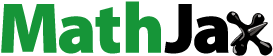
ABSTRACT
Aim: This research investigated the therapeutic effect of an allogeneic mouse brain microvascular endothelial cell vaccine on lung cancer and further elucidated its potential anti-angiogenic mechanism.
Materials & methods: The immune effect of the allogeneic bEnd.3 vaccine and DC vaccine loaded with bEnd.3 antigen on the subcutaneous transplantation of Lewis lung cancer (LLC) was assessed by ELISA, the CCK test and the CTL killing test. The mechanism was preliminarily revealed by immunohistochemistry and immunoblot analysis.
Results: This study revealed that tumor volume was decreased (p < .01) and the survival was prolonged significantly (p < .05) by the bEnd.3 vaccine in subcutaneous LLC transplantation in the vaccine prevention group. In contrast, both tumor volume in the serum therapeutic group and survival of bEnd.3 vaccine were not significantly different from those of the control group (p > .05). Importantly, tumor volume and survival of the T lymphocyte therapeutic group were decreased and prolonged (p < .05). In addition, both tumor volume and survival of DC vaccine loaded with bEnd.3 in the vaccine prevention group were decreased and prolonged significantly (p < .01). Furthermore, bEnd.3 vaccine and DC vaccine loaded with bEnd.3 both produced the activity of killing bEnd.3 target cells in vitro.The reason may induce the immune mice to produce anti-VEGFR-II, anti-endoglin and anti-integrin αν antibodies to have an anti-angiogenesis function.
Conclusion: The allogeneic mouse bEnd.3 cell vaccine can block angiogenesis and prevent the development of lung cancer transplantation tumors.
Introduction
Lung cancer is one of the most common malignant tumors. Due to the morbidity and mortality rate, lung cancer has been recognized as the first killer in cancer threatening human life and health. As of 2018, there were 2.1 million lung cancer patients worldwide, and approximately 1.8 million of them died of lung cancer. In 2018, there were 234,030 newly diagnosed lung cancer patients in the United States, among whom 154,050 eventually died.Citation1 However, due to imperfect tobacco control measures, more than 50% of newly diagnosed cases of lung cancer mainly occur in non-developed countries.Citation2 Currently, the treatment of lung cancer is mainly surgical resection, supplemented with chemotherapy and radiotherapy, as well as several recently approved immunotherapeutic for lung cancer, such as the following: checkpoint PD-1 inhibitor: PembrolizumabCitation3,Citation4 and Nivolumab; PD-L1 inhibitor: atezolizumab; antineoplastic angiogenic drugs:Citation5 Bevacizumab, Ramucirumab, apatnib and Anlotinib; and targeted drugs: Gefitinib, Erlotinib, Crizotinib, Alectinib, and Osimertinib. The new drugs bring great survival benefits to patients with lung cancer. What is even more surprising is that their combined treatment prolongs the total survival of patients with lung cancer.Citation6 However, this series of therapies still cannot completely cure the cancer. Tumor metastasis is the primary cause of failure of lung cancer treatment and the culprit of high lung cancer mortality. High prices, side effects and different driving genes and pathological types are also important factors that cannot be ignored. Therefore, it is urgent to find another effective treatment for lung cancer.
The formation of new blood vessels and angiogenesis is an important way to regulate physiological processes of the body. Recent studies have shown that angiogenesis is also a key participant in the process of tumorigenesis and development. Angiogenesis plays an important regulatory role in tumor growth and metastasis.Citation7,Citation8 Several studies have revealed that anti-angiogenic therapy can effectively prevent new angiogenesis around the tumor and block the supply of materials carried by the newly-formed pathological capillary network to the cancer tissue, which is a new anticancer strategy.Citation9,Citation10 Recently, there are many studies on proliferation-related proteins and DNA molecular vaccines against foreign vascular endothelial cells.Citation11 Although tumor vascular endothelial cells are essentially different from normal vascular endothelial cells, the immune system of the body cannot recognize and clear them, and the body has immune tolerance to tumor vascular endothelial cells.Citation12–14 Our previous studyCitation15 successfully inhibited the growth and metastasis of melanoma by blocking angiogenesis.Citation16,Citation17 A variety of targeted therapies for angiogenesis have been developed successively, showing strong antitumour activity in preclinical animal models of cancer.Citation18 During tumourigenesis, tumor vascular endothelial cells that are actively proliferating express a variety of specific cell membrane protein molecules associated with cell proliferation called vascular endothelial cell proliferation-related antigens,Citation19 such as vascular endothelial growth factor receptor II (VEGFR-II), integrin αν (CD51), tissue factor and endoglin (CD105).Citation20 These molecules are not expressed on resting endothelial cells. Vascular endothelial growth factor (VEGF) is one of the most important regulators of angiogenesis. Accordingly, VEGF-targeting drugs for treating various types of solid tumors have been widely developedCitation21 and approved for clinical application by the U.S. Food and Drug Administration as antitumour drugs alone or in combination with chemotherapy and radiotherapy.Citation22 However, a large number of clinical studies show that such targeted drugs do not have good therapeutic effects on all types of tumors. Due to the emergence of inherent or acquired drug resistance, antiangiogenic therapy has achieved only mild short-term efficacy in most patients.Citation23,Citation24 Therefore, it is urgent to develop new antitumour therapies to inhibit angiogenesis and achieve good antitumour effects.
Modern medicine has been committed to the development of effective targeted tumor antiangiogenic compounds. However, most preclinical and clinical trials show that almost all targeted drugs need to be given frequently with great toxicity and side effects. Active immunotherapy targeting tumor vascular endothelial cells has a longer maintenance time. Targeted immunotherapy does not require frequent and repeated drug administration. The specific humoral immune response or cellular immune response has fewer side effects. Therefore, inducing an active immune effect against tumor blood vessels is a longer-term treatment method. Studies have shown that the preparation of vaccines by cultivating actively proliferating xenovascular endothelial cells in vitro can successfully break immune tolerance and inhibit tumor angiogenesis.Citation25 Mouse brain microvascular endothelial cell bEnd.3 is a vascular endothelial cell line produced by American Type Culture Collection, which has been used in many laboratories at home and abroad. bEnd.3 cells highly expressed polyviral T antigen (Polyomavirus middle T antigen) transfected with NTKmT retrovirus and have been confirmed as microvascular endothelial cells (MVECs). Recent studies have found that human umbilical vein endothelial cell (HUVEC) vaccines can effectively enhance the anticancer efficacy of the chemotherapy drug docetaxel. A HUVEC vaccine containing different forms of antiangiogenesis-associated antigens has shown favorable preventive and antitumor effects in vivo.Citation26 In our previous studies, we found that the targeted HUVEC vaccine has a good effect on esophageal squamous cell carcinoma and colorectal cancer in mice,Citation27,Citation28 which indicates that the vascular endothelial cell vaccine has a good potential anticancer application. Recently, there have been many studies on proliferation-related proteins and DNA molecular vaccines against foreign vascular endothelial cells. However, these results have been controversial due to the limitations of hypersensitivity and the poor therapeutic effect of a single target. Therefore, it is imperative to find a multi-target immunotherapy with fewer side effects. Earlier studies have found that using an allogeneic preparation of liver blood sinus endothelial cells as an antigen source for a vaccine can significantly improve rectal cancer. This effect is better than that of a heterogeneous vaccine.Citation29,Citation30 Research has pioneered the treatment of the allogeneic vaccine. However, for a long time, the role and mechanism of allogeneic vaccines, especially vaccine-mediated anti-angiogenic effects, in the treatment of cancer have not been fully elucidated. Although tumor vascular endothelial cells are essentially different from normal vascular endothelial cells, the immune system of the body cannot recognize and clear them, and the body has immune tolerance to tumor vascular endothelial cells. It is one of the main mechanisms by which tumors escape the monitoring of the immune system, and the result is that the tumor grows indefinitely in the body. The maturation of dendritic cells (DCs) is one of the causes of immune tolerance. Immature DCs can induce T cells to become tolerant, while mature DCs can induce T cells to produce an effective immune response. Therefore, it is an effective method to overcome immune tolerance by using the mature DC antigen presentation function. This paper puts forward the following hypothesis: the vaccine prepared from allogeneic microvascular endothelial cells can effectively induce an immune effect against lung tumor angiogenesis and inhibit tumor growth.
