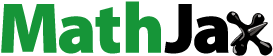
ABSTRACT
Multiepitope cancer vaccines have gained lots of attention for prophylactic and therapeutic purposes in cancer patients. In our previous study, multiepitope DNA and peptide cancer vaccines consisted of the most immunodominant epitopes of ACRBP and SYCP1 antigens were designed by bioinformatic tools. In this study, the effect of prophylactic co-immunization with these DNA and peptide cancer vaccines in the 4T1 breast cancer animal model was assessed. Serum levels of the peptide-specific IgG total, IgG2a and IgG1 were measured by enzyme-linked immunosorbent assay (ELISA). Also, the efficacy of the immunized mice splenocytes’ for producing interleukin-4 (IL-4) and interferon-γ (IFN-γ) was evaluated. The co-immunization caused a significant (P < .05) increase in the serum levels of IgG1 and IgG2a. The co-immunized mice splenocytes exhibited significantly enhanced IL-4 (6.6-fold) and IFN-γ (19-fold) production. Also, their lymphocytes exhibited higher proliferation rate (3-fold) and granzyme B production (6.5-fold) in comparison with the control. The prophylactic co-immunization significantly decreased the breast tumors’ volume (78%) and increased the tumor-bearing mice survival time (37.5%) in comparison with the control. Taking together, prophylactic co-immunization with these multiepitope DNA and peptide cancer vaccines can activate the immune system against breast cancer. However, further experiments are needed to evaluate their efficacy from different angles.
1. Introduction
Breast cancer is the most common cancer in women.Citation1,Citation2 Breast tumors arise from malignant transformation of normal mammary cells which causes uncontrolled cells’ growth, proliferation and invasion.Citation3,Citation4 Currently, the main therapeutic approaches for breast cancer are surgery, chemotherapy and radiation therapy.Citation5 Although they have reached significant improvements over the past decade, their efficacy isn’t still satisfying, especially for metastatic tumors.Citation6-8
Prophylactic cancer vaccines have attracted lots of attention for preventing cancer development, especially in persons with occupational exposure to carcinogens or healthy persons with a high risk of developing breast cancer due to hereditary mutations in the oncogenes or tumor suppressor genes.Citation9-12 Also, cancer vaccines can help breast cancer patients through inhibition of cancer recurrence or metastasis. The main aim of prophylactic immunization is to introduce cancerous antigens to the immune system for the detection of cancer cells.Citation13 Activated immune cells can reach any regions of the human body and eradicate the cancer cells.Citation13,Citation14 Also, vaccination’s effect is lifelong.Citation15
Peptide and DNA vaccines are two well-known approaches for anti-cancer immunization.Citation16 Proper stability, simplicity, and introducing multiple antigens are the advantages of DNA vaccines.Citation17,Citation18 DNA vaccines which were designed to activate the immune system against human epidermal growth factor receptor 2 (HER2/neu)Citation19 and mammaglobinCitation20 have exhibited considerable therapeutic efficacy against breast cancer.Citation17 In addition, peptide vaccines consisted of the immunodominant epitopes of carcinoembryonic antigen (CEA), mucin 1, and HER2/neu have been assessed to be used for breast cancer treatment. Phases I/II/III of clinical trials have exhibited the high ability of these vaccines to induce anti-cancer immunogenic responses without causing autoimmunity reactions.Citation21-23
Tumor-associated antigens (TAAs) are the most utilized antigens for designing cancer vaccines.Citation24 TAAs can be divided into different groups including differentiation antigens (e.g. MUC-1),Citation25 mutational antigens (e.g. CTNNB1),Citation26 overexpressed antigens (e.g. HER2),Citation27 viral antigens (e.g. human papillomavirus proteins),Citation28 and cancer/testis antigens (CTAs).Citation29,Citation30 CTAs have been named because of their restricted expression in the testis and tumor.Citation31 CTAs have high immunogenicity and many studies have reported anti-CTAs immune response in cancer patients. Furthermore, CTAs exhibit very restricted expression in normal tissues.Citation32 Therefore, they are suitable candidates for anti-tumor immunization, and this hypothesis has been demonstrated by different clinical trials.Citation33,Citation34 SYCP1 and ACRBP are two well-known CTAs and considerable immune response against both of these antigens have been detected in patients with different malignancies.Citation35-38 SYCP1 is involved in forming synaptonemal complexes between homologous chromosomes for meiosisCitation39 and ACRBP is a role-playing protein in spermatogenesis and sperm capacitation. ACRBP overexpression has been observed in different types of cancers including bladder,Citation38 ovarian,Citation37 and colorectal cancer.Citation38 Also, SYCP1 is overexpressed at the brain,Citation36,Citation40 pancreas,Citation41 and hepatobiliary tumors.Citation42 Mashino et al reported that about one-third of the breast tumors overexpressed SYCP1.Citation43 Tureci et al detected anti-SYCP1 antibodies in about 50% of the breast cancer patients.Citation36 In addition, overexpression of ACRBP in mammary adenocarcinomas has been reported by many studies.Citation38,Citation44 Therefore, these two antigens are appropriate to form breast cancer vaccines because of restricted expression at malignant tissues and high immunogenicity.Citation45,Citation46 In the context of vaccine design, immunodominant epitopes based vaccines have exhibited numerous advantages over whole-protein vaccines including safety, cost-effectiveness and high specificity due to defined epitopes.Citation47-50 In this regard, computational analyzes for recognition of TAAs’ immunodominant epitopes have gained lots of attention for designing cancer vaccines.Citation51,Citation52
In our previous study, the SYCP1 and ACRBP proteins were analyzed to identify potential immunodominant epitopes by employing computational tools. Then, the DNA and peptide cancer vaccines consisted of the most immunodominant epitopes of the SYCP1 and ACRBP antigens were designed and evaluated in silico.Citation53 In another study, we used the peptide vaccine immunization against B16-F10 melanoma in C57BL/6 mice and the tumors’ growth inhibition and increase of the tumor-bearing mice survival time was observed.
In the present study, we decided to put one step beyond and evaluate the efficacy of co-immunization with the multiepitope DNA and peptide cancer vaccines as a prophylactic vaccination regime against breast cancer in BALB/c mice. According to the best of our knowledge, this is the first time to use the multiepitope DNA and peptide cancer vaccines consisted of the immunodominant epitopes of SYCP1 and ACRBP antigens as a prophylactic breast cancer immunization regime in vivo.
2. Materials and methods
2.1. Epitopes selection
Based on our previous in silico study,Citation53 the most immunodominant fragments of the SYCP1 (RCQHKIAEMVALMEKHKHQYDKI) and ACRBP (YIQYPNYCSFKSQQCL) antigens were selected by computational analyzes and fused by an appropriate furin-sensitive linker (RVRR). The selected fragments had different epitopes with a high affinity for binding to human and mouse MHCs according to NetMHCpan, ProPred-I, and MHCpred servers analyses (Table S1). Also, the NetCTLpan, NetCTL, CTLPred servers were employed for characterizing the CTL epitopes of the selected fragments (Table S2). This data was obtained from our previously published article.Citation53 Furthermore, methionine and glycine were added to the N-terminal and the His-tag to the C-terminal, to design the final construct of the multiepitope vaccine.
