ABSTRACT
A microneedle array patch (MAP) has been developed as a new delivery system for vaccines. Preclinical and clinical trials with a vaccine MAP showed improved stability, safety, and immunological efficacy compared to conventional vaccine administration. Various vaccines can be delivered with a MAP. Currently, microneedle manufacturers can mass-produce pharmaceutical MAP and cosmetic MAP and this mass-production system can be adapted to produce a vaccine MAP. Clinical trials with a vaccine MAP have shown comparable efficacy with conventional administration, and discussions about regulations for a vaccine MAP are underway. However, there are concerns of reasonable cost, mass production, efficacy, and safety standards that meet FDA approval, as well as the need for feedback regarding the best method of administration. Currently, microneedles have been studied for the delivery of many kinds of vaccines, and preclinical and clinical studies of vaccine microneedles are in progress. For the foreseeable future, some vaccines will continue to be administered with syringes and needles while the use of a vaccine MAP continues to be improved because of the advantages of less pain, self-administration, improved stability, convenience, and safety.
KEYWORDS:
1. Introduction
The World Health Organization (WHO) estimates that immunization annually prevents 2–3 million deaths, and vaccination is the most powerful tool to protect people from infectious diseases. Despite the successes of the vaccine era, there were approximately 15 million deaths from such diseases globally in 2010.Citation1 Thus, vaccine development, vaccine coverage, and mass vaccination continue to be WHO’s top priorities because many countries still suffer from the enormous burden of disease owing to influenza, HIV/AIDS, tuberculosis, hepatitis B, and especially the emerging infectious diseases such as the Ebola virus, the Zika virus, and other pathogens. Most conventional vaccinations have been injected with needles and syringes. Syringes and needles have provided successful delivery of predetermined doses of vaccines, and many diseases have been brought under control or virtually eliminated through syringe and needle-based vaccination. However, syringes and needles have several disadvantages such as pain, needle-stick injuries, needle reuse, and poor patient compliance.Citation2 In addition, the cold chain of manufacturing, delivery, and storage of vaccines increases the cost, the likelihood of misuse, and risk during clinic practice.Citation3 The cost of vaccination programs in 94 low- and middle-income countries over the decade 2011–2020 has been about 62 USD billion. The delivery cost was 34 USD billion and the supply chain cost was 4 USD billion, corresponding to 54% and 6%, respectively, of total vaccine cost.Citation4 The high cost of vaccines is one of the main barriers to vaccination coverage in low- and middle-income countries, and according to 2015 survey data, only 60% of eligible children in these countries had received full immunization.Citation5
To overcome these limitations, vaccination with a microneedle array patch (MAP) was introduced because a MAP has the advantages of improved stability, delivery, and storage at room temperature, low bioburden, painlessness, minimally invasive nature, self-administration, and intradermal delivery of antigens into the skin, as shown in .Citation6 The length of microneedles in a MAP ranges from 100 to 1000 µm, and the vaccine can be delivered into the epidermal and dermal layers of human skin where Langhans cells and dendritic cells are located.Citation7–9 Three types of MAP – solid MAP (S-MAP), coated MAP (C-MAP), and dissolving MAP (D-MAP) – have been used to test the immunization application. As shown in , the S-MAP delivers the vaccine into the deeper layer of the skin through the holes generated by the MAP. A C-MAP delivers the vaccine formulation directly into the skin layer and releases the vaccine immediately after being inserted into the skin. A D-MAP is made of safe, inert, water-soluble materials, and the vaccine is released from the matrix of the D-MAP after it is inserted into the skin.
Table 1. Microneedle array patch (MAP) solution for vaccine needs
Figure 1. Illustration of vaccine microneedle array patch (MAP) types: (a) solid MAP (S-MAP), (b) coated MAP (C-MAP), (c) dissolving MAP (D-MAP). Arrows show the direction of vaccine diffusion. Representative images of S-MAP (1), C-MAP (2), and D-MAP (3)
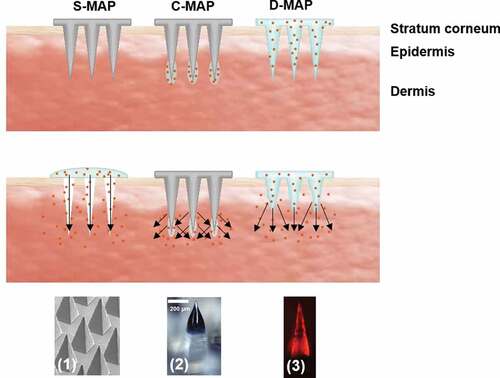
The first study of immunization by MAP was carried out in 2002,Citation10 and the significant milestones of MAP for vaccination are displayed in . The use of a D-MAP for influenza vaccination was well tolerated and generated robust antibody responses in 2017, and the first clinical trials with vaccine MAPs were conducted in 2015.
In this review, the studies of MAP for vaccination are summarized and analyzed with sections addressing preclinical studies, stability studies, and clinical trials. In addition, manufacturing issues, regulation considerations, and future possibilities are discussed to provide a total view of the application of MAP as the alternative means of vaccination. In this review, hollow microneedles are not discussed. S-MAP, C-MAP, and D-MAP for delivery of vaccine are discussed because these three types of MAP have been developed for the delivery of solidified vaccine formulations.
summarizes the criteria when selecting S-MAP, C-MAP and D-MAP. In regard to the manufacturing process, the drug is not loaded or coated onto S-MAPs, so the manufacturing cost is cheaper than for C-MAPs or D-MAPs. However, because S-MAPs have to puncture the skin to deliver the drug, the amount of drug delivered is small and inconsistent. In the case of C-MAPs and D-MAPs, the wear time (i.e., time needed to keep the microneedles attached to the skin) is longer (from a few minutes to 30 min) because sufficient adhesion time is required to deliver all of the drug in the coating formulation of the C-MAP or in the D-MAP matrix. Because the D-MAP matrix is also dissolved in the skin, the mechanical strength and biocompatibility of the microneedle material are critical concerns.Citation11 Compared to S-MAPs or precoated microneedles of C-MAPs made of water-insoluble polymer, most D-MAPs are made of soluble polymers, which potentially makes them more likely to break down as a result of contact with moisture.Citation12,Citation13
Table 2. Decision matrix for use of solid microneedle array patch (S-MAP), coated microneedle array patch (C-MAP), and dissolving microneedle array patch (D-MAP)
2. preclinical and stability studies
2.1. Preclinical studies of vaccine MAP
Preclinical studies of various vaccine MAPs have been conducted and are summarized in . Mice were used to test the efficacy of most vaccine MAPs, and a monkey was used as an animal model. Among the adjuvants, an aluminum-type adjuvant has been studied; however, it did not show high efficacy for a vaccine MAPCitation14 because it induced low T-cell mediated immune responses and it was not suitable for intradermal (ID) use.Citation15 The preclinical studies of adjuvants for MAPs are summarized in . Recently, nanoparticles (NPs) have been considered an effective adjuvant because they can act as a depot and are more efficiently taken up by dendritic cells.Citation16,Citation17 NPs prepared from chitosan induced an equally strong immune response compared to subcutaneous injection of diphtheria and tetanus (DT) vaccineCitation18 and generated a higher IgG2a titer than commercial tetanus toxin vaccine.Citation19 Liposome NPs are a candidate for vaccine MAP application; the mucosal injection elicited robust systemic and widespread immune response in hepatitis B MAP.Citation20 However, a study with the liposome formulation of DT did not induce a higher antibody than free DT.Citation21 NPs were fabricated from polymer as poly(lactic-co-glycolic acid)-PLGA with anthrax vaccine, and NPs were encapsulated in a MAP. An anthrax vaccine NP MAP induced a stronger immune response than a MAP without an NP formulation.Citation22 A similar enhancement was observed in Ebola vaccine research.Citation23 Another polymer NP formulation was prepared from pluronic-modified polyethyleneimine, and hepatitis B DNA vaccine was encapsulated in NPs. DNA NP MAPs generated higher humoral and cellular immunity than DNA MAPs.Citation24
Table 3. Vaccine and model animals regarding type of microneedle array patch (MAP)
Table 4. Preclinical studies of vaccine microneedle array patch (MAP) with adjuvants
The immune response was improved by the sustained release of vaccine and exposure of antigens to lymphoid tissues by using an implantable MAP. The D-MAP for HIV was fabricated with the silk matrix to control the release rate of the antigen for 2 weeks, and the serum IgG titer was increased 1,300-fold compared to conventional administration.Citation25 The release of influenza vaccine was extended by using the chitosan MAP, which induced an immune-enhancing effect.Citation26 The extended release of vaccines for daily vaccination provided an improved vaccination effect.Citation27
