ABSTRACT
Cervical cancer is a leading cause of high mortality in women in developing countries and has a serious impact on women’s health. Human papilloma virus (HPV) prophylactic vaccines have been produced and may hold promise for reducing the incidence of cervical cancer. However, the limitations of current HPV vaccine strategies make the development of HPV therapeutic vaccines particularly important for the treatment of HPV related lesions. Our previous work has demonstrated that LM4Δhly::E7 was safe and effective in inducing antitumor effect by antigen-specific cellular immune responses and direct killing of tumor cell on a cervical cancer model. In this study, the codon usage effect of a novel Listeria-based cervical cancer vaccine LM4Δhly::E7-1, was evaluated for effects of codon-optimized E7 expression, cellular immune response and therapeutic efficacy in a tumor-bearing murine model. Our data demonstrated that up-regulated expression of E7 was strikingly elevated by codon usage optimization, and thus induced significantly higher Th1-biased immunity, lymphocyte proliferation, and strong specific CTL activity ex-vivo compared with LM4Δhly::E7-treated mice. Furthermore, LM4Δhly::E7-1 enhanced a remarkable therapeutic effect in establishing tumors. Taken together, our results suggest that codon usage optimization is an important consideration in constructing live bacterial-vectored vaccines and is required for promoting effective T cell responses.
1. Introduction
Cancer is the second leading cause of death globally and was responsible for 8.8 million deaths in 2015. One-fifth of cancers are caused by chronic infection, including cervical cancer caused by Human papilloma virus (HPV). In particular, HPV is responsible for nearly 100% of cervical cancer cases, which remains the fourth most common malignancy in women worldwide with an estimated 530,000 new cases annually, resulting in 270,000 deaths, and will continue to increase rapidly until at least 2060.Citation1–4 Almost 85% of cervical cancers occur in the developing world, where patients have limited access to adequate treatment. As a result, the mortality rates are high for women in these countries.Citation5
Vaccines have been traditionally used as a preventative measure against infectious diseases. Several successful prophylactic vaccines have been developed for prevention of HPV infection,Citation6 i.e., Cervarix® (GlaxoSmithKline Biologicals) and Gardasil® (Merck and Co., Inc.). However, there is no strong evidence demonstrating a therapeutic effect of prophylactic vaccines in treating and clearing established HPV infections or HPV-associated lesions. Furthermore, despite their apparent availability, access to prophylactic vaccines is limited in developing countries and so HPV infection remains extremely common worldwide. Thus, there is an urgent need to develop treatments for the control of existing HPV infection and its associated diseases. Highly efficient therapeutic measures remain crucial to treating existing HPV infections and HPV-associated lesions and cancers. Several strategies have been designed to enhance the efficacy of therapeutic vaccines, i.e. nucleic acid, protein and peptide, live vector and whole cell-based vaccines, and the results from various clinical trials have shown therapeutic potential, but no vaccines for the treatment of HPV-associated cancers have been currently available for general use in clinic.Citation7 The evaluation of current and future therapeutic approaches against HPV-induced tumors is a global health priority, despite an effective prophylactic vaccine against 7 of the 12 genotypes involved in the etiology of tumors being currently available.