2.2. Construction of the multiepitope DNA and peptide vaccines
The template DNA sequence which was obtained by reverse translation of the peptide sequence was codon-optimized by GenScript (https://www.genscript.com/tools/rare-codon-analysis) to support mouse codon adaptation index. A stop codon was added to the 3΄ end and the Kozak sequence was incorporated to the 5´ end of nucleotide sequence to increase the expression rate. Finally, a DNA with appropriate restriction enzyme sites (NheI, NcoI, and XhoI) at both ends was designed. The constructed DNA sequence with 171 bps was ordered to the GeneCust Company (Dudelange, Luxemburg) in pcDNA3.1 expression vector as DNA vaccine (pcDNA3.1-PEP). The peptide (MGRCQHKIAEMVALMEKHKHQYDKIRVRRYIQYPNYCSFKSQQCLHHHHHH) was synthesized by GeneCust Company (Dudelange, Luxemburg) and purity was confirmed by analytic HPLC.
2.3. Expression of pcDNA3.1-PEP in L929 cells
The L929 cells (murine fibroblast) were purchased from the Pastor Institute of Tehran, Iran. The cells were seeded at a density of 5 × 105 cells per well with RPMI containing 10% FBS. The pcDNA3.1-PEP was transfected to L929 cells by Turbofect (Thermo Scientific, USA) according to the manufacturer’s recommendations to explore its function in the eukaryotic host. Eight hours after transfection, culture media was replaced and incubated for another 48 h. Then, the peptide expression was detected by immunoblotting.
2.4. Immunoblotting
Immunoblotting analyses were used to confirm the expression of the multiepitope peptide vaccine at the transfected L929 fibroblasts. Five min boiling in the Tris-HCl (pH = 6.8, 0.5 M), SDS (10% W/V), Glycerol (50% V/V) and Bromo Phenol Blue (0.5% W/V) buffer (Sigma, USA) was utilized to obtain the protein solution of the transfected fibroblasts. A definite volume of samples was loaded in SDS-PAGE for separating protein bonds. The protein constituents were electrophoretically blotted onto a polyvinylidene difluoride membrane (Millipore, USA). PBS (pH 7.4) buffer containing 5% skim milk/0.1% Tween 20 was employed to block the membrane. Finally, the monoclonal Anti-poly-Histidine−Peroxidase antibody and tetramethylbenzidine substrate (TMB) were used to detect the peptide vaccine.Citation54
2.5. Mice immunization
Seven to eight weeks old female BALB/c mice (23 ± 2 g) were purchased from the Pasteur Institute of Tehran, Iran. All animal experiments and procedures were approved by the Ethics Committee on Animal Experiments of the Islamic Azad University of Tehran. The mice were randomly divided into six groups (n = 20). Each mouse at each group was injected four times with one-week intervals. Mice of group 1 were s.c injected with 100 μL PBS. Mice of group 2 were s.c injected with 100 μL incomplete Freund’s adjuvant (IFA) which was formulated with PBS in 50% V/V. Mice of group 3 were intramuscularly (i.m) injected with 100 µg pcDNA3-1. Mice of group 4 were immunized by intramuscular (i.m) injection of 100 µg recombinant pcDNA (pcDNA-PEP) in 100 μL PBS. Mice of group 5 were immunized by a mixture of the multiepitope peptide vaccine (100 μg in 50 μL of PBS) emulsified with 50 μL IFA 50% V/V. Mice of group 6 were immunized with 100 µg pcDNA in 100 µL PBS (i.m) for the first and second injections. They were injected with a mixture of the multiepitope peptide vaccine (100 μg in 50 μL PBS) emulsified with 50 μL IFA 50% V/V for the third and fourth injections. illustrates the vaccination schedule.
Table 1. Vaccination’s schedule at different groups
2.6. Antibodies determination by Enzyme-linked Immunosorbent Assay (ELISA)
Two weeks after the last injection, blood samples from five mice per group (n = 5) were collected for determination of the multiepitope peptide-specific antibodies at serum. IgG total, IgG1 and IgG2a antibodies were measured in the mice sera by indirect ELISA assay kit (R&D Systems, USA) according to the manufacturer’s instructions. In brief, the 96-well microtiter plates were coated with the peptide vaccine in 50 mM carbonate buffer (pH 9.6) overnight at 4°C. At the next day, the plates were washed three times with phosphate buffer solution (PBS) containing 0.05% Tween 20. Then, bovine serum albumin (BSA) 1% was added for 1 h at 37°C as the blocking solution. Subsequently, the wells were washed twice and incubated with the sera for 1 h at 37°C. HRP-conjugated goat anti-mouse IgG total, IgG1 or IgG2a (Abcam, USA) antibodies were employed as the secondary antibody. Finally, orthophenylene diamine (Sigma, USA) and 0.15% H2O2 were added for 30 min to visualize the immune complexes. A microplate reader (Bio-Rad 680, USA) was used to measure the absorbance at 490 nm after stopping the reaction. Three wells were used for each sample.
2.7. Cytokines assay
The immunized mice’s spleen was aseptically harvested for assessment of the cytokines production (n = 3), 14 days after the last vaccination. The mice were sacrificed and the skin was disinfected with 70% ethanol. A sterile scissor was used to harvest spleen at an aseptic condition. The harvested spleen was washed with RPMI at Petri dishes. Then, a scalpel was employed to cut the spleen into small pieces (~ 2 mm). These pieces were incubated with collagenase/DNase solution for 30 min at a cell culture incubator. In addition, the partially digested splenic tissues were compressed through the mesh of a 70 µm cell strainer using a 5 mL syringe plunger. After centrifuging, the pellet of cells was resuspended in RBC lysis buffer. The splenocytes were isolated by centrifuging after quenching the RBC lysis buffer by complete RPMI medium.Citation55 The splenocytes were cultured in 96-well cell culture plates and the cell-free supernatants were collected and restimulated with the peptide vaccine (10 mg/mL) and incubated for 60 h at standard cell culture condition. Subsequently, the supernatants were collected to investigate the cytokines’ level. Interleukin-4 (IL-4), interferon gamma (IFN-γ), and granzyme B (GrB) were measured by ELISA kits (R&D Systems, USA), following the manufacturer’s instruction. Briefly, the samples were added to the wells in triplicate. FBS enriched culture media (10%) was used as negative control. The definite volume of the antibody cocktail was added to the wells and incubated for 1 h. Then, the content of wells were aspirated and each well was washed three times with 350 µL of the wash buffer. Subsequently, the TMB solution was added and incubated for 5 min. Then, the stop solution was added and wells’ ODs were read at 450 nm wavelength by a microplate reader (Bio-Rad 680, USA).
