ABSTRACT
Anthrax is a zoonotic disease caused by the gram-positive spore-forming bacteria Bacillus anthracis. There is a need for safe, highly effective, long-term storage vaccine formulations for mass vaccination. However, the development of new subunit vaccines based on recombinant protective antigen (rPA) faces the problem of vaccine antigen instability. Here, the potential of simultaneous application of two different approaches to stabilize rPA was demonstrated. Firstly, we employed spherical particles (SPs) obtained from the tobacco mosaic virus (TMV). Previously, we had reported that SPs can serve as an adjuvant and platform for antigen presentation. In the current work, SPs were shown to increase the stability of the full-size rPA without loss of its antigenic properties. The second direction was site-specific mutagenesis of asparagine residues to avoid deamidation that causes partial protein degradation. The modified recombinant protein comprising the PA immunogenic domains 3 and 4 (rPA3 + 4) was stable during storage at 4 and 25°C. rPA3 + 4 interacts with antibodies to rPA83 both individually and as a part of a complex with SPs. The results obtained can underpin the development of a recombinant vaccine with a full-size modified rPA (with similar amino acid substitutions that stabilize the protein) and SPs.
Introduction
Anthrax is a zoonotic disease caused by the gram-positive spore-forming bacterium Bacillus anthracis, which can be transmitted via the gastrointestinal, inhalation or skin routes and respectively leads to the development of intestinal, pulmonary and cutaneous form of the disease. The pulmonary form is the most dangerous – the mortality rate is 45–90%, even if the disease is diagnosed at an early stage and accompanied by antibacterial treatment.Citation1 Therefore B. anthracis aerosol-deposited spores can cause serious harm and commonly are considered to be one of the most potent substances to create biological weapons. B. anthracis is classified as a top class (Category A) bioterrorist agent by the United States Centers for Disease Control and Prevention.Citation2
Vaccination is an important method of controlling anthrax. The main direction in the development of modern vaccines against anthrax is focused on the creation of recombinant vaccines that include the protective antigen (PA). PA is a central component of toxins and plays a key role in protecting against toxigenic and encapsulated strains of B. anthracis.Citation3 PA is a four-domain protein (735 amino acid residues) with a molecular weight of 83 kDa (PA83). Most anthrax toxin-neutralizing antibodies recognize epitopes mapped on PA, and all four domains of PA contain these epitopes.Citation4 One of the main problems when creating subunit vaccines based on recombinant PA (rPA) is the instability of this protein. PA83 contains two sites sensitive to proteolytic cleavage: one by furin-like proteases (domain 1)Citation5 and one by chymotrypsin (domain 2).Citation6 It has also been shown that rPA instability can be associated with spontaneous deamidation of several asparagine residues in the protein, with deamidation rates increasing significantly with the use of aluminum hydroxide as an adjuvant.Citation7,Citation8 Deamidation of asparagine residues is a common cause of the chemical degradation of proteins.
Based on a number of recent data, one can say that rPA adsorbed on aluminum hydroxide is unstable and loses its ability to induce neutralizing antibodies during storage.Citation8-10 However, adjuvants based on aluminum hydroxide are employed in almost all rPA-based vaccine candidates currently used in clinical trials.Citation3 In our opinion, selecting a suitable adjuvant and stabilizing the full-size rPA molecule is one of the most important strategies for creating a new, effective anthrax vaccine.