To demonstrate the successful protection of immunity by MAP vaccination, a number of studies of pathogen challenges were carried out, and the results were promising. In particular, studies of influenza vaccination that compared MAP injection and a no-treatment group found that the MAP application conferred greater protective immunity.Citation28,Citation29 Similar observations were documented when comparing MAP injection with subcutaneous injectionCitation30,Citation31 and intranasal administration.Citation32 Notably, the MAP application induced not only comparable protective efficacyCitation33-37 but also better protection eventCitation38-41 compared to intramuscular (IM) administration. Of equal importance, such protective efficacy continued for several months after vaccination (6 months,Citation42 14 monthsCitation43). Another study demonstrated cross-protection when mice vaccinated with A/PR8 influenza hemagglutinin DNA did not contract pandemic 2009 H1N1.Citation44 Besides the influenza vaccine, some vaccines targeting virus outbreak pandemic pathogens, such as the Ebola virus,Citation45 the Chikungunya virus,Citation46 HIV,Citation47,Citation48 and the herpes simplex virus,Citation49,Citation50 were successfully delivered into the skin by MAP and conferred suitable protection after viral challenge. The Zika virus was especially challenged in the neonatal mouse model.Citation51 The enterovirus MAP vaccination induced full protection against lethal challenge with only 10% of the delivered antigen dose compared to IM injection.Citation52 Most of the challenge tests were studied in mice, but one study of a measles vaccine was applied in infant rhesus macaques. The results showed complete protection in the MAP administration group compared to the group that received the vaccine by a subcutaneous route.Citation53
Vaccine development for bacteria-derived pathogens plays a critical role in decreasing the burden on the health-care system throughout the world. Several such severe antigens were researched to be delivered by MAP, and mice were challenged after vaccination to prove the strong induction of protective immunity against Pseudomonas aeruginosa,Citation122 Clostridium difficile,Citation107 Streptococcus suis,Citation118 and Francisella novicida.Citation88 The anthrax vaccine was tested, and the results showed 100% protection from aerosol spore challenge in rabbits.Citation133 A tetanus vaccine was studied in another neonatal mouse group, and the group administered with MAP vaccination was totally protected, while none of the mice administered via the IM route survived.Citation132 Finally, live adenovirus-vectored malaria vaccine was researched and an equivalent protective efficacy was reported when comparing intradermal (ID) immunization by MAP with the administration via hypodermic needles.Citation61 A large number of preclinical research studies have been carried out with MAP for vaccine application; the target animals were wide ranging from mice to monkeys. More importantly, it was demonstrated that MAP-based vaccination generated strong desired immune response to specific antigens and conferred protective efficacy not only for a short time but also for a long time after vaccine administration. Different vaccines for infectious diseases were investigated, and the results indicate that it is feasible to use MAPs to combat diverse pathogens. In addition, it was found that the S-MAP gradually received less attention than the other MAP types because of the S-MAP’s complex application steps and the difficulty in measuring the right dose for delivery. Therefore, the most promising MAP types for ID vaccination are C-MAP or D-MAP applied as patches.
2.2. Stability studies of vaccine MAP
Keeping antigens stable during processing and storage has been one of the challenges for MAP vaccination. The possible loss of antigenicity can occur in the drying and storing stages. The thermostability of the MAP vaccine can be improved by the addition of a stabilizer; the appropriate stabilizer depends on the kind of vaccine being administered, as shown in .
Table 5. Stability studies of vaccine MAP
3. Clinical trials and human studies of vaccine map
3.1 Clinical trials of vaccine MAP reported at ClinicalTrial.gov
The innovation of MAP in stability, bioavailability, potency, and less adverse reactions is incorporated into the vaccine to overcome the limitations and current disadvantages of hypodermic needle injection. Using “microneedle patch” as the keyword, we found three trials registered at Clinical Trial.gov that have applied the vaccine MAP (). Three kinds of MAPs have been used in the registered trials, and these studies have been conducted to address some of the most dangerous infectious diseases to determine the feasibility of MAP vaccination in clinical practice.
Table 6. Clinical trials of vaccine MAP registered at ClinicalTrial.gov
3.2. Vaccine C-MAP for human studies
In several human studies, a vaccine C-MAP (NanopatchTM) has shown promise as a system for effective drug delivery. Both uncoated and excipient-coated NanopatchTM were administered to 18 healthy adults for 2 min of insertion and removal. On a pain scale from 0 to 10, 78% of the participants scored 0, and the average score was less than 1. No unexpected adverse events directly related to NanopatchTM were observed, and the expected erythema response faded between 3 and 7 d after vaccination.Citation138 When NanopatchTM with 15 µg of inactivated influenza virus (H1N1) was administered to healthy volunteers, adverse events were mild or moderate, and more than half (55%) of the volunteers preferred the NanopatchTM to IM administration.Citation139 In addition, the antibody response using a NanopatchTM was comparable to that with conventional IM administration.Citation139
3.3. Vaccine D-MAP for human studies
When a D-MAP patch was applied to the participants in one study, there was no pain swelling, and only mild erythema was localized to the site of patch administration. Moreover, the large majority of subjects were somewhat or fully confident with self-administration.Citation140 Consequently, influenza vaccine was encapsulated in the polymer matrix and D-MAPs were applied to volunteers for a phase 1 trial. The antibody response by self-administered D-MAP was comparable to that by IM administration.Citation141
Another D-MAP for treating influenza was prepared from a hyaluronic acid MAP named MicroHyala TM. No severe local or systemic adverse events were detected, and immunological efficacy was comparable to that of IM administration.Citation142
4. Concerns about vaccine MAP
4.1. Commercialized MAP
Several pharmaceutical companies have developed MAP devices for drug delivery systems, as shown in . OnvaxTM (BD company) consists of an array of plastic microprojections with a height of approximately 200 µm. Rubbing the skin surface with such devices led to disruption of the skin and delivery of the vaccine into the epidermal layer.Citation59,Citation143 A NanopatchTM has been produced by Vaxxas and is a solid high-density microprojection array coated with the influenza vaccine formulation. A NanopatchTM needle length is 250 µm and is administered with a spring-loaded applicator.Citation62 The ZP MAP system from Zosano Pharma consists of 1,300 microneedles in a 2 cm2 area. The drug is coated on a 190-µm-long MAP, and the ZP MAP is applied with a hand-held reusable applicator. This system administers the drug formulation into the outer skin layers and provides the desired outcome.Citation144 CosMED Pharmaceutical Ltd. has developed a D-MAP (MicroHyalaTM) made of hyaluronic acid. The D-MAP is approximately 800 µm in length and efficiently delivers various materials into the epidermis and dermis below the stratum corneum.Citation142,Citation145 3M has developed a C-MAP based on a Microstructured Transdermal System (MTS) of 500 µn; an applicator is used to deliver the drug agent into the skin. A one square-centimeter microneedle array is molded from durable medical grade polymer, and the array is attached to an adhesive patch.Citation70,Citation71 MicroCorTM was designed by the Corium company. MicroCorTM is a D-MAP with an applicator device integrated as a single piece into the MAP.Citation126,Citation147
4.2. Manufacturing issues
Concerns for vaccine MAP are dosage uniformity, reasonable cost, mass production, and production according to Good Manufacturing Practice (GMP). In regard to cost and scalability, MAPs require large-scale manufacturing machines and processes to be established.Citation148 Substantial investment is necessary for machining, casting, and forming of MAPs at the initial stages of mass production. However, after the successful establishment of these initial stages, the cost of manufacture can be predicted to be less than for injectables.Citation149 The polymer-based MAP casting technique might offer low cost because some polymers, such as cellulose derivatives, engineering plastics, and sugars, are typically inexpensive. However, because of reliance on master molds and inherently multi-step filling, handling processes can be a challenge to scale up. Furthermore, temperature may increase due to the drying process during the manufacture of vaccine MAP. Thus, a low-temperature process can be required to produce thermo-sensitive antigens. Special packaging or desiccants can be required to increase storage stability, but the addition of material to protect from moisture can increase the cost of packaging. Moreover, sterilization also is essential for vaccine MAP. Even though MAP has a low bioburden, the cost for the aseptic process should be considered. Also, validation of vaccine MAP products should be considered as a cost factor. Standardization of MAPs is also crucial for quality control during manufacturing and marketing. Manufacturers must develop an effective pharmaceutical quality assurance system, which must be a comprehensively designed and correctly implemented according to a Pharmaceutical Quality System incorporating Good Manufacturing Practice and Quality Risk Management.Citation150 Finally, the successful manufacture of vaccine MAP depends on current guidelines for conventional drugs as well as specific standards for each type of MAP.Citation151
Some previous studies have mentioned the vaccine MAP preparation environment. HBsAg D-MAP was fabricated in an aseptic Grade A isolator in a GMP pilot facility,Citation127 and influenza C-MAP developed from 3 M’s solid microstructured transdermal system was produced by a GMP-scalable process.Citation94 3 M’s proprietary GMP manufacturing and aseptic coating technology has a capacity of manufacturing up to 10,000 patches per day.Citation152 Lohmann Therapie-Systeme (LTS) AG and Corium Inc. have manufacturing licenses for MAP patches as drug delivery systems.Citation153 However, companies usually have not released detailed information about their manufacturing technology and environment.