2.8. Antigen-specific proliferation assay
The cultivated splenocytes were cultured at 96‑well plates and three wells were used for each sample. 1.5 × 105 cells were seeded at the bottom of each well. Then, the peptide vaccine (2 µg/well) was used to stimulate the cells as the recall antigen. Some wells weren’t stimulated to be used as negative control. Also, phytohemagglutinin‑A (PHA) (5 µg/mL) stimulated wells were used as positive control. After 72 h incubation in standard cell culture condition, 5‑bromo‑2 deoxyuridine (BrdU) was added to the wells and incubated for 24 h. Then the fixing solution was added. The wells were aspirated and washed three times and the primary detector antibody was added to the wells and incubated at room temperature. After aspirating and washing the wells, the HRP-conjugated antibody was added and incubated at room temperature. The wells were aspirated, washed and TMB was added to the wells. After color development, the stop solution was added. The stimulation index (SI) was calculated using the following formula:
2.9. RNA isolation and Real-Time PCR analyses
Total mRNA was extracted from the 4T1 cells and the harvested 4T1 tumors. Then, the samples were treated with DNase I (Invitrogen, USA) for avoiding DNA contamination. The cDNA was synthesized from 500 ng of total RNA primary tumor RNA, primed with oligo(dT)12–18, and reverse transcribed by SuperScript III reverse transcriptase (Invitrogen, USA) according to manufacturer’s instructions. The utilized primers for Real-Time PCR are mentioned at . RT-PCR was done on an Mx3000P QPCR System (Stratagene, CA) using QuantiTect SYBR Green PCR kit (Qiagen, Valencia, CA). Threshold values were normalized with respect to the β-actin expression. All experiments were repeated twice.Citation54
Table 2. The utilized primers for Real-Time PCR
2.10. Tumor challenge
4T1 cancer cells (murine mammary carcinoma) were purchased from the Pastor Institute of Tehran, Iran. The cells were harvested from culture flasks by trypsin (Sigma, USA) and washed three times with PBS. 106 cells which were suspended in 50 µL serum-free DMEM-F12 (Sigma, USA), were subcutaneously (s.c) injected at the fourth mammary fat pad of each mouse, two weeks after the last immunization. Tumor diameters were measured with a digital caliper directly on the mouse body every 3 days. As Figure S1 illustrates, the tumor-bearing mouse was grasped behind the neck firmly using the thumb and index finger, its body stretched along with the hand, and its tail was restrained using the last two fingers. Then, the greatest longitudinal diameter (D) and the greatest transverse diameter (d) of the tumor were measured. Tumor volume (mm3) was calculated based on the below equation.Citation56-58
2.11. Behavioral and histopathological investigations
For evaluating safety of the pcDNA3.1-PEP/Peptide regime, 10 healthy and non-tumor bearing mice were involved and randomly divided into two groups (n = 5) including 1) Control and 2) pcDNA3.1-PEP/Peptide. After immunization, the mice were monitored for general appearance and behavioral parameters for 30 days. They were under close monitored for any signs of toxicity and behavioral changes including weakness, salivation, anorexia, diarrhea, aggressiveness, eyes and ears discharge, noisy breathing, activity, convulsion, cachexia, pain or any signs of illness in each group.Citation59 At the 30th day, the mice were sacrificed and lungs, kidneys, and liver were harvested and fixed in 10% formalin neutral buffer solution. The tumors were fixed in 10% formalin neutral buffer solution. An automatic tissue processor (Sakura, Japan) was used to process the fixed specimens overnight for dehydration, clearing, and impregnation. Serial sections with 4 µm thickness were prepared from the paraffin-embedded specimens using a microtome (Leica Biosystems, Germany). The sections were stained by Hematoxylin & Eosin (H&E) protocol.Citation60,Citation61 The slides were reviewed by an expert pathologist under a light microscope (Olympus, Japan)and histological photographs were obtained.
2.12. Statistical analysis
The JMP 11.0 software was employed for performing statistical analyses. All data were compared between different groups by One-way ANOVA. the Kaplan-Meier method was utilized for comparing the survival time among the control and different treatment groups. Statistical significance was set at P < .05. (*:P < .05, **: P < .005, ***: P < .001, ns: not significant).
3. Results
3.1. Epitope analyses
The (RCQHKIAEMVALMEKHKHQYDKI) fragment from SYCP1 and (YIQYPNYCSFKSQQCL) fragment from ACRBP were selected due to containing multiple immunodominant epitopes. These selected fragments contained numerous epitopes for many alleles of human and mouse MHCs. Besides, the selected fragments had multiple T cell epitopes (CTLs) for mouse and human according to our previous in silico study.Citation53 The two selected regions were linked together with a furin-sensitive linker. Methionine and glycine at N-terminal and six histidines at C-terminal were added. At last, the total number of amino acids reached 51 residues. The schematic diagrams of the final construction of the multiepitope peptide and DNA vaccines are illustrated in .
3.2. Expression of the multiepitope peptide by the transfected murine fibroblast
One of the main determinative factors in the pcDNA effectiveness is its efficacy to cause expression of the target epitopes at the host cells. Immunoblotting analyses confirmed high expression of the multiepitope peptide by the transfected L929 fibroblasts. The peptide was located near the 10 kDa band ().
3.3. SYCP1 and ACRBP mRNA expression at 4T1 breast cancer cells in vitro and in vivo
Total mRNA from the 4T1 cell and the s.c implanted 4T1 tumors were extracted and used as the template for Real-Time PCR analyses. The Ct for the beta-actin, SYCP1 and ACRBP were 22, 22 and 30, respectively. These data can exhibit strong expression of SYCP1 and ACRBP mRNAs in 4T1 breast cancer cells in vitro and in vivo. The amplification plot is illustrated in .
Figure 3. Amplification plots of the SYCP1, ACRBP, and beta-actin mRNAs at 4T1 cells in vitro and in vivo. (a: beta-actin in vivo, b: beta-actin in vitro, c: SYCP1 in vivo, d: SYCP1 in vitro, e: ACRBP in vivo, f: ACRBP in vitro, g, h, and i: negative controls for SYCP1, ACRBP, and beta-actin, respectively)
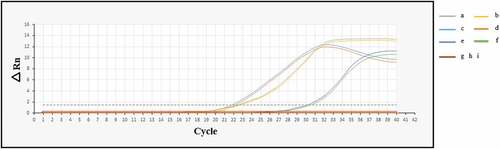
3.4. Humoral immune response
The serum level of the multiepitope peptide-specific IgG total in the immunized mice was assessed 14 days after the last injection. The pcDNA3.1-PEP, Peptide, and the pcDNA3.1-PEP/Peptide immunized mice sera had elevated IgG 1, IgG2a and IgG total levels in comparison with the control groups (PBS, IFA, and pcDNA3.1) (P < .001) (). The pcDNA3.1-PEP/Peptide immunized mice generated the highest levels of the multiepitope peptide-specific IgG total among all groups. The levels of IgG subclasses including IgG1 and IgG2a were assayed to determine the provocation of Th1 and Th2 responses (). An apparent predominance of IgG2a over IgG1 was detected in the pcDNA3.1-PEP immunized mice which suggests a shift toward the Th1 response (P < .05). However, the multiepitope peptide immunized group exhibited a Th2 shift response due to the IgG1 predominance (P < .05). Immunization with the pcDNA3.1-PEP/Peptide caused the highest level of IgG2a. In addition, the multiepitope peptide and pcDNA3.1-PEP/Peptide immunized mice exhibited the most IgG1 level, but their difference was not significant (P > .05).