At the moment, there are several classes of micro- and nanoparticles used in preclinical studies of anthrax vaccines. Their physicоchemical properties (particle size, polydispersity, shape, surface charge, etc.) may provide differences in immunogenic activity. Vaccines are usually formulated with aluminum, liposomes, emulsions and/or with other immunostimulating compounds.Citation3 In this paper, we propose a new approach for stabilizing rPA by adsorbing it on the surface of a structurally modified plant virus, which simultaneously plays the role of an effective immunostimulator and platform (depot) for presenting antigens. Lately, the development of methods of plant virology and genetic engineering techniques has led to the designing of candidate vaccines based on plant virus nanoparticles to protect against various infectious agents, cancers and autoimmune diseases in both humans and animals.Citation11 Plant virus nanoparticles are easy to upscale; they are inexpensive, resistant to external factors, biodegradable and have low toxicity as well.Citation12-15 There is evidence that nanoparticles of plant viruses will serve as central components of new prophylactic and therapeutic vaccines for broad application in humans and animals in the near future.Citation11
Previously, our laboratory has obtained structurally modified plant virus particles of spherical shape and controlled size (spherical particles – SPs), which were formed by brief heating of rod-shaped tobacco mosaic virus (TMV) to 94–98°C.Citation12,Citation16,Citation17 SPs have been shown to possess unique adsorption properties for the presentation of recombinant antigens; they are also a safe and effective adjuvant.Citation18-20
We propose a new approach for development of a recombinant anthrax vaccine based on rPA using SPs. Full-size rPA stabilization, as the main challenge, is planned to be solved in two ways: by adsorption of rPA on the surface of SPs, and by directed mutagenesis of sites that cause partial protein degradation.
To this end, we studied the stability and antigenic specificity of unmodified rPA83 upon adsorption on the surface of the SP, as well as part of the rPA molecule containing domains 3 and 4 (rPA3 + 4) with amino acid substitutions, presumably stabilizing the protein. This choice is explained by the following facts: 1) domains 3 and 4 contain at least 7 immunogenic epitopes: 581–601,Citation21 628–637,Citation22 684–688,Citation23 686–694,Citation24 692–703,Citation25 671–721Citation23 and 716–727;Citation25 monoclonal antibodies to each of them were shown to exhibit toxin-neutralizing activity; 2) two asparagine residues subjected to deamidation during storage are located in domain 4 (at the site of PA binding to the cell receptor) and their replacement by deamidation-resistant amino acid residues improves the stability of rPA, which leads to increasing the vaccine’s effectiveness and may improve its shelf-life.Citation26 At the same time, SPs in combination with rPA3 + 4 will be able to act as an effective immunostimulator and antigen depot. The obtained results can form the basis for the development of a recombinant vaccine with the genetically modified full-size rPA adsorbed on SPs.
Results and discussion
Spherical particles (SPs) of controlled size were obtained from the tobacco mosaic virus (TMV) preparation by brief heating to 98°C, as described earlier.Citation27 SPs were characterized employing two electron microscopy methods. Scanning electron microscopy image is presented in . Transmission electron microscopy micrographs were used for calculation the average SPs size that was 402 ± 67 nm.
Figure 1. Spherical particles (SPs) generated by thermal remodeling of TMV. Scanning electron microscopy, without staining. Bar, 2 μm
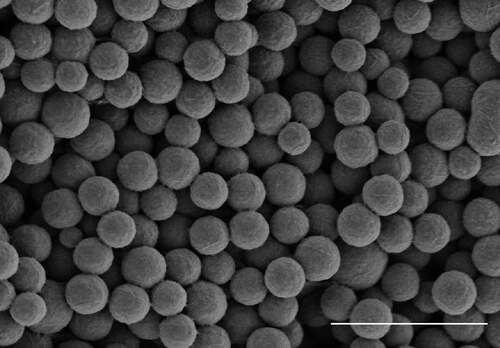
Complexes of SPs with rPA83 were obtained by incubating SPs with recombinant antigen in water (Milli-Q) at room temperature, as described previously.Citation20 To study the stability of rPA83, as well as rPA83 as part of complexes with SPs, they were incubated at +25°C for 12 days () and +4°C for 33 days (data not shown). Samples were taken every other day and analyzed in SDS–PAGE. demonstrates an improvement in the stability of rPA83 in complex with SPs, when compared to an individual protein. Thus, the bands corresponding to the position of a protein with a molecular weight of 83kDa on day 0 and day 2 of incubation had the same density either for preparations containing SPs (, lanes 1,3), or for controls without SPs (, lanes 2,4). However, as early as the 4th day of incubation, the density of the rPA83 band for preparations with SPs exceeded its density in the control. The band corresponding to individual rPA83 was clearly visible only on the 8th day (, lane 10). As for the preparation containing the SPs-rPA83 complexes, the band corresponding to rPA83 was readily detected, even on the 12th day of the experiment (, lane 13). These results indicate a positive effect of SPs on the stability of the protein to degradation during storage.
