ABSTRACT
SeVRSV is a replication-competent Sendai virus (SeV)-based vaccine carrying the respiratory syncytial virus (RSV) fusion protein (F) gene. Unmanipulated, non-recombinant SeV is a murine parainfluenza virus type 1 (PIV-1) and serves as a Jennerian vaccine for human PIV-1 (hPIV-1). SeV protects African green monkeys (AGM) from infection after hPIV-1 challenge. The recombinant SeVRSV additionally targets RSV and protects AGM from lower respiratory infections after RSV challenge. The present study is the first to report on the safety, viral genome detection, and immunogenicity following SeVRSV vaccination of healthy adults. Seventeen and four healthy adults received intranasal SeVRSV and PBS, respectively, followed by six months of safety monitoring. Virus genome (in nasal wash) and vaccine-specific antibodies (in sera) were monitored for two and four weeks, respectively, post-vaccination. The vaccine was well-tolerated with only mild to moderate reactions that were also present in the placebo group. No severe reactions occurred. As expected, due to preexisting immunity toward hPIV-1 and RSV in adults, vaccine genome detection was transient. There were minimal antibody responses to SeV and negligible responses to RSV F. Results encourage further studies of SeVRSV with progression toward a clinical trial in seronegative children.
Abbreviations: AE-adverse event; SAE-serious adverse event; SeV-Sendai virus; RSV-respiratory syncytial virus; PIV-1-parainfluenza virus-type 1; hPIV-1-human parainfluenza virus-type 1; F-RSV fusion protein; SeVRSV-recombinant SeV carrying the RSV F gene; Ab-antibody; MSW-medically significant wheezing; NOCMC-new onset chronic medical condition, mITT-modified Intent to Treat; ALRI-acute lower respiratory tract infection.
Members of the families Paramyxoviridae and Pneumoviridae include a diverse range of viruses such as human parainfluenza virus-type 1 (hPIV-1) and respiratory syncytial virus (RSV) that cause up to 30% of respiratory viral infections in children.Citation1 RSV is a major cause of acute lower respiratory tract infections (ALRI), including bronchiolitis and pneumonia in children worldwide.Citation2–5 There are approximately 34 million episodes of RSV-associated ALRI in children <5 years of age annually and up to 199,000 deaths per year due to RSV infections.Citation2 hPIV-1 is an important cause of laryngotracheobronchitis (croup) and can be associated with other infections that include upper and lower respiratory tract (URT and LRT) symptoms.Citation6 Parainfluenza viruses (types 1–3) account for 6.8% of hospital admissions in children <5 years with respiratory symptoms and up to 8% of influenza-like illnesses seen in the outpatient setting; hPIV-1 accounts for 30% of detected parainfluenza virusesCitation7,Citation8 Although there have been many previous attempts to develop vaccines against hPIV-1 and RSV, no vaccine against either virus has yet been licensed.Citation9,Citation10
Sendai virus (SeV) is a mouse parainfluenza virus-type 1 (PIV-1) that is being developed both as a vaccine for hPIV-1Citation11-13 and as a vector for other respiratory viruses like RSVCitation14-17 and metapneumovirus.Citation18 Previous studies showed that intranasal vaccination with SeV induced potent immune responses at mucosal and systemic sites and safely protected non-human primates from hPIV-1 challenge.Citation19,Citation20 Results provided proof-of-concept for this Jennerian approach; immune responses elicited by SeV were cross-reactive with the related hPIV-1 and controlled hPIV-1 infection. Further, when SeV was evaluated in a phase I clinical study in adultsCitation12 and in 3-6-year-old seropositive children,Citation13 the vaccine was well-tolerated. Despite being seropositive at study entry, some individuals developed increased SeV and hPIV-1 antibody titers after vaccination with SeV. SeV has also been used as a vector for several other vaccines including vaccines for human immunodeficiency virus (HIV).Citation21
Historically, there has been no confirmed case of human disease caused by SeV. However, when SeV was first discovered in the 1950s, it was thought to be the etiological agent for human respiratory infections. It was later noted that human samples had been passaged through mice before analyses, and it was determined that SeV had been inadvertently derived from mice, not humans.Citation22
SeVRSV is a replication-competent SeV-based vaccine carrying the RSV F gene. The product is being developed as a two-for-one vaccine to prevent hPIV-1 and RSV in infants. Although the vaccine expresses only one RSV F gene, it elicited cross-reactive neutralizing antibodies toward RSV A and B isolates and protected cotton rats from challenge with RSV A and RSV B.Citation14 It also protected African green monkeys from ALRI following RSV challengeCitation16. SeVRSV differs from protein-based or inactivated RSV vaccines, because the vector is applied intranasally and induces a long-lasting mucosal and systemic immune response. SeVRSV also differs from live-attenuated RSV vaccines, because it is not derived from a human pathogen and does not carry the concern that it will revert by mutation to its natural pathogenic form. Here we describe a phase I study in human adults to test the safety and immunogenicity of intranasal SeVRSV with the ultimate goal of developing a successful pediatric RSV/hPIV-1 vaccine.