A reasonable and affordable price of commercial MAP is important for successfully launching novel pharmaceutical dosage forms to the market. However, issues related to mass production, GMP, and costs during manufacturing are all challenges currently hindering the development of such innovative products for clinical use. Currently, seven microneedle manufacturers are developing vaccine applications (see ).
Table 7. Microneedle manufacturers developing vaccine microneedle array patch (MAP)
4.3 Regulatory issues
The most recent document from the U.S. Food and Drug Administration (FDA) describes a vaccine MAP as a product that is a combination of a biological product and a mechanical device. A product is composed of two or more regulatory products. The prefilled syringe, autoinjector, or MAP patch pre-loaded with a biological product are examples of this type of product (21 CFR 3.2e).Citation158 A vaccine MAP has been focused on because of the advantages of MAP. Vaccine and MAP were combined as a single entity. The regulation of vaccine MAP should consider the safety and effectiveness questions associated with each constituent part and the product as the whole (21 CFR Part 4 Subpart A: sec. 4.4 (b)). A vaccine MAP also should fulfill the requirements for current GMP and for postmarketing safety to transition from the laboratory to clinical use.
The National Regulatory Authorities (NRAs) of each country where the authorized vaccine MAP will be used to require the extension of current marketing authorization or a new one because vaccine MAP changes the route of drug administration. The studies comparing conventional and new vaccine products could reduce the regulatory steps.Citation159 In low-income countries, the novel vaccine must be licensed by the NRA, FDA, or European Medicines Agency, which are following the essential regulatory functions of the WHO Vaccines Pre-Qualification Program. Such organizations authorize the use of suitable vaccines for the target population and the program.Citation160 Because another advantage of vaccine MAP is self-administration, regulatory guidelines for the validity and proper use of self-vaccination will be necessary.Citation148
5. Conclusion
Preclinical studies of vaccine MAP have been conducted in a wide range of animal models, from rodents to primates. Various vaccines, including new outbreak pandemic vaccines, have been tested using the MAP system, and comparable or superior antibody response has been shown compared to IM and other routes of vaccination. Clinical studies have also been conducted to prove the stability, safety, and immunological efficacy of vaccine MAP, and positive and comparable results compared to IM administration have been demonstrated. However, concerns remain about mass production, reasonable cost, aseptic process, and reproducible quality. Vaccine microneedles are a vaccine product, and the standards for the vaccine product will be applied to the preparation of vaccine microneedles. Therefore, it will take time for the final clinical product of vaccine microneedles to come out. Furthermore, the need for a suitable applicator of vaccine MAP and additional packaging for vaccine MAP are additional cost factors. But several microneedle manufacturers with mass-production capabilities have already developed vaccine MAP in cooperation with vaccine companies, and improved immunological results have been reported. If the above-mentioned limitations are overcome, various vaccines will be incorporated into a microneedle system and administered by MAPs. In the near future, MAPs will be used as a vaccine delivery system together with syringes and needles.
Additional information
Funding
References
- Organization WH. Mortality and global health estimates. Geneva (Switzerland): World Health Organization. 2013 Retrieved May 4, 2020, from https://www.who.int/healthinfo/global_burden_disease/estimates/en/index1.html
- Shin CI, Jeong SD, Rejinold NS, Kim YC. Microneedles for vaccine delivery: challenges and future perspectives. Ther Deliv. 2017;8:447–60.
- Arya J, Prausnitz MR. Microneedle patches for vaccination in developing countries. J Control Release. 2016;240:135–41.
- Portnoy A, Ozawa S, Grewal S, Norman BA, Rajgopal J, Gorham KM, Haidari LA, Brown ST, Lee BY. Costs of vaccine programs across 94 low- and middle-income countries. Vaccine. 2015;33:A99–A108.
- Restrepo-Méndez MC, Barros AJ, Wong KL, Johnson HL, Pariyo G, França GV, Wehrmeister FC, Victora CG. Inequalities in full immunization coverage: trends in low- and middle-income countries. Bull World Health Organ. 2016;94:794–805B.
- Nguyen TT, Park JH. Human studies with microneedles for evaluation of their efficacy and safety. Expert Opin Drug Deliv. 2018;15:235–45.
- Mathers AR, Larregina AT. Professional antigen-presenting cells of the skin. Immunol Res. 2006;36:127–36.
- Kim YC, Park JH, Prausnitz MR. Microneedles for drug and vaccine delivery. Adv Drug Deliv Rev. 2012;64:1547–68.
- Mutyambizi K, Berger CL, Edelson RL. The balance between immunity and tolerance: the role of Langerhans cells. Cell Mol Life Sci. 2009;66:831–40.
- Mikszta JA, Alarcon JB, Brittingham JM, Sutter DE, Pettis RJ, Harvey NG. Improved genetic immunization via micromechanical disruption of skin-barrier function and targeted epidermal delivery. Nat Med. 2002;8:415–19.
- Lee JW, Park J-H, Prausnitz MR. Dissolving microneedles for transdermal drug delivery. Biomaterials. 2008;29:2113–24.
- Shim DH, Nguyen TT, Park P-G, Kim MJ, Park B-W, Jeong H-R, Kim D-S, Joo HW, Choi S-O, Park J-H, et al. Development of Botulinum Toxin A-Coated Microneedles for Treating Palmar Hyperhidrosis. Mol Pharm. 2019;16:4913–19.
- Lee JW, Choi SO, Felner EI, Prausnitz MR. Dissolving microneedle patch for transdermal delivery of human growth hormone. Small. 2011;7:531–39.
- Morefield GL, Tammariello RF, Purcell BK, Worsham PL, Chapman J, Smith LA, Alarcon JB, Mikszta JA, Ulrich RG. An alternative approach to combination vaccines: intradermal administration of isolated components for control of anthrax, botulism, plague and staphylococcal toxic shock. J Immune Based Ther Vaccines. 2008;6:5.
- Amorij JP, Kersten GF, Saluja V, Tonnis WF, Hinrichs WL, Slutter B, Bal SM, Bouwstra JA, Huckriede A, Jiskoot W. Towards tailored vaccine delivery: needs, challenges and perspectives. J Control Release. 2012;161:363–76.
- Wilson-Welder JH, Torres MP, Kipper MJ, Mallapragada SK, Wannemuehler MJ, Narasimhan B. Vaccine adjuvants: current challenges and future approaches. J Pharm Sci. 2009;98:1278–316.
- Vogt A, Combadiere B, Hadam S, Stieler KM, Lademann J, Schaefer H, Schaefer H, Autran B, Sterry W, Blume-Peytavi U. 40 nm, but not 750 or 1,500 nm, nanoparticles enter epidermal CD1a+ cells after transcutaneous application on human skin. J Invest Dermatol. 2006;126:1316–22.