Due to the immunosuppressive nature of the tumor microenvironment, which contributes to HPV pathogenesis, there is a need for T cell-based therapeutic vaccines to treat existing HPV infections and HPV-associated lesions and cancers. The facultative intracellular bacterium Listeria monocytogenes (L. monocytogenes, LM) is a promising vector that induces maturation of antigen-presenting cells and generation of potent innate immune responses.Citation8,Citation9 It also delivers antigens to both major histocompatibility complex (MHC) class I and MHC class II antigen processing pathways and induces CD8+ and CD4+ T cell responses as well as stimulating the generation of memory T cells, and inducing apoptosis of macrophages infected with LM.Citation10,Citation11 Therefore, LM as a vaccine vector to deliver foreign antigens has being intensively studied in molecular biology and immunology.Citation12–14 Indeed, attenuated LM has shown promise as a vector to deliver tumor-associated antigens (TAAs). In particular, a novel LM-vectored immunologic vaccine, ADXS11-001, has shown early promise in phase I/II/III a trials of cervical cancer.Citation15
Nevertheless, as with all live vector-based vaccines, LM-based vectors face a major drawback in that they pose potential safety risks, particularly for individuals with an impaired immune system.Citation16 To find the correct balance between safety and TAA-specific T cell immune responses, the strategy must be considered that is improving the safety of vaccine, reducing the immunizing dose as low as possible and enhancing the effective MHC class I-restricted T cell responses against TAAs. For the purpose of improving the cell-mediated immunity against HPV antigens delivering by bacterial vector, optimization of codon usage of genes encoded TAAs must be considered.Citation17–19 Codon usage has been identified as the single most important factor in prokaryotic gene expression, heretofore, codon biases can have a profound impact on the expression of heterologous proteins.Citation18,Citation20 Findings from some studies showed that codon usage optimization could improve high-level expression of heterologous genes from viruses or human.Citation21,Citation22 The frequencies of codon usage are found to be species-specific, so selecting optimal codons within an inserted gene to match the codon usage bias of the bacterial vector can result in enhanced translation of the target protein.Citation23,Citation24
Here, we constructed a codon-optimized HPV16 E7 recombinant vaccine based on analysis the codon usage and biases in serotype 1/2a L. monocytogenes, the expression level of HPV16 E7 and safety to mice were compared prior to codon optimization. Additionally, cellular immune response and therapeutic efficacy were evaluated in a tumor-bearing murine model.
2. Materials and methods
2.1. Strains and vectors
Serotype 1/2a LM4, LM4Δhly and LM4Δhly::E7 were preserved at the Jiangsu Key Laboratory of Zoonosis.Citation25 pAULA vector was gifted by Prof. Chakraborty (Giessen, Germany). Vector pMD20-T was purchased from TaKaRa (Dalian, China). LM4Δhly::E7-1 was constructed in this study. The cell line TC-1 was maintained in our lab.
2.2. Experimental animals
Six-week-old female C57BL/6 mice were purchased from Vital River Laboratories (Beijing, China). The mice were housed, handled, and immunized at the Animal Biosafety Facilities, and all procedures were approved by the institutional animal experimental committee of Yangzhou University.
2.3. Construction of L. monocytogenes delivering codon-optimized HPV16-E7
According to the codon usage bias of serotype 1/2a LM (http://www.kazasa.or.jp/codon/cgi-bin), codon-optimized HPV16 E7 (E7-1) was synthesized by TaKaRa (Fig. S1). The LM4 genome and E7-1 plasmid were used as templates to amplify hlya, hlyb and E7-1, then hlya-E7-1-hlyb was spliced with SOEing PCR and cloned into pMD20-T. The positive clone pMD20-T-hlya-E7-1-hlyb was digested by restriction endonuclease and ligated with pAULA vector, thus the recombinant plasmid pAULA-hlya-E7-1-hlyb was obtained with identified by sequencing. Lastly, the plasmids were transformed into competent LM4 by electroporation as previously described.Citation26 The recombinant strain LM4Δhly::E7-1 was obtained according to previously described methods.Citation27 An unrelated L. monocytogenes strain LM4Δhly constructed based on a common deletion region was included as an LM positive control. The positive clones were verified by sequencing. Primers used in this study were shown in .
Table 1. Primers used in this study
2.4. Quantitative real-time PCR
The exponential growth phase bacteria of LM4, LM4Δhly::E7 and LM4Δhly::E7-1 were collected respectively, the extraction of RNA referring RNeasy Plus Mini Kit (Qiagen) was performed. Firstly, 100 μL lysozyme and 20 μL protease K were added to the pellet, then suspending and incubation for 15 min at room temperature. Next, 350 μL cell lysis solution was added to the mixture, then RNA was eluted with RNase-free water. The cDNA from RNA through reverse transcription was obtained according to the protocol of PrimeScriptTM RT reagent kit. Resulting complementary DNA products were analyzed by quantitative real-time PCR (FastStart Universal SYBR Green Master Mix, Roche) using a 7500 Real-Time PCR system (ABI). Relative expression levels of codon-optimized E7, no-optimization and hly were normalized using gyrb as a reference control.
