ABSTRACT
Recurrent urinary tract infections (rUTI) are a serious disease associated with morbidities and mortality. Resistance to the standard of care antibiotics is now widespread because of the continued use of antibiotics among people who suffer from rUTI. We are therefore developing a vaccine to prevent recurrences among patients with rUTI. The antigen of the vaccine is FimH, a bacterial adhesin protein, and the vaccine is adjuvanted with a TLR-4 agonist. In a Phase 1 clinical study evaluating the vaccine, immunized individuals produced FimH-binding antibodies. Here we describe the optimization, qualification, and use of an assay to assess the functionality of these anti-FimH antibodies. The suitability of the assay for its intended purpose was demonstrated by selectivity, specificity, sensitivity, and intra-assay and inter-assay precision. The acceptance criteria were achieved for all parameters including intra-assay precision with ≤10% relative standard deviations and inter-assay precision with ≤25% relative standard deviations. The results presented herein suggest this functional assay will be important for supporting the vaccine’s efficacy in future human studies. Furthermore and of great significance, these results prove that vaccine-induced functional antibodies can be elicited in rUTI patients against an essential virulence factor, FimH.
Introduction
According to the National Disease and Therapeutic Index (NDTI, IMS Health, Plymouth Meeting, PA) about 3 million patients experience recurrent urinary tract infections (rUTI) each year in the US.Citation1,Citation2 Historically, antibiotic treatment has successfully treated individual recurrences among rUTI sufferers. But while antibiotics can treat these recurrences, they do not prevent the intense pain and anxiety felt during a UTI. In many cases, acute antibiotic regimens fail, and physicians and patients turn to prophylactic antibiotic therapy, in which antibiotics are taken daily for months at a time.Citation2 Not surprisingly, continued antibiotic use has led to increased antibiotic resistance among rUTI patients.Citation3
New alternatives to reduce bacterial infections are desperately needed to reduce current and future morbidities and mortalities. In fact, the last new antibiotic class approved and indicated for the treatment of gram-negative bacterial infections was discovered in the early 1960s.Citation4–6 As a solution, experts recently called upon a global effort to develop new vaccines targeting antibiotic-resistant bacteria.Citation7,Citation8
We are developing a vaccine to prevent UTI recurrences among rUTI patients. The active immunogenic component of the vaccine is FimCH, a non-covalent complex of the bacterial adhesin protein FimH and its chaperone protein FimC. FimH is an adhesin protein on the tip of type 1 pili, bacterial appendages that promote attachment of bacteria to host cells. Attachment occurs via branched oligomannose-containing glycoproteins that are highly conserved on the surfaces of all mammalian bladders. Type 1 pili and FimH are critical in several stages of UTI pathogenesis and are highly conserved among uropathogenic Escherichia coli and Klebsiella spp. FimH is essential for E. coli to colonize bladders in mice and cynomolgus monkeys, and antibodies raised against FimH antigens block the attachment of uropathogenic E. coli to human bladder cells.Citation9–13 In addition, five independent laboratories have demonstrated that immunization against FimH prevents E. coli from persisting in the bladders of mice and monkeys.Citation11,Citation12,Citation14–16
Sequoia’s UTI vaccine was recently evaluated in a phase 1 clinical trial to assess its safety and immunogenicity. This vaccine is adjuvanted with Phosphorylated HexaAcyl Disaccharide (PHAD®), a TLR-4 agonist. The vaccine was well-tolerated. ELISA-based immunogenicity data from the trial demonstrated that the vaccine elicited an antibody response against the N-terminus of FimH, which contains the mannose binding pocket. Elevated antibody titers, however, are not always indicative of a protective effect. Therefore, qualifying a clinically relevant and reproducible functional assay early in the development of a vaccine is paramount to supporting its efficacy in future human studies and proposing a correlate of protection.
A functional assay that measures the ability of anti-FimH sera from mice, rabbits, and monkeys to block binding of E. coli to cultured bladder cells in vitro has previously been reported.Citation11–13 The anti-FimH sera in these reports were obtained from animals immunized with FimCH adjuvanted with Freund’s or MF59. In this published assay, bladder cells are adhered onto 96-well tissue culture plates. Fluorescently labeled E. coli are pre-incubated with test or control sera and then added to the wells; after washing, the level of remaining fluorescence is indicative of the level of binding of E. coli to the bladder cells. To determine the degree of binding specifically mediated by type 1 pili, mannose was used as a positive control; high concentrations of mannose compete for the FimH binding site and reduce binding to bladder cells. A positive control specific for type 1 pili is critical for this functional assay because depending on the strain and in vitro culture conditions, E. coli has dozens of adhesins that can potentially be expressed and affect reproducibility of the assay. These adhesins have been shown to bind to a variety of oligosaccharides on cultured cells and plastic surfaces.Citation11–13
Herein we first describe the optimization of this functional assay to improve its reproducibility in preparation for analyzing thousands of samples from future human studies. Vaccines are typically in clinical development for ten or more years, so reproducibility of a functional assay is necessary to be able to compare results from human studies years apart. We then qualify this assay to prove its suitability to assess the functionality of vaccine-induced antibodies. We then use this qualified assay to demonstrate that antibodies induced by the FimH vaccine in humans with histories of rUTI reduce the adhesion of E. coli to cultured bladder cells in vitro. This is the first report demonstrating that a vaccine adjuvanted with a TLR-4 agonist elicits functional antibodies from humans against clinically relevant concentrations of E. coli in vitro. These results support the future evaluation of novel vaccines adjuvanted with TLR-4 agonists in humans to target gram-negative bacterial infections.