- Bal SM, Ding Z, Kersten GFA, Jiskoot W, Bouwstra JA. Microneedle-based transcutaneous immunisation in mice with N-trimethyl chitosan adjuvanted diphtheria toxoid formulations. Pharm Res 2010;27:1837–47.
- Siddhapura K, Harde H, Jain S. Immunostimulatory effect of tetanus toxoid loaded chitosan nanoparticles following microneedles assisted immunization. Nanomedicine. 2016;12:213–22.
- Wang T, Zhen Y, Ma X, Wei B, Li S, Wang N. Mannosylated and lipid A-incorporating cationic liposomes constituting microneedle arrays as an effective oral mucosal HBV vaccine applicable in the controlled temperature chain. Colloids Surf B Biointerfaces. 2015;126:520–30.
- Ding Z, Bal SM, Romeijn S, Kersten GF, Jiskoot W, Bouwstra JA. Transcutaneous immunization studies in mice using diphtheria toxoid-loaded vesicle formulations and a microneedle array. Pharm Res 2011;28:145–58.
- Kumar A, Wonganan P, Sandoval MA, Li X, Zhu S, Cui Z. Microneedle-mediated transcutaneous immunization with plasmid DNA coated on cationic PLGA nanoparticles. J Control Release. 2012;163:230–39.
- Yang HW, Ye L, Guo XD, Yang C, Compans RW, Prausnitz MR. Ebola Vaccination Using a DNA Vaccine Coated on PLGA-PLL/gammaPGA Nanoparticles Administered Using a Microneedle Patch. Adv Healthc Mater. 2017;6:1600750.
- Yin D, Liang W, Xing S, Gao Z, Zhang W, Guo Z, Gao S. Hepatitis B DNA vaccine-polycation nano-complexes enhancing immune response by percutaneous administration with microneedle. Biol Pharm Bull. 2013;36:1283–91.
- Boopathy AV, Mandal A, Kulp DW, Menis S, Bennett NR, Watkins HC, Wang W, Martin JT, Thai NT, He Y, et al. Enhancing humoral immunity via sustained-release implantable microneedle patch vaccination. Proc Natl Acad Sci. 2019;116:16473–78.
- Chen Y-H, Lai K-Y, Chiu Y-H, Wu Y-W, Shiau A-L, Chen M-C. Implantable microneedles with an immune-boosting function for effective intradermal influenza vaccination. Acta Biomaterialia. 2019; 97:230–38.
- Joyce JC, Sella HE, Jost H, Mistilis MJ, Esser ES, Pradhan P, Toy R, Collins ML, Rota PA, Roy K, et al. Extended delivery of vaccines to the skin improves immune responses. J Control Release. 2019;304:135–45.
- Kim Y-C, Quan F-S, Compans RW, Kang S-M, Prausnitz MR. Formulation of microneedles coated with influenza virus-like particle vaccine. AAPS PharmSciTech. 2010;11:1193–201.
- Shin JH, Noh JY, Kim KH, Park JK, Lee JH, Jeong SD, Jung DY, Song CS, Kim YC. Effect of zymosan and poly (I:C) adjuvants on responses to microneedle immunization coated with whole inactivated influenza vaccine. J Control Release. 2017;265:83–92.
- Weldon WC, Martin MP, Zarnitsyn V, Wang B, Koutsonanos D, Skountzou I, Prausnitz MR, Compans RW. Microneedle vaccination with stabilized recombinant influenza virus hemagglutinin induces improved protective immunity. Clin Vaccine Immunol. 2011;18:647–54.
- Nakatsukasa A, Kuruma K, Okamatsu M, Hiono T, Suzuki M, Matsuno K, Kida H, Oyamada T, Sakoda Y. Potency of whole virus particle and split virion vaccines using dissolving microneedle against challenges of H1N1 and H5N1 influenza viruses in mice. Vaccine. 2017;35:2855–61.
- Shin JH, Park JK, Lee DH, Quan FS, Song CS, Kim YC. Microneedle Vaccination Elicits Superior Protection and Antibody Response over Intranasal Vaccination against Swine-Origin Influenza A (H1N1) in Mice. PLoS One. 2015;10:e0130684.
- Sullivan SP, Koutsonanos DG, Del Pilar Martin M, Lee JW, Zarnitsyn V, Choi SO, Murthy N, Compans RW, Skountzou I, Prausnitz MR. Dissolving polymer microneedle patches for influenza vaccination. Nat Med. 2010;16:915–20.
- Zhu Q, Zarnitsyn VG, Ye L, Wen Z, Gao Y, Pan L, Skountzou I, Gill HS, Prausnitz MR, Yang C, et al. Immunization by vaccine-coated microneedle arrays protects against lethal influenza virus challenge. Proc Natl Acad Sci U S A. 2009;106:7968–73.
- Koutsonanos DG, Martin MdP, Zarnitsyn VG, Sullivan SP, Compans RW, Prausnitz MR, Transdermal Influenza Immunization with Vaccine-Coated Microneedle Arrays. PLoS One. 2009;4:e4773.
- Kim YC, Quan FS, Compans RW, Kang SM, Prausnitz MR. Formulation and coating of microneedles with inactivated influenza virus to improve vaccine stability and immunogenicity. J Control Release. 2010;142:187–95.
- Kim M-C, Lee JW, Choi H-J, Lee Y-N, Hwang HS, Lee J, Kim C, Lee JS, Montemagno C, Prausnitz MR, et al. Microneedle patch delivery to the skin of virus-like particles containing heterologous M2e extracellular domains of influenza virus induces broad heterosubtypic cross-protection. J Control Release. 2015;210:208–16.
- Wang BZ, Gill HS, He C, Ou C, Wang L, Wang YC, Feng H, Zhang H, Prausnitz MR, Compans RW. Microneedle delivery of an M2e-TLR5 ligand fusion protein to skin confers broadly cross-protective influenza immunity. J Control Release. 2014;178:1–7.
- Kim YC, Song JM, Lipatov AS, Choi SO, Lee JW, Donis RO, Compans RW, Kang SM, Prausnitz MR. Increased immunogenicity of avian influenza DNA vaccine delivered to the skin using a microneedle patch. Eur J Pharm Biopharm. 2012;81:239–47.
- Song J-M, Kim Y-C OE, Compans RW, Prausnitz MR, Kang S-M. DNA vaccination in the skin using microneedles improves protection against influenza. Molecular Therapy: The Journal of the American Society of Gene Therapy. 2012;20:1472–80.
- Quan F-S, Kim Y-C, Vunnava A, Yoo D-G, Song J-M, Prausnitz MR, Compans RW, Kang S-M. Intradermal vaccination with influenza virus-like particles by using microneedles induces protection superior to that with intramuscular immunization. J Virol. 2010;84:7760–69.
- Koutsonanos DG, Del Pilar Martin M, Zarnitsyn VG, Jacob J, Prausnitz MR, Compans RW, Skountzou I. Serological memory and long-term protection to novel H1N1 influenza virus after skin vaccination. J Infect Dis. 2011;204:582–91.
- Quan FS, Kim YC, Song JM, Hwang HS, Compans RW, Prausnitz MR, Kang SM. Long-term protective immunity from an influenza virus-like particle vaccine administered with a microneedle patch. Clin Vaccine Immunol. 2013;20:1433–39.
- Kim Y-C, Yoo D-G, Compans RW, Kang S-M, Prausnitz MR. Cross-protection by co-immunization with influenza hemagglutinin DNA and inactivated virus vaccine using coated microneedles. J Control Release. 2013;172:579–88.
- Liu Y, Ye L, Lin F, Gomaa Y, Flyer D, Carrion R Jr., Patterson JL, Prausnitz MR, Smith G, Glenn G, et al. Intradermal Vaccination With Adjuvanted Ebola Virus Soluble Glycoprotein Subunit Vaccine by Microneedle Patches Protects Mice Against Lethal Ebola Virus Challenge. J Infect Dis. 2018;218:S545–s52.
- Prow TW, Chen X, Prow NA, Fernando GJ, Tan CS, Raphael AP, Chang D, Ruutu MP, Jenkins DW, Pyke A, et al. Nanopatch-targeted skin vaccination against West Nile Virus and Chikungunya virus in mice. Small. 2010;6:1776–84.
