ABSTRACT
The most widely used influenza vaccines are prepared by chemical inactivation. However, chemical, especially formalin, treatment-induced modifications of the antigenic structure of the virus are frequently associated with adverse effects including low efficacy of protection, unexpected immune responses, or exacerbation of disease. Gamma-irradiation was suggested as an alternative influenza virus inactivation method due to its great features of completely inactivating virus while not damaging the structures of protein antigens, and cross-protective ability against heterologous strains. However, immunological features of gamma radiation-inactivated influenza vaccine have not been fully understood. In this study, we aimed to investigate the humoral and cellular immune responses of gamma radiation-inactivated influenza vaccine. The gamma irradiation-inactivated influenza vaccine (RADVAXFluA) showed complete viral inactivation but retained normal viral structure with functional activities of viral protein antigens. Intranasal immunization of RADVAXFluA provided better protection against influenza virus infection than formalin-inactivated influenza virus (FIV) in mice. RADVAXFluA greatly enhanced the production of virus-specific serum IgG and alveolar mucosal IgA, which effectively neutralized HA (hemagglutinin) and NA (neuraminidase) activities, and blocked viral binding to the cells, respectively. Further analysis of IgG subclasses showed RADVAXFluA-immunized sera had higher levels of IgG1 and IgG2a than those of FIV-immunized sera. In addition, analysis of cellular immunity found RADVAXFluA induced strong dendritic cells (DC) activation resulting in higher DC-mediated activation of CD8+ T cells than FIV. The results support improved immunogenicity by RADVAXFluA.
Introduction
Influenza viruses are major human respiratory viral pathogens causing seasonal epidemics and pandemics with significant morbidity, mortality, and economic loss worldwide.Citation1 It is estimated to cause 3–5 million severe infections and 250,000–500,000 deaths globally per year, which mostly include the elderly and young children.Citation2 The World Health Organization (WHO) specified that vaccination is the most powerful and effective way of preventing severe outcomes caused by influenza virus infection.Citation3 However, current influenza vaccines have to be re-formulated and re-administrated on an annual basis because of viral antigenic drifts and low immunogenic efficacy.Citation4 Although several new advanced vaccines, including virus-like particle (VLP), live attenuated vaccine, or vectored vaccine, have been proposed, the most widely used vaccines are prepared by chemical inactivation to eliminate infectivity and ensure the safety.
In general, inactivated influenza vaccine is produced by treatment with chemical reagents such as formalin or β-propiolactone (BPL) followed by splitting with Triton X-100 (Fluzone®), sodium deoxycholate (Flulaval® and Fluzrix®), or nonylphenol ethoxylate (Fluvirin®).Citation5 Although split influenza vaccines are more commonly used nowadays than whole inactivated vaccines, they are associated with several limitations, including lower productivity, difficulties in quality control, higher cost, and low efficacy against novel pandemic viruses.Citation6–8 In addition, previous studies suggested that formalin treatment alters the structure of viral proteins by cross-linking proteins and nucleic acids, which might induce several unexpected immune responses. For example, formalin-inactivated respiratory syncytial virus (RSV) in naive infants failed to prevent disease, and 80% of vaccine recipients were hospitalized after encountering circulating RSV.Citation9 These adverse reactions act as bottlenecks in vaccine-based strategies for effectively and safely preventing the onset of infectious diseases. Therefore, alternative ways of inactivating viruses while improving efficacy and safety are required to overcome these drawbacks.
To overcome the problems associated with the existing inactivation methods, several new methods have been proposed that can preserve the integrity of the antigens while maximizing the immuno-potency of the inactivated viruses. Amanna et al.Citation10 proposed a hydrogen peroxide-based viral inactivation method, the oxidative reaction of which causes irreversible damage to DNA and RNA but relatively lesser damage to common protein antigens. The killed but metabolically active (KBMA) vaccine was produced by the combined treatment of psoralen and ultraviolet A (UVA) irradiation. Microbial propagation was inhibited in KBMA, but it was metabolically active to invade the host immune cell, followed by activation of the host cell-mediated immune response.Citation11,Citation12 KBMA was also used in an animal model for tumor therapy.Citation13
Gamma-irradiation has been investigated as an alternative inactivation method for vaccine production as it is relatively simple, cost-effective, and does not require the use of any toxic chemicals.Citation14 Although the usage of gamma-irradiation was most widely studied in food and medical device sterilization industries, it is also used to develop inactivated whole-cell vaccines for many tricky pathogens. SAV0001, an inactivated human immunodeficiency virus (HIV) vaccine by gamma-irradiation, showed significantly increased levels of gp120- and P24-specific antibodies without adverse effects in phase I clinical trial.Citation15 The study with gamma-irradiated Brucella melitensis showed that it lost replication capacity, but retained protective features against live Brucella, such as induction of cell-mediated cytotoxic T cell response in mouse model.Citation16 Intranasal immunization with gamma-irradiated Streptococcus pneumoniae elicited IL-17 production from innate immune cells, which was required to clear the pneumococcal colonization.Citation17 In addition, gamma-irradiated influenza A virus vaccine (γ-flu) also has been reported previously, which induced 100% cross-protective immunity against not only homologous but also heterologous influenza A strains via the activation of humoral and cytotoxic T-cell responses.Citation18,Citation19
Although gamma-irradiation method shows a promising potential for the development of hetero-protective universal influenza vaccine, immunological features underlying gamma-irradiated influenza virus-induced protection have not been fully understood yet. In this study, an inactivated whole-cell influenza A virus vaccine was developed using gamma-irradiation and its humoral and cellular immunological features and protective efficacy were investigated and compared with chemically inactivated one. Gamma-irradiated influenza A virus (RADVAXFluA) showed a complete viral inactivation while retaining normal viral structure with functionally active viral protein antigens, such as neuraminidase (NA) and hemagglutinin (HA). Intranasal immunization of RADVAXFluA in mice provided complete protection against influenza A virus infection, whereas some of the mice immunized with formalin-inactivated influenza virus vaccine (FIV) suffered from infection. The subsequent analysis of humoral immune responses of RADVAXFluA-immunized mice found superior serum IgG and airway mucosal IgA antibody productions, which were functionally effective in neutralizing HA and NA activities and viral binding to airway epithelial cells, compared to FIV-immunized mice. Unlike great induction of both IgG2a and IgG1 antibodies in RADVAXFluA-immunized mice, FIV immunization failed to induce IgG1 antibody production, which may explain no neutralizing effect of FIV-immunized serum against HA and NA. Moreover, RADVAXFluA induced stronger activation of dendritic cells (DCs), which induced T helper (Th) cell-independent CD8+ T cell activation by DCs. These results show superior immunization effects of RADVAXFluA compared to FIV and reveal unique immunological features induced by RADVAXFluA toward developing universal influenza vaccine using gamma-irradiation.
Materials and methods
Reagents
Dulbecco’s modified Eagle’s medium (DMEM)/nutrient mixture F-12 (DMEM/F-12) was purchased from Sigma-Aldrich (Saint Louis, MO, USA). Roswell Park Memorial Institute (RPMI)-1640 medium and fetal bovine serum (FBS) were purchased from Gibco (Grand Island, NY, USA). Penicillin-streptomycin solution was purchased from HyClone (Logan, UT, USA). Recombinant murine granulocyte macrophage-colony stimulating factor (GM-CSF) was obtained from R & D Systems (Minneapolis, MN, USA). Anti-influenza A virus nucleoprotein antibody was obtained from Abcam (Cambridge, UK). 4ʹ,6-diamidino-2-phenylindole (DAPI) was purchased from Invitrogen (Carlsbad, CA, USA). PE-labeled anti-mouse CD80 (clone: 16–10A1), APC-labeled anti-mouse CD86 (clone: GL1), and PE/Cy5-labeled anti-mouse MHC-II (clone: M5/114.15.2) antibodies were purchased from eBioscience (San Diego, CA, USA). FITC-labeled anti-mouse CD3 (clone: 17A2), PerCP-labeled anti-mouse CD4 (clone: RM 4–5), PerCP-labeled anti-mouse CD8 (clone: 53–6.7), and PE-labeled anti-mouse IFN-γ (clone: XMG 1.2) antibodies were purchased from BD Biosciences (San Diego, CA, USA). Horseradish peroxidase (HRP)-conjugated anti-mouse IgG, IgA, IgG2a, and IgG1 were purchased from Southern Biotech (Birmingham, AL, USA).
