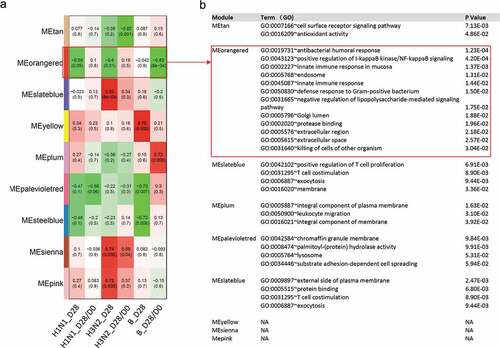
In the next step, gene ontology enrichment analysis was performed to study the functional significance of each module. The functional significance of the modules was examined by testing the enriched functions of genes in the modules. MEoranged and MEslateblue modules were strongly associated with influenza H3N2, H1N1, and B Nab response, thus we eventually focused on them. The MEslateblue module, which had direct correlation with influenza H3N2 Nab after vaccination was significantly enriched for genes in the regulation of T cells proliferation (P = .007) and T cell costimulation (P = .009). The MEoranged module, which had inverse correlation with influenza H1N1, H3N2, and B after vaccination, was significantly enriched for genes in antibacterial-related response (P = .0001) ().
Discussion
HIV-infected patients possess increased risk for influenza virus infections and are highly recommended for influenza vaccination. The aim of this study is to characterize how immune response affects the production of neutralization antibody in ART-treated HIV+ individuals and also to find some genetic clues in different vaccine response groups (responders and non-responders). We found that only a portion of the individuals (H1N1:40%, H3N2:22.5%, B:37.5%) showed increased level of Nab after receiving influenza vaccination. Moreover, anti-influenza vaccine (H1N1) responses were correlated with CD4 T-cell and B-cell proliferation and inversely correlated with apoptosis of these cells in ART-treated HIV patients. A selected list of biological pathways (H1N1and H3N2: olfactory transduction, B: phagosome) enriched with transcripts were significantly altered in influenza induced antibody production. The hematopoietic cell lineage response gene pathway was enhanced in influenza H1N1 and H3N2 non-responders.
Studies in human and animal models indicate that HIV infection affects the germinal center reaction, increases immune activation/exhaustion of lymphocytes, and results in qualitative deficiency of T and B cell functions.Citation24,Citation25 Influenza vaccine studies have provided a valuable model system to analyze the immune system in vaccine-induced antibody .Citation26 Anna M. Powell reported that 9 of 26 (34.6%) HIV-infected individuals and 7 of 16 (43.8%) healthy controls were classified as responders to influenza vaccines.Citation16 Similarly, we found increased levels of Nab after receiving influenza vaccination only in certain proportion of individuals.
Many previous studies have reported a significant loss of circulating pTfh cells in chronic viremic HIV-infected subjects as compared to HIV-uninfected people.Citation27,Citation28 Twelve months of ART that incorporates Raltegravir resulted in elevated number of pTfh cells.Citation28 However, pTfh cells from HIV+ virologically suppressed patients on ART exhibited functional impairment in their ability to provide adequate B cell help in a number of systems.Citation29,Citation30 Eirini Moysi reported that post-vaccination lymph node samples in HIV+ individuals had significantly reduced Tfh cell numbers compared with pre-vaccination samples, with no evidence of peripheral Tfh (pTfh) cell reduction. Vaccination is believed to induce relevant memory B cell populations at a tissue level in ART-treated HIV+ subjects.Citation31 Consistent with a report from Luo et al., we identified decreased absolute counts of peripheral CD4+T cell and increased frequencies of proliferating and apoptotic B cells at baseline in HIV-infected subjects relative to healthy controls.Citation17 In addition to that, we found that only H1N1 D28 correlated with Ki-67 and not H1N1 D28/D0 in ART-treated HIV patients. Ki-67 was used as a marker of proliferation and cell cycling that response to immunization. Only the H1N1 D28 correlated with Ki-67 but not H1N1 D28/D0 of our results suggested H1N1 induced neutralization antibody might not relate to the baseline expression of Ki-67. The vaccine-induced T cells and B cells proliferation and cell cycling might facilitate to the neutralization antibody production. Another interesting result is that we observed only H1N1 D28/D0 correlated with apoptosis and not H1N1 D28. This may because even after long-term ART treatment, the HIV+ individuals still had higher apoptosis of T cells and B cells when compared with healthy control. The apoptosis of T cells and B cells might impair the immune response induced by vaccination. We speculated that more heightened apoptosis at baseline, more heavy impaired response may generate after vaccination, which means that the baseline level of apoptosis could affect the further immune response. Interestingly, we demonstrated that only H1N1 NAb responses were correlated with apoptosis and increased Ki-67 but not with H3N2 and Influenza B Nab responses. Although, they were mostly influenza strains, many studies found that H1N1 could induce expansion of pTfh cells with secretion of IL-21 that could regulate proliferation of T cells, B cells and NK cells.Citation32,Citation33 However, there is little in literature about immune responses induced by H3N2 & B Influenza vaccination. Further research is needed in the future to deeply explore the immune responses of different vaccines.