- Zaric M, Becker PD, Hervouet C, Kalcheva P, Ibarzo Yus B, Cocita C, O’Neill LA, Kwon S-Y, Klavinskis LS. Long-lived tissue resident HIV-1 specific memory CD8+ T cells are generated by skin immunization with live virus vectored microneedle arrays. J Control Release. 2017;268:166–75. doi:10.1016/j.jconrel.2017.10.026.
- Becker PD, Hervouet C, Mason GM, Kwon S-Y, Klavinskis LS. Skin vaccination with live virus vectored microneedle arrays induce long lived CD8+ T cell memory. Vaccine. 2015;33(37):4691–98. doi:10.1016/j.vaccine.2015.04.046.
- Chen X, Kask AS, Crichton ML, McNeilly C, Yukiko S, Dong L, Marshak JO, Jarrahian C, Fernando GJ, Chen D, et al. Improved DNA vaccination by skin-targeted delivery using dry-coated densely-packed microprojection arrays. J Control Release. 2010;148:327–33.
- Kask AS, Chen X, Marshak JO, Dong L, Saracino M, Chen D, Jarrahian C, Kendall MA, Koelle DM. DNA vaccine delivery by densely-packed and short microprojection arrays to skin protects against vaginal HSV-2 challenge. Vaccine. 2010;28:7483–91.
- Kim E, Erdos G, Huang S, Kenniston T, Falo LD Jr., Gambotto A. Preventative Vaccines for Zika Virus Outbreak: preliminary Evaluation. EBioMedicine. 2016;13:315–20.
- Zhu Z, Ye X, Ku Z, Liu Q, Shen C, Luo H, Luan H, Zhang C, Tian S, Lim C, et al. Transcutaneous immunization via rapidly dissolvable microneedles protects against hand-foot-and-mouth disease caused by enterovirus 71. J Control Release. 2016;243:291–302.
- Joyce JC, Carroll TD, Collins ML, Chen MH, Fritts L, Dutra JC, Rourke TL, Goodson JL, McChesney MB, Prausnitz MR, et al. A Microneedle Patch for Measles and Rubella Vaccination Is Immunogenic and Protective in Infant Rhesus Macaques. J Infect Dis. 2018;218:124–32.
- Ding Z, Verbaan FJ, Bivas-Benita M, Bungener L, Huckriede A, van den Berg DJ, Kersten G, Bouwstra JA. Microneedle arrays for the transcutaneous immunization of diphtheria and influenza in BALB/c mice. J Control Release 2009;136:71–78.
- Zhou Q, Wang F, Yang F, Wang Y, Zhang X, Sun S. Augmented humoral and cellular immune response of hepatitis B virus DNA vaccine by micro-needle vaccination using Flt3L as an adjuvant. Vaccine. 2010;28:1357–62.
- Hirschberg H, van Kuijk S, Loch J, Jiskoot W, Bouwstra J, Kersten G, Amorij JP. A combined approach of vesicle formulations and microneedle arrays for transcutaneous immunization against hepatitis B virus. Eur J Pharm Sci. 2012;46:1–7.
- Guo L, Qiu Y, Chen J, Zhang S, Xu B, Gao Y. Effective transcutaneous immunization against hepatitis B virus by a combined approach of hydrogel patch formulation and microneedle arrays. Biomed Microdevices. 2013;15:1077–85.
- Ding Z, Van Riet E, Romeijn S, Kersten GF, Jiskoot W, Bouwstra JA. Immune modulation by adjuvants combined with diphtheria toxoid administered topically in BALB/c mice after microneedle array pretreatment. Pharm Res 2009;26:1635–43.
- Mikszta JA, Sullivan VJ, Dean C, Waterston AM, Alarcon JB, Dekker JP 3rd, Brittingham JM, Huang J, Hwang CR, Ferriter M, et al. Protective immunization against inhalational anthrax: a comparison of minimally invasive delivery platforms. J Infect Dis. 2005;191:278–88.
- Carey JB, Vrdoljak A, O’Mahony C, Hill AV, Draper SJ, Moore AC. Microneedle-mediated immunization of an adenovirus-based malaria vaccine enhances antigen-specific antibody immunity and reduces anti-vector responses compared to the intradermal route. Sci Rep. 2014;4:6154.
- Pearson FE, O’Mahony C, Moore AC, Hill AV. Induction of CD8(+) T cell responses and protective efficacy following microneedle-mediated delivery of a live adenovirus-vectored malaria vaccine. Vaccine 2015;33:3248–55.
- Carey JB, Pearson FE, Vrdoljak A, McGrath MG, Crean AM, Walsh PT, Doody T, O’Mahony C, Hill AVS, Moore AC. Microneedle Array Design Determines the Induction of Protective Memory CD8+ T Cell Responses Induced by a Recombinant Live Malaria Vaccine in Mice. PLoS One 2011;6:e22442.
- Chen Y-C, Chen S-J, Cheng H-F, Yeh M-K. Development of Yersinia pestis F1 antigen-loaded liposome vaccine against plague using microneedles as a delivery system. J Drug Deliv Sci Technol. 2020;55:101443.
- Dean CH, Alarcon JB, Waterston AM, Draper K, Early R, Guirakhoo F, Monath TP, Mikszta JA. Cutaneous delivery of a live, attenuated chimeric flavivirus vaccine against Japanese encephalitis (ChimeriVax)-JE) in non-human primates. Hum Vaccin. 2005;1:106–11.
- Kim YC, Quan FS, Yoo DG, Compans RW, Kang SM, Prausnitz MR. Improved influenza vaccination in the skin using vaccine coated microneedles. Vaccine. 2009;27:6932–38.
- Kim YC, Quan FS, Yoo DG, Compans RW, Kang SM, Prausnitz MR. Enhanced memory responses to seasonal H1N1 influenza vaccination of the skin with the use of vaccine-coated microneedles. J Infect Dis. 2010;201:190–98.
- Kim YC, Quan FS, Song JM, Vunnava A, Yoo DG, Park KM, Compans RW, Kang SM, Prausnitz MR. Influenza immunization with trehalose-stabilized virus-like particle vaccine using microneedles. Procedia Vaccinol. 2010;2:15–19.
- Quan F-S, Kim Y-C, Compans RW, Prausnitz MR, Kang S-M. Dose sparing enabled by skin immunization with influenza virus-like particle vaccine using microneedles. J Control Release. 2010;147:326–32.
- Song JM, Kim YC, Barlow PG, Hossain MJ, Park KM, Donis RO, Prausnitz MR, Compans RW, Kang SM. Improved protection against avian influenza H5N1 virus by a single vaccination with virus-like particles in skin using microneedles. Antiviral Res. 2010;88:244–47.
- Song J-M, Kim Y-C, Lipatov AS, Pearton M, Davis CT, Yoo D-G, Park K-M, Chen L-M, Quan F-S, Birchall JC, et al. Microneedle delivery of H5N1 influenza virus-like particles to the skin induces long-lasting B- and T-cell responses in mice. Clin Vaccine Immunol. 2010;17:1381–89.
- Jeong H-R, Park S, Park J-H, Bae J-Y, Kim G-Y, Baek S-K, Park M-S, Park J-H. Preparation of H1N1 microneedles by a low-temperature process without a stabilizer. Eur J Pharm Biopharm. 2019;143:1–7.
- Del Pilar Martin M, Weldon WC, Zarnitsyn VG, Koutsonanos DG, Akbari H, Skountzou I, Jacob J, Prausnitz MR, Compans RW. Local response to microneedle-based influenza immunization in the skin. MBio. 2012;3:e00012–12.
- Fernando GJ, Chen X, Primiero CA, Yukiko SR, Fairmaid EJ, Corbett HJ, Frazer IH, Brown LE, Kendall MA. Nanopatch targeted delivery of both antigen and adjuvant to skin synergistically drives enhanced antibody responses. J Control Release. 2012;159:215–21.
- Fernando GJP, Chen X, Prow TW, Crichton ML, Fairmaid EJ, Roberts MS, Frazer IH, Brown LE, Kendall MAF. Potent Immunity to Low Doses of Influenza Vaccine by Probabilistic Guided Micro-Targeted Skin Delivery in a Mouse Model. PLoS One. 2010;5:e10266.