3.5. Cytokines production
The immunized mice spleens were harvested 14 days after the last injection and their splenocytes were cultured. The splenocytes were stimulation in vitro with the multiepitope peptide. Then, IFN-γ and IL-4 cytokines production were measured (). The main aim of this experiment was to evaluate the Th1Citation62,Citation63 or Th2 responsesCitation64 at different immunization regimes according to the predominance of the secreted cytokines. All immunization regimes exhibited a significant increase in both cytokines production. The multiepitope peptide and pcDNA3.1-PEP/Peptide vaccinations exhibited the highest IL-4 production levels (). But they didn’t exhibit a significant difference in comparison with each other (P > .05). In addition, the pcDNA3.1-PEP/Peptide immunization regime caused the highest level of IFN-γ (P < .05) in comparison with other therapeutic regimes (). To detect cytotoxic activity, granzyme B production was measured. The splenocytes of all the immunized groups exhibited an enhanced ability to produce GrB in comparison with control groups (). The pcDNA3.1-PEP/Peptide vaccination group had the highest GrB production levels (P = .0001)
3.6. Lymphocytes proliferation
The lymphocytes which were obtained from the immunized groups (pcDNA3.1-PEP, Peptide and the pcDNA3.1-PEP/Peptide) exhibited significantly higher proliferation rates after the peptide vaccine stimulation in comparison with the PBS, adjuvant and pcDNA3.1 groups (). In addition, the obtained lymphocytes from the pcDNA3.1-PEP/Peptide immunized animals exhibited the highest SI among all groups (P < .05).
3.7. Tumors’ growth progression
4T1 murine mammary cancer cells were s.c implanted in the immunized mice to evaluate the prophylactic efficacy of the peptide and DNA vaccines at different immunization regimes. As illustrates, all the immunized mice exhibited reduced tumor growth in comparison with the PBS and IFA groups as controls. The PBS and IFA groups’ mean tumors’ volumes were almost the same at the last day of the challenge. While the pcDNA3.1-PEP/Peptide immunized group exhibited the most anti-tumor effect in comparison with controls (P < .001). Also, the pcDNA3.1-PEP/Peptide group had significantly decreased tumors’ volume in comparison with the multiepitope peptide vaccine and pcDNA3.1-PEP immunized mice (P < .05).
3.8. Tumor-bearing mice survival time
Survival days after 4T1 cancer cell implantation were evaluated in different groups (). The PBS or IFA injected groups didn’t exhibit any statistically significant difference between their mean survival times. However, mice immunized with the pcDNA3.1-PEP/Peptide regime exhibited the latest onset of death (beginning at 63rd day after the cancer cells implantation) and the longest survival time (83 days) among all groups (P < .001).
3.9. Vaccines’ safety
The cancer vaccines’ safety is very determinative for further clinical application. Therefore, the safety of the most effective immunization regime according to the tumors’ growth and survival analyzes was investigated. Non-tumor-bearing mice that were immunized with pcDNA3.1-PEP/Peptide regime were exactly monitored according to general appearance, behavioral parameters for 30 days and histopathological evaluation of the vital organs on the 30th day. No sign of changes at the mice’s appearance, behavioral pattern and food intake were observed during the 30 days after the last injection (Tables 3 S). On the 30th day, the animals were sacrificed and their vital organs including lungs, kidneys, and liver were harvested for histopathological investigations (). No-sign of organ damage was observed in H&E sections of the pcDNA3.1-PEP/Peptide group in comparison with the control.
4. Discussion
Utilizing from immunodominant epitopes of cancer-testis antigens for anti-breast cancer immunization has exhibited high efficacy.Citation34 In our previous study, different bioinformatic tools were employed for selecting the most immunodominant epitopes of ACRBP and SYCP1 antigens to design multiepitope DNA and peptide vaccines with significant ability to activate the immune system against breast cancer. CD8+ lymphocytes’ epitopes are very determinative in vaccine immunogenicity against tumors due to the high importance of cellular immunity in fighting malignant cells.Citation65,Citation66 The depletion of CD8+ lymphocytes can cause a significant decrease in the anti-tumor protection efficacy of the HER2/Neu DNA vaccine.Citation67 Therefore, the epitopes’ ability to bind to MHC-I and activating CTLs were the first parameters for the selection of the SYCP1 and ACRBP antigens’ fragments. The selected fragments were fused by a furin-sensitive linker. Utilizing from this linker can facilitate the epitopes’ presentation after processing in the trans-Golgi network and endoplasmic reticulum.Citation68 The final amino acid sequence was reverse-translated to nucleotides sequences and inserted in a pcDNA3.1 to construct the multiepitope DNA vaccine.
In vivo investigation of the in silico designed vaccines is necessary. Therefore, the DNA and peptide vaccines immunization regimes were assessed in the 4T1 murine mammary carcinoma model in BALB/c mice. 4T1 is a triple-negative breast cancer cell line that exhibits poor immunogenicity. Also, this cancer animal model has high similarities with human triple-negative breast cancer.Citation69,Citation70 The prerequisite condition for an effective immunization is the expression of the selected antigens by the tumor. Therefore, the expression of both SYCP1 and ACRBP were assessed. The high levels of these antigens’ mRNAs expression were detected in 4T1 cells both in vitro and in vivo. The pcDNA3.1-PEP immunization regime caused a significant increase at IgG total (4.5-fold, P < .001), IgG subclasses, IL-4 (4.3-fold, P < .001), and IFN-γ (11-fold, P < .001) in comparison with the negative controls (PBS and IFA). The multiepitope peptide vaccine was injected as a mixture with incomplete Freund’s adjuvant. Previous studies have shown that the use of adjuvants as the immune system activators causes different immune responses. However, IFA has a limited effect on cellular immunity activation.Citation71,Citation72 Therefore, all the changes at cytokines and antibodies profiles of the multiepitope peptide vaccine immunized mice can be attributed to the peptide vaccine per se. The multiepitope peptide vaccine immunization caused a significant increase at IgG total (9-fold, P < .001), IgG subclasses (with IgG1 dominance), IL-4 (9.9-fold, P < .001), and IFN-γ (12-fold, P < .001) in comparison with the control groups. This regime caused a higher increase at IgG1 than IgG2a isotype which identifies activation of Th2 and humoral immunity by the multiepitope peptide vaccine immunization. However, the pcDNA3.1-PEP caused cellular immunity dominance according to the significant secretion of IgG2a. Although the multiepitope peptide immunization regime had a significant superiority in comparison with the pcDNA3.1-PEP according to cytokines and antibodies production, the pcDNA3.1-PEP group had smaller tumors size. This can be attributed to the high preference of this regime for Th1 activation and subsequently stimulating the cell-mediated immune response. The obtained results showed that the most significant immune response and the antitumor effect was related to pcDNA3.1-PEP/Peptide immunization. Besides, the significant increase of tumor-bearing mice survival time was observed in the pcDNA3.1-PEP/Peptide group in comparison with the other groups. These results can be related to the stimulation of both T and B cells’ immune responses. Although some studies have reported the pivotal role of CD8+ lymphocytes for antitumor immune response, it should be noted that humoral immunity plays an undeniable role in fighting tumors through antibody-dependent cell cytotoxicity, opsonization and efficient antigen presentation.Citation73 Taking together, co-immunization with the DNA and peptide vaccines consisted of the immunodominant epitopes of SYCP1 and ACRBP antigens (pcDNA3.1-PEP/Peptide) could significantly activate both humoral and cellular immune responses against the murine triple-negative breast cancer to inhibit tumors’ growth.