By performing gene expression profiles from PBMCs before and after vaccination, we analyzed the DEGs from HIV-infected subjects and found that different gene pathways were altered by different influenza strains. Hence, olfactory transduction gene alteration was observed both in influenza H1N1 and H3N2 Nab HIV+ responders after vaccination. Previous studies showed that cyclic AMP (cAMP) was essential in the olfactory transduction, Citation34,Citation35 where it remains to be an important signal in the induction of antibody production by B cells.Citation36 The cAMP response element-binding protein transcription complex could regulate the function of lymphocytes via TRAF3102 in a powerful and multitalented manner.Citation37 The specific role of the olfactory transduction gene pathway on influenza induced Nab production requires further investigation. In order to obtain additional perspective at transcriptional profiles level, we explored WGCNA to study the Nab response with the co-expression network. Some gene expression modules exhibited a high score with Nab production. The regulation of T cell proliferation and T cell costimulation was found to be associated with H3N2 Nab production. Furthermore, the antibacterial co-expression genes were inversely related to the Nab against influenza H1N1, H3N2, and B. Bacterial infections have increased prevalence in patients with HIV infection.Citation37–39 The fragments or products of bacteria stimulated the immune cells, such as monocyte, macrophage, or directly the B cells which would impair the vaccine-induced immune response. In this study, the antibacterial co-expression genes were enriched, and apoptosis of B cells and CD4+T cells were inversely related to the Nab production, indicating that the bacterial infection might impair the protective immune response in HIV+ individuals after vaccination.
There were some limitations of the present study. Firstly, the finding in this study may not be extrapolated to other groups of population such as healthy people or HIV individuals without ART-treatment. Secondly, among the antibody-production-related genes obtained through bioinformatics analysis, the genes with high correlation could be selected to further explore the detailed function in antibody-production after influenza vaccination in ART- treated HIV patients.
In conclusion, our study demonstrated that seasonal influenza vaccination effectively induced protective Nab response only in some of the ART-treated HIV+ individuals. The apoptosis and proliferation of CD4+T cells and B cells at D7 after vaccination were related to the delayed humoral immune response. Additionally, we identified that gene expression signatures associated with immunological responses and regulatory networks were involved in response to vaccination. Our study may provide useful information for increased vaccine efficacy.
Disclosure of potential conflicts of interest
No potential conflicts of interest were disclosed.
Authorship
Shenghui Qin conceived of and directed the project, wrote the manuscript. Xia Ying and Fuli Mi performed the laboratory sample testing and analyses. Xia Ying and Guoqiang Du were involved in critically revising the manuscript.
Accession numbers
Microarray data are available in GEO (Gene Expression Omnibus, https://www.ncbi.nlm.nih.gov/geo/query/acc.cgiacc=348922) with the accession number E-MTAB-8084.
Correction Statement
This article has been corrected with minor changes. These changes do not impact the academic content of the article.