- Chen X, Corbett HJ, Yukiko SR, Raphael AP, Fairmaid EJ, Prow TW, Brown LE, Fernando GJP, Kendall MAF. Site-Selectively Coated, Densely-Packed Microprojection Array Patches for Targeted Delivery of Vaccines to Skin. Adv Funct Mater. 2010;21:464–73.
- Chen X, Fernando GJ, Crichton ML, Flaim C, Yukiko SR, Fairmaid EJ, Corbett HJ, Primiero CA, Ansaldo AB, Frazer IH, et al. Improving the reach of vaccines to low-resource regions, with a needle-free vaccine delivery device and long-term thermostabilization. J Control Release. 2011;152:349–55.
- Depelsenaire ACI, Meliga SC, McNeilly CL, Pearson FE, Coffey JW, Haigh OL, Flaim CJ, Frazer IH, Kendall MAF. Colocalization of cell death with antigen deposition in skin enhances vaccine immunogenicity. J Invest Dermatol 2014;134:2361–70.
- Koutsonanos DG, Vassilieva EV, Stavropoulou A, Zarnitsyn VG, Esser ES, Taherbhai MT, Prausnitz MR, Compans RW, Skountzou I. Delivery of subunit influenza vaccine to skin with microneedles improves immunogenicity and long-lived protection. Sci Rep. 2012;2:357.
- Weldon WC, Zarnitsyn VG, Esser ES, Taherbhai MT, Koutsonanos DG, Vassilieva EV, Skountzou I, Prausnitz MR, Compans RW. Effect of Adjuvants on Responses to Skin Immunization by Microneedles Coated with Influenza Subunit Vaccine. PLoS One. 2012;7:e41501.
- Fernando GJ, Zhang J, Ng HI, Haigh OL, Yukiko SR, Kendall MA. Influenza nucleoprotein DNA vaccination by a skin targeted, dry coated, densely packed microprojection array (Nanopatch) induces potent antibody and CD8(+) T cell responses. J Control Release. 2016;237:35–41.
- Gill HS, Soderholm J, Prausnitz MR, Sallberg M. Cutaneous vaccination using microneedles coated with hepatitis C DNA vaccine. Gene Ther. 2010;17:811–14.
- Nguyen TT, Choi J-A, Kim JS, Park H, Yang E, Lee WJ, Baek S-K, Song M, Park J-H. Skin immunization with third-generation hepatitis B surface antigen using microneedles. Vaccine. 2019;37(40):5954–61. doi:10.1016/j.vaccine.2019.08.036.
- Schipper P, van der Maaden K, Groeneveld V, Ruigrok M, Romeijn S, Uleman S, Oomens C, Kersten G, Jiskoot W, Bouwstra J. Diphtheria toxoid and N-trimethyl chitosan layer-by-layer coated pH-sensitive microneedles induce potent immune responses upon dermal vaccination in mice. J Control Release. 2017;262:28–36.
- Moon S, Wang Y, Edens C, Gentsch JR, Prausnitz MR, Jiang B. Dose sparing and enhanced immunogenicity of inactivated rotavirus vaccine administered by skin vaccination using a microneedle patch. Vaccine. 2013;31:3396–402.
- Jung D, Rejinold NS, Kwak JE, Park SH, Kim YC. Nano-patterning of a stainless steel microneedle surface to improve the dip-coating efficiency of a DNA vaccine and its immune response. Colloids Surf B Biointerfaces. 2017;159:54–61.
- Vrdoljak A, McGrath MG, Carey JB, Draper SJ, Hill AV, O’Mahony C, Crean AM, Moore AC. Coated microneedle arrays for transcutaneous delivery of live virus vaccines. J Control Release. 2012;159:34–42.
- Muller DA, Depelsenaire ACI, Shannon AE, Watterson D, Corrie SR, Owens NS, Agyei-Yeboah C, Cheung STM, Zhang J, Fernando GJP, et al. Efficient delivery of Dengue virus subunit vaccines to the skin by microprojection arrays. Vaccines (Basel). 2019;7(189):1–13.
- Chandler CE, Harberts EM, Laemmermann T, Zeng Q, Opene BN, Germain RN, Jewell CM, Scott AJ, Ernst RK. Intradermal Delivery of Bacteria by Using Microneedle Arrays. Infect Immun. 2018;86:e00406–18.
- Pearson FE, McNeilly CL, Crichton ML, Primiero CA, Yukiko SR, Fernando GJP, Chen X, Gilbert SC, Hill AVS, Kendall MAF. Dry-Coated Live Viral Vector Vaccines Delivered by Nanopatch Microprojections Retain Long-Term Thermostability and Induce Transgene-Specific T Cell Responses in Mice. PLoS One 2013;8:e67888.
- Moreno E, Schwartz J, Calvo A, Blanco L, Larrea E, Irache JM, Sanmartin C, Coulman SA, Soto M, Birchall JC, et al. Skin vaccination using microneedles coated with a plasmid DNA cocktail encoding nucleosomal histones of Leishmania spp. Int J Pharm. 2017;533:236–44.
- Edens C, Collins ML, Ayers J, Rota PA, Prausnitz MR. Measles vaccination using a microneedle patch. Vaccine. 2013;31:3403–09.
- Muller DA, Pearson FE, Fernando GJ, Agyei-Yeboah C, Owens NS, Corrie SR, Crichton ML, Wei JC, Weldon WC, Oberste MS, et al. Inactivated poliovirus type 2 vaccine delivered to rat skin via high density microprojection array elicits potent neutralising antibody responses. Sci Rep 2016;6:22094.
- van der Maaden K, Sekerdag E, Schipper P, Kersten G, Jiskoot W, Bouwstra J. Layer-by-Layer Assembly of Inactivated Poliovirus and N-Trimethyl Chitosan on pH-Sensitive Microneedles for Dermal Vaccination. Langmuir. 2015;31:8654–60.
- Kommareddy S, Baudner BC, Bonificio A, Gallorini S, Palladino G, Determan AS, Dohmeier DM, Kroells KD, Sternjohn JR, Singh M, et al. Influenza subunit vaccine coated microneedle patches elicit comparable immune responses to intramuscular injection in guinea pigs. Vaccine. 2013;31:3435–41.
- Ma Y, Tao W, Krebs SJ, Sutton WF, Haigwood NL, Gill HS. Vaccine delivery to the oral cavity using coated microneedles induces systemic and mucosal immunity. Pharm Res. 2014;31:2393–403.
- Andrianov AK, DeCollibus DP, Gillis HA, Kha HH, Marin A, Prausnitz MR, Babiuk LA, Townsend H, Mutwiri G. Poly[di(carboxylatophenoxy)phosphazene] is a potent adjuvant for intradermal immunization. Proc Natl Acad Sci U S A. 2009;106:18936–41.
- Hiraishi Y, Nandakumar S, Choi SO, Lee JW, Kim YC, Posey JE, Sable SB, Prausnitz MR. Bacillus Calmette-Guerin vaccination using a microneedle patch. Vaccine. 2011;29:2626–36.
- DeMuth PC, Li AV, Abbink P, Liu J, Li H, Stanley KA, Smith KM, Lavine CL, Seaman MS, Kramer JA, et al. Vaccine delivery with microneedle skin patches in nonhuman primates. Nat Biotechnol. 2013;31:1082–85.
- DeMuth PC, Min Y, Huang B, Kramer JA, Miller AD, Barouch DH, Hammond PT, Irvine DJ. Polymer multilayer tattooing for enhanced DNA vaccination. Nat Mater. 2013;12:367.
- Choi YH, Perez-Cuevas MB, Kodani M, Zhang X, Prausnitz MR, Kamili S, O’Connor SM. Feasibility of Hepatitis B Vaccination by Microneedle Patch: cellular and Humoral Immunity Studies in Rhesus Macaques. J Infect Dis. 2019;220:1926–34.
- Koutsonanos DG, Esser ES, McMaster SR, Kalluri P, Lee JW, Prausnitz MR, Skountzou I, Denning TL, Kohlmeier JE, Compans RW. Enhanced immune responses by skin vaccination with influenza subunit vaccine in young hosts. Vaccine. 2015;33:4675–82.