Materials and methods
Buffers and reagents
Labeling buffer contains 150 mM sodium chloride, 100 mM sodium carbonate. Pre-wash buffer contains 4% (w/v) fetal bovine serum albumin (MilliporeSigma), 1% (v/v) glycerol in phosphate buffered saline (PBS). Post-wash buffer contains 5% (v/v) fetal bovine serum in PBS. Pooled normal human serum (NHS; MilliporeSigma; 3016626) is diluted to 4% (v/v) in post-wash buffer.
Bacterial growth and labeling
E. coli J96, a uropathogenic strain from a human UTI was obtained from ATCC and stored as glycerol stocks in liquid nitrogen. Bacteria are grown and fluorescently labeled with fluorescein isothiocyanate (FITC; Sigma F7250) for each assay. All centrifugation steps are performed at 3000 × g for 5 min at 4°C. Bacterial CFU are initially estimated using a NanoDrop One (Thermo Fisher) spectrophotometer and then confirmed by counting colony-forming units (CFU) via agar plates. Bacteria are streaked onto plates at several points during the fluorescent labeling procedure to check for contamination. Expression of type 1 pili is verified by demonstrating the mannose-sensitive hemagglutination of guinea pig erythrocytes (Colorado Serum Company, 30100).
Bacteria are grown statically by inoculating 10 mL of Luria-Bertani (LB) broth in a 50 mL flask with cells scraped from a glycerol stock with an inoculating loop. The flask is capped loosely and grown overnight at 37°C without shaking. Then, 10 µL of the culture is used to inoculate 10 mL of fresh media in a new flask; this culture is grown overnight under the same conditions and harvested by centrifugation. The pelleted bacteria are resuspended in 4 mL of labeling buffer, centrifuged, and then resuspended in 4 mL of labeling buffer. FITC (40 µL of 1% w/v in DMSO) is then added. The mixture is incubated for 1 h at room temperature in the dark, and then centrifuged. The bacterial pellet is then washed three times with PBS (Sigma P3813), removing supernatant, resuspending and centrifuging the bacteria each time. At the final centrifugation, an aliquot is removed for counting CFU via agar plates. NanoDrop is used to determine the bacterial concentration to dilute FITC-labeled bacteria in PBS to 2.5 × 108 CFU/mL the day of dilution. This bacterial stock is used to prepare the assay plates. The concentration of the bacterial stock estimated by Nanodrop is adjusted accordingly by the results of counting CFU via agar plates.
Cell culture
T24 bladder epithelial cells were obtained from ATCC and are maintained in McCoys 5A (modified) media (Gibco, 16600). For the assay, cells are washed with fresh media containing bovine serum albumin (Sigma F4135), resuspended at 4 × 105 cells/mL, and added to the wells of a 96-well flat bottom white walled tissue culture plate (100 µL/well). Cells are then allowed to adhere to the plate overnight at 37°C, 5% CO2.
Antisera and antibodies
Characterized rabbit anti-FimH IgG reference standard
A FimCH vaccine was used to generate rabbit antiserum at Cocalico Biologicals, Inc. as previously described with the following modifications.Citation12 Three New Zealand white rabbits were immunized using 100 µg of FimCH that was mixed with 40 µg of phosphorylated hexaacyl disaccharide (PHAD®; Avanti Polar Lipids) in a dipalmitoyl-sn-glycero-3-phosphochiline suspension prior to each vaccination. The adjuvant suspension was prepared as detailed in Example 1 of US Patent 9,017,698.Citation22 The FimCH/PHAD vaccine (0.25 mL) was administered intramuscularly in two alternating sites on the hind flank on days 0, 21, 42 and 63, and exsanguination occurred on day 72. Unpurified serum from a test bleed of one rabbit at day 31 was used in development experiments. Purified IgG standard was prepared using antiserum from the exsanguination sera of the other two rabbits. IgG was purified using protein A chromatography, and characterized using SDS-PAGE, western blot analysis, ELISA and protein concentration according to the protocol and report in Supplementary File 1. This research complied with all applicable regulations governing the care and use of laboratory animals. This study was approved by the Cocalico Animal Care and Use Committee and Sequoia Sciences’ Institutional Animal Care and Use Committee.
Phase 1 human study and characterized human anti-FimH IgG reference standard
Sixty-seven adult females generally in good health were enrolled in seven cohorts in an open-label, 6-center, dose-escalating phase 1 study of the FimCH vaccine. The enrolled subjects were 18 to 64 years of age. Subjects had anti-FimH titers less than the limit of quantitation of a validated ELISA (Supplementary File 3). The first five cohorts consisted of 37 females who did not have a history of UTI. Females in cohort 1 received unadjuvanted FimCH (107 µg), and females in cohorts 2 to 5 received FimCH (50 µg) adjuvanted with either 10 µg, 20 µg, or 40 µg of PHAD®. Sixteen females were enrolled in cohort 6 and fourteen were enrolled in cohort 7, and all had a history of 5 or more documented UTI. Females in cohort 6 received 50 µg of FimCH with 40 µg of PHAD®, and females in cohort 7 received 107 µg of FimCH with 43 µg of PHAD®. Vaccinations (0.5 mL) were administered intramuscularly in the deltoid muscle of the non-dominant arm at days 1, 31, 90, and 180. Sera were collected immediately prior to the first vaccination, and at days 31, 60, 90, 120, 180, 210, and 360. Five females in cohorts 6 and 7 left the study during the 18-month study for protocol violations as reviewed by the medical monitor. The study protocol and all amendments were reviewed and approved by Schulman Institutional Review Board and each investigator, and were allowed by the Center for Biologics Evaluation and Research, Food and Drug Administration. All subjects provided written informed consent before enrollment into the study. See the protocol in Supplementary File 2 for all inclusions, exclusions, and responsibilities of the safety review committee.