Animal models have played an important role in cancer vaccine development and their translation to the clinical application.Citation74 However, cancer animal models have many limitations and most of the critical pitfalls of cancer vaccines can only be detected in clinical trials. This is due to the significant difference between the tumors and the immune system of humans and animals. Therefore, many orthotopic injection models, genetically engineered mice, xenograft and humanized models have been developed. However, they were all unable to completely recapitulate the complicated mechanism of malignant transformation which cause cancer development in human.Citation75 Murine cancer models including 4T1 breast cancer in BALB/c mice which was used in this study, provide important proof of concept for human studies. They are more economical and convenient than other animal cancer models. However, the necessity of utilizing from larger animal models can be felt for cancer vaccines investigation due to encompassing a fully competent immune system. Many studies have recommended utilizing from feline and canine models which may provide some advantages over other animal models like resolving immune tolerance issue. However, housing, ethical regulation, and breeding difficulties limit the employing of big animal models. Furthermore, even these models have limitations, and the idea of a universal model for cancer vaccine investigations currently seems unrealistic.Citation76,Citation77 Different patients exhibit significant heterogeneity at the same type of tumor. Even, apparent heterogeneity between different regions of the same tumor as well as between the primary tumor and its local or distant recurrent lesions at the same patient were reported by different studies. This heterogenicity can significantly affect the immunization outcome and vaccine efficacy.Citation78,Citation79 This vast heterogenicity may be absent in a particular cancer cell line or animal model. Therefore, further multiple animal experiments and clinical trials for the investigation of different aspects of these cancer vaccines’ efficacy is necessary.
Currently, surgical resection of the primary tumor is the main therapeutic approach for most of the patients with solid tumors. Unfortunately, a high proportion of these patients face tumor relapse and metastasis within 5 years.Citation80 Many clinical and preclinical studies have reported that surgical resection can facilitate tumor recurrence and metastasis due to surgery-induced immune system suppression. This postoperative immunosuppression window can create an opportunity for the expansion of residual cancer cells and metastasis formation.Citation81-84 However, emerging preclinical and clinical studies have revealed that postoperative immune suppression is reversible. Patients recovering from surgery don’t receive adjuvant chemotherapy or radiotherapy due to the negative effects of these interventions on wound repair process. On the other hand, some previous studies have reported that the failures of cancer vaccines to control tumor’s growth is due to the negative regulatory mechanisms invoked by the primary tumor to unable the anti-tumor immune response to achieve its full potential. Most tumors that become clinically evident have already escaped natural immunosurveillance by using these negative regulatory mechanisms. Therefore, the postoperative period is a golden opportunity for activation of the ultimate anti-tumor immune response as the primary tumor’s negative regulatory effects have been omitted and residual cancer cells are susceptible to immune response. Therefore, the combination of surgery and immunotherapy to prevent postoperative cancer recurrence and metastasis has gained lots of attention.Citation85,Citation86 As surgery is the main therapeutic approach for most of the breast cancer patients; we suggest the application of these novel cancer vaccines for the prevention of breast cancer recurrence and metastasis. Breast cancer patients can be immunized by these cancer vaccines at the postoperative period for activating the immune system to prevent tumor recurrence and metastasis. Before vaccination, evaluation of SYCP1 and ACRBP expression status at the patient’s resected tumor is necessary and only patients with high expression of both target antigens at the resected primary tumor should enter the trail to increase the success probability. However, further animal experiments, especially big animals’ cancer models and humanized mice experiments are needed to evaluate these vaccines’ efficacy from different angles before planning for further clinical trials. In further animal experiments, we suggest to use these vaccines after the primary tumor resection at the postoperative period to investigate their efficacy for preventing and inhibiting of the tumor recurrence and metastasis.
5. Conclusions
Cancer vaccines have gained lots of attention for the prevention of tumor recurrence and metastasis. The multiepitope DNA and peptide vaccines consisted of the most immunodominant epitopes of SYCP1 and ACRBP as two well-known cancer/testis antigens could effectively activate both cellular and humoral immune responses against 4T1 murine breast tumor. Also, this prophylactic co-immunization could significantly inhibit the growth of this murine triple-negative breast tumor. However, more animal experiments are needed to demonstrate these vaccines’ efficacy in different aspects.
Disclosure of potential conflicts of interest
There are no competing interests to be declared.
Acknowledgments
This study is a part of a Ph.D. thesis and was supported by the Science and Research Branch of Islamic Azad University (Grant number: 11-940915), Tehran, Iran.
Additional information
Funding
References
- Bray F, Ferlay J, Soerjomataram I, Siegel RL, Torre LA, Jemal A. Global cancer statistics 2018: GLOBOCAN estimates of incidence and mortality worldwide for 36 cancers in 185 countries. CA: Cancer J Clinicians. 2018;68(6):394–424.
- Siegel RL, Miller KD, Jemal AJCACJFC. Cancer statistics, 2019. CA Cancer J Clin. 2019;69(1):7–34.
- Erenpreisa J, Cragg MS. Three steps to the immortality of cancer cells: senescence, polyploidy and self-renewal. Cancer Cell Int. 2013;13(1):92. doi:10.1186/1475-2867-13-92.
- Ghahremani F, Shahbazi-Gahrouei D, Kefayat A, Motaghi H, Mehrgardi MA, Javanmard SH. AS1411 aptamer conjugated gold nanoclusters as a targeted radiosensitizer for megavoltage radiation therapy of 4T1 breast cancer cells. RSC Adv. 2018;8(8):4249–58. doi:10.1039/C7RA11116A.
- Sharma GN, Dave R, Sanadya J, Sharma P, Sharma KK. Various types and management of breast cancer: an overview. J Adv Pharm Technol Res. 2010;1(2):109.
- Khanfir A, Lahiani F, Bouzguenda R, Ayedi I, Daoud J, Frikha M. Prognostic factors and survival in metastatic breast cancer: a single institution experience. Rep Pract Oncol Radiother. 2013;18(3):127–32. doi:10.1016/j.rpor.2013.01.001.
- Arpino G, Milano M, De Placido S. Features of aggressive breast cancer. Breast. 2015;24(5):594–600. doi:10.1016/j.breast.2015.06.001.
- Redig AJ, McAllister SS. Breast cancer as a systemic disease: a view of metastasis. J Intern Med. 2013;274(2):113–26. doi:10.1111/joim.12084.
- Montano, D.J.J.o.O.M. and Toxicology. Chemical and biological work-related risks across occupations in Europe: a review. J Occup Med Toxicol. 2014;9(1):28.
- Stuckey AR, Onstad MA, Gynecology. Hereditary breast cancer: an update on risk assessment and genetic testing in 2015. Am J Obstetrics Gynecol. 2015;213(2):161–65.
- Rath MG, Masciari S, Gelman R, Miron A, Miron P, Foley K, Richardson AL, Krop IE, Verselis SJ, Dillon DA, et al. Prevalence of germline TP53 mutations in HER2+ breast cancer patients. Breast Cancer Res Treat. 2013;139(1):193–98.