Virus and cell culture
The influenza virus A/California/04/09 (CA0409) was provided by the International Vaccine Institute (Seoul, Korea). The viruses were grown in the allantoic cavities of 10-day-old embryonated chicken eggs for 48 h at 37°C. Then, the viruses were harvested from the allantoic fluid by sucrose density gradient centrifugation and stored at −80°C until further use. The human alveolar epithelial A549 cell was obtained from the American Type Culture Collection (Manassas, VA, USA). A549 cells were cultured in DMEM/F-12 medium supplemented with 10% heat-inactivated FBS, 100 U/ml of penicillin, and 100 μg/ml of streptomycin at 37°C in a humidified incubator with 5% CO2.
Preparation of RADVAXFluA and FIV
Harvested CA0409 (1.5 × 106 TCID50/ml) were irradiated using a 60Co-gamma irradiator (point source AECL, IR-79, MDS Nordion International Co., Ottawa, ON, Canada) at the Advanced Radiation Technology Institute of Korea Atomic Energy Research Institute (Jeongeup, Korea) with absorbed dose of 6.25, 12.5, or 25 kGy for 1 h at room temperature (RT). CA0409 (1.5 × 106 TCID50/ml) were incubated with 0.2% (v/v) formalin (JUNSEI, Tokyo, Japan) under mild agitation at 4°C for a week. Then, the formalin was removed by dialysis using normal saline as previously described.Citation20
HA activity and hemagglutination inhibition (HI) assay
To examine the HA activity of influenza virus, live and inactivated CA0409 were serially diluted two-fold in 96-well U-bottom microplates (SPL, Pocheon, Korea), followed by incubation with 0.5% chicken red blood cells (cRBC; Lonza Bioscience, Basel, Switzerland) for 30 min at RT. To determine the serum HI antibody titers, serum samples were first pretreated with receptor destroying enzyme (Denka Seiken, Tokyo, Japan) for 16 h at 37°C and then heat-inactivated for 30 min at 56°C. Two-fold serial diluted serum were incubated with 8 HA units of CA0409 for 2 h at RT, followed by incubation with 0.5% cRBC for 30 min at RT. HI antibody titers were defined as the highest dilution of each serum that inhibited virus-mediated hemagglutination.
NA activity and neuraminidase inhibition (NI) assay
NA enzymatic activities of live and inactivated CA0409 were measured using a commercial NA activity assay kit (Sigma-Aldrich). Briefly, 20 µl of virus dilutions and 80 µl of reaction mix (assay buffer, substrate, cofactors, enzyme, and dye reagent) were mixed in each well. After 50 min incubation at 37°C, the absorbance was measured at 570 nm using Victor X3 light plate reader (Perkin-Elmer, Waltham, MA, USA). The unit activity of NA was calculated following the manufacturer’s instruction. To determine the serum NI antibody activity against the CA0409, serum samples were heat-inactivated for 30 min at 56°C and mixed with CA0409 for 2 h at RT. The activity of NI antibody was expressed as fold changes.
Confocal laser scanning microscopy
A549 cells (3 × 105 cells/ml) were plated on glass-bottom dishes (Ibidi GmbH, Martinsried, Germany) for 24 h at 37°C and incubated with indicated dose of live or inactivated CA0409 for 6 h. The cells were washed thrice with phosphate buffered saline (PBS), followed by fixing with 4% paraformaldehyde and permeabilization with 0.3% Triton X-100 in PBS. After blocking with 2% bovine serum albumin (BSA) in 0.3% Triton X-100 in PBS, the cells were stained with anti-influenza A virus nucleoprotein rabbit IgG (Abcam) for 2 h. After washing with PBS, the cells were stained with FITC-conjugated anti-rabbit IgG antibody for 1 h. The cells were washed with PBS and incubated with DAPI for 5 min. The images were visualized using LSM800 confocal laser scanning microscopy (Zeiss, Jena, Germany)
Transmission electronic microscopy (TEM)
Live CA0409, RADVAXFluA, and FIV were absorbed into 3-mm formvar amorphous carbon-coated copper grids for 2 min. Excess solution was removed by blotting. Samples were negatively stained with 2% uranyl acetate in water for 2 min, then blotted and left to dry at RT for 10 min before visualization using JEM-1400 Flash transmission electron microscope (JEOL, Tokyo, Japan).
Immunization and viral challenge
All animal experiments were conducted under the approval of the Committee on The Use and Care of Animals at Korea Atomic Energy Research Institute (KAERI-IACUC-2018-009) and performed according to accepted veterinary standards. 7-week-old female BALB/c mice were purchased from Orient Bio (Seongnam, Korea). Mice were vaccinated twice at 7 days interval intranasally with 30 µl of RADVAXFluA or FIV in PBS. Two weeks after the last vaccination, blood and spleen were collected to measure CA0409-specific antibodies and T cell responses. To measure the protective effects, vaccinated mice were challenged intranasally with CA0409, and mice survival and body weight were monitored and recorded for 10 days daily. Mice were sacrificed if they lost 20% of the starting body weight.
Measurement of influenza A virus-specific antibody
Mice blood and bronchoalveolar lavage fluid (BALF) were obtained at 2 weeks after the last vaccination. Serum was isolated from blood samples using centrifugation. BALF was obtained by inserting an intravenous catheter into trachea and repeating injection and retrieval with 400 µl PBS. To examine the levels of CA0409-specific IgG, IgA, IgG2a, and IgG1, CA0409 (1.5 × 104 TCID50/ml) were immobilized on 96-well plates for 16 h at 4°C, followed by blocking with 1% BSA in PBS. After washing thrice with PBS with 0.05% Tween 20 (PBS-T; Sigma-Aldrich), serially diluted mice serum was added onto each well. After 1 h incubation at RT, the plates were washed thrice with PBS-T to remove unbound antibodies, and the bound antibodies were detected using rabbit anti-mouse IgG, IgA, IgG1, and IgG2a-specific antibodies conjugated with HRP (horseradish peroxidase).
Ex vivo experiments using mouse bone marrow-derived dendritic cells (BMDC)
BMDC were prepared from 7-week old mice as described previously.Citation21 Bone marrow cells were isolated by flushing tibiae and femora with PBS. Red blood cells were removed using red blood cell lysis buffer. The bone marrow-derived cells were differentiated into immature BMDC with RPMI-1640 supplemented with 10% FBS, 100 U/ml of penicillin and 100 μg/ml of streptomycin in the presence of 20 ng/ml of GM-CSF for 7 days. Immature BMDC (5 × 105 cells/ml) were stimulated with 5 × 104 TCID50/ml of RADVAXFluA or FIV in the presence of GM-CSF (10 ng/ml) for 24 h. The cells were then harvested and stained with fluorochrome-conjugated monoclonal antibodies specific for CD80, CD86, or MHC-II for 30 min on ice. The geometric mean fluorescence intensity (MFI) of each group was determined using MACSQuant flow cytometry (Miltenyi Biotec, Bergisch Gladbach, Germany).