Materials & methods
Cell culture
In this study, the mouse brain microvascular endothelial cell bEnd.3 was a brain microvascular endothelial cell strain recorded by ATCC (American Type Culture Collection) and was a kind gift from the genetic Institute of Fudan University of CHINA. Mouse fibroblast endothelial cell line NIH3T3 was purchased from Wuhan Cell Collection Center. The mouse Lewis lung cancer cell line was purchased from Shanghai Cell Bank, Chinese Academy of Sciences. The cells were cultured in high-glucose Dulbecco’s modified Eagle medium (DMEM, Gibco, USA) containing 10% fetal bovine serum (FBS). The incubator was set at 37°C and 5% CO2.
Animals
SPF/VAF C57BL/6 mice weighed 16–18 g and were 6–8 weeks old, female, and purchased from Beijing Weitong Lihua Experimental Animal Technology Co., Ltd., license No. SCXK (Beijing) 2006/0008.
Immunohistochemical staining
Immunohistochemical staining was performed in accordance with conventional methods. Briefly, the tissue sections were fixed with 4% paraformaldehyde at room temperature for 30 min, washed with phosphate-buffered saline (PBS) 3 times, permeabilized with 0.25% Triton X-100 (30–40 μl/sample) for 10 min, and washed with PBS 3 times. Then, H2O2 (30% H2O2:methanol = 1:50) was added, and the sections were incubated at room temperature for 30 min, washed with PBS 3 times and incubated in bovine serum albumin for 30 min. The primary antibody was incubated at 4°C overnight, and the secondary antibody was incubated at room temperature for 20 min. Strept avidin-biotin complex (SABC) and diaminobenzidine were added for staining, and the staining degree was observed by microscopy. Finally, hematoxylin was added. The expression of the target protein was observed by phase contrast inverted microscopy and scanning with a Biosens Digital Imaging System Grey Scanner.
Culture of mouse bone marrow-derived DCs
The C57BL/6 mice were killed by neck pulling and soaked in 75% alcohol for 5 min. The femur and tibia were aseptically peeled and rinsed with GKN solution twice. The bone marrow cavity was pierced with a 2.5-ml syringe, and the bone marrow was rinsed repeatedly until the bone whitened. The flushing solution was injected into the centrifugal tube at 1700 r/min, and the precipitated cells were collected by centrifugation for 6 min. The erythrocyte lysate preheated at 37°C was added to the mixture at a 1:10 ratio. Then, the mixture was erythrolysed at room temperature for 5 min and terminated with GKN solution for 10 ml, centrifuged at 1700 r/min for 6 min, and washed twice. Then RPMI 1640 medium containing rmGM-CSF (10 ng/ml) and rmIL-4 (5 ng/ml) was used, and the cell concentration of 5 × 106/ml was inoculated in a 24-well culture plate with 1 ml/hole, followed by culture in a culture box at 37°C at 5% CO2. Forty-eight hours later, the suspended cells were gently discarded, and the liquid was changed in half a volume. On the 4th day, the cell growth and morphological changes were observed by a phase contrast microscope. On the 6th day, LPS 0.5 μl/ml was added and cultured to the 8th day. The expression of CD86 and CD11a on the surface of mature mouse DCs was detected by flow cytometry, and morphology of the mature mouse DCs was observed by electron microscopy.
Real-time polymerase chain reaction (RT-PCR)
Total RNA was extracted by Trizol. mRNA reverse transcription was performed according to the kit instructions. The PCR reaction included 9 μl of RNase Free ddH2O, 4 μl of AMV/Tfi 5 × buffer, 1 μl of 25 mM MgSO4, 0.5 μl of dNTP Mixture, 0.5 μl of AMV antiretroviral enzyme, 0.5 μl of Tfi DNA polymerase, 0.75 μl of upstream primer and 0.75 μl of downstream primer. The cycle parameters were as follows: full denaturation at 94°C for 2 min, denaturation at 94°C for 2 s, annealing at 58°C for 1 min, extension at 68°C for 2 min, 40 cycles, and full extension at 68°C for 7 min. PCR products were separated by electrophoresis with a 2% agarose gel, and semi-quantitative optical density scanning was then performed with a gel imaging system. β-actin was used as an internal reference gene to calculate the relative expression. The sequence of primers is shown in .
Table 1. Sequence of primers and amplified fragments
Vaccine preparation
BEnd.3 cells, HUVECs and NIH3T3 cells were cultured. They were washed twice with PBS and digested at 37°C for 5 min with 0.05% trypsin containing 0.02% ethylene diamine tetraacetic acid when their confluency in a monolayer reached 80%. When the cells became round, an appropriate amount of high-sugar DMEM medium containing 10% FBS was added to stop digestion. Cells were collected and centrifuged at 2000 r/min for 6 min. The supernatant was discarded, 1–2 ml 0.025% glutaraldehyde was added, and the samples were fixed at 4°C for 20 min and then washed twice with PBS. Cell counting was performed by adding 1 ml of PBS to every 2.5 × 107 cells, placing them into a 1.5-ml Eppendorf (EP) tube, mixing them thoroughly, and injecting them immediately or storing them at −80°C for later use.
The 80% confluent bEnd.3 cells, HUVECs and NIH3T3 cells were washed twice with PBS, digested with trypsin, neutralized with DMEM high-glucose medium containing 10% FBS, counted, put into a 15-ml centrifuge tube, placed into liquid nitrogen for 5 min, and then immediately put into a 37°C water bath for 5 min to melt. This process was repeated four times to freeze-thaw the antigen. The Bradford protein quantitative method was used to detect the antigen concentration. On the fifth day after DCs were derived from mouse bone marrow, the freeze-thaw antigen was added at a concentration of 100 μg/ml. On the eighth day, the density was adjusted to a concentration of 5 × 106 cells/ml, suspended in PBS, to generate the antigen-loaded DC vaccine.
Animal experiments
Female C57BL/6 mice [purchased from Beijing Weitonglihua Experimental Animal Technology Co., Ltd.; License no. SCXK (Beijing) 2006–0008], 6–8 weeks old, with body weights of 16 ± 0.2 g, were housed in a specific pathogen-free-grade animal culture room with a temperature of 21 ± 2°C, relative humidity of 30 – 70%, 12/24 h light/dark cycles, and an air exchange rate of 8–10 times/h. The cages, feed, water and bedding materials were all sterilized under high pressure and replaced every 3 days under aseptic conditions. The animals were housed in an aseptic laminar flow frame. Ultraviolet disinfection of the outer environment of the feeding box was carried out every day.
Animals were divided into prevention and treatment groups. In the prevention group, the bEnd.3 vaccine, HUVEC vaccine (as a positive control because it is xenogenic, NIH3T3 vaccine (as a negative control because it consists of fibroblasts without vascular endothelial properties) and PBS were injected subcutaneously around the axillary lymph nodes of mice. The concentrations of the bEnd.3 vaccine, HUVEC vaccine, and NIH3T3 vaccine were 2.5 × 107 cells/ml, and the mice were vaccinated once a week for a total of five times. After five immunizations, blood was collected from the tail vein of each mouse. Antibody titer in the blood was detected by an enzyme-linked immunosorbent assay (ELISA). On the 7th day of the last immunization, 0.2 ml of Lewis lung cancer cells (3 × 106 cells per mouse) were subcutaneously injected into the shoulder of each mouse. The treatment group was divided into the serum treatment group and T cell treatment group. In the serum treatment group, one week after the final immunization, one mouse from each group was taken and anesthetized by inhaling diethyl ether for 1 to 2 minutes. When the mouse was in a coma, the eyeball was removed, and the blood was collected in a 1.5-ml EP tube, which was placed at room temperature for 1 h and then at 4°C overnight. On the following day, the yellow clear serum from the top of the EP tube was gently transferred to a new EP tube, avoiding the cloudy part. After centrifugation at 4°C at 1500 r/min for 10 min, the clear upper serum was transferred into another EP tube and labeled. On alternate days, the mice were injected with 50 μl of anti-serum into the muscles of both lower limbs 6 times. In the T cell treatment group, mixed T lymphocytes from immunized mice were prepared and then resuspended in PBS. The density was adjusted to 5 × 107 cells/ml, and the cells were subcutaneously injected around tumor blocks every other day. A total of 0.2 ml (1 × 107 cells/ml) was injected into each mouse 6 times.