Sera from four of the subjects were selected for preparation of a human anti-FimH IgG reference standard. These subjects were enrolled in cohorts 3 and 4 and had anti-FimH titers more than 10-fold over baseline as demonstrated by a validated ELISA one month after the second or third vaccination. IgG from each antiserum was purified at American International Biotechnology Services using protein A chromatography, and then characterized using SDS-PAGE, amino acid analysis (AAA), and ELISA. Equal amounts of IgG from each of the four subjects, based on AAA, were pooled to generate a human anti-FimH IgG standard. Pooling sera from four subjects ensured that a sufficient yield of standard would be produced for use in the phase 1 study and future studies. This reference standard is 97.1% pure by SDS-PAGE, and has a protein concentration of 2.71 mg/mL by AAA. Total IgG according to reactive IgG ELISA was 4.62 mg/mL. It is a clear solution in phosphate buffered saline (pH 7.5) stored at −70°C (Supplementary File 4).
Qualified assay protocol
This procedure describes the optimized and qualified assay procedure. Parameters for method development experiments differed as described in the text and figure legends. Test and control sera or antibodies are heat inactivated for 1 h at 56°C and then diluted to 2× the final assay concentration in 4% NHS. Mannose is dissolved at 5% (w/v) in 4% NHS. FITC-labeled E. coli are diluted with PBS to 2× the final titer. A pre-incubation plate is set up with the same layout as the assay plate and loaded with 60 µL of the appropriate dilution of E. coli plus 60 µL of the appropriate dilution of serum, antibody, or mannose. This plate is incubated for 1 h at 37°C. Meanwhile, the assay plate containing 4 × 104 T24 cells/well is washed 3× with 100 µL pre-wash buffer/well containing bovine serum albumin. The pre-incubation mixture is then transferred to the assay plate (50 µL/well), centrifuged for 1 min at 500 × g, and incubated for 1 h at room temperature in the dark. The plate is then washed 4× with post-wash buffer containing fetal bovine serum (100 µL/well) by gentle pipetting. The final wash is not removed from the wells, and the plate is read on a fluorescent plate reader (BioTek Synergy 2, BioTek Instruments) using an excitation wavelength of 485 nm and a detection wavelength of 528 nm.
Results
Initial optimization experiments of the functional assay
Initial optimization experiments focused on increasing the fluorescence signal of the bound E. coli. We tested whether a centrifugation step immediately following addition of bacteria to the cell culture plate would facilitate the reproducible adhesion of bacteria to the T24 bladder cells. A 1–3 min centrifugation step increased the signal by approximately 5- to 10-fold. This increase was not observed in wells without T24 cells, indicating that the centrifugation step did not increase nonspecific adherence of the bacteria to the plate ().
Figure 1. A. Effect of centrifugation on fluorescence. Assays were performed as described in the Methods section, except that T24 cell numbers were varied as indicated, and appropriately diluted E. coli was added directly to the adhered T24 cells, without a pre-incubation with test or control serum or antibodies. For each T24 cell number, two plates were prepared: one was centrifuged as described in the Methods, and one was not. Plotted here is the ratio of fluorescence from the centrifuged plate to fluorescence from the non-centrifuged plate. N = 2 wells per condition, and error bars represent standard deviation. B. Fluorescence signal as a function of CFU/well. Assays were performed as described in the Methods section, except that appropriately diluted E. coli was added directly to the adhered T24 cells, without a pre-incubation with test or control serum or antibodies. N = 6 or 12 wells per condition, and error bars represent standard deviations. C. Inhibition of fluorescence by mannose with varied CFU E. coli/well. Assays were performed as described in the Methods section, with a range of mannose concentrations. N = 2 wells per condition, and error bars represent standard deviation. D. Inhibition of fluorescence by mannose or rabbit anti-FimH. Assays were performed as described in the Methods section, with varied incubation time of E. coli with inhibitor. N = 6 (30 min and 120 min incubations) or 12 wells (controls, 60 min inubations) per condition, and error bars represent standard deviation. Two replicate plates were run on two separate days; data from one representative plate are shown
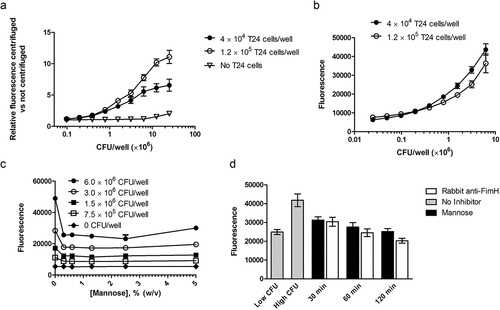
We then focused on understanding the fluorescence signal as a function of CFU/well binding to either 4 × 104 or 1.2 × 105 T24 cells/well. The published assay used in preclinical studies used 4 × 104 bladder cells and 2.5 × 107 CFU of E. coli per well. We tested binding of serial dilutions of uropathogenic E. coli strain J96 (starting at 6.25 × 106 CFU/well) and found that fluorescence increased with increasing CFU/well as expected with a wide dynamic range (). Using 1.2 × 105 T24 cells did not increase the signal compared to using 4 × 104 cells, and so we continued to use 4 × 104 cells per well in subsequent assays.