Co-culture of BMDC with T cells
CD4+ and CD8+ T cells were isolated from splenocytes of 7-week-old female mice by negative selection using MACS microbead cell separation kits according to the manufacturer’s instructions (Miltenyi Biotec). Briefly, total spleen cells were incubated with biotin-conjugated antibody cocktail against non-CD4+ T cells or non-CD8+ T cells. Then the cells were labeled with anti-biotin microbeads, followed by depletion of the magnetically labeled cells using the column in the magnetic field of a MACS separator. The unlabeled target cells in flow-through, representing the enriched CD4+ or CD8+ T cells, were harvested. The purity of CD4+ and CD8+ T cells was greater than 90%, as evaluated by flow cytometry analysis (data not shown). Immature BMDC (5 × 105 cells/ml) were stimulated with 5 × 104 TCID50/ml of RADVAXFluA or FIV in the presence of GM-CSF (10 ng/ml) and β-mercaptoethanol (50 μM) for 16 h. RADVAXFluA- or FIV-primed BMDC were co-cultured with isolated CD4+ T cells (5 × 105 cells/ml) or CD8+ T cells (5 × 105 cells/ml) for 4 days. The cells were then stained with anti-mouse CD4+ or anti-mouse CD8+ and anti-mouse IFN-γ antibodies, followed by analysis using MACSQuant flow cytometry (Miltenyi Biotec).
Statistics analysis
The mean value ± standard deviation (S.D.) was obtained from triplicate samples for each treatment group. Statistical significance was determined by one-way ANOVA and Tukey posttest. Survival was analyzed using Kaplan-Meier analysis, and significant difference was determined using the log rank test in GraphPad Prism (GraphPad Software, La Jolla, CA, USA). Asterisk (*) indicates the treatment groups that were significantly different from the control group at P < .05.
Results
Production of inactivated influenza A whole-cell vaccine using gamma-irradiation
As described previously, we generated gamma radiation-inactivatedCitation18 or formalin-treated influenza A virus vaccine.Citation20 Influenza A virus (A/California/04/2009 H1N1; CA0409) were harvested and irradiated with increased doses of gamma rays (6.25, 12.5, or 25 kGy) or incubated with 0.2% (v/v) formalin (FIV) for a week, and the loss of infectivity and viral replication was determined by plaque assay in MDCK cells. Following exposure to the gamma radiation, virus titer was significantly decreased at 6.25 kGy treatment and completely lost at 12.5 kGy and 25 kGy treatment (). The complete viral inactivation was also observed in FIV. Although chemical treatment with 0.2% formalin has been effectively used to inactivate influenza virus, it causes severe antigenic alteration on the viral protein antigens, leading to limited immune responses against viral antigens. To determine if viral structure was altered during gamma-irradiation, the morphological change of inactivated viruses was visualized using TEM. As shown in , FIV showed structural alteration and tended to aggregate. Morphological change was also observed in the virus treated with 25 kGy gamma ray but much lesser than FIV (), whereas both 6.25 and 12.5 kGy-treated viruses maintained intact viral structures. Based on these results, the treatment dose of gamma ray for production of RADVAXFluA was selected as 12.5 kGy, a radiation dose of which completely inactivates virus but does not affect viral morphology, for the subsequent experiments.
Figure 1. Inactivation of influenza virus using gamma-irradiation. (a, b) CA0409 (1.5 × 106 TCID50/ml) were exposed to 6.25, 12.5, or 25 kGy of gamma-irradiation at room temperature, or incubated with 0.2% (v/v) formalin at 4°C, followed by plaque assay to determine the infectivity of inactivated CA0409 (a) or transmission electron microscopy (TEM) analysis to observe morphological changes of virus (b). Data expressed in bar graph are the mean values ± SD of triplicate samples. *P < .05 compared to the non-treated group. ND, not detected
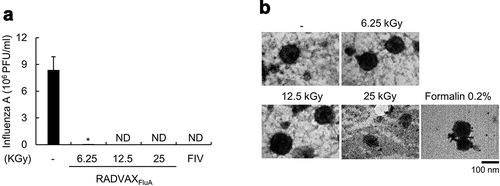
NA and HA activities of RADVAXFluA
One of the major weakness of chemically inactivated conventional influenza vaccine is a diminished immunogenicity due to significant alterations on the viral protein antigens.Citation22,Citation23 Although RADVAXFluA made by treated with 12.5 kGy gamma ray maintained normal viral structure, it was not sure if enzymatic activities of viral protein antigens were not affected. To determine the effect of gamma irradiation-induced viral inactivation on the enzymatic activities of viral protein antigens, the enzymatic activities of the two representative surface protein antigens of influenza A virus, NA and HA, were measured from RADVAXFluA and compared with those of FIV. As shown in , the potency of NA activity was not affected in RADVAXFluA, but FIV showed great reduction on NA activity compared to that of RADVAXFluA (P = .00003) and untreated virus (P = .002) (). Although HA activity was diminished by gamma-irradiation in RADVAXFluA (53.3 ± 10.6 units), it was significantly higher than that of FIV (16 units) (). As both proteins, NA and HA, are important for the attachment and entry of influenza A virus into host cells, viral attachment of CA0409, RADVAXFluA, and FIV to human lung type II epithelial cells (A549 cells) was measured in vitro. A549 cells were incubated with live CA0409, RADVAXFluA, or FIV for 6 h and influenza virus was visualized using FITC-conjugated anti-influenza A nucleoprotein (NP) antibodies and confocal microscopy. As shown in , the fluorescent signals, which indicate the level of viral infection, were detected in A549 cells infected with 1 × 104 TCID50/ml and 5 × 104 TCID50/ml of live CA0409. Although it was much lower than live CA0409, RADVAXFluA treated cells showed significant extent of fluorescent signal, but no fluorescent signal was detected in FIV-treated cells (). These findings suggest that RADVAXFluA made by the treatment at 12.5 kGy retains functional activities of viral proteins, HA and NA, by considering the loss of viral replication activity.
Figure 2. Effect of gamma-irradiation on neuraminidase (NA) and hemagglutinin (HA) activities. (a, b) CA0409 (1.5 × 106 TCID50/ml) were exposed to 12.5kGy gamma-irradiation at room temperature, or incubated with 0.2% (v/v) formalin at 4°C, followed by NA (a) and HA enzymatic assays (b). Data are the mean values ± SD of triplicate samples. *P < .05 compared to FIV-treated group. (c) A549 cells (3 × 105 cells/ml) were infected with live CA0409 (1 × 104 or 5 × 104 TCID50/ml), RADVAXFluA (5 × 104 TCID50/ml), or FIV (5 × 104 TCID50/ml) for 6 h and virus attached to A549 cells were visualized using confocal laser scanning microscope (CLSM), with DAPI channel detecting cell nuclei, and FITC channel detecting CA0409. Scale bar = 50 μm
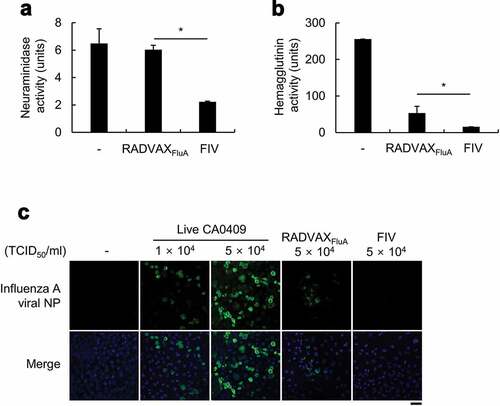
Humoral and mucosal immune responses induced by RADVAXFluA vaccination
The neutralization of viral antigens by mucosal surface IgA plays a critical role for protecting infection and invasion of respiratory pathogens including influenza virus at the early stage of infection.Citation24,Citation25 However, following the invasion of virus into the circulation, IgG1 acts as a major neutralizing antibody in serum.Citation25,Citation26 Therefore, both mucosal and systemic humoral immune responses are required for the effective protection against influenza virus infection. To evaluate the immunization effects of RADVAXFluA and FIV, mice were intranasally immunized twice with RADVAXFluA or FIV on days 0 and 7. Two weeks after the last immunization, the mice serum were harvested and the levels of CA0409-specific IgG were measured. As shown in , immunization with FIV induced CA0409-specific IgG production, but the levels of serum IgG of RADVAXFluA immunization group were greatly higher than those of FIV immunization group. Since different subclasses of IgG show different immunological functions against infections, the levels of IgG subtypes, IgG2a and IgG1, were measured. Although FIV induced antigen-specific IgG1 and IgG2a titers, they were 2 log2 and 4 log2 lower than those induced by RADVAXFluA, respectively, indicating that the ability of FIV to induce IgG1 production is remarkably weaker compared to the ability to induce IgG2a (). Next, to determine the functional activities of CA0409-specific antibodies produced by RADVAXFluA and FIV immunization, HI titers and NA inhibition rates of serum antibodies were determined. When tested with 8 HA units of CA0409, no HI titers were detected in the sera isolated from FIV-immunized mice, whereas more than 4.7 ± 0.2 log2 HI titers were detected in those from RADVAXFluA-immunized mice (). Furthermore, RADVAXFluA-immunized serum exhibited fourfold higher inhibition rate against NA activity of CA0409 than FIV-immunized serum (). To investigate whether RADVAXFluA immunization induces effective mucosal immune responses, CA0409-specific IgA levels were measured from the BALF of mice immunized with RADVAXFluA or FIV. As shown in , intranasal immunization of RADVAXFluA induced significantly higher CA0409-specific mucosal IgA titers than FIV immunization. Furthermore, the neutralization assay of BALF from RADVAXFluA-immunized mice showed an efficient inhibition effect against CA0409 infection in A549 cells, which was greatly higher than that of FIV-immunized mice (). These results show that RADVAXFluA immunization is superior to FIV immunization for the induction of humoral influenza-specific IgG antibody, especially for the production of neutralizing IgG1 subclass, and for the induction of mucosal IgA antibody, both of which contribute to the enhanced neutralizing effects against CA0409 compared to FIV.