We conducted a randomized, double-blind placebo-controlled single center, phase 1 study of the RSV/hPIV-1 vaccine candidate (SeVRSV). The institutional review board at Cincinnati Children’s Hospital Medical Center, Cincinnati, OH (CCHMC) approved the study protocol and informed consent documents before enrollment (clinicaltrials.gov NCT03473002). The primary objectives of the study were to 1) evaluate the safety and reactogenicity of a single intranasal dose of study vaccine, 2) assess the immunogenicity of SeV and the RSV F protein 28 days post receipt of the study vaccine, and 3) measure vaccine virus RNA from nasal washes up to 14 days after receipt of the study vaccine. Serious adverse events and experiences (SAEs), new onset chronic medical conditions (NOCMCs), medically significant wheezing, and non-routine medical visits (including lab values that met SAE criteria) were documented from the time of study vaccination through approximately 6 months after study vaccination. The date of first enrollment was June 11, 2018 and the date of last completed was February 14, 2019.
Study participants were healthy adults 18–45 years of age. Participants were excluded for acute illness within 72 hours prior to study vaccination, chronic medical conditions (such as chronic sinus disease, anatomic disorders of the nares, and some respiratory diseases), pregnancy or breastfeeding during the study period, and hypersensitivity to eggs or components of the study vaccine. Participants were also excluded for receipt of immunosuppressive agents, blood, or immunoglobulin products or receipt of a live vaccine within 30 days or an inactivated vaccine within 14 days of the study vaccination. Additionally, participants in contact with children <6 months of age or immunocompromised patients, healthcare workers in contact with children, or daycare providers were not enrolled.
The study vaccine, SeVRSV, was a modified, replication-competent recombinant SeV that was propagated in chick eggs and harvested from the allantoic cavity.Citation12,Citation13 SeVRSV was produced by insertion of the full-length RSV F gene, isolated from RSV-A2, between the F and HN genes of SeV.Citation14,Citation15 The vaccine was formulated in phosphate buffered saline (PBS) with human serum albumin and was stored frozen at −70°C to −90°C. The vaccine was administered on study day 0 using a syringe to apply the virus suspension intranasally. Venous blood was collected immediately prior to vaccination on study day 0 and on study day 28 for evaluations of vaccine-induced IgG antibodies with specificities for SeV or the RSV F protein by ELISA.
Participant health screening included history and physical, ECG, and laboratory assessment of erythrocyte sedimentation rate, human immunodeficiency virus, hepatitis B surface antigen, hepatitis C virus, urine opiates, white blood cell count, absolute neutrophil count, hemoglobin, platelets, total bilirubin, liver enzymes, creatinine, urine protein, and HgbA1c. Safety labs, including white blood cell count, absolute neutrophil count, hemoglobin, platelets, fibrinogen, total bilirubin, liver enzymes, creatinine, c-reactive protein and electrolytes, were repeated on study day 7. Since this was a first-in-human phase 1 study, enrollment of participants began with two sentinel participants who received the SeVRSV vaccine. After safety was established, the remaining participants were randomized in a 4:1 ratio (SeVRSV:placebo) to receive a single intranasal dose of 1 × 107 EID50 (Egg Infectious Dose infecting 50% of eggs) or PBS placebo delivered by syringe in 0.22 ml to each nostril (total 0.44 ml). The randomization scheme was generated by the Statistical and Data Coordinating Center (SDCC) and provided to unblinded study personnel. A designated individual at the site maintained a code list for emergency unblinding purposes.