- Weiderpass E, Meo M, Vainio H. Risk factors for breast cancer, including occupational exposures. Saf Health Work.. 2011;2(1):1–8.
- Finn O. Immuno-oncology: understanding the function and dysfunction of the immune system in cancer. Ann Oncol. 2012;23(suppl_8):viii6–viii9. doi:10.1093/annonc/mds256.
- Shore ND. Advances in the understanding of cancer immunotherapy. BJU Int. 2015;116(3):321–29. doi:10.1111/bju.12692.
- Umar A. Cancer immunoprevention: a new approach to intercept cancer early. Cancer Prev Res. 2014 p. canprevres. 0213.2014;7(11):1067–71. doi:10.1158/1940-6207.CAPR-14-0213.
- Vinay DS, Ryan EP, Pawelec G, Talib WH, Stagg J, Elkord E, Lichtor T, Decker WK, Whelan RL, Kumara HMCS, et al. Immune evasion in cancer: mechanistic basis and therapeutic strategies. Semin. Cancer Biol. 2015 Dec;35 Suppl:S185–S198.
- Yang B, Jeang J, Yang A, Wu TC, Hung C-F. DNA vaccine for cancer immunotherapy. Hum Vaccin Immunother. 2014;10(11):3153–64. doi:10.4161/21645515.2014.980686.
- Tiptiri-Kourpeti A, Spyridopoulou K, Pappa A, Chlichlia K. DNA vaccines to attack cancer: strategies for improving immunogenicity and efficacy. Pharmacol Ther. 2016;165:32–49. doi:10.1016/j.pharmthera.2016.05.004.
- Norell H, Poschke I, Charo J, Wei WZ, Erskine C, Piechocki MP, Knutson KL, Bergh J, Lidbrink E, Kiessling R, et al. Vaccination with a plasmid DNA encoding HER-2/neu together with low doses of GM-CSF and IL-2 in patients with metastatic breast carcinoma: a pilot clinical trial. J Transl Med. 2010;8(1):53. doi:10.1186/1479-5876-8-53.
- Tiriveedhi V, Fleming TP, Goedegebuure PS, Naughton M, Ma C, Lockhart C, Gao F, Gillanders WE, Mohanakumar T. Mammaglobin-A cDNA vaccination of breast cancer patients induces antigen-specific cytotoxic CD4+ ICOS hi T cells. Breast Cancer Res Treat. 2013;138(1):109–18. doi:10.1007/s10549-012-2110-9.
- de Paula Peres L, da Luz FAC, Dos Anjos Pultz B, Brígido PC, de Araújo RA, Goulart LR, Silva MJB. Peptide vaccines in breast cancer: the immunological basis for clinical response. Biotechnol Adv. 2015;33(8):1868–77. doi:10.1016/j.biotechadv.2015.10.013.
- Thundimadathil J. Cancer treatment using peptides: current therapies and future prospects. J Amino Acids. 2012;2012:967347. doi:10.1155/2012/967347.
- Finn OJ. Cancer vaccines: between the idea and the reality. Nat Rev Immunol. 2003;3(8):630. doi:10.1038/nri1150.
- Vigneron N. Human tumor antigens and cancer immunotherapy. Biomed Res Int. 2015;2015:1–17. doi:10.1155/2015/948501.
- Coulie PG, Brichard V, Van Pel A, Wölfel T, Schneider J, Traversari C, Mattei S, De Plaen E, Lurquin C, Szikora JP, et al. A new gene coding for a differentiation antigen recognized by autologous cytolytic T lymphocytes on HLA-A2 melanomas. The Journal of Experimental Medicine. 1994;180(1):35–42. doi:10.1084/jem.180.1.35.
- Robbins PF, El-Gamil M, Li YF, Kawakami Y, Loftus D, Appella E, Rosenberg SA. A mutated beta-catenin gene encodes a melanoma-specific antigen recognized by tumor infiltrating lymphocytes. The Journal of Experimental Medicine. 1996;183(3):1185–92. doi:10.1084/jem.183.3.1185.
- Kraus MH, Popescu NC, Amsbaugh SC, King CR. Overexpression of the EGF receptor-related proto-oncogene erbB-2 in human mammary tumor cell lines by different molecular mechanisms. Embo J. 1987;6(3):605. doi:10.1002/j.1460-2075.1987.tb04797.x.
- Demetris Iacovides SM, Achilleos C, Strati K. Shared mechanisms in stemness and carcinogenesis: lessons from oncogenic viruses. Front Cell Infect Microbiol. 2013;3. doi:10.3389/fcimb.2013.00072.
- Lurquin C, De Smet C, Brasseur F, Muscatelli F, Martelange V, De Plaen E, Brasseur R, Monaco AP, Boon T. Two members of the humanmagebgene family located in Xp21. 3 are expressed in tumors of various histological origins. Genomics. 1997;46(3):397–408. doi:10.1006/geno.1997.5052.
- Chomez P, De Backer O, Bertrand M, De Plaen E, Boon T, Lucas S. An overview of the MAGE gene family with the identification of all human members of the family. Cancer Res. 2001;61:5544–51.
- Tarnowski M, Czerewaty M, Deskur A, Safranow K, Marlicz W, Urasińska E, Ratajczak MZ, Starzyńska T. Expression of cancer testis antigens in colorectal cancer: new prognostic and therapeutic implications. Dis Markers. 2016;2016:1–9. doi:10.1155/2016/1987505.
- Wadle A, Kubuschok B, Imig J, Wuellner B, Wittig C, Zwick C, Mischo A, Waetzig K, Romeike BFM, Lindemann W, et al. Serological immune response to cancer testis antigens in patients with pancreatic cancer. Int J Cancer. 2006;119(1):117–25. doi:10.1002/ijc.21744.
- Zajac P, Schultz-Thater E, Tornillo L, Sadowski C, Trella E, Mengus C, Iezzi G, Spagnoli GC. MAGE-A antigens and cancer immunotherapy. Front Med. 2017;4:18. doi:10.3389/fmed.2017.00018.
- Gjerstorff MF, Andersen MH, Ditzel HJ. Oncogenic cancer/testis antigens: prime candidates for immunotherapy. Oncotarget. 2015;6(18):15772. doi:10.18632/oncotarget.4694.
- Neumann F, Wagner C, Preuss K-D, Kubuschok B, Schormann C, Stevanovic S, Pfreundschuh M. Identification of an epitope derived from the cancer testis antigen HOM-TES-14/SCP1 and presented by dendritic cells to circulating CD4+ T cells. Blood. 2005;106(9):3105–13. doi:10.1182/blood-2005-04-1487.
- Türeci O, Sahin U, Zwick C, Koslowski M, Seitz G, Pfreundschuh M. Identification of a meiosis-specific protein as a member of the class of cancer/testis antigens. Proc Natl Acad Sci. 1998;95(9):5211–16. doi:10.1073/pnas.95.9.5211.
- Tammela J, Uenaka A, Ono T, Noguchi Y, Jungbluth AA, Mhawech-Fauceglia P, Qian F, Schneider S, Sharma S, Driscoll D, et al. OY-TES-1 expression and serum immunoreactivity in epithelial ovarian cancer. Int J Oncol. 2006;29(4):903–10.