Next, the mouse bone marrow-derived DCs were prepared to load different antigens, and the DC vaccine immune effect was assessed in vitro and in vivo. The DC vaccine groups were as follows: Group 1: bEnd.3 freeze-thaw antigen-loaded DC vaccine group (bEnd.3-DCs); Group 2: HUVEC freeze-thaw antigen-loaded DC vaccine group (HUVEC-DCs); Group 3: NIH3T3 freeze-thaw antigen-loaded DC vaccine group (NIH3T3-DCs); Group 4: DC vaccine group without antigen loading (DCs); and Group 5: PBS group that received subcutaneous injection of PBS (PBS). On the 5th day of DC culture, the liquid was completely changed, and freeze-thawed antigen was added according to the concentration of freeze-thaw antigen at the concentration of 100 μg/ml. Culture was continued in the incubator at 5% CO2 and 37°C until the 8th day, and DCs were collected.
The bEnd.3-DC vaccine, HUVEC-DC vaccine, NIH3T3-DC vaccine, DC vaccine and PBS were subcutaneously injected into the axillary lymph nodes of the mice at a volume of 0.2 ml each. The vaccine concentration was 5 × 106 cells/ml. Then, the mice were vaccinated once a week for a total of five times. After 5 immunizations, blood was collected from the tail vein of the mice, and the antibody titers were detected by ELISA. On the 7th day after the final immunization, 0.2 ml (3 × 106 cells/mouse) of Lewis lung cancer cells was subcutaneously injected into the shoulder of each mouse.
Observation of tumor growth
The tumor formation rate, tumor incubation period and tumor volume of Lewis lung cancer in the prevention and treatment groups were observed. The incubation period is the time from inoculation of tumor cells to palpable tumor nodules subcutaneously. The maximum long diameter and vertical short diameter (mm), measured by a Vernier caliper, were expressed by a and b, respectively. According to the formula V(mm3) = π/6 × a× b2, the tumor volume was calculated and measured every 3 days for 6 weeks.
Hematoxylin-eosin (H&E) staining
Tumor tissues were resected completely, fixed with 4% paraformaldehyde solution, dewaxed by xylene, dehydrated by an ethanol gradient, made transparent, waxed, embedded, sliced, stained and sealed, and observed and photographed under an optical microscope.
Cell counting kit (CCK)-8 analysis
Cell proliferation was detected using a CCK-8 kit (Tongren Institute of Chemical Research, Japan). bEnd.3 cells, HUVECs and NIH3T3 cells in the logarithmic growth phase were inoculated into a 96-well plate at 200 target cells per well at 50 μl/well. T lymphocytes were extracted from the immunized mice in the non-tumor-bearing prevention group as effector cells and were labeled as follows: bEnd.3-Ts (bEnd.3-T cells), HUVEC-Ts, NIH3T3-Ts and PBS-Ts. the following ratios of effector to target cells were used: 100:1, 50:1, and 25:1. Four replicate wells were prepared in each group and incubated for 48 h. Then, 10 μl of CCK was added per well, and the plates were incubated at 37°C for 4 h in an incubator with 5% CO2. The absorbance (A450) at a wavelength of 450 nm was detected by ELISA to calculate the killing rate: killing rate (%) = [1 – (A450 value in the experimental group – A450 value in the effector group)/(A450 value in the target cell group)] × 100%.
Flow cytometry
Splenic T lymphocytes were extracted and counted. A total of 5 × 106 cells were taken from each group and then resuspended with 100 μl of PBS containing 10% FBS. Then, CD3ε was added at 1 μl/tube and CD8α was added at 2.5 μl/tube. The samples were incubated at 4°C for 30 min. Mice injected subcutaneously with PBS were the control group. After antibody labeling for 30 min, the cells were washed twice with PBS, centrifuged at 1700 r/min, and finally suspended in 1 ml of PBS containing 10% FBS for flow cytometry detection.
ELISA
The relevant proteins were detected according to ELISA kit instructions. A 96-well detachable enzyme standard plate was used, and the membrane protein was diluted with a buffer (3 μg ml). It was added to the plate hole (100 μl/hole) and incubated overnight at 4°C, with 5 compound holes per group. The coating fluid was discarded, followed by direct addition of sealing fluid at 37°C for 2 h. The sealing fluid was then discarded, and it was washed 6 times with PBST mixture for 2 min each time. The dilution solution was added to create dilutions of 1:50, 1:100, 1:200, 1:400, 1:800, 1:1600, 1:3200, and 1:6400 immunized mice antiserum. The positive control and negative control groups were also set. The parameters were the following: 40 μl/hole, 37°C hatch for 1 h, followed by discarding of the antiserum and washing it 6 times with PBST mixture for 2 min each time. HRP-labeled goat anti-mouse IgG secondary antibody was added and diluted (1:10000), with parameters of 50 μl/hole, 37°C hatch for 30 min. The secondary antibody was discarded, followed by washing 6 times with PBST mixture for 2 min each time. TMB substrate solution was added (100 μl/hole, 37°C, coloration 10–20 min), followed by the addition of a terminating liquid (50 μl/hole) and cessation of the reaction. Determination of the absorbance value of each hole at 450 nm was performed by an enzyme-labeling instrument. The blank control had zero adjustment, P/N ≥ 2.1, and was judged to be positive (P: positive antigenic hole A value, N: negative antigenic hole A value). The antisera of the bEnd.3 DCs vaccine, HUVEC DCs vaccine, NIH3T3 DCs vaccine, DCs vaccine and PBS are indicated by bEnd.3DCsX, HUVECDCsX, NIH3T3DCsX, DCsX, and PBSX, respectively, and the normal mouse serum is expressed as NX.
Immunoblot analysis
The protein concentration in each sample was determined by the bicinchoninic acid method. A 10% polyacrylamide gel was used for electrophoresis and then transferred to a polyvinylidene fluoride membrane. The primary antibody was incubated overnight at 4°C, and the secondary antibody was incubated at room temperature for approximately 2 h. Target proteins were imaged in a Gel-Doc UV Gel Imaging System (Quantity One 1-d Analysis Software) with chemiluminescence reagent.
Adverse reaction assay
Two weeks after the last immunization, each group of immunized mice was anesthetized with ether, anesthetized to a coma, and sterilized at 75% alcohol on the right chest and abdomen. A “+”-shaped incision was performed with a surgical blade, the incision was deep to the outer layer of the peritoneum, and wound healing was observed.
Statistical analysis
All test data are represented by () Analysis was performed with SPSS 13.0 statistical software. One-way analysis of variance was used for statistical analysis, and the least significant difference method was used for pairwise comparison. The survival times, survival rates and mean survival times were determined by the Kaplan-Meier method with α = 0.05 as the test standard.