- Vrdoljak A, Allen EA, Ferrara F, Temperton NJ, Crean AM, Moore AC. Induction of broad immunity by thermostabilised vaccines incorporated in dissolvable microneedles using novel fabrication methods. J Control Release. 2016;225:192–204.
- Vassilieva EV, Kalluri H, McAllister D, Taherbhai MT, Esser ES, Pewin WP, Pulit-Penaloza JA, Prausnitz MR, Compans RW, Skountzou I. Improved immunogenicity of individual influenza vaccine components delivered with a novel dissolving microneedle patch stable at room temperature. Drug Deliv Transl Res. 2015;5:360–71.
- Raphael AP, Prow TW, Crichton ML, Chen X, Fernando GJ, Kendall MA. Targeted, needle-free vaccinations in skin using multilayered, densely-packed dissolving microprojection arrays. Small. 2010;6:1785–93.
- Kommareddy S, Baudner BC, Oh S, Kwon SY, Singh M, O’Hagan DT. Dissolvable microneedle patches for the delivery of cell-culture-derived influenza vaccine antigens. J Pharm Sci. 2012;101:1021–27.
- Wang J, Li B, Wu MX. Effective and lesion-free cutaneous influenza vaccination. Proc Natl Acad Sci U S A. 2015;112:5005–10.
- Stinson JA, Raja WK, Lee S, Kim HB, Diwan I, Tutunjian S, Panilaitis B, Omenetto FG, Tzipori S, Kaplan DL. Silk Fibroin Microneedles for Transdermal Vaccine Delivery. ACS Biomaterials Science & Engineering. 2017;3:360–69.
- Ono A, Azukizawa H, Ito S, Nakamura Y, Asada H, Quan YS, Kamiyama F, Katayama I, Hirobe S, Okada N. Development of novel double-decker microneedle patches for transcutaneous vaccine delivery. Int J Pharm. 2017;532:374–83.
- Schepens B, Vos PJ, Saelens X. van der Maaden K. Vaccination with influenza hemagglutinin-loaded ceramic nanoporous microneedle arrays induces protective immune responses. Eur J Pharm Biopharm. 2019;136:259–66.
- Qiu Y, Guo L, Zhang S, Xu B, Gao Y, Hu Y, Hou J, Bai B, Shen H, Mao P. DNA-based vaccination against hepatitis B virus using dissolving microneedle arrays adjuvanted by cationic liposomes and CpG ODN. Drug Deliv. 2016;23:2391–98.
- Perez Cuevas MB, Kodani M, Choi Y, Joyce J, O’Connor SM, Kamili S, Prausnitz MR. Hepatitis B vaccination using a dissolvable microneedle patch is immunogenic in mice and rhesus macaques. Bioengineering & Translational Medicine. 2018;3(3):186–96. doi:10.1002/btm2.10098.
- Bachy V, Hervouet C, Becker PD, Chorro L, Carlin LM, Herath S, Papagatsias T, Barbaroux J-B, Oh S-J, Benlahrech A. Langerin negative dendritic cells promote potent CD8+ T-cell priming by skin delivery of live adenovirus vaccine microneedle arrays. Proc Natl Acad Sci. 2013;110(8):3041. doi:10.1073/pnas.1214449110.
- Pattani A, McKay PF, Garland MJ, Curran RM, Migalska K, Cassidy CM, Malcolm RK, Shattock RJ, McCarthy HO, Donnelly RF. Microneedle mediated intradermal delivery of adjuvanted recombinant HIV-1 CN54gp140 effectively primes mucosal boost inoculations. J Control Release. 2012;162(3):529–37. doi:10.1016/j.jconrel.2012.07.039.
- Zaric M, Becker PD, Hervouet C, Kalcheva P, Doszpoly A, Blattman N, A. O’ Neill L, Yus BI, Cocita C, Kwon S-Y, et al. Skin immunisation activates an innate lymphoid cell-monocyte axis regulating CD8+ effector recruitment to mucosal tissues. Nat Commun. 2019;10(1):2214. doi:10.1038/s41467-019-09969-2.
- Turvey ME, Uppu DSSM, Mohamed Sharif AR, Bidet K, Alonso S, Ooi EE, Ooi EE, Hammond PT. Microneedle-based intradermal delivery of stabilized dengue virus. Bioengineering & Transla Med. 2019;4:e10127.
- Resch TK, Wang Y, Moon -S-S, Joyce J, Li S, Prausnitz M, Jiang B. Inactivated rotavirus vaccine by parenteral administration induces mucosal immunity in mice. Sci Rep. 2018;8:561.
- Donadei A, Kraan H, Ophorst O, Flynn O, O’Mahony C, Soema PC, Moore AC. Skin delivery of trivalent Sabin inactivated poliovirus vaccine using dissolvable microneedle patches induces neutralizing antibodies. J Control Release. 2019;311-312:96–103.
- Hsueh KJ, Chen MC, Cheng LT, Lee JW, Chung WB, Chu CY. Transcutaneous immunization of Streptococcus suis bacterin using dissolving microneedles. Comp Immunol Microbiol Infect Dis. 2017;50:78–87.
- Liu S, Zhang S, Duan Y, Niu Y, Gu H, Zhao Z, Zhang S, Yang Y, Wang X, Gao Y. Transcutaneous immunization of recombinant Staphylococcal enterotoxin B protein using a dissolving microneedle provides potent protection against lethal enterotoxin challenge. Vaccine. 2019;37:3810–19.
- Chen F, Yan Q, Yu Y, Wu MX. BCG vaccine powder-laden and dissolvable microneedle arrays for lesion-free vaccination. J Control Release. 2017;255:36–44.
- Gala RP, Zaman RU, D’Souza MJ, Zughaier SM. Novel Whole-Cell Inactivated Neisseria Gonorrhoeae Microparticles as Vaccine Formulation in Microneedle-Based Transdermal Immunization. Vaccines (Basel). 2018;6:1–17.
- Rodgers AM, MTC M, Vincente-Perez EM, Dubois AV, Ingram RJ, Larraneta E, Kissenpfennig A, Donnelly RF. Design and characterisation of a dissolving microneedle patch for intradermal vaccination with heat-inactivated bacteria: A proof of concept study. Int J Pharm. 2018;549:87–95.
- Lee C, Kim H, Kim S, Lahiji SF, Ha NY, Yang H, Kang G, Nguyen HYT, Kim Y, Choi MS, et al. Comparative Study of Two Droplet-Based Dissolving Microneedle Fabrication Methods for Skin Vaccination. Adv Healthc Mater. 2018;7:e1701381.
- Matsuo K, Hirobe S, Yokota Y, Ayabe Y, Seto M, Quan YS, Kamiyama F, Tougan T, Horii T, Mukai Y, et al. Transcutaneous immunization using a dissolving microneedle array protects against tetanus, diphtheria, malaria, and influenza. J Control Release. 2012;160:495–501.
- Arshad MS, Fatima S, Nazari K, Ali R, Farhan M, Muhammad SA, Abbas N, Hussain A, Kucuk I, Chang M-W, et al. Engineering and characterisation of BCG-loaded polymeric microneedles. J Drug Target. 2019;28:525–32.
- Bonificio A, Ghartey-Tagoe E, Gallorini S, Baudner B, Chen G, Singh P, O’Hagan DT, Kommareddy S. Fabrication of cell culture-derived influenza vaccine dissolvable microstructures and evaluation of immunogenicity in guinea pigs. Vaccine. 2015;33:2930–38.
- Poirier D, Renaud F, Dewar V, Strodiot L, Wauters F, Janimak J, Shimada T, Nomura T, Kabata K, Kuruma K, et al. Hepatitis B surface antigen incorporated in dissolvable microneedle array patch is antigenic and thermostable. Biomaterials. 2017;145:256–65.
- Christiansen D, Earnest-Silveira L, Grubor-Bauk B, Wijesundara DK, Boo I, Ramsland PA, Vincan E, Drummer HE, Gowans EJ, Torresi J. Pre-clinical evaluation of a quadrivalent HCV VLP vaccine in pigs following microneedle delivery. Sci Rep. 2019;9:9251.