Figure 2. The stability of rPA83 within complexes with SPs. 1, 3, 5, 7, 9, 11, 13 – rPA83 within SPs-rPA83 complexes incubated under +25°C for 0, 2, 4, 6, 8, 10 and 12 days, respectively. 2, 4, 6, 8, 10, 12, 14 – Individual rPA83 incubated under +25°C for 0, 2, 4, 6, 8, 10 and 12 days, respectively. 15 – Protein molecular weight markers (molecular weights in kDa are indicated in the right).Red arrows indicate the considerable difference in stability between individual rPA83 and rPA83 within SPs-rPA83 complexes. Electrophoresis analysis, staining by Coomassie G-250
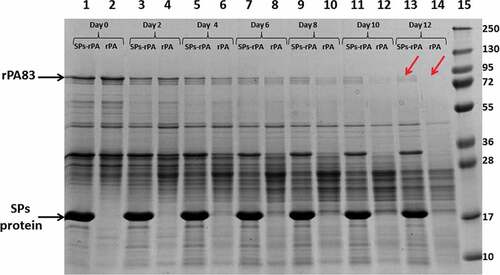
The efficiency of rPA83 adsorption on the surface of SPs and the antigenic specificity of rPA83 in the complex were analyzed by fluorescence microscopy and immune electron microscopy. rPA83 was conjugated with the fluorescent dye FITC (fluorescein isothiocyanate) as described earlier,Citation18 incubated with SPs in a mass ratio of 1:10, and SPs-rPA83FITC complexes were analyzed by fluorescence microscopy. Comparison of the images obtained in phase contrast (, I(a)) and fluorescence modes (, I(b)) enabled the establishment that all SPs formed complexes with fluorescently labeled rPA83. Aggregates of free rPA83FITC were not detected. The antigen specificity of rPA83 in the complex with SPs was analyzed by immune electron microscopy, as described previously.Citation20 As primary antibodies, we used mice anti-rPA83 serum; secondary antibodies were conjugated with colloidal gold. It was demonstrated that SPs-rPA83 complexes were associated with colloidal gold particles, which indicates that the proteins adsorbed on the surface of SPs retained their antigenic structure and specificity (, I(c)).
Figure 3. Bacillus anthracis recombinant antigens form complexes with SPs and retain antigenic specificity within complexes. (I) SPs-rPA83 complexes; (II) SPs-rPA3 + 4 complexes. (I) Phase contrast (a) and fluorescence microscopy (b) of SPs-rPA83FITC. (c) Immunoelectron microscopy of SPs-rPA83 complex. (II) Phase contrast (a) and fluorescence microscopy (b) of SPs-rPA3 + 4FITC. (c) Immunoelectron microscopy of SPs-rPA3 + 4 complex. rPA83FITC – rPA83 labeled with fluorescein isothiocyanate, rPA3 + 4FITC – rPA3 + 4 labeled with fluorescein isothiocyanate. For detection by immunogold microscopy was used primary antibodies to the rPA83 and secondary antibodies conjugated with colloidal gold (d = 12 nm) (“Jackson immunoresearch”, USA)
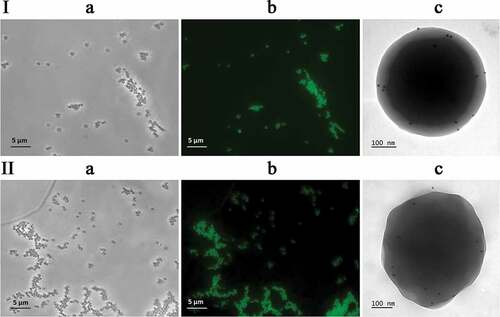
Thus, the results obtained with the full-size rPA83 showed that: 1) the rPA83 protein retained its antigenic activity after adsorption on SPs; 2) adsorption of rPA83 on the SP surface led to partial protection of the target antigen from proteolysis during storage. However, the resistance of the rPA83 protein (in complex with SPs) to degradation was incomplete. In this regard, it made sense to try a different approach to stabilize rPA.