Figure 3. Humoral and mucosal immune responses by RADVAXFluA. (a-c) Mice (n = 5 per group) were intranasally immunized twice with 5 × 104 TCID50 of RADVAXFluA or FIV on days 0 and 7, and the levels of CA0409-specific serum IgG (a), serum IgG2a (b), and serum IgG1 (c) were analyzed 2 weeks after last immunization using immunoassay. The end-point titers were determined by optical density cutoff values of 0.1. (d, e) Mice (n = 5 per group) were intranasally immunized with 1.5 × 104 TCID50 of RADVAXFluA or FIV for HA inhibition (HI) antibody titration (d), or immunized with 5 × 104 TCID50 of inactivated virus for NA inhibition (NI) assay (e) on days 0 and 7, and sera harvested at 2 weeks after the last immunization were used for HI titration and NI assay against CA0409. (F, G) Mice (n = 5 per group) were intranasally immunized twice with 5 × 104 TCID50 of RADVAXFluA or FIV on days 0 and 7, and the BALF was harvested 2 weeks after last immunization. (F) The level of CA0409-specific IgA was analyzed in the harvested BALF. (G) A549 cells (3 × 105 cells/ml) were infected with live CA0409 (5 × 104 TCID50/ml) treated with the BALF at 1:32 dilution for 6 h, and virus attached to the cells were visualized using CLSM, with DAPI channel detecting cell nuclei and FITC channel detecting CA0409. Scale bar = 50 μm. Data are representative of three independent experiments and shown as the means ± S.D. *P < .05 compared to the FIV-immunized group. #P < .05 compared to the unimmunized group

CD8+ T cell activation by RADVAXFluA-sensitized dendritic cells
Dendritic cells (DCs) are professional antigen presenting cells linking innate and adaptive immune responses, and its vaccine-induced maturation plays a profound role in T cell activation and polarization. To understand the molecular mechanisms underlying different features of RADVAXFluA and FIV on the activation of cellular immunity, direct effects of RADVAXFluA and FIV on the activation of DCs were investigated. Mouse bone marrow-derived DC (BMDC) were differentiated by GM-CSF and stimulated with 3 × 104 TCID50/ml of RADVAXFluA or FIV for 24 h, followed by assessment of the expression levels of phenotypic maturation markers on BMDC, such as CD80, CD86, and MHC-II. As shown in , the expression levels of all three DC maturation makers were significantly enhanced by RADVAXFluA, whereas in FIV-stimulated DCs a significant increase was found only in MHC-II, although it was significantly lower than that of RADVAXFluA (). Since we found enhanced expression of CD80 and CD86, both of which play critical roles for DC-induced activation of T cells, during RADVAXFluA-induced DC maturation, we, next, decided to determine whether RADVAXFluA- or FIV-induced DC maturation would have a different effect on T cell activation by measuring intracellular IFN-γ production, a key cytokine involved in host defense and primarily produced by activated CD4+ and CD8+ T cells. The co-culture system of BMDCs and T cells in vitro is a classic and proven method to assess the ability of antigen-primed DCs to regulate kinetics, magnitude, and property of T cells.Citation27,Citation28 To access if the differential priming effect of vaccines on DCs affects the T cell activation property of DCs, BMDCs were stimulated with 3 × 104 TCID50/ml of RADVAXFluA or FIV for 16 h, followed by co-culturing with isolated CD4+ or CD8+ T cells from mice splenocytes for 4 days. When CD4+ or CD8+ T cells were co-cultured with unprimed BMDCs, no significant increase in IFN-γ positive cell number was observed. However, both RADVAXFluA- or FIV-sensitized BMDCs significantly induced the IFN-γ producing CD4+ T cell population, but no significant difference was observed between RADVAXFluA- and FIV-sensitized groups (). Surprisingly, FIV-sensitized BMDCs showed a weak induction of IFN-γ producing CD8+ T cells, which was not significantly different from those produced by unprimed BMDCs. However, RADVAXFluA-sensitized BMDCs efficiently activated IFN-γ producing CD8+ T cells ().
Figure 4. CD8+ T cell activation via BMDC sensitized with RADVAXFluA. (a) BMDC (5 × 105 cells/ml) were sensitized with 3 × 104 TCID50/ml of RADVAXFluA or FIV for 24 h, followed by analysis of the expression of the phenotypic maturation markers of CD80, CD86, and MHC-II using flow cytometry. (b, c) BMDCs primed with RADVAXFluA or FIV were co-incubated with 5 × 105 cells/ml of isolated mouse splenocytic CD4+ T cells or CD8+ T cells for 4 days to analyze the DC-induced activation of CD4+ IFN-γ+ cells (b) and CD8+ IFN-γ+ cells (c) by flow cytometry. Data are representative of three independent experiments and shown as the means ± S.D. *P < .05 compared to the FIV-stimulated group. (d) Mice (n = 5 per group) were intranasally immunized twice with 5 × 104 TCID50 of RADVAXFluA or FIV on days 0 and 7. Splenocytes isolated from the RADVAXFluA- or FIV-immunized mice were stimulated with 3 × 104 TCID50/ml of RADVAXFluA or FIV, respectively, for 6 h in the presence of 2 μg/ml of brefeldin A, followed by analysis of CD3+ CD8+ IFN-γ+ cells in splenocytes pooled from a group of 5 mice using flow cytometry. The numbers on the gated cells indicate the percentages of the cells
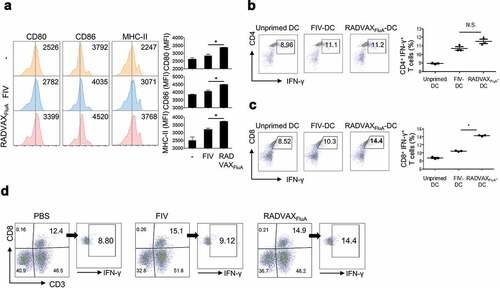
Next, we investigated whether enhanced activation of CD8+ T cells is also induced by RADVAXFluA immunization in vivo. Mice were intranasally immunized with RADVAXFluA or FIV on days 0 and 7. Two weeks after the last immunization, splenocytes were isolated from the RADVAXFluA- or FIV-immunized mice and restimulated with RADVAXFluA or FIV, respectively, for 6 h in the presence of brefeldin A. Then the cells were labeled with fluorescent-conjugated anti-CD3, anti-CD8, and anti-IFN-γ antibodies, followed by assessment of activated CD8+ T cell population (CD3+, CD8+, IFN-γ+) using flow cytometry. The population of CD8+ T cells was similarly increased in both RADVAXFluA- and FIV-immunized mice, but the percentage of IFN-γ producing CD8+ T cells was more significantly increased by immunization of RADVAXFluA than that of FIV (). These results indicate that RADVAXFluA is superior to induce DC maturation and subsequent activation of CD8+ T cells than FIV.