Results
Cell identification and expression of molecular markers
vWF factor, CD31 and CD144 are the molecular markers specific to vascular endothelial cells. VEGFR-II and integrin αν are proliferation-associated antigens of vascular endothelial cells. Microscopically, bEnd.3 cells are fusiform or elongated when the cells are spread out, and they grow like pave stones. The cell body looks like a vortex, with stars or polygons formed when the cell density is low (). vWF factor is strongly positive and distributed in the cytoplasm, and CD31 is strongly positive, visualized as fine, brown granules, and is mainly distributed throughout the cell membrane and cytoplasm (). In addition, most HUVECs were small triangles and short fusiforms, and they converged into a single layer at 2–3 days. The cell boundaries were clear, the cytoplasm was rich, the nuclei were round or oval, and there were sometimes dual nuclei. The cells cultured by passage were full in shape, extending in long spindle shapes and growing in vortex shapes, and the cells were firmly attached to the walls. The cells passaged at the 5th generation had a lumen-like structure (). Immunocytochemistry (SABC) staining showed that the primary antibody was not replaced by PBS and that the vWF factor in HUVECs was also strongly positive, with brown coarse particles that were occasionally clumpy, and was mainly distributed in the cytoplasm. CD144 was weakly positive, was brown and finely granular, and was mainly distributed in the membrane and cytoplasm (). RT-PCR results showed that the fragment length of vWF in the bEnd.3 murine vascular endothelial cells and human HUVECs was approximately 186 bp and 434 bp, respectively, and that of β-actin was approximately 315 bp (). Expression of the mouse bEnd.3 and HUVEC-related antigens VEGFR-II and integrin αν was strong, with VEGFR-II at approximately 219 bp, integrin αν at approximately 278 bp, and β-actin at approximately 315 bp (). Amplification of LLC under the same conditions revealed that the integrin αν amplification bands were correct, while the VEGFR-II bands were not correct, as the band was not 219 bp, and β-actin bands were of similar size. This result suggested that these LLCs do not contain VEGFR-II genes and that NIH3T3 cells do not contain VEGFR-II and integrin αν genes (). The above results showed that both bEnd.3 cells and HUVECs were vascular endothelial cell lines, and they highly expressed unique markers of vascular endothelial cell vWF, VEGFR-II, and integrin αν, while LLCs and NIH3T3 cells, which were control groups in our study, were misexpressed or not expressed. These results served as the basis for later experimental conclusions.
Figure 1. Preparation of cellular vaccines. (A) bEnd.3 morphology of microvascular endothelial cells in rat brains (10×). (B) Immunohistochemical examination of bEnd.3 expression of vWF and CD31 in cells. (C) HUVEC morphology (10 ×). (D) Immunohistochemical detection of vWF and CD31 expression in HUVECs. (E–G) RT-PCR detection of VEGFR-II and integrin αν gene expression in bEnd.3 cells, HUVECs and LLCs
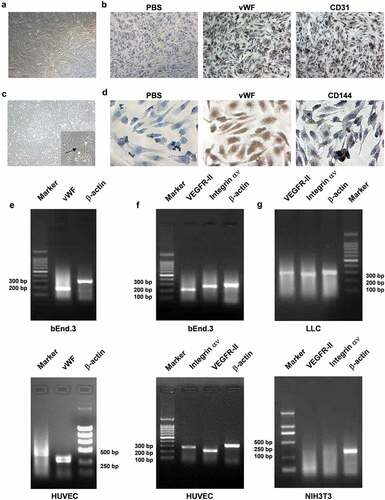
The bEnd.3 vaccine inhibited the growth of subcutaneous lung cancer grafts in mice and prolonged the survival of mice
In the vaccine prevention group, the growth rate of subcutaneous Lewis lung cancer grafts in the bEnd.3 group was slow, and after 3 weeks of growth, the tumor volume was significantly reduced by approximately 20 mm3 and then in a relatively stagnant state, with a significant difference from that of the control group (p < .05) (). The median survival time of the bEnd.3 group was 90 days (termination of experiment), which was significantly greater than that of the control group (44 days) (p < .05) (). Tumor H&E staining showed that the bEnd.3 group and HUVEC group had fat and muscle tissue without cancer cells, while cancer cells were found in the NIH3T3 and PBS groups (). In the T cell treatment group, the mice in the bEnd.3 group showed decreased tumor growth and significantly longer survival times than those in the NIH3T3 and PBS groups (p < .05) ( and D). The bEnd.3 and HUVEC groups showed numerous necrotic structures in the tissues (arrows) (). As expected, serum therapy achieved results similar to T cell therapy but was less effective than in the T cell group, mainly due to the relatively short survival period and the absence of significant tumor tissue necrosis (p > .05) ( -H). Therefore, the allogeneic mouse bEnd.3 cell vaccine significantly inhibited the subcutaneous tumor formation of Lewis lung cancer, thereby extending the survival of the mice.
Table 2. Changes of tumor volume in subcutaneous Lewis lung cancer transplantation in the vaccine prevention group (mm3) [n = 8, ()]
Figure 2. The bEnd.3 vaccine inhibited the growth of subcutaneous grafts of lung cancer in mice and prolonged the survival of mice. (A) Survival curve of mice in the prevention group..The mice in the bEnd.3 group showed significantly longer survival times than those in the NIH3T3 and PBS groups (*p < .05) (B) Tumor tissue samples and HE staining in the prevention group. (C) Tumor volume changes in the T cell treatment group.The mice in the bEnd.3 group showed smaller than those in the NIH3T3 and PBS groups (*p < .05) (D) Survival curve of T cell-treated mice.The mice in the bEnd.3 group showed significantly longer survival times than those in the NIH3T3 and PBS groups (*p < .05) (E) H&E staining of tumor tissues in the cell therapy group. (F) Changes in tumor volume in the serum treatment group. (G) Survival curve of mice in the serum treatment group. Serum therapy achieved results similar to T cell therapy but was less effective than in the T cell group, mainly due to the relatively short survival period and the absence of significant tumor tissue necrosis (p > .05) (H) H&E staining of tumor tissues in the serum treatment group. 1, bEnd.3 group; 2, HUVEC group; 3, NIH3T3 group; 4, PBS group
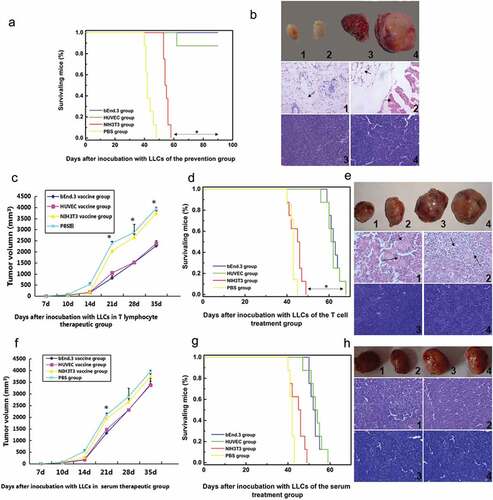
bEnd.3 vaccines induced specific cytotoxic T lymphocytes (CTLs) and antibody production in immunized mice
The flow cytometry figure shows the distribution of CD3+ and CD3+ CD8+ cells in the four groups of cultured cells, and the proportion of CD3+ CD8+ cells in UR is as follows: bEnd.3-T group 24.3%, HUVEC-T group 26.37%, and NIH3T3-T group 23.25%, PBS-T group 22.36%. The results showed that the percentage of CD3+CD8+ T cells in the bEnd.3 vaccine group and HUVEC vaccine group was not higher than that in the control group (p > .05) ( and B). The results of the CCK assay showed that the bEnd.3 vaccine group and HUVEC vaccine group had stronger CTL killing activity when the effector to target ratio was 25:1 (). The average titer of the HUVEC vaccine group was 1:1600 and 1:6400 (). The ELISA results showed that the antiserum of the bEnd.3 vaccine group and the HUVEC vaccine group had a specific immune response to bEnd.3 and HUVEC membrane proteins and cells in vitro () and could inhibit the proliferation of bEnd.3 cells and HUVECs in vitro (). Cellular immunohistochemical staining further confirmed this result ( & B).Immunoblot results showed that specific bands of antiserum appeared at 220 kDa and 180 kDa in both the bEnd.3 group and HUVEC group, while specific bands appeared at 130 kDa in the HUVEC group and 130 kDa in the bEnd.3 group. There was no specific reaction or bands between the antiserum and LLC membrane protein in the two groups (). It should be noted that although there was slight oozing of blood in the wounds in the bEnd.3 and HUVEC groups, the bEnd.3 group bleeding was more obvious than that of the HUVEC group, while the wounds in the NIH3T3 group and PBS group basically healed (). In conclusion, the allogeneic bEnd.3 vaccine induced specific CTL killing activity and antibody production in immunized mice but had little effect on normal wound healing.