To determine the working range of the assay specific to type 1 pili mediated binding, different concentrations of the positive control mannose were added to wells that contained 4 × 104 T24 cells and serial dilutions of E. coli. The lowest CFU/well that resulted in detectable reduction of signal in the presence of mannose was approximately 7.5 × 105 CFU/well to 1.5 × 106 CFU/well (). Based on these data, the lower range of the assay was set to 3.0 × 106 CFU/well. Using 6 × 106 CFU/well, replicate wells exhibited < 20% relative standard deviation (RSD), and inhibition by mannose was clear, providing evidence that this level of CFU/well was suitable as the upper range of the assay. This corresponds to a bacterial cell to bladder cell ratio of 150:1, which is consistent with published methods.Citation11,Citation13 Since mannose concentrations >2.5% did not further inhibit E. coli binding to T24 cells, 2.5% mannose (140 mM) was selected as the standard concentration for positive controls; this is also consistent with publisher reports.
We also varied the lengths of the two incubation times: pre-incubation of bacteria with serum or positive control, and incubation of bacteria with T24. Pre-incubation of bacteria with positive control or a rabbit anti-FimH serum for 0.5, 1, or 2 h gave similar results (); 1 h pre-incubations were adopted for subsequent assays. The majority of method development experiments used a 1 h incubation of bacteria with T24 cells; experiments with 5 or 20 min incubation did not reduce background signal (data not shown).
Analysis of rabbit and human sera and optimization of the positive and negative controls
Following the initial assay optimization, we evaluated anti-FimH serum from a rabbit immunized with FimCH adjuvanted with PHAD® on two replicate plates each day on two separate days (). Three dilutions of rabbit serum taken either before or after immunization were incubated with E. coli before binding to T24 cells. Post-immune serum at 1:10, 1:20, and 1:40 dilutions reduced binding of E. coli to a similar extent as the 2.5% mannose positive control. Incubation with pre-immune serum resulted in fluorescence signals comparable to the high CFU/well of E. coli, demonstrating the selectivity of the assay.
Figure 2. A. Inhibition of binding by rabbit serum. Serum was collected from a single rabbit before and 4 weeks after immunization with the FimCH vaccine. The assay was performed as described in the Methods section. Low CFU wells contained 3 × 106 CFU/well; all others contained 6 × 106 CFU/well. Two replicate plates were run on two separate days; data from one representative plate are shown. N = 6 wells per condition, and error bars represent standard deviation. The RSDs of the average fluorescence signals on each plate were ≤12%. B. Inhibition of binding by purified human anti-FimH IgG. Low CFU wells contained 3 × 106 CFU/well; all others contained 6 × 106 CFU/well. Two replicate plates were run on two separate days; data from one representative plate are shown. N = 6 wells per condition, and error bars represent standard deviation. The RSDs of the average fluorescence signals were <12%. C. Effect of heat inactivation of serum on fluorescence. Assays were performed as described in the Methods section, except that heat inactivation time varied. Low CFU wells contained 3 × 106 CFU/well, and mid CFU wells contained 4.5 × 106 CFU/well; all others contained 6 × 106 CFU/well. Serum taken from one subject before and after vaccination were assayed after 0, 1, or 2 h heat inactivation. N = 6 wells per condition, and error bars represent standard deviation
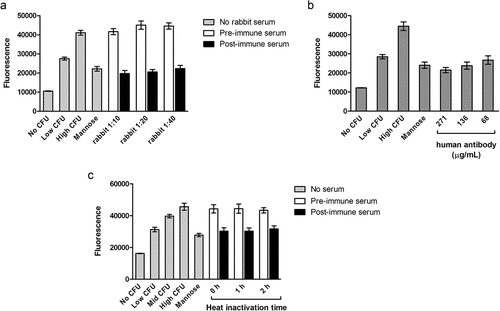
We then examined binding inhibition by a human anti-FimH IgG reference standard diluted in normal human serum (). Each dilution of the human anti-FimH IgG reference standard reduced fluorescence to levels observed in the 2.5% mannose wells and the low CFU wells, indicating a decrease in binding of >3 x 106 CFU/well.
To address the possibility that complement-dependent cytotoxicity could interfere with the binding assay, addition of a heat inactivation step of human serum was tested. Incubation with pre-immune serum inactivated for 0, 1, or 2 h (N = 18) wells did not decrease fluorescence signals relative to the high CFU/well (). Similarly, heat treatment of the post-immune serum did not affect its binding inhibition. Although it appeared to have no effect, the heat treatment step was retained in the method to ensure complement activation would not affect the reproducibility of the assay in the future.
The 2.5% mannose initially chosen as a positive control typically decreased the assay signal by about 3 × 106 CFU/well; we therefore considered defining the signal from 2.5% mannose wells as 100% inhibition for the assay. However, the average signal from mannose wells compared to the average signal of the control wells containing 3 × 106 CFU/well varied by >20% week-to-week during 12 weeks of experiments. Given the heterogeneous expression of E. coli adhesins under phase variation, this variability is not surprising. Based on this observation and to avoid skewing the data by defining 100% inhibition with a moving target, on each plate we included 6 negative control wells that contained T24 cells plus 6 × 106 CFU/well (high CFU/well; same as in test wells), 6 negative control wells that contained T24 cells plus 4.5 × 106 CFU/well (intermediate CFU/well), 6 negative control wells that contained T24 cells plus 3 × 106 CFU/well (low CFU/well) of E. coli, and 6 positive control wells that contained T24 cells plus 6 × 106 CFU/well of E. coli plus mannose. (See for the plate map) Including these 24 control wells on each plate negated the variability of day-to-day E. coli adhesins under phase variation. The 4.5 × 106 CFU/well (intermediate) established a 50% inhibition control for each plate to control against the variable expression of adhesins. We therefore defined each dilution of a sample as passing, or positive for inhibition, if it produced an assay signal less than that of the intermediate CFU/well, which correlates to a reduction of about 1.5 × 106 CFU/well (3 × 107 CFU/mL).