The vaccine or placebo was administered on Study day 0 and adverse events (AEs) including local (nasal/throat) and systemic symptoms as well as temperatures were recorded daily by the participant on a memory log through Day 14. Memory aids were reviewed with the participants by study staff at study visits on days 2, 4, 7, and 14. Solicited and unsolicited AEs were summarized by severity (none, mild, moderate, or severe) for each day after vaccination. Nasal wash samples were collected on days 2, 4, 7, and 14 and used to measure the vaccine virus RNA genome. A portion of the nasal wash was reserved and frozen at −65°C to test for intercurrent respiratory infections if/when any respiratory AEs were reported. Interim medical history was obtained at follow-up visits on days 2, 4, 7, 14, and 28 to assess for new medical conditions. A phone call was placed on days 60 and 180 to evaluate the health of the participant and potential SAEs, NOCMCs, medically significant wheezing (MSW), and non-routine medical visits. If a participant developed wheezing or respiratory distress (or if there was a new medication prescribed for wheezing or respiratory distress or use of daily bronchodilator therapy), the participant was to be seen by the study team.
Safety, reactogenicity, and vaccine virus genome detection after vaccination were the primary outcomes measured and data analyses included all participants who received one dose of vaccine (either SeVRSV or placebo). The protocol was designed with a target size of 16 vaccinees (20 total participants), suggested as a convenience sample to detect with a > 80% probability an AE that occurred at a frequency of 10% and with a 97% probability an AE that occurred at a frequency of 20%. Statistical analyses of study results were performed with GraphPad Prism Software and SAS 9.4.
A total of 46 participants were screened for study eligibility and 21 participants were enrolled (13 females, 8 males). Seventeen participants received SeVRSV and four participants received placebo. Of the 21 participants enrolled, 20 completed the study per protocol. One participant from the placebo group completed all visits except for the day 180 visit and one participant in the SeVRSV group was found to be ineligible at baseline after receiving study vaccine because of unreported hyperthyroidism. A modified Intent-to-Treat (mITT) analysis was performed and included all participants (21/21), based on their contribution of a Day 0 and Day 28 venous blood sample. A per protocol analysis was also completed (excluding the one ineligible participant) and results were found to be similar to the mITT analysis. Thus, only the mITT analysis is reported here. The majority of participants were non-Hispanic (95%) and white (67%) with a mean age of 30.6 years (range 18–41 years) ().
Table 1. Summary of baseline characteristics and solicited local/systemic events
AEs were common during the study period with 16 of 21 participants (76%) experiencing at least one local solicited AE and 12 of 21 participants (57%) reporting one or more systemic AE (). No severe solicited or unsolicited reactions occurred. Solicited AEs occurred in 13 of the 17 participants in the SeVSRV group with the most common systemic symptom of headache (8, 47%) and the most common local symptoms of runny nose (8, 47%) and sore throat (8, 47%). Symptoms were not consistently associated with detection of virus genome (described below) as four of five vaccinees with no detectable virus genome developed respiratory symptoms. Local symptoms were also noted in three (75%) of the placebo recipients. In the SeVRSV group, 10 of 17 participants (59%) reported a treatment-related unsolicited AE with a nasal mucosal disorder and/or pharyngeal erythema being most common. The placebo group reported a treatment-related unsolicited AE in 1 of 4 (25%) participants with nasal inflammation, nasal mucosal disorder, and pharyngeal erythema. No significant changes in laboratory chemistries or hematology results related to study treatment were detected.
Vaccine virus genome detection was by PCR. Briefly, nasal wash samples were extracted by use of the Qiagen Viral RNA kit (Qiagen, Germantown, MD). For PCR, one set of primers and probe (Biosearch Technologies, Inc, Radnor, PA) targeted the SeV nucleoprotein gene (GenBank accession number M30202). Evaluation of these primers and probe in various software programs determined that they would detect SeV but not hPIV-1 and hPIV-3 based on the sequences of the viruses and primers. Another set of primers and probes were developed to detect the RSV F gene (GenBank accession number KJ155694.1). An internal control, MS2, was added to the nasal wash samples prior to extraction to control for extraction efficiency and any PCR inhibitors contained in the nasal wash samples.