Table 3. Comparison of CTL killing activity of spleen T lymphocytes in immunized mice against different target cells in vitro [n = 4, () %]
Table 4. Inhibitory effect of antiserum from different immunized mice on proliferation of different target cells [n = 4, ()]
Figure 3. Specific CTL cytotoxicity induced by the same bEnd.3. (A and B) Expression of CD3 and CD8 on the surface of T lymphocytes in the spleen of immunized mice. (C) ELISA was performed to detect antibody titer in immunized mice. (D) ELISA was used to detect the specific immune response to antiserum in immunized mice
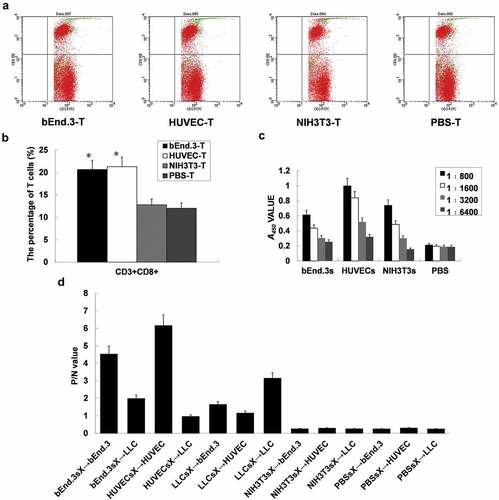
Figure 4. bEnd.3 antibody production induced by the vaccine in immunized mice. (A and B) Immunohistochemical staining was used to detect the specific anti-serum reaction of immunized mice. (C) Immunoblotting was performed to assess the anti-VEGFR-II and integrin αν antibodies in the immune serum of mice. (D) Wound healing response
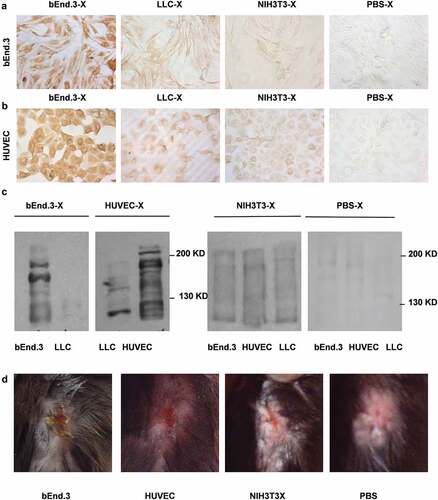
DCs loaded with bEnd.3 antigen promoted T lymphocyte proliferation and induced CTL killing activity of vascular endothelial cells
The CCK test results showed that DCs loaded with bEnd.3 antigen and HUVEC antigen promoted the proliferation of splenic T lymphocytes in vitro, and DCs loaded with NIH3T3 antigen also promoted the proliferation of splenic T lymphocytes in mice (p < .05) (). The MTT assay results showed that DCs loaded with bEnd.3 antigen and HUVEC antigen had an obvious killing effect on bEnd.3 cells and HUVECs (p < .05), while CTLs induced by DCs loaded with NIH3T3 antigen had no obvious killing effect on bEnd.3 cells and HUVECs (). In summary, DCs loaded with bEnd.3 antigen can promote T lymphocyte proliferation and induce CTL killing activity against endothelial cells.
Table 5. Detection of the proliferation ability of autologous lymphocytes stimulated by DCs [n = 8, ) %]
Table 6. Comparison of killing rates of bEnd.3 and HUVEC in target cells by DCs loaded with different antigens [n = 5, () %]
Detection of the immune effect of the DC vaccine loaded with bEnd.3 antigen on subcutaneous transplantation of Lewis lung cancer in mice
DCs loaded with bEnd.3 antigen were cultured and matured for approximately 8 days. After immunizing the mice, the tumor growth rate of the mice in the bEnd.3 DC group was slow. There was a significant difference between the NIH3T3 DCs group and the DCs group (**p < .01) (). The tumor growth rate in the NIH3T3 DC group and the DC group was slower than that in the PBS group (p < .05). The median survival time of mice in the bEnd.3 DCs group was 65 days, and those in the NIH3T3 DC and DC groups were 55 and 57 days, respectively. The median survival time of mice in the bEnd.3 DC group was significantly longer than that in the control group (**p < .01) (). The proportion of CD3+ CD8+ cells in UR was as follows: bEnd.3-DC-T group 37.86%, HUVEC-DC-T group 36.82%, NIH3T3-DC-T group 35.54%, DC-T group 31.78%, and PBS-T group 33.62%. The percentage of splenic CD3+CD8+ T lymphocytes in mice after immunization was determined by measuring bEnd.3-DC-Ts and HUVEC-DC-Ts, which was not higher than that in the NIH3T3-DC-T, DC-T and PBS-T groups (p > .05) ( and D). The results of the CCK assay showed that splenic T lymphocytes from the bEnd.3-DCs group of immunized mice had a lethal effect on bEnd.3 CTL proliferation in vitro (). The NIH3T3 cells showed the same proliferation effect as bEnd.3 cells and HUVECs. However, the NIH3T3 cells had no targeted cell killing effect in the killing test. The reason for this result may be related to the test conditions and need to be obseverd further. The ELISA results of the immune antiserum before and after tumor implantation showed that there was no significant difference in the immune effect between bEnd.3-DCs antiserum and bEnd.3 before and after tumor formation (p > .05), and all the antiserum of the mice after tumor formation could react with bEnd.3 cells (). Using the serum of immunized mice as the primary antibody for immunohistochemical detection, BEnd.3-DC-X antiserum (1:10) showed a positive reaction with bEnd.3 cells. HUVEC-DC-X antiserum (1:10) also showed a positive reaction with HUVECs. However, the NIH3T3-DC-X and DC-X groups (1:10) showed weak positive reactions with bEnd.3 cells and HUVECs. PBS-X antiserum (1:10) was negative ( and B). Immunoblot results showed that specific bands appeared in the antiserum of the bEnd.3-DC group; there were also specific bands at 220 and 180 kDa and no specific bands at 130 kDa. In the HUVEC-DC group, there were specific bands at 220, 180 and 130 kDa and no specific reactions and bands with LLC membrane proteins (). In summary, the bEnd.3 antigen-loaded DC vaccine can inhibit the growth of subcutaneously transplanted Lewis lung cancer tumors in mice, prolong the survival of the mice and exert effects against tumor angiogenesis.