Prior to qualification, human sera from three randomly selected subjects with a history of urinary tract infections from the phase 1 trial were tested according to the developed procedure (). Sera collected pre-vaccination at day 1 and after the fourth vaccination at day 210 were analyzed on one plate per subject on two separate days. Each plate contained the 24 control wells described above. Each plate included six replicate wells of each dilution of each sample. At lease one dilution from each subject demonstrated less fluorescence than the intermediate CFU/well controls. All wells with pre-vaccination sera demonstrated equivalent fluorescence to the wells of high CFU negative control wells. All dilutions (1:20, 1:400, and 1:100) of day 210 sera from subjects 101–6004-1, 102–6005-1, and 103–6001-1 demonstrated less fluorescence than the intermediate CFU control wells the same plate, indicating a decrease in adherence of >1.5 x 106 CFU/well (3 x 107 CFU/mL). Based on the data from these samples, we adopted the plate maps in for analysis of additional subject sera. Subject sera were first analyzed using Plate Map 1 to screen for positive samples. Sera that tested positive at a 1:40 dilution were then further diluted and analyzed according to Plate Map 2.
Figure 3. Inhibition of E. coli binding by anti-FimH sera from immunized humans with a history of urinary tract infections. Pre-immune sera were collected prior to the first vaccination, and post-immune sera were collected 30 days after the fourth vaccination. Serum from subjects 101–6004, 102–6005, and 103–6001 were each tested on two separate days as noted in the graph titles. N = 6 wells per condition, and error bars represent standard deviation
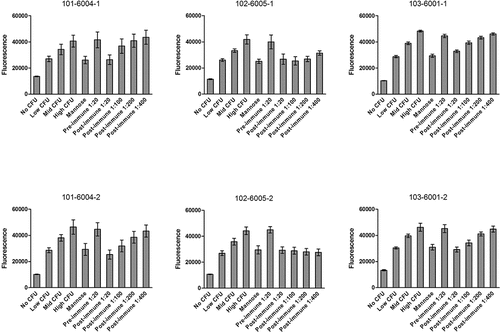
Qualification
Based on method development work, the following acceptance criteria were proposed for qualification:
All assay control replicates within a plate (low, intermediate, high CFU/well of E. coli, positive mannose control, and T24 only) must have an RSD ≤ 20%.
Mean signal of low CFU/well of E. coli < mean of intermediate CFU/well of E. coli < mean of high CFU/well of E. coli
A serum sample is deemed passing (positive for inhibition) if it demonstrates a mean signal, at 1:10 or greater dilution, that is less than the mean signal of the intermediate CFU/well of E. coli.
The lowest post-immune dilution meeting the criteria for a passing result must have a mean signal with an RSD ≤ 20%.
Assay qualification was performed to assess selectivity, specificity, sensitivity, and inter-assay and intra-assay precision.Citation23
During the qualification, non-immunized sera were analyzed in 6 wells of each plate for the selectivity, specificity, sensitivity, and inter-assay and intra-assay precision experiments. In a total of 12 plates over 6 days of these qualification experiments, NHS demonstrated equivalent fluorescence signals to the high CFU/well negative controls, demonstrating selectivity of the assay ( to 4). Selectivity was also evaluated by analyzing rabbit anti-FimH IgG reference standard spiked at 100 µg/mL (final in-well concentration) into a range of dilutions of the NHS. Fluorescence signals across all spiked dilutions ranged from 28810 (NHS 1:80) to 32086 (NHS 1:20; ). The difference in signals from the lowest dilution (32036 at 1:10) to the highest dilution (28949 at 1:640) was within 2 standard deviations of the means of all signals.
Table 1. Fluorescence as a function of concentration of rabbit anti-FimH IgG RS (reference standard) spiked into a pool of normal human sera. The full range of spiking concentrations were measured across two assay plates
Table 2. Selectivity and Specificity; rabbit anti-FimH IgG spiked at 100 µg/mL into a range of dilutions of NHS
The specificity and relative assay sensitivity were determined by running an 8-point concentration curve of rabbit anti-FimH IgG reference standard spiked into 10% NHS (final well concentration), ranging from 300 µg/mL to 2.334 µg/mL anti-FimH IgG ( and ), according to the method. Each concentration of IgG reference standard was run in 6 wells. The RSDs of the signals of all 8 concentrations were <7%. The relative assay sensitivity was defined as the lowest concentration on the curve that produced a signal equivalent to the signal of the intermediate CFU/well control, which is the concentration of anti-FimH IgG that reduces adherence of >1.5 x 106 CFU/well (3 x 107 CFU/mL). The assay sensitivity was determined as 20.2 µg/mL of the anti-FimH IgG reference standard.