PCR signals were noted in most study vaccine recipients early after vaccination, but detection decreased rapidly (). SeV RNA was detected in 11 of the 17 (65%) vaccine recipients on Day 2, three participants (18%) on Day 4, two participants (12%) on Day 7, and no participants on day 14. RSV F RNA was detected in seven of the 17 (41%) vaccine recipients on day 2, in two participants (12%) on day 4, in two participants (12%) on Day 7, and in no participants on day 14. Altogether, vaccine virus RNA was detected on at least one day in 71% of SeVRSV vaccine recipients by PCR and no vaccine virus RNA was identified in the placebo group (N = 4, data not shown). In previous studies of wildtype SeV in adultsCitation12 or seropositive children aged 3–6 years,Citation13 an assay that measured replication-competent virus rather than RNA was used. No replication competent virus was detected in any adult or 3–6 year-old-seropositive child after vaccination in these studies.
Figure 1. Detection of SeV or RSV F protien sequence by PCR
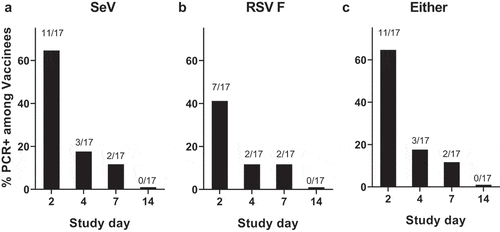
Antibody titers were determined by ELISAs using plates coated with either RSV F protein (Sino Biological Inc., Wayne PA) or parainfluenza virus type 1 strain Sendai (Meridian Life Sciences, Inc., Cincinnati, OH) overnight. Serum samples were serially diluted and added to the plates along with two reference standards which were assigned values of 8,000 Units/mL or 500 Units/mL respectively. After incubations of sera on ELISA plates (and washes), biotin conjugated anti-human IgG (Jackson ImmunoResearch, West Grove, PA) was added. The PK6100 reagent kit (Vector Laboratories, Inc., Burlingame, CA) was used to form a biotin-avidin-horse radish peroxidase complex. The substrate, O-phenylenediamine (Sigma-Aldrich Corp., St. Louis, MO) was then added and the reaction stopped. Optical densities were read at 492 nm and the quantity of either RSV or SeV-specific IgG was calculated from curves generated by the reference standards.
Of the 17 participants in the SeVRSV study vaccine group, only three (18%) exhibited a four-fold increase in SeV (PIV-1) antibody titers after vaccination and none exhibited a four-fold rise in RSV antibody titers (). The geometric mean fold rise (GMFR) for SeV-specific antibody was 2.0 and for RSV F-specific antibody was 1.1 in the vaccine group (). No recipients in the placebo group (N = 4) had evidence of antibody rise to either SeV or RSV. Of note, SeVRSV vaccine recipients with the greatest change in immune responses were young (≤20-years-old) with low baseline SeV antibody titers prior to study vaccine receipt (; see Supplementary Figure 1 for graphs of longitudinal antibody titers for each study participant). When all study vaccine recipients were evaluated, baseline SeV antibody titers correlated inversely and significantly with changes in antibody titers between days 0 and 28 ().
Table 2. Antibody response by treatment group in the modified intent-to-treat population
Figure 2. Antibody responses toward SeV and RSV F
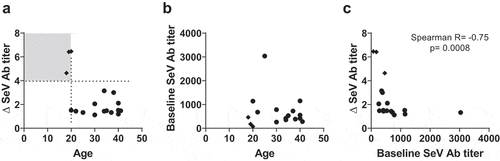
This study is the first to report on the safety, immunogenicity, and viral genome detection of SeVRSV in healthy adults. Since virtually all adults have previously been infected with hPIV-1 and RSV and all participants had detectable antibody prior to vaccination, we expected little to no SeV replication and a minimal immune response to vaccination. The four-fold increases in antibody responses to SeV (PIV-1) in three vaccinees may have been induced by the SeV particles administered as study vaccine and may not have indicated vaccine virus replication. This is further supported by the lack of an RSV F-specific antibody response. An RSV F-specific antibody response would require RSV F protein expression by infected cells, because the protein is not part of the SeVRSV particle. Previous clinical trials with unmanipulated, non-recombinant SeV showed similar results in adults.Citation12 There were higher antibody response rates toward non-recombinant SeV in childrenCitation13 compared to adults, although the number of participants immunized in those studies was small.