2.5. Western blot analysis of secreted proteins
Strains of LM4Δhly, LM4Δhly::E7 and LM4Δhly::E7-1 were inoculated in BHI liquid culture medium (100 mL), and cultured at 37°C, the supernatant was collected when the concentration of bacteria reached OD600 = 0.80. TCA with a final concentration of 10% was added and incubated at 4°C overnight. The following day, the solution was centrifuged at 9300 g for 15 min to collect the precipitate, it was then washed twice with acetone, and dissolved with Tris-HCl (pH8.0). The concentration of protein was determined with BCA Protein Assay Kit (Beyotime), and analyzed by SDS-PAGE (10 µg/well), then followed by Western blot, using anti-HPV16 E7 mouse monoclonal antibody (Clone 8C9; Invitrogen Life Technologies, Carlsbad, CA, USA) as primary antibody and HRP-labeled goat anti-mouse IgG as secondary antibody.
2.6. Hemolytic activity assay
Strains of LM4, LM4Δhly, LM4Δhly::E7 and LM4Δhly::E7-1 were inoculated in identical volumes of BHI liquid culture media and the supernatant was collected after culture at 37°C for 15 h. The supernatants were adjusted to the same OD600 value, and 70 μL was diluted with PBS (pH 6.0) to 7 doubling dilutions, and placed in a 96-well V-bottomed coagulation plate. Then, 30 μL of fresh 1% sheep red blood cell suspension (Solarbio) were added to each well, mixed gently, and incubated at 37°C for 1 h to observe hemolysis.
2.7. Growth curve of recombinant listerial strains
Listerial strains LM4Δhly, LM4Δhly::E7 and LM4Δhly::E7-1 were inoculated in BHI liquid culture medium and cultured at 37°C overnight. On the next day, an appropriate volume of the bacterial liquid culture was transferred to 10 mL of fresh BHI liquid culture medium, giving an OD600 value of 0.05, and incubated at 37°C in a shaker. Samples were collected at 0, 1.5, 3.0, 4.5, 6.0, 7.5, and 9.0 h to determine the OD600 values and plot the growth curve.
2.8. In vivo infection dynamics and pathological observation of recombinant LM4Δhly::E7-1
Three groups of C57BL/6 mice were intraperitoneally inoculated with 3 × 108 CFU bacteria of LM4Δhly::E7-1, LM4Δhly::E7 and LM4Δhly, respectively.Citation28 The mice were sacrificed at days 1, 3, 5, and 7, spleen and liver tissues were weighed, homogenized and plated. For the purpose of observation histopathology, another three groups of mice with same dosage and route were infected, a control group was inoculated with equivalent volume of PBS, mice were sacrificed at days 7 after the second immunization, the spleens and livers were harvested and fixed with 10% formaldehyde. Tissue changes were analyzed after the issue sections were storage in formaldehyde for 1 week.
2.9. Immunization procedure and tissue collection
Six-week-old female C57BL/6 (H-2 Db) mice were divided into four groups: LM4Δhly::E7-1, LM4Δhly::E7, LM4Δhly immunization groups and PBS control group (n = 5 per group). The mice were given both primary and booster immunizations by intraperitoneal injection (0.1 LD50 3 × 108 CFU), an interval of one week. The mice were then sacrificed at day 8 post booster immunization. A single spleen cell suspension was obtained after filtration with 200 μm mesh. The red blood cells were then lysed with red blood cell lysis buffer, then centrifuged at 199 g at 4°C for 10 min, after wash with PBS and culture medium for one time, respectively, the cells were re-suspended in culture medium and stored on ice after adjusting the density into 1 × 107 cells/mL.