- Ono T, Kurashige T, Harada N, Noguchi Y, Saika T, Niikawa N, Aoe M, Nakamura S, Higashi T, Hiraki A, et al. Identification of proacrosin binding protein sp32 precursor as a human cancer/testis antigen. Proc Natl Acad Sci. 2001;98(6):3282–87. doi:10.1073/pnas.041625098.
- Costa Y, Speed R, Öllinger R, Alsheimer M, Semple CA, Gautier P, Maratou K, Novak I, Höög C, Benavente R and Cooke HJ, Two novel proteins recruited by synaptonemal complex protein 1 (SYCP1) are at the centre of meiosis. J Cell Sci. 2005;118(12):2755–62. doi:10.1242/jcs.02402.
- Sahin U, Koslowski M, Türeci O, Eberle T, Zwick C, Romeike B, Moringlane JR, Schwechheimer K, Feiden W, Pfreundschuh M, et al. Expression of cancer testis genes in human brain tumors. Clin Cancer Res. 2000;6(10):3916–22.
- Kubuschok B, Xie X, Jesnowski R, Preuss K-D, Romeike BFM, Neumann F, Regitz E, Pistorius G, Schilling M, Scheunemann P, et al. Expression of cancer testis antigens in pancreatic carcinoma cell lines, pancreatic adenocarcinoma and chronic pancreatitis. Int J Cancer. 2004;109(4):568–75. doi:10.1002/ijc.20006.
- Luo G, Huang S, Xie X, Stockert E, Chen YT, Kubuschok B, Pfreundschuh M. Expression of cancer-testis genes in human hepatocellular carcinomas. Cancer Immun Arch. 2002;2(1):11.
- Mashino K, Sadanaga N, Tanaka F, Yamaguchi H, Nagashima H, Inoue H, Sugimachi K, Mori M. Expression of multiple cancer-testis antigen genes in gastrointestinal and breast carcinomas. Br J Cancer. 2001;85(5):713. doi:10.1054/bjoc.2001.1974.
- Whitehurst AW, Xie Y, Purinton SC, Cappell KM, Swanik JT, Larson B, Girard L, Schorge JO, White MA. Tumor antigen acrosin binding protein normalizes mitotic spindle function to promote cancer cell proliferation. Cancer Res. 2010;70(19):7652–61. doi:10.1158/0008-5472.
- Salmaninejad A, Zamani MR, Pourvahedi M, Golchehre Z, Hosseini Bereshneh A, Rezaei N. Cancer/testis antigens: expression, regulation, tumor invasion, and use in immunotherapy of cancers. Immunol Invest. 2016;45(7):619–40. doi:10.1080/08820139.2016.1197241.
- Fratta E, Coral S, Covre A, Parisi G, Colizzi F, Danielli R, Marie Nicolay HJ, Sigalotti L, Maio M. The biology of cancer testis antigens: putative function, regulation and therapeutic potential. Mol Oncol. 2011;5(2):164–82. doi:10.1016/j.molonc.2011.02.001.
- Tang C-T, Li P-C, Liu I-J, Liao M-Y, Chiu C-Y, Chao D-Y, Wu H-C. An epitope-substituted DNA vaccine improves safety and immunogenicity against dengue virus type 2. PLoS Negl Trop Dis. 2015;9(7):e0003903. doi:10.1371/journal.pntd.0003903.
- Okuyama H, Tominaga A, Fukuoka S, Taguchi T, Kusumoto Y, Ono S. Spirulina lipopolysaccharides inhibit tumor growth in a Toll-like receptor 4-dependent manner by altering the cytokine milieu from interleukin-17/interleukin-23 to interferon-γ. Oncol Rep. 2017;37(2):684–94. doi:10.3892/or.2017.5346.
- Beatty GL, Gladney WL. Immune escape mechanisms as a guide for cancer immunotherapy. Clin Cancer Res. 2015;21(4):687–92. doi:10.1158/1078-0432.CCR-14-1860.
- Song S, Wang F, He X, He Y, Li D, Sun S. Evaluation of antitumor immunity efficacy of epitope-based vaccine with B16 cell line coexpressing HLA-A2/H-2kb and CTL multiepitope in HLA transgenic mice. Vaccine. 2007;25(25):4853–60. doi:10.1016/j.vaccine.2006.12.029.
- Mahdavi M, Mohabatkar H, Keyhanfar M, Dehkordi AJ, Rabbani M. Linear and conformational B cell epitope prediction of the HER 2 ECD-subdomain III by in silico methods. Asian Pac J Cancer Prev. 2012;13(7):3053–59. doi:10.7314/APJCP.2012.13.7.3053.
- Chen P, Rayner S, Hu K-H. Advances of bioinformatics tools applied in virus epitopes prediction. Virol Sin. 2011;26(1):1–7. doi:10.1007/s12250-011-3159-4.
- Safavi A, Kefayat A, Sotoodehnejadnematalahi F, Salehi M, Modarressi MH. In silico analysis of synaptonemal complex protein 1 (SYCP1) and acrosin binding protein (ACRBP) antigens to design novel multiepitope peptide cancer vaccine against breast cancer. Int J Pept Res Ther. 2018;25(4):1343–59.
- Safavi A, Kefayat A, Sotoodehnejadnematalahi F, Salehi M, Modarressi MH. Production, purification, and in vivo evaluation of a novel multiepitope peptide vaccine consisted of immunodominant epitopes of SYCP1 and ACRBP antigens as a prophylactic melanoma vaccine. Int Immunopharmacol. 2019;76:105872. doi:10.1016/j.intimp.2019.105872.
- Arora P, Porcelli SAJJ. An efficient and high yield method for isolation of mouse dendritic cell subsets. J Vis Exp. 2016;(110):e53824. doi:10.3791/53824.
- Kefayat A, Ghahremani F, Motaghi H, Mehrgardi M. Investigation of different targeting decorations effect on the radiosensitizing efficacy of albumin-stabilized gold nanoparticles for breast cancer radiation therapy. Eur J Pharm Sci. 2019;130:225–33. doi:10.1016/j.ejps.2019.01.037.
- Bailey-Downs LC, Thorpe JE, Disch BC, Bastian A, Hauser PJ, Farasyn T, Berry WL, Hurst RE, Ihnat MA. Development and characterization of a preclinical model of breast cancer lung micrometastatic to macrometastatic progression. PLoS One. 2014;9(5):e98624. doi:10.1371/journal.pone.0098624.
- Safavi A, Kefayat A, Ghahremani F, Mahdevar E, Moshtaghian J. Immunization using male germ cells and gametes as rich sources of cancer/testis antigens for inhibition of 4T1 breast tumors’ growth and metastasis in BALB/c mice. Int Immunopharmacol. 2019;74:105719. doi:10.1016/j.intimp.2019.105719.
- Rajeh MAB, Kwan YP, Zakaria Z, Latha LY, Jothy SL, Sasidharan S. Acute toxicity impacts of Euphorbia hirta L extract on behavior, organs body weight index and histopathology of organs of the mice and Artemia salina. Pharmacogn Res. 2012;4(3):170.