References
- Kaplan JE, Benson C.et al. Guidelines for prevention and treatment of opportunistic infections in HIV-infected adults and adolescents: recommendations from CDC, the National Institutes of Health, and the HIV Medicine Association of the Infectious Diseases Society of America.MMWR Recomm Rep. 2009;58(RR-4):1-207.
- Tasker SA, Treanor JJ, Paxton WB, Wallace MR. Efficacy of influenza vaccination in HIV-infected persons: a randomized, double-blind, placebo-controlled trial. Ann Intern Med. 1999;131(6):430–33. doi:10.7326/0003-4819-131-6-199909210-00006.
- Fowke KR, DʼAmico R, Chernoff DN, Pottage JC, Benson CA, Sha BE, Kessler HA, Landay AL, Shearer GM. Immunologic and virologic evaluation after influenza vaccination of HIV-1-infected patients. Aids. 1997;11(8):1013–21. doi:10.1097/00002030-199708000-00010.
- Mallajosyula VV, Citron M, Ferrara F, Lu X, Callahan C, Heidecker GJ, Sarma SP, Flynn JA, Temperton NJ, Liang X, et al. Influenza hemagglutinin stem-fragment immunogen elicits broadly neutralizing antibodies and confers heterologous protection. Proc Nat Acad Sci. 2014;111(25):E2514–E2523. doi:10.1073/pnas.1402766111.
- Wei C-J, Yassine HM, McTamney PM, Gall JGD, Whittle JRR, Boyington JC, Nabel GJ. Elicitation of broadly neutralizing influenza antibodies in animals with previous influenza exposure. Sci Transl Med. 2012;4(147):147ra114–147ra114. doi:10.1126/scitranslmed.3004273.
- Yu X, Tsibane T, McGraw PA, House FS, Keefer CJ, Hicar MD, Tumpey TM, Pappas C, Perrone LA, Martinez O, et al. Neutralizing antibodies derived from the B cells of 1918 influenza pandemic survivors. Nature. 2008;455(7212):532–36. doi:10.1038/nature07231.
- Itoh Y, Yoshida R, Shichinohe S, Higuchi M, Ishigaki H, Nakayama M, Pham VL, Ishida H, Kitano M, Arikata M, et al. Protective efficacy of passive immunization with monoclonal antibodies in animal models of H5N1 highly pathogenic avian influenza virus infection. PLoS Pathog. 2014;10(6):e1004192. doi:10.1371/journal.ppat.1004192.
- DiLillo DJ, Tan GS, Palese P, Ravetch JV. Broadly neutralizing hemagglutinin stalk–specific antibodies require FcγR interactions for protection against influenza virus in vivo. Nat Med. 2014;20(2):143. doi:10.1038/nm.3443.
- Rajão DS, Pérez DR. Universal vaccines and vaccine platforms to protect against influenza viruses in humans and agriculture. Front Microbiol. 2018;9:123. doi:10.3389/fmicb.2018.00123.
- Wikramaratna PS, Sandeman M, Recker M, Gupta S. The antigenic evolution of influenza: drift or thrift? Philos Trans R Soc B. 2013;368(1614):20120200. doi:10.1098/rstb.2012.0200.
- Sautto GA, Kirchenbaum GA, Ross TM. Towards a universal influenza vaccine: different approaches for one goal. Virol J. 2018;15(1):17. doi:10.1186/s12985-017-0918-y.
- Minton K. B cell memory: a second chance for antibodies. Nat Rev Immunol. 2015;15(3):131–131. doi:10.1038/nri3829.
- Victora GD, Nussenzweig MC. Germinal centers. Annu Rev Immunol. 2012;30:429–57. doi:10.1146/annurev-immunol-020711-075032.
- Lederman MM, Calabrese L, Funderburg NT, Clagett B, Medvik K, Bonilla H, Gripshover B, Salata RA, Taege A, Lisgaris M, et al. Immunologic failure despite suppressive antiretroviral therapy is related to activation and turnover of memory CD4 cells. J Infect Dis. 2011;204:1217–26. doi:10.1093/infdis/jir507.