Protective vaccine efficacy of RADVAXFluA
Next, to assess the protective efficacy of vaccination, mice were immunized with RADVAXFluA or FIV, followed by challenge with a lethal dose of CA0409 (1.5 × 104 TCID50). All the mock (PBS)-immunized mice exhibited rapid weight loss, and 100% of death was observed within 8 days post-challenge (). FIV-immunized mice group also showed drastic weight loss and death of two mice following detrimental challenge with CA0409, and the remaining mice were recovered from some weight loss (~10%) within 10 days (). However, mice immunized with RADVAXFluA provided 100% protection against the lethal challenge of CA0409 with no significant loss in body weight (). These results demonstrate that RADVAXFluA immunization provides robust protective immunity against influenza A virus infection compared to FIV.
Figure 5. Protective efficacy of RADVAXFluA against CA0409 infection. (a-c) Mice were intranasally immunized twice with RADVAXFluA (n = 7, 5 × 104 TCID50), FIV (n = 6, 5 × 104 TCID50), or PBS (n = 6), followed by challenging with CA0409 (1.5 × 104 TCID50) 2 weeks after the final immunization. Body weight changes of (a) PBS-, (b) FIV-, and (c) RADVAXFluA-immunized mice were observed and recorded for 14 days. (d) Mice survival rates were monitored daily for up to 10 days post-infection. Mice were euthanized if they lost 20% of starting body weight. *P < .05 compared to the FIV-vaccinated group
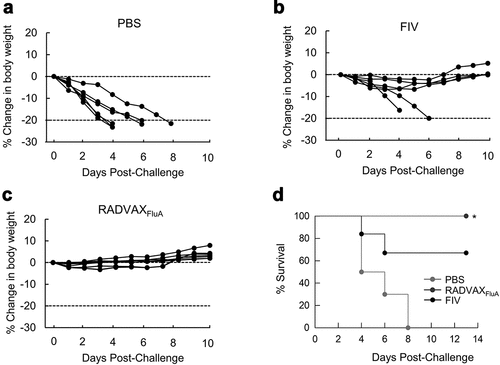
Discussion
After chemical inactivation using formalin or BPL, virus vaccines are manufactured in various forms, including whole inactivated influenza vaccine (WIV), split vaccines, or nucleocapsid removing subunit vaccines. Although WIV has more potential immunogenicity than others, split and subunit influenza vaccines are commonly used in the market as they are associated less with several adverse immune responses, which might be caused by unexpected antigen damage during chemical inactivation. In addition, several limitations are associated with conventional chemical inactivated vaccines, such as long inactivation time and difficulties in removal of chemicals used for inactivation. Therefore, new influenza virus inactivation methods have been intensively studied to overcome the drawbacks of conventional inactivated vaccines. Gamma-irradiation has been used extensively as an alternative inactivation method,Citation29,Citation30 because it has high penetrability, which allows viral inactivation in large volumes within a short time via damage of nucleic acid (usually less than 2 h).
Several previous reports have suggested that nucleic acids rather than proteins are the primary targets of gamma-irradiation to inactivate microorganisms. For example, Venezuelan equine encephalitis virus (VEEV) vaccine, produced after inactivation with 50 kGy gamma-irradiation, still demonstrated 32–50% retention rate of surface epitope integrity, which was sufficient to provide significant protection against a lethal VEEV challenge in a mouse model.Citation31 Furthermore, Miekka, et al.Citation32 suggested gamma-irradiation as a method of sterilizing protein biotherapeutics by demonstrating that 20 to 50 kGy gamma radiation does not affect the structural integrity of plasma-derived biotherapeutic protein, such as human albumin, and perfectly inactivates viral contaminants such as porcine parvovirus and encephalomyocarditis virus. Furuya, et al.Citation33 showed that inactivated influenza virus with 10 kGy gamma-irradiation conferred significant protection in mice than formalin- or UV-inactivated influenza virus. These indicated that gamma-irradiation efficiently inactivates viruses with less impact on their antigenic structure, leading to accurate and robust immune response. In contrast, formalin has been known to induce the formation of methylene bridges between amino groups, resulting in protein cross-linking.Citation34 For example, mass spectrometric analysis revealed covalent modification of more than 60% of HA epitope peptides after formalin treatment.Citation35 In addition, binding ability of anti-flavivirus envelope glycoprotein (E protein) monoclonal antibody to Japanese encephalitis virus (JEV) inactivated by formalin treatment was significantly diminished compared to binding to live JEV because of the antigenic modification of the epitope region of E protein after formalin treatment.Citation36 As we found in this study, gamma-irradiated influenza A virus vaccine (RADVAXFluA) might cause lesser damage or structural transformation of influenza surface protein antigens, HA and NA, than the conventional chemical inactivated influenza A virus, which is required to induce effective humoral and cell-mediated immune responses against natural influenza infections. Since HA and NA are the most important antigens for infectivity, antigenicity, and host specificity of influenza virus, the induction and neutralizing effect of the antibodies against HA and NA are critical factors to determine vaccine efficacy and licensing.Citation22,Citation37 As we have shown in functional assay of HA and NA, RADVAXFluA retained functionally effective HA and NA activities, which resulted in the induction of antibodies effectively neutralizing the activities of natural HA and NA of live virus. These evidences suggest that gamma-irradiation is less likely to alter antigenic structure than formalin treatment, which might affect the superior neutralizing effect of antibodies induced by vaccination with RAVAXFluA.
For the effective protection against respiratory pathogens, such as influenza virus, mucosal surface defense, especially initial neutralization of antigens, is important as a first line of defense to prevent an invasion of microbial pathogens into cells and circulation.Citation24,Citation25 However, currently available conventional influenza vaccines have limitations to induce effective mucosal immune responses, such as mucosal IgA antibody.Citation38,Citation39 Although cold-adapted live vaccines are developed and used to overcome this limitation, it also has a great limitation due to a nature of its infectivity. By considering the importance of mucosal immune defense against influenza infections, the development of alternative influenza vaccines has been requested to fulfill the demand of an effective influenza-specific neutralizing IgA antibody production. When mice were intranasally immunized with RADVAXFluA, the level of influenza-specific IgA antibodies was highly upregulated, especially compared to that of FIV, and live CA0409 virus infection into airway epithelial cells was effectively blocked by BALF from RADVAXFluA–immunized mice. Although FIV was also intranasally administered, RADVAXFluA was superior to FIV for both mucosal IgA production and neutralizing against live CD0409 virus. The enhanced protective mucosal immune responses observed in RADVAXFluA might be correlated with its improved retention of antigenic structures and suggest the conservation of antigenic properties. In addition to the mucosal IgA, serum level of pathogen-specific IgG is frequently used to evaluate the vaccine efficacy against specific pathogens following immunization. In more recent studies, it has been suggested that not only the total amount of IgG, but also expression levels of specific IgG subclasses contribute to the protection against infections.Citation25,Citation40 The immunological features of IgG subclasses show quite different effector functions, as IgG1, one of the two most effectively induced IgG subclasses by infections in mice, is not able to activate complement or does not activate Fc receptor (FcR)-mediated immune responses due to low affinity of Fc of IgG1 to activatory FcR.Citation26,Citation41 However, IgG1 is superior to other IgG subclasses with respect to higher inhibitory activities against HA and higher viral neutralizing effect, and thereby acts as a major neutralizing antibody among IgG subclasses.Citation26,Citation42 Unlike IgG1 antibody, an increase in IgG2a level was known to be not associated with viral neutralization, but rather associated with the clearance activities, mainly through the activation of complement and FcR-mediated activation of immune cells including natural killer cells, macrophages, and neutrophil.Citation41,Citation43 As the neutralization of viral antigens and particles have limited capacity to protect against infection, the effective clearance mechanism of viral particles and virus-infected cells, which is mainly activated by antigen-bound IgG2a, is also required for the complete resolution from infections. In this study, RADVAXFluA immunization enhanced serum IgG production as compared to FIV immunization, particularly IgG1 titers. These results may explain significant neutralizing effects of RADVAXFluA-immunized serum against HA and NA, but no inhibition by FIV-immunized serum. Moreover, it has been known that IgG1 is the most resistant IgG subclass to the degradation by proteases, which would enhance its neutralizing effect when it is secreted onto mucosal surface.Citation44 In addition to the higher level of IgG1, IgG2a was also greatly enhanced in RADVAXFluA-immunized serum than FIV, which may critically contribute to the resolution of influenza infection in vivo by effectively activating the viral clearance mechanisms, such as complement activation, antibody-dependent cell cytotoxicity, and antibody-dependent activation of neutrophils and macrophages.