Figure 5. Fluorescence as a function of concentration of rabbit anti-FimH IgG RS (reference standard) spiked into a pool of normal human sera. Individual values are shown in Table 1
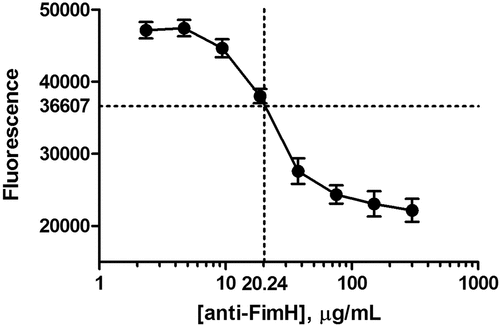
Intra-assay and inter-assay precision were evaluated by making multiple measurements of 4% (v/v) NHS spiked with rabbit anti-FimH IgG reference standard at a final concentration of 300 µg/mL (low positive control; LPC) or 700 µg/mL (high positive control; HPC). Negative control (NC) sera consisted of 4% (v/v) NHS with no spike. As described in the methods section, these controls were prepared as 10× stocks in undiluted NHS, and then diluted at the time of the assay to mimic handling of patient serum samples. Three separate plates were run on three different days (1 plate/day) by one analyst. Each plate included 6 wells of HPC, 6 wells of LPC, and 6 wells of NC in the established assay plate format. Intra-assay precision was assessed by measuring variation in fluorescence among the six replicate wells within a plate; the HPC, LPC, and NC had <10% RSDs for nearly all three runs (). Inter-assay precision was assessed by measuring the variation in average fluorescence across the three plates; HPC, LPC, and NC had RSDs of 24.8%, 18.2%, and 3.6%, respectively ().
Table 3. Intra-Assay Precision; average, SD, and RSD across replicate wells within a single plate
Table 4. Inter-Assay Precision; average, SD, and RSD of results from three different plates
Analyses of sera from subjects with a history of UTI who completed the 18-month phase 1 study
The qualified assay was then used to analyze sera from twenty-six human subjects with histories of UTI who completed the 18-month phase 1 study. Sera collected prior to the first vaccination (day 1) and 30 days after the fourth vaccination (day 210) were analyzed. (Day 1 serum was unavailable for one subject). Twenty-one subjects had their day 1 sera tested in the assay at a 1:10 dilution and 4 subjects had their day 1 sera tested at a 1:20 dilution. Day 1 sera from 22 of the subjects did not inhibit adherence at either 1:10 or 1:20 dilution; for three subjects, a 1:10 dilution of the day 1 sera did show some apparent inhibition of adherence, with fluorescence equivalent to that of the intermediate CFU/well control. An increase in functional antibodies after vaccination was observed in 24 of the subjects for which pre-and post-vaccination sera were available. The greatest dilution of day 210 sera that reduced adherence by >1.5 x 106 CFU/well (3 x 107 CFU/mL) are shown in .
Table 5. Dilutions of Sera from FimH-Immunized Humans that Inhibit the Adherence of E. coli to Human Bladder Cells
Discussion
Adherence of E. coli to bladder cells is the initiating event of UTI. This adherence occurs when the FimH adhesin on the surface of bacteria binds to branched oligomannose-expressing uroplakin on the surface of bladder epithelial cells. The in vitro functional assay described herein detects this binding mode to immortalized human bladder cells. Serum antibodies are evaluated against 6 × 106 CFU/well or 1 × 108 CFU/mL in this assay. Since humans experiencing UTI symptoms typically have 1 × 103 CFU/mL to ≥ 1 × 105 CFU/mL of bacteria in their urines, a reduction of binding of 3 × 107 CFU/mL of E. coli in this assay represents a clinically relevant result. This CFU/well is also about 2 to 3 orders of magnitude greater than that typically used in opsonization functional assays for many approved vaccines.
Most importantly, the qualified assay was employed to evaluate serum antibodies elicited from women immunized with a FimH vaccine adjuvanted with a TLR-4 agonist. During these analyses, the performance of the assay was consistent with the results of the qualification. When comparing sera collected before the study to sera collected 30 days after the fourth vaccination, all subjects showed an increase in functional antibodies. These results are significant for rUTI sufferers because these women have previously been exposed to FimH and type 1 pili during their rUTI. Despite these previous exposures, a FimH vaccine adjuvanted with a TLR-4 agonist was able to induce functional antibodies that reduced binding of E. coli to bladder cells in vitro.
Despite years of research evaluating vaccines in animals and humans, no vaccine indicated for reducing rUTI is approved for use. A vaccine against O-antigens of E. coli is currently under development for extra-intestinal pathogenic E. coli infections, potentially including UTI.Citation24–26 Two reportsCitation25,Citation27 demonstrate that this vaccine elicits functional antibody responses as measured by an opsonization killing assay, suggesting a different mechanism of action from the vaccine described here. No correlate of protection has been established for the O-antigen vaccine.
Vaccines typically display a correlation between antibody titer and protection from disease, but the exact correlation depends on many factors including characteristics of the patient, the vaccine, the disease, and the assays used.Citation28 Comparing correlates of protection among different vaccines is further complicated by the different units, not readily interconverted, for measuring the concentrations of antibody required (e.g. IU/mL, µg/mL, fractional titer of serum). Future phase 2 and phase 3 studies of our UTI vaccine will examine the correlations between total anti-FimH titer, functional responses, and rates of UTI recurrence. The assay described here is well suited to measure functional antibody responses in the range required for protection for other bacterial vaccines (e.g. 1:8 serum dilution for a pneumococcal vaccine, 1:4 serum dilution for a meningococcal vaccine), and viral vaccines (e.g. 1:4 serum dilution for hepatitis A, 1:40 serum dilution for influenza, 1:4–1:8 serum dilution for polio, and 1:20 for smallpox).Citation28,Citation29
We recognized the challenges of qualifying this assay prior to its start. The expression of type 1 pili is under phase variation, meaning that it is heterogeneously produced in E. coli populations. Therefore, even under laboratory growth conditions optimized to promote type 1 pili expression, pili expression varies day-to-day and among individual E. coli cells; individual E. coli may have 0–200 pili or more.Citation30 Therefore, laboratory assays investigating type 1 pili typically require dense populations of E. coli to ensure day-to-day reproducibility. We believe the 18 negative control wells per plate, 6 positive controls wells per plate, and the intermediate CFU/well control used as a cutoff for dilutions considered positive, resolved potential reproducibility issues. The intra-assay and inter-assay precision results support the reproducibility of the assay.