- Luo Z, Li M, Wu Y, Meng Z, Martin L, Zhang L, Ogunrinde E, Zhou Z, Qin S, Wan Z, et al. Systemic translocation of Staphylococcus drives autoantibody production in HIV disease. Microbiome. 2019;7(1):25. doi:10.1186/s40168-019-0646-1.
- Powell AM, Luo Z, Martin L, Wan Z, Ma L, Liao G, Song Y, Li X, Michael Kilby J, Huang L, et al. The induction of CD80 and apoptosis on B cells and CD40L in CD4+ T cells in response to seasonal influenza vaccination distinguishes responders versus non-responders in healthy controls and aviremic ART-treated HIV-infected individuals. Vaccine. 2017;35(5):831–41. doi:10.1016/j.vaccine.2016.12.026.
- Luo Z, Ma L, Zhang L, Martin L, et al. Key differences in B cell activation patterns and immune correlates among treated HIV-infected patients versus healthy controls following influenza vaccination. Vaccine. 2016;34:1945–1955.
- WHO. Manual for the laboratory diagnosis and virological surveillance of influenza.WHO Global Influenza Surveillance Network; 2011.
- Smyth GK, Speed T. Normalization of cDNA microarray data. Methods. 2003;31(4):265–73. doi:10.1016/S1046-2023(03)00155-5.
- Wrammert J, Smith K, Miller J, Langley WA, Kokko K, Larsen C, Zheng N-Y, Mays I, Garman L, Helms C, et al. Rapid cloning of high-affinity human monoclonal antibodies against influenza virus. Nature. 2008;453(7195):667–71. doi:10.1038/nature06890.
- Nayak JL, Fitzgerald TF, Richards KA, Yang H, Treanor JJ, Sant AJ. CD4+ T-cell expansion predicts neutralizing antibody responses to monovalent, inactivated 2009 pandemic influenza A (H1N1) virus subtype H1N1 vaccine. J Infect Dis. 2012;207(2):297–305. doi:10.1093/infdis/jis684.
- Malkin E, Yogev R, Abughali N, Sliman J, Wang CK, Zuo F, Yang C-F, Eickhoff M, Esser MT, Tang RS, et al. Safety and immunogenicity of a live attenuated RSV vaccine in healthy RSV-seronegative children 5 to 24 months of age. PLoS One. 2013;8(10):e77104. doi:10.1371/journal.pone.0077104.
- Chang Y-T, Guo C-Y, Tsai M-S, Cheng -Y-Y, Lin M-T, Chen C-H, Shen D, Wang J-R, Sung J-M. Poor immune response to a standard single dose non-adjuvanted vaccination against 2009 pandemic H1N1 influenza virus A in the adult and elder hemodialysis patients. Vaccine. 2012;30(33):5009–18. doi:10.1016/j.vaccine.2012.05.016.
- Perreau M, Savoye AL, De Crignis E, Corpataux JM, Cubas R, Haddad EK, De Leval L, Graziosi C, Pantaleo G. Follicular helper T cells serve as the major CD4 T cell compartment for HIV-1 infection, replication, and production. J Exp Med. 2013;210(1):143–56. doi:10.1084/jem.20121932.
- Lindqvist M, van Lunzen J, Soghoian DZ, Kuhl BD, Ranasinghe S, Kranias G, Flanders MD, Cutler S, Yudanin N, Muller MI, et al. Expansion of HIV-speci c T follicular helper cells in chronic HIV infection. J Clin Invest. 2012;122(9):3271–80. doi:10.1172/JCI64314.
- Herati RS, Reuter MA, Dol DV, Mans Eld KD, Aung H, Badwan OZ, Kurupati RK, Kannan S, Ertl H, Schmader KE, et al. Circulating CXCR5+PD-1+ response predicts influenza vaccine antibody responses in young adults but not elderly adults. J Immunol. 2014;193(7):3528–37. doi:10.4049/jimmunol.1302503.