Current influenza vaccines have been reported to elicit only 30–40% protective effect among the elderly because of limited boosting effect on antibody response and negligible cell-mediated immune response.Citation45–48 This concern has shifted the focus to CD8+ T cell response, which plays a key role in protection and clearance of intracellular pathogens including influenza virus for better protective efficacy of the vaccine.Citation49 Previous reports indicated that vaccination with irradiated Listeria monocytogenes and B. melitensis increased IFN-γ secreting CD8+ T cells, providing protection effect against pathogenic infection.Citation16,Citation50 Moreover, gamma radiation-inactivated influenza virus was found to effectively activate CD8+ T cells, which is also required for the cross-protection against heterologous influenza strains.Citation18,Citation33 However, the underlying molecular mechanisms by which inactivated vaccine effectively induces cytotoxic T cells, which is one of the major limitation of inactivated vaccines, has not been clearly understood yet. Following the direct stimulation with RADVAXFluA, the expression of DC maturation markers, such as CD80, CD86, and MHC-II, were significantly increased, which implies stronger activation and maturation of DCs by RADVAXFluA. Especially, the expression of CD80 and CD86, both of which play critical roles on the activation of T cells, was significantly enhanced in RADVAXFluA–primed DCs compared to no significant induction in FIV-primed DCs. Interestingly, the activation of CD4+ T cells by RADVAXFluA–primed DC and FIV-primed DC were not significantly differed, but RADVAXFluA-primed DC was superior to the activation of CD8+ T cells, and RADVAXFluA vaccination also potently induced IFN-γ producing CD8+ T cells in vivo. This phenomenon can be explained by various hypotheses. Indeed, DC-SIGN, a C-type lectin present on the DC surface, is involved in attachment and endocytosis of influenza A virus via interaction with HA, which enhances viral antigen cross-presentation on MHC-I.Citation51,Citation52 Thus, it is not surprising that RAVAXFluA-primed DC, which contains relatively high intact HA, can activate CD8+ T cells more than FIV-primed DC. In influenza virus, other conserved proteins such as nucleoprotein (NP), polymerase basic protein (PB1 and PB2), and matrix protein (M1 and M2) have been reported to provide epitopes to reactive CD8+ T cells. Gotch, et al.Citation53 showed that vaccination with Vaccinia virus expressing influenza NP, PB2, and M1 can recognize and lyse influenza-infected cells via the activation of influenza-specific CD8+ T cells. Furthermore, another study used mass spectrometry to demonstrate that NP- and PB1-derived peptides are consistently presented on MHC-I of HeLa cells infected with influenza A virus.Citation54 Thus, we can hypothesize that the preserved antigenic structure of conserved proteins in RADVAXFluA might induce more efficient cell-mediated immune response than FIV. In addition, since there was no difference on the CD4+ T cell activation between RADVAXFluA- and FIV-primed DCs, enhanced CD8+ T cell activation by RADVAXFluA-primed DCs might be independent from CD4+ T cells and explained by stronger maturation of DCs as strongly activated DCs are able to activate CD8+ T cells without the co-stimulatory effect of CD4+ T cells.
WHO reviews the world epidemiological situation twice annually and recommends new vaccine strains based on the available evidence for development of seasonal influenza vaccines.Citation55,Citation56 However, the difficulty in predicting circulating strains has frequently resulted in mismatch, leading to vaccine failure. These issues have prompted the need for universal influenza vaccine development. Previous reports demonstrated that the activation of CD8+ T cells against influenza virus is a crucial mechanism to exhibit cross-protective ability of influenza vaccine.Citation57 Mullbacher, et al.Citation58 showed that the activation of CD8+ T cells by gamma-irradiated influenza A virus is essential for providing cross-protective efficacy against lethal challenge of heterologous influenza A virus. NP and M2e, the conserved proteins of influenza virus, that provide epitopes to CD8+ T cells, have been used to develop universal vaccine in pre-clinical and clinical studies.Citation59 In addition, NP DNA vaccine and virus-like particle (VLP) expressing high levels of M2 induce high levels of influenza-specific CD8+ T cell response, which provides cross-protection irrespective of influenza virus subtypes in mice.Citation60–62 These studies support our hypothesis that the conserved proteins that are relatively intact in influenza A virus inactivated by gamma-irradiation are likely to act as universal antigenic targets of the cross-protective CD8+ T cells, although further supporting studies are required to clarify the precise mechanisms.
Understanding the vaccine antigens processing pathway at the cellular level is crucial to establish the precise mechanisms by which protective immunity conferred by the vaccine. Although we found that the fluorescence signals of RADVAXFluA were relatively higher on human lung epithelial cells in vitro than those of FIV in this study, it may only mean the binding of RADVAXFluA to the cells and does not ensure the meaning of cytosolic pathway associated with the antigen entry or processing. To better understand the action mechanism and superior efficacy of RADVAXFluA, further studies to clarify immunological process of the vaccine including conformational change of HA, endosomal membrane fusion, cytosolic release of antigens, and antigens presentation, are required.
In conclusion, this study demonstrates that HA and NA activities were significantly retained in RADVAXFluA, which showed superior induction of humoral and cell-mediated immunity compared to FIV. Therefore, gamma-irradiated viruses could provide potent immunogenicity and high protective effect against lethal challenge of influenza A virus. Notably, by considering outstanding features of gamma radiation-inactivated influenza virus as universal influenza vaccines, our observations on the unique immunological characteristics may be used to develop vaccines that can prevent influenza virus infections.
Disclosure of potential conflicts of interest
No potential conflicts of interest were disclosed.
Additional information
Funding
References
- Fischer WA 2nd, Gong M, Bhagwanjee S, Sevransky J. Global burden of influenza as a cause of cardiopulmonary morbidity and mortality. Glob Heart. 2014;9:325–36. doi:10.1016/j.gheart.2014.08.004.
- de Boer PT, Kelso JK, Halder N, Nguyen TP, Moyes J, Cohen C, Barr IG, Postma MJ, Milne GJ. The cost-effectiveness of trivalent and quadrivalent influenza vaccination in communities in South Africa, Vietnam and Australia. Vaccine. 2018;36:997–1007. doi:10.1016/j.vaccine.2017.12.073.
- Carrillo-Santisteve P, Ciancio BC, Nicoll A, Lopalco PL. The importance of influenza prevention for public health. Hum Vaccin Immunother. 2012;8:89–95. doi:10.4161/hv.8.1.19066.