2.9.1. Expression levels of IFN-γ and IL-4 in spleens measured by sandwich ELISA
The concentration of spleen single-cell suspension was adjusted to a density of 1 × 106/50 μL in each well. Fifty microliters of E749-57 (final concentration, 10 μg/mL) were added to corresponding wells for stimulation. Negative control (no stimulation), positive control (cells stimulated with ConA) and blank control groups were also set up, and each group was run in triplicate wells. After incubation for 48 h, the supernatant was collected, the level of IFN-γ and IL-4 was detected with sandwich ELISA. IFN-γ mAb (R4-6A2) and IL-4 mAb (BVD6-1D11) were used as coated antibody, correspondingly, IFN-γ-biotin mAb (R4-6A2) and IL-4-biotin mAb (BVD6-24G2) were used as captured antibody, then streptavidin HRP was added to catalyze reaction, the quantitative content of IFN-Y and IL-4 were detected according to the value of OD450 and the standard curve of IFN-γ and IL-4.
2.9.2. Lymphocyte proliferation
The single spleen cell suspension was adjusted to a density of 5 × 104 cells/50 μL in each well. Fifty microliters of E749-57 (final concentration, 10 μg/mL) was added to corresponding wells for stimulation, and a negative control group (no stimulation) was set. The plate was incubated at 37°C and 5% CO2 for 48 h, then 10 μL BrdU labeling liquid was added to each well and the plate incubated at the same condition for 12 h. The cell suspension was centrifuged at 199 g for 8 min at 4°C to remove the labeling liquid. The cells were cultured at 60°C for 1 h to completely remove the residual water. Fixdenat (200 μL/well) was added, incubated at 37°C for 30 min, and then removed. Anti-BrdU-POD working solution was added to each well, incubated at 37°C for 90 min, and then removed. The cells were washed three times with PBS, substrate was added (100 μL/well) and reacted at 37°C. Finally, 1 M H2SO4 (25 μL) was added to each well to terminate the reaction. The absorbance was measured at OD450.
2.9.3. Cytotoxic T lymphocyte (CTL) assay
To determine the cytotoxicity in vivo, the same immunization procedure was used as described as 2.9. On days 8 after boost immunization, spleen cells were pooled from naive C57BL/6 mice and divided into two groups; the cell suspension for one group was incubated with E7 peptide, at 37°C for 45 min and subsequently labeled with 2.5 μM carboxy fluorescein succinimidyl ester (CFSEhigh; MolecularProbes; Invitrogen) buffer at 37°C for 10 min; the other group was incubated without peptide and labeled with CFSElow (0.25 μM) buffer. The equal numbers of the two groups of cells were mixed, then 1 × 107 cells were intravenously injected into mice that had previously prime-boost immunized with PBS, LM4Δhly, LM4Δhly::E7-1 and LM4Δhly::E7 respectively. The next day, cell suspensions from spleens of mice were analyzed by flow cytometry technique (FACScan, Becton Dickinson) in which each population was detected according to the differing CFSE fluorescence intensities. Percentage specific lysis ratio was determined with the following formula: percent specific lysis = 100 – [100 × (percentage CFSEhigh immunized/percentage CFSElow immunized)/(percentage CFSEhighcontrol/percentage CFSElow control)].
2.10. Evaluation of recombinant LM4Δhly::E7-1 therapeutic effect
Six-week-old C57BL/6 mice were divided into four groups (n = 8 per group), and subcutaneously injected into the left flank of C57BL/6 mice with 5 × 105 TC-1 cells. After 7 days, tumor-bearing mice were immunized with LM4Δhly::E7-1, LM4Δhly::E7, LM4Δhly (at dose of 3 × 108 CFU) or PBS by intraperitoneal injection ant one week interval one. The tumor volume was measured with a vernier caliper every 3 days. The longest and shortest diameters were L and W. The tumor volume was calculated using equation: L× W2/2. The survival of each mouse was observed and recorded continuously for 36 days.
2.11. Data analysis
Statistical analyses were performed with Prism 6 (GraphPad Software, version 8). Two-way ANOVA with Tukey’s multiple comparisons test was used to compare the means of three or more groups. Statistically significant differences are: *P < .05; **P < .01; ***P < .001; ****P < .0001. Statistically nonsignificant (ns) was denoted when P-value was >0.05.