At the next stage of this work, part of the rPA molecule containing domains 3 and 4 (rPA3 + 4) was modified by directed mutagenesis. This choice was based on the analysis of previous studies of another workgroups, which indicates that, firstly, domains 3 and 4 contain at least 7 epitopes with toxin-neutralizing activity (); and, secondly, the substitution of two asparagine residues located in domain 4 with glutamine residues was demonstrated to improve the stability of the full-size rPA83, which led to increased vaccine efficacy and may extend its shelf life.Citation26 The work by Flick-Smith et al. (2002), in which it was shown that, after immunization with a recombinant protein containing domains 3 and 4, mice challenged with 103 MLDs (lethal doses) of B. anthracis were protected and survived,Citation28 also had an impact on our decision.
Figure 4. Schematic representation of rPA3 + 4 protein. The amino acid sequence coordinates are given according to UniProt Q08G54. Epitopes and asparagine (N) to glutamine (Q) substitutions are indicated. The scheme is not to scale

An amino acid sequence of PA domains 3 and 4 from B. anthracis (GenBank 1TZN_A) str. A2012 was taken as the basis for further expression in E. coli.Citation29 Residues 1–248 corresponded to residues 488–735 of full-length Uniprot sequence Q08G54. Asparagines (N) 713 and 719 were substituted with glutamines (Q) in order to improve the stability of the target protein by avoiding deamidation, in accordance with the results by Verma and Burns (2018).Citation26
During the first stage, the initial amino acid sequence was subjected to reverse translation in silico using option “best E. coli genetic code” from the EditSeq program included in the Lasergene 11 software package. Additional optimization of the nucleotide sequence was carried out in two steps. The first step involved replacement of some frequently occurring codons without loss of protein synthesis efficiency. In particular, it affected several phenylalanine (Phe, ttt to ttc), asparagine (Asn, aat to aac), isoleucine (Ile, att to atc) and serine (Ser, tcc to tct) codons. The second step was aimed at finding and removing alleged cis-acting negative elements, such as cryptic bacterial transcription promoters and Shine-Dalgarno sequences, as well as extended A-rich stretches and direct repeats keeping the amino acid sequence intact. For example, one of the lysine (Lys) codons (aaa) was replaced with aag to split the sequence consisting of 7A (nucleotides 709–715). An optimized variant of synthetic PA3 + 4 gene was assembled from overlapping oligonucleotides by the Evrogen company (Moscow, Russia) and cloned into a pQE-30 expression vector (Qiagen, Germany) between BamHI and HindIII restriction sites. A schematic representation of rPA3 + 4 is shown in .
Expression of the rPA3 + 4 protein was performed in E. coli cells (strain SG). Protein accumulation after induction was high (Fig. S1(a)). Metal ion affinity chromatography with Ni-NTA-agarose (Qiagen, Germany) was employed for protein purification. The molecular weight of the rPA3 + 4 protein corresponded to 27.5 kDa, both during expression and after purification (Fig. S1(a) and S1(b)). The protein yield was approximately 20 mg per 1 liter of culture medium. Western blotting using mouse antiserum against unmodified full-size rPA (rPA83) and secondary antibodies conjugated with HRP demonstrated that the antiserum recognized purified rPA3 + 4 protein efficiently (Fig. S2(a) and S2(b), lane 1).
Analysis of the stability of purified rPA3 + 4 protein and its complexes with SPs at temperatures of +4°C (data not shown) and +25°C by SDS-PAGE was carried out. It showed that the protein remained stable for at least 16 days at either +4°C or +25°C, both in complexes with SPs (data not shown) and preparations without SPs (Fig. S3). In contrast to rPA83 (), degradation products were not detected.