- Krammer F. Novel universal influenza virus vaccine approaches. Curr Opin Virol. 2016;17:95–103. doi:10.1016/j.coviro.2016.02.002.
- Kon TC, Onu A, Berbecila L, Lupulescu E, Ghiorgisor A, Kersten GF, Cui YQ, Amorij JP, Van der Pol L. Influenza vaccine manufacturing: effect of inactivation, splitting and site of manufacturing. Comparison of influenza vaccine production processes. PLoS One. 2016;11:e0150700. doi:10.1371/journal.pone.0150700.
- Barry DW, Staton E, Mayner RE. Inactivated influenza vaccine efficacy: diminished antigenicity of split-product vaccines in mice. Infect Immun. 1974;10:1329–36.
- Barry DW, Mayner RE, Staton E, Dunlap RC, Rastogi SC, Hannah JE, Blackburn RJ, Nortman DF, Graze PR. Comparative trial of influenza vaccines. I. Immunogenicity of whole virus and split product vaccines in man. Am J Epidemiol. 1976;104:34–46.
- Ortbals DW, Liebhaber H. Comparison of immunogenicity of a whole virion and a subunit influenza vaccine in adults. J Clin Microbiol. 1978;8:431–34.
- Moghaddam A, Olszewska W, Wang B, Tregoning JS, Helson R, Sattentau QJ, Openshaw PJ. A potential molecular mechanism for hypersensitivity caused by formalin-inactivated vaccines. Nat Med. 2006;12:905–07. doi:10.1038/nm1456.
- Amanna IJ, Raue HP, Slifka MK. Development of a new hydrogen peroxide-based vaccine platform. Nat Med. 2012;18:974–79. doi:10.1038/nm.2763.
- Skoble J, Beaber JW, Gao Y, Lovchik JA, Sower LE, Liu W, Luckett W, Peterson JW, Calendar R, Portnoy DA, et al. Killed but metabolically active Bacillus anthracis vaccines induce broad and protective immunity against anthrax. Infect Immun. 2009;77:1649–63. doi:10.1128/IAI.00530-08.
- Dubensky TW Jr., Skoble J, Lauer P, Brockstedt DG. Killed but metabolically active vaccines. Curr Opin Biotechnol. 2012;23:917–23. doi:10.1016/j.copbio.2012.04.005.
- Brockstedt DG, Bahjat KS, Giedlin MA, Liu W, Leong M, Luckett W, Gao Y, Schnupf P, Kapadia D, Castro G, et al. Killed but metabolically active microbes: a new vaccine paradigm for eliciting effector T-cell responses and protective immunity. Nat Med. 2005;11:853–60. doi:10.1038/nm1276.
- Seo HS. Application of radiation technology in vaccines development. Clin Exp Vaccine Res. 2015;4:145–58. doi:10.7774/cevr.2015.4.2.145.
- Choi E, Michalski CJ, Choo SH, Kim GN, Banasikowska E, Lee S, Wu K, An HY, Mills A, Schneider S, et al. First Phase I human clinical trial of a killed whole-HIV-1 vaccine: demonstration of its safety and enhancement of anti-HIV antibody responses. Retrovirology. 2016;13:82. doi:10.1186/s12977-016-0317-2.
- Magnani DM, Harms JS, Durward MA, Splitter GA. Nondividing but metabolically active gamma-irradiated Brucella melitensis is protective against virulent B. melitensis challenge in mice. Infect Immun. 2009;77:5181–89. doi:10.1128/IAI.00231-09.
- Babb R, Chen A, Ogunniyi AD, Hirst TR, Kara EE, McColl SR, Alsharifi M, Paton JC. Enhanced protective responses to a serotype-independent pneumococcal vaccine when combined with an inactivated influenza vaccine. Clin Sci (Lond). 2017;131:169–80. doi:10.1042/CS20160475.
- David SC, Lau J, Singleton EV, Babb R, Davies J, Hirst TR, McColl SR, Paton JC, Alsharifi M. The effect of gamma-irradiation conditions on the immunogenicity of whole-inactivated Influenza A virus vaccine. Vaccine. 2017;35:1071–79. doi:10.1016/j.vaccine.2016.12.044.
- Alsharifi M, Furuya Y, Bowden TR, Lobigs M, Koskinen A, Regner M, Trinidad L, Boyle DB, Mullbacher A. Intranasal flu vaccine protective against seasonal and H5N1 avian influenza infections. PLoS One. 2009;4:e5336. doi:10.1371/journal.pone.0005336.
- Takada A, Matsushita S, Ninomiya A, Kawaoka Y, Kida H. Intranasal immunization with formalin-inactivated virus vaccine induces a broad spectrum of heterosubtypic immunity against influenza A virus infection in mice. Vaccine. 2003;21:3212–18.
- Kim HY, Kim SK, Seo HS, Jeong S, Ahn KB, Yun CH, Han SH. Th17 activation by dendritic cells stimulated with gamma-irradiated Streptococcus pneumoniae. Mol Immunol. 2018;101:344–52. doi:10.1016/j.molimm.2018.07.023.
- Gomez Lorenzo MM, Fenton MJ. Immunobiology of influenza vaccines. Chest. 2013;143:502–10. doi:10.1378/chest.12-1711.
- Herrera-Rodriguez J, Signorazzi A, Holtrop M, de Vries-idema J, Huckriede A. Inactivated or damaged? Comparing the effect of inactivation methods on influenza virions to optimize vaccine production. Vaccine. 2019;37:1630–37. doi:10.1016/j.vaccine.2019.01.086.
- Gould VMW, Francis JN, Anderson KJ, Georges B, Cope AV, Tregoning JS. Nasal IgA provides protection against human influenza challenge in volunteers with low serum influenza antibody titre. Front Microbiol. 2017;8:900. doi:10.3389/fmicb.2017.00900.
- Renegar KB, Small PA Jr., Boykins LG, Wright PF. Role of IgA versus IgG in the control of influenza viral infection in the murine respiratory tract. J Immunol. 2004;173:1978–86. doi:10.4049/jimmunol.173.3.1978.
- Huber VC, McKeon RM, Brackin MN, Miller LA, Keating R, Brown SA, Makarova N, Perez DR, Macdonald GH, McCullers JA. Distinct contributions of vaccine-induced immunoglobulin G1 (IgG1) and IgG2a antibodies to protective immunity against influenza. Clin Vaccine Immunol. 2006;13:981–90. doi:10.1128/CVI.00156-06.
- Lanzavecchia A, Sallusto F. Regulation of T cell immunity by dendritic cells. Cell. 2001;106:263–66. doi:10.1016/s0092-8674(01)00455-x.
- Hancock DG, Guy TV, Shklovskaya E, Fazekas de St Groth B. Experimental models to investigate the function of dendritic cell subsets: challenges and implications. Clin Exp Immunol. 2013;171:147–54. doi:10.1111/cei.12027.
- Reitman M, Tonik EJ. Immunity to aerosol challenge in guinea pigs immunized with gamma-irradiated Venezuelan equine encephalitis vaccines. Appl Microbiol. 1971;21:688–92.
- Reitman M, Tribble HR Jr., Green L. Gamma-irradiated Venezuelan equine encephalitis vaccines. Appl Microbiol. 1970;19:763–67.
- Martin SS, Bakken RR, Lind CM, Garcia P, Jenkins E, Glass PJ, Parker MD, Hart MK, Fine DL. Comparison of the immunological responses and efficacy of gamma-irradiated V3526 vaccine formulations against subcutaneous and aerosol challenge with Venezuelan equine encephalitis virus subtype IAB. Vaccine. 2010;28:1031–40. doi:10.1016/j.vaccine.2009.10.126.