While the testing of a first-in-human vaccine must be initiated in adults for safety purposes, the information obtained from SeVRSV testing in adults is limited. This is because the preexisting immune responses in adults toward hPIV-1 cross-react with SeV and inhibit vaccine replication.Citation12 A thorough safety study of SeVRSV will require tests in sero-negative children. Similarly, because the SeVRSV vaccine does not ‘take’ in adults, a thorough test of SeVRSV immunogenicity must await analyses in sero-negative children, wherein robust immune responses toward hPIV-1 and RSV are expected.
In conclusion, the study showed that the vaccine was well-tolerated, with only mild to moderate reactions that were also present in the placebo vaccine group. No clear relationship between vaccine virus detection and symptoms were noted. As expected, due to preexisting immunity in the study population, the vaccine genome detection was transient. There was a minimal antibody response to SeV and a negligible response to the RSV F protein in these seropositive adults. Previous clinical evaluations and the findings presented here suggest that further SeVRSV vaccine studies with progression toward the vaccine’s target population of seronegative children are appropriate.
Supplemental Material
Download MS Word (51.2 KB)Acknowledgments
We thank Sherri L. Surman and Dr. Rhiannon R. Penkert for assistance with vaccine development, Dr. Elisabeth Adderson for assistance with vaccine protocol development, Sonnie Kim and Robin Mason of the NIH for assistance with logistical and regulatory affairs, and Dr. Susan Sleep for assistance with vaccine testing. This work was supported in part by funds from ALSAC, the National Cancer Institute, P30CA21765 and the National Institute of Allergy and Infectious Diseases, the National Institutes of Health, under contracts HHSN272200800006C (Cincinnati Children’s Hospital) and HHSN272201500002C (The Emmes Company LLC).
Supplemental material
Supplemental data for this article can be accessed on the publisher’s website.
Additional information
Funding
References
- Griffin MR, Walker FJ, Iwane MK, Weinberg GA, Staat MA, Erdman DD. Epidemiology of respiratory infections in young children: insights from the new vaccine surveillance network. Pediatr Infect Dis J. 2004;23:S188–92. doi:10.1097/01.inf.0000144660.53024.64.
- Nair H, Nokes DJ, Gessner BD, Dherani M, Madhi SA, Singleton RJ, O’Brien KL, Roca A, Wright PF, Bruce N, et al. Global burden of acute lower respiratory infections due to respiratory syncytial virus in young children: a systematic review and meta-analysis. Lancet. 2010;375(9725):1545–55. doi:10.1016/S0140-6736(10)60206-1.
- Shi T, McLean K, Campbell H, Nair H. Aetiological role of common respiratory viruses in acute lower respiratory infections in children under five years: A systematic review and meta–analysis. J Glob Health. 2015;5:010408.
- Couch RB, Englund JA. Respiratory viral infections in immunocompetent and immunocompromised persons. Am J Med. 1997;102:2–9. doi:10.1016/S0002-9343(97)00003-X.
- Hall CB, Weinberg GA, Iwane MK, Blumkin AK, Edwards KM, Staat MA, Auinger P, Griffin MR, Poehling KA, Erdman D, et al. The burden of respiratory syncytial virus infection in young children. N Engl J Med. 2009;360:588–98. doi:10.1056/NEJMoa0804877.
- Counihan ME, Shay DK, Holman RC, Lowther SA, Anderson LJ. Human parainfluenza virus-associated hospitalizations among children less than five years of age in the United States. Pediatr Infect Dis J. 2001;20:646–53. doi:10.1097/00006454-200107000-00003.
- Steffens A, Finelli L, Whitaker B, Fowlkes A. Population-based surveillance for medically attended human parainfluenza viruses from the influenza incidence surveillance project, 2010-2014. Pediatr Infect Dis J. 2016;35:717–22. doi:10.1097/INF.0000000000001140.
- Weinberg GA, Hall CB, Iwane MK, Poehling KA, Edwards KM, Griffin MR, Staat MA, Curns AT, Erdman DD, Szilagyi PG, et al. Parainfluenza virus infection of young children: estimates of the population-based burden of hospitalization. J Pediatr. 2009;154:694–99. doi:10.1016/j.jpeds.2008.11.034.
- Schmidt AC, Schaap-Nutt A, Bartlett EJ, Schomacker H, Boonyaratanakornkit J, Karron RA, Collins PL. Progress in the development of human parainfluenza virus vaccines. Expert Rev Respir Med. 2011;5(4):515–26. doi:10.1586/ers.11.32.