Previously Flick-Smith and coauthors (2002) obtained the protein consisted of the 3rd and the 4th domains of B.anthracis as N-terminal GST fusion.Citation28 A nucleotide sequence was amplified from the natural source – Bacillus anthracis strain Sterne, expression was conducted in E. coli. The authors reported some degradation of purified GST-PA3 + 4 protein detected by Western blotting. The main distinctions of rPA3 + 4 obtained in present study are resistance to degradation (Fig. S3) and a very high expression level (Fig. S1(a)). These advantages are resulted by the presence of asparagine substitutions and the fact that expression of genetically modified rPA3 + 4 fused with 6-histidine tag was driven by the synthetic optimized PA3 + 4 gene ().
The purified rPA3 + 4 was conjugated with the fluorescent dye FITC and incubated with SPs (mass ratio 1:10) to obtain complexes. Analysis of this preparation was performed using phase contrast (, II(a)) and fluorescence microscopy (, II(b)). It demonstrated that all SPs were associated with the target protein molecules, while antigen aggregates were not detected. The antigenic specificity of rPA3 + 4 as a part of the complex with SPs was proved by indirect immune electron microscopy with antiserum to rPA83 as primary antibodies and secondary anti-mouse antibodies conjugated with colloidal gold. It was shown that SPs-rPA3 + 4 complexes were associated with gold particles (, II(c)). Hence, rPA3 + 4 was efficiently adsorbed on the surface of SPs and retained its antigenic activity as part of the complex.
To summarize in this study we have shown that SPs partially stabilize rPA83 and also have obtained stable protein – rPA3 + 4, consisted of domains 3 and 4 of rPA. For rPA3 + 4 the possibility to adsorption on SPs has been also demonstrated. It is important that both proteins being within complexes with SPs have been effectively recognized by anti-rPA83 antibodies. Therefore both approaches (mixing with SPs and introducing some stability-improving modifications into protein sequence) have been demonstrated to be applicable for further anthrax vaccine development.
However, this study has a limitation, which is concerned with the risk of insufficiency of only two domains of rPA to provide the full protection. In this respect in continuation we are planning to combine two methods examined in the present study and obtain the full-size rPA antigen stabilized by amino acid substitutions in combination with spherical particles (SPs). We suppose that studies of immunogenicity and protective efficacy make sense, after obtaining the modified full-size rPA83 in combination with spherical particles.
Disclosure of potential conflicts of interest
There are no conflicts of interest to declare.
Supplemental Material
Download MS Word (1.6 MB)Supplementary material
Supplemental data for this article can be accessed online at http://dx.doi.org/10.1080/21645515.2020.1772632.
Additional information
Funding
References
- Mourez M. Anthrax toxins. Rev Physiol Biochem Pharmacol. 2004;152:135–64. PMID: 15549606. doi:10.1007/s10254-004-0028-2.
- Rotz LD, Khan AS, Lillibridge SR, Ostroff SM, Hughes JM. Public health assessment of potential biological terrorism agents. Emerg Infect Dis. 2002;8:225–30. PMID: 11897082. doi:10.3201/eid0802.010164.
- Kondakova OA, Nikitin NA, Evtushenko EA, Ryabchevskaya EM, Atabekov JG, Karpova OV. Vaccines against anthrax based on recombinant protective antigen: problems and solutions. Expert Rev Vaccines. 2019;18(8):813–28. PMID: 31298973. doi:10.1080/14760584.2019.1643242.
- McComb RC, Martchenko M. Neutralizing antibody and functional mapping of Bacillus anthracis protective antigen - The first step toward a rationally designed anthrax vaccine. Vaccine. 2016;34(1):13–19. PMID: 26611201. doi:10.1016/j.vaccine.2015.11.025.
- Klimpel K, Molloy S, Thomas G, Leppla SH. Anthrax toxin protective antigen is activated by a cell-surface protease with the sequence specificity and catalytic properties of furin. Proc Natl Acad Sci USA. 1992;89(21):10277–81. PMID: 1438214. doi:10.1073/pnas.89.21.10277.