Figure 5. DCs loaded with bEnd.3 antigen inhibited tumor formation and promoted T lymphocyte proliferation. (A) Changes in tumor volume of subcutaneous Lewis lung cancer grafts. There was a significant difference between the NIH3T3 DC group and the DC group (**p < .01). (B) Survival curve of mice. The median survival time of mice in the bEnd.3 DC group was significantly longer than that in the control group (**p < .01). (C and D) Expression of CD3 and CD8 on the surface of T lymphocytes in the spleen of immunized mice
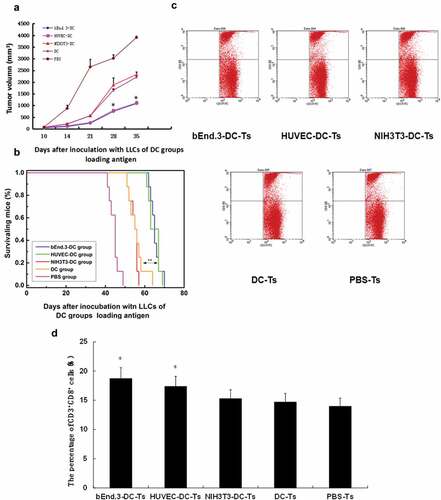
Figure 6. DCs loaded with antigen induced endothelial cell CTL killing activity. (A) Different immune mouse spleen T lymphocytes kill different target cells. bEnd.3DC-T killing activity of bEnd.3 cells and HUVECDC-T killing activity of HUVECs were significantly greater than those of the NIH3T3DC-T, DC-T and PBS-T groups (*p < .05). (B) Specific response of serum antibodies before and after tumor bearing. The bEnd.3DC-T group and HUVECDC-T group were significantly stronger than the NIH3T3DC-T, DC-T and PBS-T groups (*p < .05)
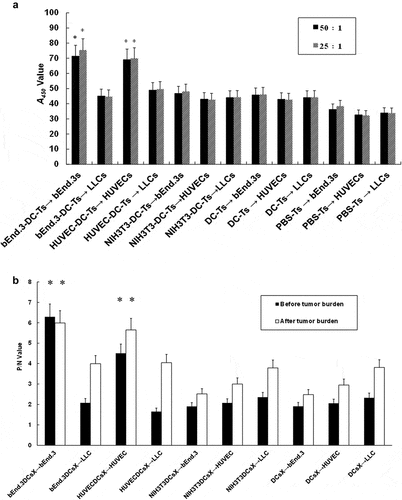
Figure 7. DCs loaded with bEnd.3 antigen induced antibody production in immunized mice. (A and B) Immunocytochemistry was used to detect the specific reaction of anti-serum antibodies in immunized mice. (C) The antiserum antibodies VEGFR-II and integrin αν in mice immunized with C57 were detected by immunoblot
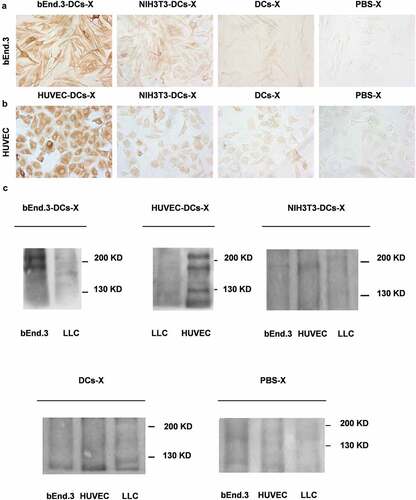
Discussion
In recent years, cancer immunothrapy has gradually developed into the fourth tumor treatment model after surgery, chemotherapy and radiotherapy, attracting extensive attention from the medical community.Citation31–33 Tumor immunotherapy can inhibit tumor growth mainly by mobilizing or enhancing the inherent antitumour ability of the body to avoid the serious limitations of current tumor treatment modalities. Currently, most immunotherapies are targeted at lung cancer cells, but due to unstable genetic traits and a high frequency of gene mutations in lung cancer cells, immune tolerance or mutated drug resistance occurs easily, making the therapeutic effect unsatisfactory. Compared with lung cancer cells, lung cancer vascular endothelial cells have genomic stability and do not easily mutate, so antitumour vascular therapy targeting vascular endothelial cells is a more promising treatment strategy.Citation34–37
In this study, the mouse cerebral microvascular endothelial cell bEnd.3 was selected as the vaccine. For the mice receiving the vaccine, HUVECs are xenogenic. bEnd.3 cells express not only the inherent markers of endothelial cells but also the genes VEGFR-II and endoglin, which are related to endothelial cell proliferation. HUVECs were selected as the control because HUVECs originate from the large vein and have the potential for differentiation and proliferation and the characteristic of neovascularization. Mouse NIH3T3 fibroblasts were selected as control cells because NIH3T3 fibroblasts are non-vascular endothelial cells that do not express vascular endothelial proliferation-related antigens, and they are murine cells and allogeneic. bEnd.3 cells were sub-cultured many times, and we first identified endothelial cell markers and proliferation-related antigens on their surface. Subsequently, we selected C57BL/6 inbred mice as vaccine recipients and prepared tumor models. Mouse Lewis lung cancer cells were derived from C57BL/6 mice with a high metastasis rate,Citation38 which is also one of the solid tumors with a high blood supply, and it is commonly used to study tumor angiogenesis and anti-angiogenic therapy.Citation39,Citation40 The indicators of antitumour effects included tumor volume, survival time, and in vivo-induced cell and humoral immunity.Citation41 The trend of tumor voloum and survival is consistent with previous research results.Citation41 Therefore, the vascular endothelial cell vaccine in this study can resist tumor vascular endothelial cells, which is similar to the results of a study by Xiang et al.Citation42 According to the study results, we speculate that anti-VEGFR-II antibody and anti-endoglin antibody may exist in bEnd.3-X serum. The delayed healing of the post-immunized wound is consistent with previous studies.Citation43–45
Using DC antigen presentation is an effective method to break immune tolerance. Multiple studies have shown that DCs can be loaded in vitro with various forms of tumor antigen (tumor-specific antigen peptide, tumor cell lysate, cell fusion, tumor cell RNA or DNA) and made into a DC vaccine, which can be injected back into the body to induce a specific immune response.Citation46,Citation47 DCs can be divided into two categories: myeloid DCs and lymphatic DCs.Citation48,Citation49 Achieving DC-based immunotherapy requires a sufficient amount of DCs. Although DCs are widely distributed throughout the body, they are very small in quantity. The mice were small in size, with only 1–2 ml of peripheral blood, and we were unlikely to obtain sufficient DCs from peripheral blood. Thus, we chose to culture a large number of DCs from mouse bone marrow. Determining the optimal antigen dose to load the DCs is the key to sensitizing DCs. In this study, mouse spleen-derived immature DCs were loaded with freeze-thaw antigen from mouse bEnd.3 cells cultured in vitro. Rapid maturity was found to effectively stimulate autologous lymphocyte proliferation. CTLs induced by DCs could specifically kill vascular endothelial cells, proliferate in vitro and inhibit tumor growth in vivo. Compared with the whole endothelial cell vaccine, DCs were loaded with the same amount of antigen, but the antigen-loaded DC vaccine was not as effective as the whole endothelial cell vaccine in inhibiting tumors. However, vascular endothelial cells originating from two different genera and different sources (bEnd.3 from brain microvessels, HUVECs from umbilical veins) had similar antitumour effects and showed no significant differences.
The reason why this experiment studied allogeneic vaccines is mainly to find an effective and less side-effect vaccine clinically. In humans, umbilical vein endothelial cells of maternal umbilical cord are very easy to obtain. The preparation of anti-tumor vascular vaccine in vitro is a very well method. But now there is no such anti-tumor angiogenesis vaccine clinically. This experiment is only a preclinical study, and it takes many steps to really apply it to the clinic.
Although the vaccine in mice has a tendency to prolong survival, there are still many difficulties in applying it to the human body, such as vaccine preparation, ethics, and RCT research, so significantly more research is needed.
Conclusions
In summary, this study showed that the allogeneic mouse bEnd.3 cell vaccine and the DC vaccine loaded with mouse bEnd.3 antigen induced the production of VEGFR-II antibodies, anti-endoglin antibodies and anti-integrin αν antibodies by inducing CTLs and targeting proliferating vascular endothelial cells, which induced the cellular and humoral immune responses against tumor vessels, blocked the formation of blood vessels and inhibited the growth of tumors. Because its target is tumor blood vessels, this vaccine can target different pathological types of tumor cells. With the increase in treatment methods for lung cancer,Citation50 the advent of immunotherapy, and the combination of different drugs, patients with lung cancer are experiencing prolonged survival rates. If the allogeneic mouse bEnd.3 cell vaccine and the DC vaccine loaded with mouse bEnd.3 antigen were combined with targets and immune drugs that were already on the market and not yet on the market, better results would likely be achieved, which is the direction of our next study. Due to the heterogeneity of tumors and the complexity of the immune system,Citation51 there are still many ways to overcome lung cancer. In short, the results of this study will provide a new research direction and strategies for therapies against tumor angiogenesis.