- Ghahremani F, Kefayat A, Shahbazi-Gahrouei D, Motaghi H, Mehrgardi MA and Haghjooy-Javanmard S. AS1411 aptamer-targeted gold nanoclusters effect on the enhancement of radiation therapy efficacy in breast tumor-bearing mice. Nanomedicine. 2018;13(20):2563–78.
- Ibrahim K, Al-Mutary M, Bakhiet A, Khan H. Histopathology of the liver, kidney, and spleen of mice exposed to gold nanoparticles. Molecules. 2018;23(8):E1848. doi:10.3390/molecules23081848.
- Smeltz RB, Chen J, Ehrhardt R, Shevach EM. Role of IFN-γ in Th1 differentiation: IFN-γ regulates IL-18Rα expression by preventing the negative effects of IL-4 and by inducing/maintaining IL-12 receptor β2 expression. J Immunol. 2002;168(12):6165–72. doi:10.4049/jimmunol.168.12.6165.
- Bradley LM, Dalton DK, Croft M. A direct role for IFN-gamma in regulation of Th1 cell development. J Immunol. 1996;157:1350–58.
- Paludan S. Interleukin-4 and interferon-gamma: the quintessence of a mutual antagonistic relationship. Scand J Immunol. 1998;48(5):459–68. doi:10.1046/j.1365-3083.1998.00435.x.
- Krishna S, Anderson KS. T-cell epitope discovery for therapeutic cancer vaccines. Vaccine Design. Methods Mol Biol. 2016;1403:779–96. doi:10.1007/978-1-4939-3387-7_45.
- Zhang H, Huang W. Fusion proteins of Hsp70 with tumor-associated antigen acting as a potent tumor vaccine and the C-terminal peptide-binding domain of Hsp70 being essential in inducing antigen-independent anti-tumor response in vivo. Cell Stress Chaperones. 2006;11(3):216. doi:10.1379/CSC-191R.1.
- Lin -C-C, Chou C-W, Shiau A-L, Tu C-F, Ko T-M, Chen Y-L, Yang B-C, Tao M-H, Lai M-D. Therapeutic HER2/Neu DNA vaccine inhibits mouse tumor naturally overexpressing endogenous neu. Mol Ther. 2004;10(2):290–301. doi:10.1016/j.ymthe.2004.05.015.
- Lu J, Higashimoto Y, Appella E, Celis E. Multiepitope Trojan antigen peptide vaccines for the induction of antitumor CTL and Th immune responses. J Immunol. 2004;172(7):4575–82. doi:10.4049/jimmunol.172.7.4575.
- Pulaski BA, Ostrand‐Rosenberg S. Mouse 4T1 breast tumor model. Curr Protoc Immunol. 2000;39(1):20.2. 1–20.2. 16. doi:10.1002/0471142735.im2002s39.
- Kaur P, Nagaraja GM, Zheng H, Gizachew D, Galukande M, Krishnan S, Asea A. A mouse model for triple-negative breast cancer tumor-initiating cells (TNBC-TICs) exhibits similar aggressive phenotype to the human disease. BMC Cancer. 2012;12(1):120. doi:10.1186/1471-2407-12-120.
- Sivakumar S, Safhi MM, Kannadasan M, Sukumaran N. Vaccine adjuvants – current status and prospects on controlled release adjuvancity. Saudi Pharm J. 2011;19(4):197–206. doi:10.1016/j.jsps.2011.06.003.
- Machluf N, Arnon R. Therapeutic MUC1-based cancer vaccine expressed in flagella-efficacy in an aggressive model of breast cancer. World J Vaccines. 2012;2(3):109. doi:10.4236/wjv.2012.23015.
- Miyako H, Kametani Y, Katano I, Ito R, Tsuda B, Furukawa A, Saito Y, Ishikawa D, Ogino K, Sasaki S, et al. Antitumor effect of new HER2 peptide vaccination based on B cell epitope. Anticancer Res. 2011;31(10):3361–68.
- Gerdts V, Littel-van den Hurk SVD, Griebel PJ, Babiuk, LA. Use of animal models in the development of human vaccines. Future Microbiol. 2007;2(6):667–75.
- Overgaard NH, Fan TM, Schachtschneider KM, Principe DR, Schook LB and Jungersen G. Of mice, dogs, pigs, and men: choosing the appropriate model for Immuno-oncology research. ILAR J. 2018;59(3):247–62.
- Wei W-Z, Jones RF, Juhasz C, Gibson H, Veenstra J. Evolution of animal models in cancer vaccine development. Vaccine. 2015;33(51):7401–07.
- Lopes A, Vandermeulen, G and Préat V. Cancer DNA vaccines: current preclinical and clinical developments and future perspectives. J Exp Clin Cancer Res. 2019;38(1):146.
- Bedard PL, Hansen AR, Ratain MJ, Siu LL. Tumour heterogeneity in the clinic. Nature. 2013;501(7467):355–64.
- Andrechek ER, Nevins JRJJOMM. Mouse models of cancers: opportunities to address heterogeneity of human cancer and evaluate therapeutic strategies. J Mol Med. 2010;88(11):1095–100.
- Seth R, Tai, LH, Falls, T, de Souza, CT, Bell, JC, Carrier, M, Atkins, H, Boushey, R and Auer, RA. Surgical stress promotes the development of cancer metastases by a coagulation-dependent mechanism involving natural killer cells in a murine model. Ann Surg. 2013;258(1):158–68.
- Tai L-H, de Souza CT, Bélanger S, Ly L, Alkayyal AA, Zhang J, Rintoul JL, Ananth AA, Lam T, Breitbach CJ, Falls TJ. Preventing postoperative metastatic disease by inhibiting surgery-induced dysfunction in natural killer cells. Cancer Res. 2013;73(1):97–107.
- Ananth AA, Tai LH, Lansdell C, Alkayyal AA, Baxter KE, Angka L, Zhang J, de Souza CT, Stephenson KB, Parato K and Bramson, JL. Surgical stress abrogates pre-existing protective T cell mediated anti-tumor immunity leading to postoperative cancer recurrence. PLoS One. 2016;11(5).
- Yakar I, Melamed R, Shakhar G, Shakhar K, Rosenne E, Abudarham N, Page GG, Ben-Eliyahu S. Prostaglandin E 2 suppresses NK activity in vivo and promotes postoperative tumor metastasis in rats. Ann Surg Oncol. 2003;10(4):469.
- Melamed R, Rosenne, E., Shakhar, K., Schwartz, Y., Abudarham, N. and Ben-Eliyahu, S. Marginating pulmonary-NK activity and resistance to experimental tumor metastasis: suppression by surgery and the prophylactic use of a β-adrenergic antagonist and a prostaglandin synthesis inhibitor. Brain Behav Imm. 2005;19(2):114–26.
- Maeng H, Terabe M, Berzofsky JAJCOII. Cancer vaccines: translation from mice to human clinical trials. Curr Opin Immunol. 2018;51:111–22.
- Bakos O, Lawson C, Rouleau S, Tai LH. Combining surgery and immunotherapy: turning an immunosuppressive effect into a therapeutic opportunity. J Immunother Cancer. 2018;6(1):1–11.