- Singh Y, Klimpel K, Arora N, Sharma M, Leppla SH. The chymotrypsin-sensitive site, Ffd315, in anthrax toxin protective antigen is required for translocation of lethal factor. J Biol Chem. 1994;269(46):29039–46. PMID: 7961869.
- Verma A, Ngundi MM, Burns DL. Mechanistic Analysis of the Effect of Deamidation on the Immunogenicity of Anthrax Protective Antigen. Clin Vaccine Immunol. 2016;23(5):396–402. PMID: 26912784. doi:10.1128/CVI.00701-15.
- D’Souza AJ, Mar KD, Huang J, Majumdar S, Ford BM, Dyas B, Ulrich RG, Sullivan VJ. Rapid deamidation of recombinant protective antigen when adsorbed on aluminum hydroxide gel correlates with reduced potency of vaccine. J Pharm Sci. 2013;102(2):454–61. PMID: 23242822. doi:10.1002/jps.23422.
- Wagner L, Verma A, Meade BD, Reiter K, Narum DL, Brady RA, Little SF, Burns DL. Structural and immunological analysis of anthrax recombinant protective antigen adsorbed to aluminum hydroxide adjuvant. Clin Vaccine Immunol. 2012;19(9):1465–73. PMID: 22815152. doi:10.1128/CVI.00174-12.
- Domínguez-Castillo RI, Verma A, Amador-Molina JC, Sirota L, Arciniega JL. Ability of ELISA and a toxin neutralization assay to detect changes in immunogenicity of a recombinant Bacillus anthracis protective antigen vaccine upon storage. Biologicals. 2013;41(2):111–14. PMID: 23137818. doi:10.1016/j.biologicals.2012.10.002.
- Balke I, Zeltins A. Use of plant viruses and virus-like particles for the creation of novel vaccines. Adv Drug Deliv Rev. 2019;145:119–29. PMID: 30172923. doi:10.1016/j.addr.2018.08.007.
- Nikitin NA, Malinin AS, Rakhnyanskaya AA, Trifonova EA, Karpova OV, Yaroslavov AA, Atabekov JG. Use of a polycation spacer for noncovalent immobilization of albumin on the thermally modified virus particles. Polym Sci Ser. 2011;53:1026–31. doi:10.1134/S0965545X11110083.
- Nikitin NA, Trifonova EA, Karpova OV, Atabekov JG. Biosafety of plant viruses for human and animals. Mosc Univ Biol Sci Bull. 2016;71(3):128–34. doi:10.3103/S0096392516030081.
- Nikitin NA, Zenin VA, Trifonova EA, Ryabchevskaya EM, Kondakova OA, Fedorov AN, Atabekov JG, Karpova OV. Assessment of structurally modified plant virus as a novel adjuvant in toxicity studies. Regul Toxicol Pharmacol. 2018;97:127–33. PMID: 29932979. doi:10.1016/j.yrtph.2018.06.010.
- Nikitin NA, Zenin VA, Trifonova EA, Ryabchevskaya EM, Yurkova MS, Kondakova OA, Fedorov AN, Atabekov JG, Karpova OV. Data in support of toxicity studies of structurally modified plant virus to safety assessment. Data in Brief. 2018;21:1504–07. doi:10.1016/j.dib.2018.10.102.
- Atabekov JG, Nikitin NA, Arkhipenko MV, Chirkov SV, Karpova OV. Thermal transition of native TMV and RNA-free viral proteins into spherical nanoparticles. J Gen Virol. 2011;92:453–56. PMID: 20980527. doi:10.1099/vir.0.024356-0.
- Atabekov JG, Nikitin NA, Karpova OV. New type of platforms for in vitro vaccine assembly. Moscow Univ Biol Sci Bull. 2015;70:177–83. doi:10.3103/S0096392515040045.