Future perspective
With the increase in treatment methods for lung cancer, the advent of immunotherapy, and the combination of different drugs, patients with lung cancer are benefiting increasingly more. If the allogeneic mouse bEnd.3 cell vaccine and the DC vaccine loaded with mouse bEnd.3 antigen were combined with targets and immune drugs that were already on the market and not yet on the market, better results may be achieved. Especially in radical surgery for lung cancer, as well as other cancers potentially, the use of these treatments combined with “hot tumor” drugs such as PD1 could help prevent recurrence and metastasis. They will likely lead to better results, which is the direction of our future study. Commercialization of this therapy will require significantly more research.
Author contributions
JZ, JMZ, and JL conceived and designed the study. JZ and XLZ performed the experiments. YYD and LRZ collected and analysed the data. JZ wrote the original manuscript. ZMD, JMZ, and JL reviewed and edited the manuscript. All authors have read and approved the current manuscript.
Data sharing statement
The datasets used and/or analysed during the current study are available from the corresponding author on reasonable request.
Ethical disclosure
All the protocols described in the present study were reviewed and approved by the Ethics Committee of Zhengzhou University (Zhengzhou, Henan, China).
Information pertaining to writing assistance
Writing assistance from American Journal Experts was utilized in the production of this manuscript, which was funded by the Natural Science Foundation of China (81572972) and the Supporting Plan of Scientific and Technological Innovation Team in Universities of Henan Province (20IRTSTHN029).
Acknowledgments
N/A
Additional information
Funding
References
- Siegel RL, Miller KD, Jemal A. Cancer statistics, 2018. CA Cancer J Clin. 2018;68(1):7–30. doi:10.3322/caac.21442.
- Bray F, Ferlay J, Soerjomataram I, Siegel RL, Torre LA, Jemal A. Global cancer statistics 2018: GLOBOCAN estimates of incidence and mortality worldwide for 36 cancers in 185 countries. CA Cancer J Clin. 2018;68(6):394–424. doi:10.3322/caac.21492.
- Herbst RS, Baas P, Kim DW, Felip E, Perez-Gracia JL, Han JY, Molina J, Kim J-H, Arvis CD, Ahn M-J, et al. Pembrolizumab versus docetaxel for previously treated, PD-L1-positive, advanced non-small-cell lung cancer (KEYNOTE-010): a randomised controlled trial. Lancet. 2016;387(10027):1540–50. doi:10.1016/S0140-6736(15)01281-7.
- Velcheti V, Chandwani S, Chen X, Pietanza MC, Piperdi B, Burke T. Outcomes of first-line pembrolizumab monotherapy for PD-L1-positive (TPS >/=50%) metastatic NSCLC at US oncology practices. Immunotherapy. 2019;11(18):1541–54. doi:10.2217/imt-2019-0177.
- Janning M, Loges S. Anti-angiogenics: their value in lung cancer therapy. Oncol Res Treat. 2018;41:172–80. doi:10.1159/000488119.
- Manegold C, Dingemans AC, Gray JE, Nakagawa K, Nicolson M, Peters S, Reck M, Wu Y-L, Brustugun OT, Crinò L, et al. The potential of combined immunotherapy and antiangiogenesis for the synergistic treatment of advanced NSCLC. J Thoracic Oncol: Off Publ Int Assoc Study Lung Cancer. 2017;12(2):194–207. doi:10.1016/j.jtho.2016.10.003.
- Folkman J. Role of angiogenesis in tumour growth and metastasis. Semin Oncol. 2002;29:15–18. doi:10.1053/sonc.2002.37263.
- Van Dreden P, Epsilonlalamy I, Gerotziafas GT. The role of tissue factor in cancer-related hypercoagulability, tumor growth, angiogenesis and metastasis and future therapeutic strategies. Crit Rev Oncog. 2017;22(3–4):219–48. doi:10.1615/CritRevOncog.2018024859.
- Yang Y, Jin G, Liu H, Liu K, Zhao J, Chen X, Wang D, Bai R, Li X, Jang Y, et al. Metformin inhibits esophageal squamous cell carcinoma-induced angiogenesis by suppressing JAK/STAT3 signaling pathway. Oncotarget. 2017;8(43):74673–87. doi:10.18632/oncotarget.20341.
- Jin G, Yang Y, Liu K, Zhao J, Chen X, Liu H, Bai R, Li X, Jiang Y, Zhang X, et al. Combination curcumin and (-)-epigallocatechin-3-gallate inhibits colorectal carcinoma microenvironment-induced angiogenesis by JAK/STAT3/IL-8 pathway. Oncogenesis. 2017;6(10):e384. doi:10.1038/oncsis.2017.84.
- Lopes A, Vandermeulen G, Preat V. Cancer DNA vaccines: current preclinical and clinical developments and future perspectives. J Exp Clin Cancer Res: CR. 2019;38(1):146. doi:10.1186/s13046-019-1154-7.
- Strioga MM, Darinskas A, Pasukoniene V, Mlynska A, Ostapenko V, Schijns V. Xenogeneic therapeutic cancer vaccines as breakers of immune tolerance for clinical application: to use or not to use? Vaccine. 2014;32(32):4015–24. doi:10.1016/j.vaccine.2014.05.006.
- Pinto MP, Sotomayor P, Carrasco-Avino G, Corvalan AH, Owen GI. Escaping Antiangiogenic Therapy: strategies Employed by Cancer Cells. Int J Mol Sci. 2016;17(9):1489.
- Rahma OE, Hodi FS. The intersection between tumor angiogenesis and immune suppression. Clin Cancer Res: Off J Am Assoc Cancer Res. 2019;25(18):5449–57. doi:10.1158/1078-0432.CCR-18-1543.
- Zhao J, Lu J, Liu YQ, Yang HY, Huang YT, Zhao JM, Li S, Zhai J-M, Zhao M-Y, Zhang X, et al. Study on the specific immunity induced by dendritic cell vaccine loading allogeneic microvascular endothelial cell bEnd.3 antigen against U14 cervical cancer cell in mice. Zhonghua Fu Chan Ke Za Zhi. 2011;46(1):52–57.
- Lu J, Tang Y, Farshidpour M, Cheng Y, Zhang G, Jafarnejad SM, Yip A, Martinka M, Dong Z, Zhou J, et al. JWA inhibits melanoma angiogenesis by suppressing ILK signaling and is an independent prognostic biomarker for melanoma. Carcinogenesis. 2013;34(12):2778–88. doi:10.1093/carcin/bgt318.
- Lu J, Tang Y, Cheng Y, Zhang G, Yip A, Martinka M, Dong Z, Zhou J, Li G. ING4 regulates JWA in angiogenesis and their prognostic value in melanoma patients. Br J Cancer. 2013;109(11):2842–52. doi:10.1038/bjc.2013.670.
- Cao Y. Future options of anti-angiogenic cancer therapy. Chin J Cancer. 2016;35(1):21. doi:10.1186/s40880-016-0084-4.
- Zhao J, Lu J, Zhou L, Dong Z. Efficacy for lung metastasis induced by the allogeneic bEnd3 vaccine in mice. Hum Vaccin Immunother. 2018;14(5):1294–304. doi:10.1080/21645515.2018.1427532.
- Wang J, Yang L, Liang F, Chen Y, Yang G. Integrin alpha x stimulates cancer angiogenesis through PI3K/Akt signaling-mediated VEGFR2/VEGF-A overexpression in blood vessel endothelial cells. J Cell Biochem. 2019;120(2):1807–18. doi:10.1002/jcb.27480.
- Al-Abd AM, Alamoudi AJ, Abdel-Naim AB, Neamatallah TA, Ashour OM. Anti-angiogenic agents for the treatment of solid tumors: potential pathways, therapy and current strategies - A review. J Adv Res. 2017;8(6):591–605. doi:10.1016/j.jare.2017.06.006.
- Niu G, Chen X. Vascular endothelial growth factor as an anti-angiogenic target for cancer therapy. Curr Drug Targets. 2010;11(8):1000–17. doi:10.2174/138945010791591395.
- Dey N, De P, Brian LJ. Evading anti-angiogenic therapy: resistance to anti-angiogenic therapy in solid tumors. Am J Transl Res. 2015;7:1675–98.