- Rossey I, Saelens X. Vaccines against human respiratory syncytial virus in clinical trials, where are we now? Expert Rev Vaccines. 2019;18(10):1053–67. doi:10.1080/14760584.2019.1675520.
- Hurwitz JL. Development of recombinant Sendai virus vaccines for prevention of human parainfluenza and respiratory syncytial virus infections. Pediatr Infect Dis J. 2008;27(Supplement):S126–8. doi:10.1097/INF.0b013e318168b780.
- Slobod KS, Shenep JL, Lujan-Zilbermann J, Allison K, Brown B, Scroggs RA, Portner A, Coleclough C, Hurwitz JL. Safety and immunogenicity of intranasal murine parainfluenza virus type 1 (Sendai virus) in healthy human adults. Vaccine. 2004;22(23–24):3182–86. doi:10.1016/j.vaccine.2004.01.053.
- Adderson E, Branum K, Sealy RE, Jones BG, Surman SL, Penkert R, Freiden P, Slobod KS, Gaur AH, Hayden RT, et al. Safety and immunogenicity of an intranasal Sendai virus-based human parainfluenza virus type 1 vaccine in 3- to 6-year-old children. Clin Vaccine Immunol. 2015;22(3):298–303. doi:10.1128/CVI.00618-14.
- Zhan X, Hurwitz JL, Krishnamurthy S, Takimoto T, Boyd K, Scroggs RA, Surman S, Portner A, Slobod KS. Respiratory syncytial virus (RSV) fusion protein expressed by recombinant Sendai virus elicits B-cell and T-cell responses in cotton rats and confers protection against RSV subtypes A and B. Vaccine. 2007;25(52):8782–93. doi:10.1016/j.vaccine.2007.10.038.
- Zhan X, Slobod KS, Jones BG, Sealy RE, Takimoto T, Boyd K, Surman S, Russell CJ, Portner A, Hurwitz JL, et al. Sendai virus recombinant vaccine expressing a secreted, unconstrained respiratory syncytial virus fusion protein protects against RSV in cotton rats. Int Immunol. 2015;27(5):229–36. doi:10.1093/intimm/dxu107.
- Jones BG, Sealy RE, Rudraraju R, Traina-Dorge VL, Finneyfrock B, Cook A, Takimoto T, Portner A, Hurwitz JL. Sendai virus-based RSV vaccine protects African green monkeys from RSV infection. Vaccine. 2012;30(5):959–68. doi:10.1016/j.vaccine.2011.11.046.
- Jones BG, Sealy RE, Surman SL, Portner A, Russell CJ, Slobod KS, Dormitzer PR, DeVincenzo J, Hurwitz JL. Sendai virus-based RSV vaccine protects against RSV challenge in an in vivo maternal antibody model. Vaccine. 2014;32(26):3264–73. doi:10.1016/j.vaccine.2014.03.088.
- Russell CJ, Jones BG, Sealy RE, Surman SL, Mason JN, Hayden RT, Tripp RA, Takimoto T, Hurwitz JL. A Sendai virus recombinant vaccine expressing a gene for truncated human metapneumovirus (hMPV) fusion protein protects cotton rats from hMPV challenge. Virology. 2017;509:60–66. doi:10.1016/j.virol.2017.05.021.
- Hurwitz JL, Soike KF, Sangster MY, Portner A, Sealy RE, Dawson DH, Coleclough C. Intranasal Sendai virus vaccine protects African green monkeys from infection with human parainfluenza virus-type one. Vaccine. 1997;15(5):533–40. doi:10.1016/S0264-410X(97)00217-X.
- Surman SL, Rudraraju R, Sealy R, Jones B, Hurwitz JL. Vitamin A deficiency disrupts vaccine-induced antibody-forming cells and the balance of IgA/IgG isotypes in the upper and lower respiratory tract. Viral Immunol. 2012;25:341–44. doi:10.1089/vim.2012.0023.
- Ishii H, Matano T. Development of an AIDS vaccine using Sendai virus vectors. Vaccine. 2015;33:6061–65. doi:10.1016/j.vaccine.2015.06.114.
- Lamb RA and Griffith DP. Paramyxoviridae: The viruses and their replication. In: Fields Virology. Editors: Knipe DM and Howley PM, Philadelphia, PA: Lippincott Williams & Wilkins; 2007:1449-96.