Another potential limitation of this assay is that E. coli has been demonstrated to bind to a variety of surfaces including polyvinyl chloride, polypropylene, polycarbonate, polystyrene, and borosilicate glass. E. coli can also bind to mammalian cells using alternative mechanisms not requiring type 1 pili; however, the medical relevance of these binding modes in UTI has not been established. This limitation of the assay was resolved by including 6 wells of mannose as a positive control on each plate to ensure that FimH-mediated binding is being measured. Furthermore, a detection method based on colony counting is not suitable for measuring thousands of serum samples from phase 2 and phase 3 human studies. The fluorescent readout of this assay is much better suited to the need for high-throughput analysis.
In summary, we optimized, qualified, and used a functional assay for measuring anti-FimH-mediated inhibition of E. coli binding to human bladder cells. This assay is designed to detect functional anti-FimH antibodies present in human serum samples after vaccination with the FimH antigen.
Contributors
GRE conceived of the ideas here and oversaw study design. All authors were involved in the experimental design and interpretation of data. JC and PMB performed the experiments. SMM provided advice and expertise. CMS and GRE wrote the manuscript.
Disclosure of potential conflicts of interest
This study was funded by Sequoia Sciences. All authors were employees, contractors, consultants, or shareholders of Sequoia Sciences.
Abbreviations
NHS | = | normal human serum |
RSD | = | relative standard deviation |
UTI | = | urinary tract infection |
rUTI | = | recurrent urinary tract infection |
Supplemental Material
Download Zip (10.7 MB)Acknowledgments
Rabbit antibodies were produced at Cocalico Biologicals, Inc. Human antibody was purified at American International Biotechnology Services.
Supplemental data
Supplemental data for this article can be accessed online at https://doi.org/10.1080/21645515.2020.1770034
Additional information
Funding
References
- IMS Health. National disease and therapeutic index, ims health, Plymouth Meeting. Plymouth Meeting (PA): IMS Health; 2014.
- Urologist UTI Survey. Sequoia Sciences, Inc. and HRA research. 2010.
- Trevino SE, Babcock HM, Henderson JP, Lane MA, Beekmann SE, Polgreen PM, Marschall J. Perceptions and behaviours of infectious diseases physicians when managing urinary tract infections due to MDR organisms. J Antimicrob Chemother. 2015;70:3397–400. doi:10.1093/jac/dkv271.
- Deak D, Outterson K, Powers JH, Kesselheim AS. Progress in the fight against multidrug-resistant bacteria? A review of U.S. Food and drug administration-approved antibiotics, 2010-2015. Ann Intern Med. 2016;165:363–72. doi:10.7326/M16-0291.
- Norrby SR, Nord CE, Finch R. Lack of development of new antimicrobial drugs: a potential serious threat to public health. Lancet Infect Dis. 2005;5:115–19. doi:10.1016/S1473-3099(05)70086-4.
- Payne DJ, Gwynn MN, Holmes DJ, Pompliano DL. Drugs for bad bugs: confronting the challenges of antibacterial discovery. Nat Rev Drug Discov. 2007;6:29–40. doi:10.1038/nrd2201.
- Rappuoli R, Bloom DE, Black S. Deploy vaccines to fight superbugs. Nature. 2017;552:165–67. doi:10.1038/d41586-017-08323-0.
- Abbott A. Vaccines promoted as key to stamping out drug-resistant microbes. Nature. 2017. doi:10.1038/nature.2017.22324.
- Wright KJ, Seed PC, Hultgren SJ. Development of intracellular bacterial communities of uropathogenic Escherichia coli depends on type 1 pili. Cell Microbiol. 2007;9:2230–41. doi:10.1111/j.1462-5822.2007.00952.x.
- Martinez JJ, Mulvey MA, Schilling JD, Pinkner JS, Hultgren SJ. Type 1 pilus-mediated bacterial invasion of bladder epithelial cells. Embo J. 2000;19:2803–12.
- Langermann S, Palaszynski S, Barnhart M, Auguste G, Pinkner JS, Burlein J. Prevention of mucosal Escherichia coli infection by FimH-adhesin-based systemic vaccination. Science. 1997;276:607–11. doi:10.1126/science.276.5312.607.
- Langermann S, Mollby R, Burlein JE, Palaszynski SR, Auguste CG, DeFusco A, Strouse R, Schenerman M, Hultgren S, Pinkner J, et al. Vaccination with fimH adhesin protects cynomolgus monkeys from colonization and infection by uropathogenic Escherichia coli. J Infect Dis. 2000;181:774–78. doi:10.1086/315258.
- Meiland R, Geerlings SE, Langermann S, Brouwer EC, Coenjaerts FE, Hoepelman AI. FimCH antiserum inhibits the adherence of Escherichia coli to cells collected by voided urine specimens of diabetic women. J Urol. 2004;171:1589–93. doi:10.1097/01.ju.0000118402.01034.fb.