- Jayson GC, Kerbel R, Ellis LM, Harris AL. Antiangiogenic therapy in oncology: current status and future directions. Lancet. 2016;388(10043):518–29. doi:10.1016/S0140-6736(15)01088-0.
- Wei YQ, Wang QR, Zhao X, Yang L, Tian L, Lu Y, Kang B, Lu C-J, Huang M-J, Lou -Y-Y. Immunotherapy of tumors with xenogeneic endothelial cells as a vaccine. Nat Med. 2000;6(10):1160–66. doi:10.1038/80506.
- Lu M, Yao Q, Liu H, Zhong W, Gao J, Si C, Zhou L, Zhang S, Xu M. Combination of human umbilical vein endothelial cell vaccine and docetaxel generates synergistic anti-breast cancer effects. Cancer Biother Radiopharm. 2019;34(7):464–71. doi:10.1089/cbr.2018.2721.
- Liu H, Zhao J, Yang Y, Jin G, Zhang X, Wang D, Xie C, Liu K, Chen X, Li X. Human umbilical vein endothelial cell vaccine suppresses the angiogenesis of esophageal squamous cell carcinoma in a humanized mouse model. Oncol Rep. 2018;40(5):3006–14. doi:10.3892/or.2018.6653.
- Zhang Q, Xie C, Wang D, Yang Y, Liu H, Liu K, Zhao J, Chen X, Zhang X, Yang W, et al. Improved antitumor efficacy of combined vaccine based on the induced HUVECs and DC-CT26 against colorectal carcinoma. Cells. 2019;8(5):494. doi:10.3390/cells8050494.
- Okaji Y, Tsuno NH, Kitayama J, Saito S, Takahashi T, Kawai K, Yazawa K, Asakage M, Hori N, Watanabe T, et al. Vaccination with autologous endothelium inhibits angiogenesis and metastasis of colon cancer through autoimmunity. Cancer Sci. 2004;95(1):85–90. doi:10.1111/j.1349-7006.2004.tb03175.x.
- Yoneyama S, Okaji Y, Tsuno NH, Kawai K, Yamashita H, Tsuchiya T, Yamada J, Sunami E, Osada T, Kitayama J, et al. A study of dendritic and endothelial cell interactions in colon cancer in a cell line and small mammal model. Eur J Surg Oncol: J Eur Soc Surg Oncol Br Assoc Surg Oncol. 2007;33(10):1191–98. doi:10.1016/j.ejso.2007.01.013.
- Liu SH, Zhang M, Zhang WG. Strategies of antigen-specific T-cell-based immunotherapy for cancer. Cancer Biother Radiopharm. 2005;20(5):491–501. doi:10.1089/cbr.2005.20.491.
- Griffioen AW. Anti-angiogenesis: making the tumour vulnerable to the immune system. Cancer Immunol ImmunoTher: CII. 2008;57(10):1553–58. doi:10.1007/s00262-008-0524-3.
- Mufson RA. Tumor antigen targets and tumour immunotherapy. Front Biosci: J Virtual Lib. 2006;11:337–43. doi:10.2741/1801.
- Boehm T, Folkman J, Browder T, O’Reilly MS. Antiangiogenic therapy of experimental cancer does not induce acquired drug resistance. Nature. 1997;390(6658):404–07. doi:10.1038/37126.
- Alessi P, Ebbinghaus C, Neri D. Molecular targeting of angiogenesis. Biochim Biophys Acta. 2004;1654(1):39–49. doi:10.1016/j.bbcan.2003.08.001.
- Bloemendal HJ, Logtenberg T, Voest EE. New strategies in anti-vascular cancer therapy. Eur J Clin Invest. 1999;29(9):802–09. doi:10.1046/j.1365-2362.1999.00534.x.
- Goh PP, Sze DM, Roufogalis BD. Molecular and cellular regulators of cancer angiogenesis. Curr Cancer Drug Targets. 2007;7(8):743–58. doi:10.2174/156800907783220462.
- Sato T, Takusagawa K, Asoo N, Kumano N, Hasuike M, Konno K. Conversion of Lewis lung carcinoma into ascitic form. Oncology. 1989;46(3):188–92. doi:10.1159/000226712.
- Wen J, Fu AF, Chen LJ, Xie XJ, Yang GL, Chen XC, Wang YS, Li J, Chen P, Tang MH, et al. Liposomal honokiol inhibits VEGF-D-induced lymphangiogenesis and metastasis in xenograft tumour model. Int J Cancer. 2008;124:2709–18. doi:10.1002/ijc.24244.
- Hodish I, Tal R, Shaish A, Varda-Bloom N, Greenberger S, Rauchwerger A, Breitbart E, Bangio L, Ben-Shushan D, Pfeffer R, et al. Systemic administration of radiation-potentiated anti-angiogenic gene therapy against primary and metastatic cancer based on transcriptionally controlled HSV-TK. Cancer Biol Ther. 2009;8(5):424–32. doi:10.4161/cbt.8.5.7589.
- Chen XY, Zhang W, Zhang W, Wu S, Bi F, Su YJ, Tan XY, Liu JN, Zhang J. Vaccination with viable human umbilical vein endothelial cells prevents metastatic tumors by attack on tumour vasculature with both cellular and humoral immunity. Clin Cancer Res: Off J Am Assoc Cancer Res. 2006;12:5834–40. doi:10.1158/1078-0432.CCR-06-1105.
- Xiang R, Mizutani N, Luo Y, Chiodoni C, Zhou H, Mizutani M, Ba Y, Becker JC, Reisfeld RA. A DNA vaccine targeting survivin combines apoptosis with suppression of angiogenesis in lung tumour eradication. Cancer Res. 2005;65:553–61.
- Niethammer AG, Xiang R, Becker JC, Wodrich H, Pertl U, Karsten G, Eliceiri BP, Reisfeld RA. A DNA vaccine against VEGF receptor 2 prevents effective angiogenesis and inhibits tumour growth. Nat Med. 2002;8(12):1369–75. doi:10.1038/nm1202-794.
- He QM, Wei YQ, Tian L, Zhao X, Su JM, Yang L, Lu Y, Kan B, Lou -Y-Y, Huang M-J. Inhibition of tumour growth with a vaccine based on xenogeneic homologous fibroblast growth factor receptor-1 in mice. J Biol Chem. 2003;278(24):21831–36. doi:10.1074/jbc.M300880200.
- Tan GH, Wei YQ, Tian L, Zhao X, Yang L, Li J, He QM, Wu Y, Wen YJ, Yi T, et al. Active immunotherapy of tumors with a recombinant xenogeneic endoglin as a model antigen. Eur J Immunol. 2004;34:2012–21. doi:10.1002/eji.200424933.
- Zhou Y, Bosch ML, Salgaller ML. Current methods for loading dendritic cells with tumour antigen for the induction of antitumor immunity. J Immunother. 2002;25:289–303. doi:10.1097/00002371-200207000-00001.
- Hsu FJ, Benike C, Fagnoni F, Liles TM, Czerwinski D, Taidi B, Engleman EG, Levy R. Vaccination of patients with B-cell lymphoma using autologous antigen-pulsed dendritic cells. Nat Med. 1996;2(1):52–58. doi:10.1038/nm0196-52.
- Boudreau J, Koshy S, Cummings D, Wan Y. Culture of myeloid dendritic cells from bone marrow precursors. J Visualized Exp: JoVE. 2008;(17):769.
- Krawczyk P, Wojas K, Milanowski P, Rolinski J. Myeloid and lymphoid dendritic cells and cytotoxic T lymphocytes in peripheral blood of non-small cell lung cancer patient–a pilot study. Adv Med Sci. 2006;51:160–63.
- Liu WJ, Du Y, Wen R, Yang M, Xu J. Drug resistance to targeted therapeutic strategies in non-small cell lung cancer. Pharmacol Ther. 2020;206:107438.
- Catacchio I, Scattone A, Silvestris N, Mangia A. Immune prophets of lung cancer: the prognostic and predictive landscape of cellular and molecular immune markers. Transl Oncol. 2018;11:825–35.