- Arya JM, Dewitt K, Scott-Garrard M, Chiang YW, Prausnitz MR. Rabies vaccination in dogs using a dissolving microneedle patch. J Control Release. 2016;239:19–26.
- Edens C, Dybdahl-Sissoko NC, Weldon WC, Oberste MS, Prausnitz MR. Inactivated polio vaccination using a microneedle patch is immunogenic in the rhesus macaque. Vaccine. 2015;33:4683–90.
- Edens C, Collins ML, Goodson JL, Rota PA, Prausnitz MR. A microneedle patch containing measles vaccine is immunogenic in non-human primates. Vaccine. 2015;33:4712–18.
- Esser ES, Romanyuk A, Vassilieva EV, Jacob J, Prausnitz MR, Compans RW, Skountzou I. Tetanus vaccination with a dissolving microneedle patch confers protective immune responses in pregnancy. J Control Release. 2016;236:47–56.
- Mikszta JA, Dekker JP 3rd, Harvey NG, Dean CH, Brittingham JM, Huang J, Sullivan VJ, Dyas B, Roy CJ, Ulrich RG. Microneedle-based intradermal delivery of the anthrax recombinant protective antigen vaccine. Infect Immun. 2006;74:6806–10.
- Kim YC, Quan FS, Compans RW, Kang SM, Prausnitz MR. Stability kinetics of influenza vaccine coated onto microneedles during drying and storage. Pharm Res. 2011;28:135–44.
- Chu LY, Ye L, Dong K, Compans RW, Yang C, Prausnitz MR. Enhanced Stability of Inactivated Influenza Vaccine Encapsulated in Dissolving Microneedle Patches. Pharm Res. 2016;33:868–78.
- Mistilis MJ, Joyce JC, Esser ES, Skountzou I, Compans RW, Bommarius AS, Prausnitz MR Long-term stability of influenza vaccine in a dissolving microneedle patch. Drug Deliv Transl Res. 2017;7:195–205.
- Hiraishi Y, Nakagawa T, Quan YS, Kamiyama F, Hirobe S, Okada N, Nakagawa S. Performance and characteristics evaluation of a sodium hyaluronate-based microneedle patch for a transcutaneous drug delivery system. Int J Pharm. 2013;441:570–79.
- Griffin P, Elliott S, Krauer K, Davies C, Rachel Skinner S, Anderson CD, Forster A. Safety, acceptability and tolerability of uncoated and excipient-coated high density silicon micro-projection array patches in human subjects. Vaccine. 2017;35:6676–84.
- Fernando GJP, Hickling J, Jayashi Flores CM, Griffin P, Anderson CD, Skinner SR, Davies C, Witham K, Pryor M, Bodle J, et al. Safety, tolerability, acceptability and immunogenicity of an influenza vaccine delivered to human skin by a novel high-density microprojection array patch (Nanopatch™). Vaccine. 2018;36:3779–88.
- Arya J, Henry S, Kalluri H, McAllister DV, Pewin WP, Prausnitz MR. Tolerability, usability and acceptability of dissolving microneedle patch administration in human subjects. Biomaterials. 2017;128:1–7.
- Rouphael NG, Paine M, Mosley R, Henry S, McAllister DV, Kalluri H, Pewin W, Frew PM, Yu T, Thornburg NJ, et al. The safety, immunogenicity, and acceptability of inactivated influenza vaccine delivered by microneedle patch (TIV-MNP 2015): a randomised, partly blinded, placebo-controlled, phase 1 trial. The Lancet. 2017;390:649–58.
- Hirobe S, Azukizawa H, Hanafusa T, Matsuo K, Quan YS, Kamiyama F, Katayama I, Okada N, Nakagawa S Clinical study and stability assessment of a novel transcutaneous influenza vaccination using a dissolving microneedle patch. Biomaterials. 2015;57:50–58.
- Laurent PE, Bourhy H, Fantino M, Alchas P, Mikszta JA. Safety and efficacy of novel dermal and epidermal microneedle delivery systems for rabies vaccination in healthy adults. Vaccine. 2010;28:5850–56.
- Daddona PE, Matriano JA, Mandema J, Maa Y-F. Parathyroid hormone (1-34)-coated microneedle patch system: clinical pharmacokinetics and pharmacodynamics for treatment of osteoporosis. Pharm Res. 2011;28:159–65.
- Hirobe S, Azukizawa H, Matsuo K, Zhai Y, Quan YS, Kamiyama F, Suzuki H, Katayama I, Okada N, Nakagawa S. Development and clinical study of a self-dissolving microneedle patch for transcutaneous immunization device. Pharm Res. 2013;30:2664–74.
- Indermun S, Luttge R, Choonara YE, Kumar P. du Toit LC, Modi G, Pillay V. Current Advances in the Fabrication of Microneedles for Transdermal Delivery J Control Release. 2014;185:130–38.
- Mikolaj Milewski YK, Ding Z, Zhang J, Ghartey-Tagoe E, Manser K, Nissley B, Petrescu I, Xu L, Duffield B, Chen G, et al. Stabilization and transdermal delivery of an investigational peptide using Microcor® solid-state dissolving microstructure arrays. J Pharm Sci. 2020;109:1288–96.
- Norman JJ, Arya JM, McClain MA, Frew PM, Meltzer MI, Prausnitz MR. Microneedle patches: usability and acceptability for self-vaccination against influenza. Vaccine. 2014;32:1856–62.
- Prausnitz MR. Engineering Microneedle Patches for Vaccination and Drug Delivery to Skin. Annu Rev Chem Biomol Eng. 2017;8:177–200.
- Commission E. Chapter 1: pharmaceutical Quality System Legal. EudraLex Good Manuf Pract Guidel. 2013;4:1–8.
- Lutton RE, Moore J, Larraneta E, Ligett S, Woolfson AD, Donnelly RF. Microneedle characterisation: the need for universal acceptance criteria and GMP specifications when moving towards commercialisation. Drug Deliv Transl Res. 2015;5:313–31.
- Zhang Y, Brown K, Siebenaler K, Determan A, Dohmeier D, Hansen K. Development of lidocaine-coated microneedle product for rapid, safe, and prolonged local analgesic action. Pharm Res. 2012;29:170–77.
- Richter-Johnson J, Kumar P, Choonara YE. du Toit LC, Pillay V. Therapeutic Applications and Pharmacoeconomics of Microneedle Technology Expert Rev Pharmacoecon Outcomes Res. 2018;18:359–69.
- Forster AH, Witham K, Depelsenaire AC, Veitch M, Wells JW, Wheatley A, Pryor M, Lickliter JD, Francis B, Rockman S. Safety, tolerability, and immunogenicity of influenza vaccination with a high-density microarray patch: results from a randomized, controlled phase I clinical trial. PLoS Med. 2020;17:e1003024.
- Kennedy RB, Tosh PK, Goergen KM, Grill DE, Oberg AL, Poland GA. Statistical modeling using early markers of innate immunity to explain variation in humoral responses to influenza vaccine in older adults. Vaccine 2015;33:3682–88.
- Kim NW, Kim S-Y, Lee JE, Yin Y, Lee JH, Lim SY, Kim ES, Duong HTT, Kim HK, Kim S. Enhanced cancer vaccination by in situ nanomicelle-generating dissolving microneedles. ACS Nano. 2018;12:9702–13.
- Lee C, Kim H, Kim S, Lahiji SF, Ha NY, Yang H, Kang G, Nguyen HYT, Kim Y, Choi MS. Comparative Study of Two Droplet‐Based Dissolving Microneedle Fabrication Methods for Skin Vaccination. Adv Healthc Mater. 2018;7:1701381.
- US Food and Drug Administration. Combination products therapeutic and diagnostic products that combine drugs, devices, and/or biological products. [Accessed 2018 Dec 18]. https://www.fda.gov/CombinationProducts
- Peyraud N, Zehrung D, Jarrahian C, Frivold C, Orubu T, Giersing B. Potential use of microarray patches for vaccine delivery in low- and middle- income countries. Vaccine. 2019;37:4427–34.
- Dellepiane N,DW. Twenty-five years of eht WHO vaccines prequalification programme (1987-2012): lessons learned and future perspectives. Vaccine. 2015;33:52–61.