- Thankavel K, Madison B, Ikeda T, Malaviya R, Shah AH, Arumugam PM, Abraham SN. Localization of a domain in the FimH adhesin of Escherichia coli type 1 fimbriae capable of receptor recognition and use of a domain-specific antibody to confer protection against experimental urinary tract infection. J Clin Invest. 1997;100:1123–36. doi:10.1172/JCI119623.
- Poggio TV, La Torre JL, Scodeller EA. Intranasal immunization with a recombinant truncated FimH adhesin adjuvanted with CpG oligodeoxynucleotides protects mice against uropathogenic Escherichia coli challenge. Can J Microbiol. 2006;52:1093–102. doi:10.1139/w06-065.
- O’Brien VP, Hannan TJ, Yu L, Livny J, Roberson EDO, Schwartz DJ, Souza S, Mendelsohn CL, Colonna M, Lewis AL, et al. A mucosal imprint left by prior Escherichia coli bladder infection sensitizes to recurrent disease. Nature Microbiol. 2016;2:16196. doi:10.1038/nmicrobiol.2016.196.
- Wurpel DJ, Beatson SA, Totsika M, Petty NK, Schembri MA. Chaperone-usher fimbriae of Escherichia coli. PLoS One. 2013;8:30. doi:10.1371/journal.pone.0052835.
- Korea CG, Badouraly R, Prevost MC, Ghigo JM, Beloin C. Escherichia coli K-12 possesses multiple cryptic but functional chaperone-usher fimbriae with distinct surface specificities. Environ Microbiol. 2010;12:1957–77. doi:10.1111/j.1462-2920.2010.02202.x.
- Rossez Y, Holmes A, Lodberg-Pedersen H, Birse L, Marshall J, Willats WG, Toth IK, Holden NJ. Escherichia coli Common Pilus (ECP) targets arabinosyl residues in plant cell walls to mediate adhesion to fresh produce plants. J Biol Chem. 2014;289:34349–65. doi:10.1074/jbc.M114.587717.
- Wurpel DJ, Totsika M, Allsopp LP, Hartley-Tassell LE, Day CJ, Peters KM, Sarkar S, Ulett GC, Yang J, Tiralongo J, et al. F9 fimbriae of uropathogenic Escherichia coli are expressed at low temperature and recognise Galβ1-3GlcNAc-containing glycans. PLoS One. 2014;9. doi:10.1371/journal.pone.0093177.
- Larsonneur F, Martin FA, Mallet A, Martinez-Gil M, Semetey V, Ghigo JM, Beloin C. Functional analysis of E scherichia coli Yad fimbriae reveals their potential role in environmental persistence. Environ Microbiol. 2016;18:5228–48. doi:10.1111/1462-2920.13559.
- Eldridge G, Martin SM Compositions of vaccines and adjuvants and methods for the treatment of urinary tract infections. US Patent 9,017,698, ed. St. Louis (MO): Sequoia Sciences, Inc.; 2015.
- Bioanalytical Method Validation Guidance for Industry. 2018. U.S. Department of health and human services food and drug administration center for drug evaluation and research (CDER). Silver Spring (MD): Center for Veterinary Medicine (CfVMC).
- Huttner A, Hatz C, van den Dobbelsteen G, Abbanat D, Hornacek A, Frolich R, Dreyer AM, Martin P, Davies T, Fae K, et al. Safety, immunogenicity, and preliminary clinical efficacy of a vaccine against extraintestinal pathogenic Escherichia coli in women with a history of recurrent urinary tract infection: a randomised, single-blind, placebo-controlled phase 1b trial. Lancet Infect Dis. 2017;17:528–37. doi:10.1016/S1473-3099(17)30108-1.
- Frenck RW Jr., Ervin J, Chu L, Abbanat D, Spiessens B, Go O, Haazen W, van den Dobbelsteen G, Poolman J, Thoelen S, et al. Safety and immunogenicity of a vaccine for extra-intestinal pathogenic Escherichia coli (ESTELLA): Aphase 2 randomised controlled trial. Lancet Infect Dis. 2019;19:631–40. doi:10.1016/S1473-3099(18)30803-X.
- Janssen Research & Development LLC. A study of three different doses of VAC52416 (ExPEC10V) in adults aged 60 to 85 years in stable health. [accessed 2020 Apr 3]. Clinicaltrials.gov Accession NCT03819049
- Abbanat D, Davies TA, Amsler K, He W, Fae K, Janssen S, Poolman JT, van den Dobbelsteen GPJM. Development and qualification of an opsonophagocytic killing assay to assess immunogenicity of a bioconjugated Escherichia coli vaccine. Clin Vaccinei Immunol. 2017;24:e00123–17. doi:10.1128/CVI.00123-17.
- Plotkin SA. Correlates of protection induced by vaccination. Clin Vaccine Immunol. 2010;17:1055–65. doi:10.1128/CVI.00131-10.
- Krah DL, Amin RD, Nalin DR, Provost PJ. A simple antigen-reduction assay for the measurement of neutralizing antibodies to hepatitis A virus. J Infect Dis. 1991;163:634–37. doi:10.1093/infdis/163.3.634.
- Greene SE, Hibbing ME, Janetka J, Chen SL, Hultgren SJ. Human urine decreases function and expression of type 1 pili in uropathogenic Escherichia coli. MBio. 2015;6:00820–15. doi:10.1128/mBio.00820-15.