- Miekka SI, Forng RY, Rohwer RG, MacAuley C, Stafford RE, Flack SL, MacPhee M, Kent RS, Drohan WN. Inactivation of viral and prion pathogens by gamma-irradiation under conditions that maintain the integrity of human albumin. Vox Sang. 2003;84:36–44.
- Furuya Y, Regner M, Lobigs M, Koskinen A, Mullbacher A, Alsharifi M. Effect of inactivation method on the cross-protective immunity induced by whole ‘killed’ influenza A viruses and commercial vaccine preparations. J Gen Virol. 2010;91:1450–60. doi:10.1099/vir.0.018168-0.
- Thavarajah R, Mudimbaimannar VK, Elizabeth J, Rao UK, Ranganathan K. Chemical and physical basics of routine formaldehyde fixation. J Oral Maxillofac Pathol. 2012;16:400–05. doi:10.4103/0973-029X.102496.
- Lee YH, Jang YH, Byun YH, Cheong Y, Kim P, Lee YJ, Lee YJ, Sung JM, Son A, Lee HM, et al. Green tea catechin-inactivated viral vaccine platform. Front Microbiol. 2017;8:2469. doi:10.3389/fmicb.2017.02469.
- Fan YC, Chiu HC, Chen LK, Chang GJ, Chiou SS. Formalin inactivation of Japanese encephalitis virus vaccine alters the antigenicity and immunogenicity of a neutralization epitope in envelope protein domain III. PLoS Negl Trop Dis. 2015;9:e0004167. doi:10.1371/journal.pntd.0004167.
- Petrie JG, Ohmit SE, Johnson E, Truscon R, Monto AS. Persistence of antibodies to influenza hemagglutinin and neuraminidase following one or two years of influenza vaccination. J Infect Dis. 2015;212:1914–22. doi:10.1093/infdis/jiv313.
- Pilette C, Ouadrhiri Y, Godding V, Vaerman JP, Sibille Y. Lung mucosal immunity: immunoglobulin-A revisited. Eur Respir J. 2001;18:571–88. doi:10.1183/09031936.01.00228801.
- Suzuki T, Ainai A, Hasegawa H. Functional and structural characteristics of secretory IgA antibodies elicited by mucosal vaccines against influenza virus. Vaccine. 2017;35:5297–302. doi:10.1016/j.vaccine.2017.07.093.
- Tamura S, Kurata T. Defense mechanisms against influenza virus infection in the respiratory tract mucosa. Jpn J Infect Dis. 2004;57:236–47.
- Bruhns P. Properties of mouse and human IgG receptors and their contribution to disease models. Blood. 2012;119:5640–49. doi:10.1182/blood-2012-01-380121.
- Hocart MJ, Mackenzie JS, Stewart GA. The immunoglobulin G subclass responses of mice to influenza A virus: the effect of mouse strain, and the neutralizing abilities of individual protein A-purified subclass antibodies. J Gen Virol. 1989;70(Pt 9):2439–48. doi:10.1099/0022-1317-70-9-2439.
- Barnes N, Gavin AL, Tan PS, Mottram P, Koentgen F, Hogarth PM. FcgammaRI-deficient mice show multiple alterations to inflammatory and immune responses. Immunity. 2002;16:379–89.
- Parham P. On the fragmentation of monoclonal IgG1, IgG2a, and IgG2b from BALB/c mice. J Immunol. 1983;131:2895–902.
- Furuya Y. Return of inactivated whole-virus vaccine for superior efficacy. Immunol Cell Biol. 2012;90:571–78. doi:10.1038/icb.2011.70.
- Govaert TM, Thijs CT, Masurel N, Sprenger MJ, Dinant GJ, Knottnerus JA. The efficacy of influenza vaccination in elderly individuals. A randomized double-blind placebo-controlled trial. JAMA. 1994;272:1661–65.
- Brandriss MW, Betts RF, Mathur U, Douglas RG Jr. Responses of elderly subjects to monovalent A/USSR/77 (H1N1) and Trivalent A/USSR/77 (H1N1)-A/TEXAS/77 (H3N2)-B/Hong Kong/72 vaccines. Am Rev Respir Dis. 1981;124:681–84. doi:10.1164/arrd.1981.124.6.681.
- Gross PA, Quinnan GV Jr., Weksler ME, Setia U, Douglas RG Jr. Relation of chronic disease and immune response to influenza vaccine in the elderly. Vaccine. 1989;7:303–08.
- Harty JT, Bevan MJ. Responses of CD8(+) T cells to intracellular bacteria. Curr Opin Immunol. 1999;11:89–93.
- Datta SK, Okamoto S, Hayashi T, Shin SS, Mihajlov I, Fermin A, Guiney DG, Fierer J, Raz E. Vaccination with irradiated Listeria induces protective T cell immunity. Immunity. 2006;25:143–52. doi:10.1016/j.immuni.2006.05.013.
- Hillaire ML, Nieuwkoop NJ, Boon AC, de Mutsert G, Vogelzang-van Trierum SE, Fouchier RA, Osterhaus AD, Rimmelzwaan GF. Binding of DC-SIGN to the hemagglutinin of influenza A viruses supports virus replication in DC-SIGN expressing cells. PLoS One. 2013;8:e56164. doi:10.1371/journal.pone.0056164.
- van Montfoort N, van der Aa E, Woltman AM. Understanding MHC class I presentation of viral antigens by human dendritic cells as a basis for rational design of therapeutic vaccines. Front Immunol. 2014;5:182. doi:10.3389/fimmu.2014.00182.
- Gotch F, McMichael A, Smith G, Moss B. Identification of viral molecules recognized by influenza-specific human cytotoxic T lymphocytes. J Exp Med. 1987;165:408–16.
- Wahl A, Schafer F, Bardet W, Buchli R, Air GM, Hildebrand WH. HLA class I molecules consistently present internal influenza epitopes. Proc Natl Acad Sci U S A. 2009;106:540–45. doi:10.1073/pnas.0811271106.
- Kitler ME, Gavinio P, Lavanchy D. Influenza and the work of the World Health Organization. Vaccine. 2002;20:S5–14.
- Alonso WJ, Yu C, Viboud C, Richard SA, Schuck-Paim C, Simonsen L, Mello WA, Miller MA. A global map of hemispheric influenza vaccine recommendations based on local patterns of viral circulation. Sci Rep. 2015;5:17214. doi:10.1038/srep17214.
- Soema PC, van Riet E, Kersten G, Amorij JP. Development of cross-protective influenza a vaccines based on cellular responses. Front Immunol. 2015;6:237. doi:10.3389/fimmu.2015.00237.
- Mullbacher A, Ada GL, Hla RT. Gamma-irradiated influenza A virus can prime for a cross-reactive and cross-protective immune response against influenza A viruses. Immunol Cell Biol. 1988;66(Pt 2):153–57. doi:10.1038/icb.1988.19.
- Berlanda Scorza F, Tsvetnitsky V, Donnelly JJ. Universal influenza vaccines: shifting to better vaccines. Vaccine. 2016;34:2926–33. doi:10.1016/j.vaccine.2016.03.085.
- Ulmer JB, Donnelly JJ, Parker SE, Rhodes GH, Felgner PL, Dwarki VJ, Gromkowski SH, Deck RR, DeWitt CM, Friedman A, et al. Heterologous protection against influenza by injection of DNA encoding a viral protein. Science. 1993;259:1745–49.
- Ulmer JB, Fu TM, Deck RR, Friedman A, Guan L, DeWitt C, Liu X, Wang S, Liu MA, Donnelly JJ, et al. Protective CD4+ and CD8+ T cells against influenza virus induced by vaccination with nucleoprotein DNA. J Virol. 1998;72:5648–53.
- Song JM, Wang BZ, Park KM, Van Rooijen N, Quan FS, Kim MC, Jin HT, Pekosz A, Compans RW, Kang SM. Influenza virus-like particles containing M2 induce broadly cross protective immunity. PLoS One. 2011;6:e14538. doi:10.1371/journal.pone.0014538.