- Boswell KL, Paris R, Boritz E, Ambrozak D, Yamamoto T, Darko S, Wloka K, Wheatley A, Narpala S, McDermott A, et al. Loss of circulating CD4 T cells with B cell helper function during chronic HIV infection. PLoS Pathog. 2014;10(1):e1003853. doi:10.1371/journal.ppat.1003853.
- Pallikkuth S, Fischl MA, Pahwa S. Combination antiretroviral therapy with raltegravir leads to rapid immunologic reconstitution in treatment-naive patients with chronic HIV infection. J Infect Dis. 2013;208(10):1613–23. doi:10.1093/infdis/jit387.
- Cubas R, van Grevenynghe J, Wills S, Kardava L, Santich BH, Buckner CM, Muir R, Tardif V, Nichols C, Procopio F, et al. Reversible reprogramming of circulating memory T follicular helper cell function during chronic HIV infection. J Immunol. 2015;195(12):5625–36. doi:10.4049/jimmunol.1501524.
- Cohen K, Altfeld M, Alter G, Stamatatos L. Early preservation of CXCR5+ PD-1+ helper T cells and B cell activation predict the breadth of neutral- izing antibody responses in chronic HIV-1 infection. J Virol. 2014;88(22):13310–21. doi:10.1128/JVI.02186-14.
- Moysi E, Pallikkuth S, De Armas LR, Gonzalez LE, Ambrozak D, George V, Huddleston D, Pahwa R, Koup RA, Petrovas C, et al. Altered immune cell follicular dynamics in HIV infection following influenza vaccination. J Clin Invest. 2018;128(7):3171–85. doi:10.1172/JCI99884.
- Pallikkuth S, Pilakka Kanthikeel S, Silva SY, Fischl M, Pahwa R, Pahwa S. Upregulation of IL-21 receptor on B cells and IL-21 secretion distin- guishes novel 2009 H1N1 vaccine responders from nonresponders among HIV-infected persons on combination antiretroviral therapy. J Immunol. 2011;186(11):6173–81. doi:10.4049/jimmunol.1100264.
- de Armas LR, Cotugno N, Pallikkuth S, Pan L, Rinaldi S, Sanchez MC, Gonzalez L, Cagigi A, Rossi P, Palma P, et al. Induction of IL21 in peripheral T follicular helper cells is an indicator of influenza vaccine response in a previously vaccinated HIV-infected pediatric cohort. J Immunol. 2017;198(5):1995–2005. doi:10.4049/jimmunol.1601425.
- Breer H, Boekhoff I, Tareilus E. Rapid kinetics of second messenger formation in olfactory transduction. Nature. 1990;345(6270):65. doi:10.1038/345065a0.
- Beavo JA, Brunton LL. Cyclic nucleotide research—still expanding after half a century. Nat Rev Mol Cell Biol. 2002;3(9):710. doi:10.1038/nrm911.
- Gilbert K, Hoffmann M. cAMP is an essential signal in the induction of antibody production by B cells but inhibits helper function of T cells. J Immunol. 1985;135:2084–89.
- Brenchley JM, Price DA, Schacker TW, Asher TE, Silvestri G, Rao S, Kazzaz Z, Bornstein E, Lambotte O, Altmann D, et al. Microbial translocation is a cause of systemic immune activation in chronic HIV infection. Nat Med. 2006;12(12):1365. doi:10.1038/nm1511.
- Sewankambo N, Gray RH, Wawer MJ, Paxton L, McNairn D, Wabwire-Mangen F, Serwadda D, Li C, Kiwanuka N, Hillier SL, et al. HIV-1 infection associated with abnormal vaginal flora morphology and bacterial vaginosis. Lancet. 1997;350(9077):546–50. doi:10.1016/S0140-6736(97)01063-5.
- Selwyn PA, Feingold AR, Hartel D, Schoenbaum EE, Alderman MH, Klein RS, Friedland GH. Increased risk of bacterial pneumonia in HIV-infected intravenous drug users without AIDS. AIDS. 1988;2(4):267–72. doi:10.1097/00002030-198808000-00005.