- Karpova OV, Nikitin NA, Chirkov SN, Trifonova EA, Sheveleva AA, Lazareva EA, Atabekov JG. Immunogenic compositions assembled from tobacco mosaic virus-generated spherical particle platforms and foreign antigens. J Gen Virol. 2012;93:400–07. PMID: 22049093. doi:10.1099/vir.0.036293-0.
- Trifonova EA, Nikitin NA, Gmyl AA, Lazareva EA, Karpova OV, Atabekov JG. Complexes assembled from TMV-derived spherical particles and entire virions of heterogeneous nature. J Biomol Struct Dyn. 2014;32:1193–201. PMID: 24099636. doi:10.1080/07391102.2013.816868.
- Trifonova EA, Zenin VA, Nikitin NA, Yurkova MS, Ryabchevskaya EM, Putlyaev EV, Donchenko EK, Kondakova OA, Fedorov AN, Atabekov JG, et al. Study of rubella candidate vaccine based on a structurally modified plant virus. Antivir Res. 2017;144:27–33. PMID: 28511994. doi:10.1016/j.antiviral.2017.05.006.
- Little SF, Novak JM, Lowe JR, Leppla SH, Singh Y, Klimpel KR, Lidgerding BC, Friedlander AM. Characterization of lethal factor binding and cell receptor binding domains of protective antigen of Bacillus anthracis using monoclonal antibodies. Microbiology. 1996;142:707–15. PMID: 8868446. doi:10.1099/13500872-142-3-707.
- Crowe SR, Ash LL, Engler RJM, Ballard JD, Harley JB, Farris AD, James JA. Select human anthrax protective antigen epitope-specific antibodies provide protection from lethal toxin challenge. J Infect Dis. 2010;202:251–60. PMID: 20533877. doi:10.1086/653495.
- Little S, Leppla S, Cora E. Production and characterization of monoclonal-antibodies to the protective antigen component of Bacillus–Anthracis toxin. Infect Immun. 1988;56:1807–13. PMID: 3384478. doi:10.1128/IAI.56.7.1807-1813.1988.
- Laffly E, Danjou L, Condemine F, Vidal D, Drouet E, Lefranc MP, Bottex C, Thullier P. Selection of a macaque Fab with framework regions like those in humans, high affinity, and ability to neutralize the protective antigen (PA) of Bacillus anthracis by binding to the segment of PA between residues 686 and 694. Antimicrob Agents Chemother. 2005;49:3414–20. PMID: 16048955. doi:10.1128/AAC.49.8.3414-3420.2005.
- Kelly-Cirino CD, Mantis NJ. Neutralizing monoclonal antibodies directed against defined linear epitopes on domain 4 of anthrax protective antigen. Infect Immun. 2009;77:4859–67. PMID: 19703971. doi:10.1128/IAI.00117-09.
- Verma A, Burns DL. Improving the stability of recombinant anthrax protective antigen vaccine. Vaccine. 2018;36(43):6379–82. PMID: 30228030. doi:10.1016/j.vaccine.2018.09.012.
- Trifonova EA, Nikitin NA, Kirpichnikov MP, Karpova OV, Atabekov JG. Obtaining and characterization of spherical particles – new biogenic platforms. Moscow Univ Biol Sci Bull. 2015;70:194–97. doi:10.3103/S0096392515040094.
- Flick-Smith HC, Walker NJ, Gibson P, Bullifent H, Hayward S, Miller J, Titball RW, Williamson ED. A recombinant carboxy-terminal domain of the protective antigen of Bacillus anthracis protects mice against anthrax infection. Infect Immun. 2002;70(3):1653–56. PMID: 11854261. doi:10.1128/iai.70.3.1653-1656.2002.
- Lacy DB, Wigelsworth DJ, Melnyk RA, Harrison SC, Collier RJ. Structure of heptameric protective antigen bound to an anthrax toxin receptor: a role for receptor in pH-dependent pore formation. Proc Natl Acad Sci U S A. 2004;101:13147–51. PMID: 15326297. doi:10.1073/pnas.0405405101.