3. Results
3.1. Expressed fusion protein E7-1-LLO retaining immunogenicity
The genome was extracted and a positive clone was identified by PCR and sequencing by the Genescript, the results demonstrated that codon optimized HPV E7 was successfully inserted into the hly gene, which encodes the hemolysin protein Listeriolysin O (LLO) of LM4, resulting in the recombinant strain LM4Δhly::E7-1. qRT-PCR results showed that the transcript level of E7 was 12,000 times higher than that of LM4, and the transcript level of E7-1 was 450,000 times higher than that of LM4 (Fig S2). Using the TCA precipitation method, secreted proteins were extracted from the culture of LM4Δhly::E7-1, LM4Δhly::E7 and LM4Δhly, and analyzed by western blot. Equal loading of proteins was ascertained with the Coomassie brilliant blue R-250 staining (). A clear band at 66 kDa was detected with the anti-HPV16E7 monoclonal antibody (), indicating that LM4Δhly::E7-1 could secrete the fusion protein E7-LLO. In particular, the protein expression of codon-optimized E7 was remarkably higher than E7, it verified that optimization of codon in Listerial strain contributed to enhance the expression level of heterologous gene.
Figure 1. Characterization of the LM4Δhly::E7-1 strain
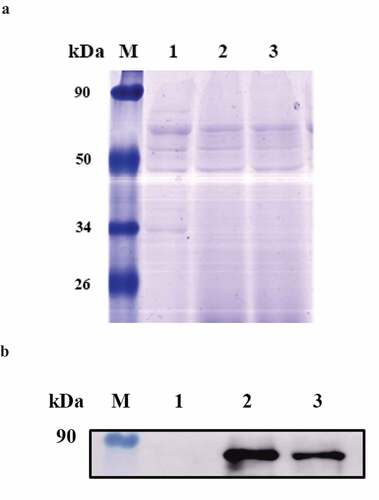
3.2. Reduced hemolytic activity
The hemolysis experiments demonstrated that the lysate titer of recombinant LM4Δhly:: E7-1 reached 2,Citation2 which reduced 16-fold comparing with wild-type LM4, 8 times lower than LM4Δhly, and similar to LM4Δhly::E7 (), showing significantly reduced hemolytic activity. These results suggested that with the E7-1 gene insertion down-stream of hly signal peptide sequence in LM4, the hemolytic activity of the expressed LLO was partially lost, suggesting improved safety in the two recombinant bacterial strains, and keeping the potential adjuvant function of LLO.
3.3. Decreased virulence to mice
The growth curves of three strains were no difference in vitro (), following immunization, bacteria in the spleen and liver of LM4Δhly::E7-1 group and LM4Δhly::E7 group were significantly lower than LM4Δhly group at most time points, they were completely eliminated by day 5, and two days earlier than LM4Δhly::E7 group. Additionally, the numbers of bacteria detected in individual spleen and liver in LM4Δhly::E7-1 group were significantly lower than that of in LM4Δhly::E7 group on day 3 post-infection, suggesting that recombinant LM4Δhly::E7-1 had increased safety in mice (). While the bacterial load in LM4Δhly-infected mice decreased slowly, the bacterial loads in liver and spleen were still higher than that of the other two groups on day 7 post-infection ().
Figure 3. Growth curves and infection kinetics in the liver and spleen of LM4Δhly::E7-1
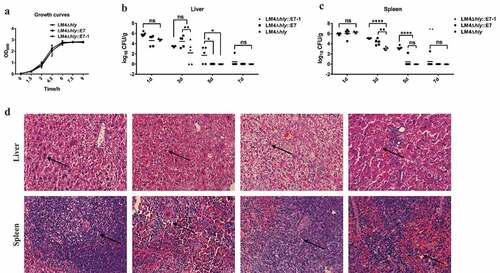
Histopathology observation illustrated that part of liver cells were vacuolar degeneration in the mice infected with LM4Δhly::E7, while there is no difference between LM4Δhly::E7 and control group. Additionally, there were no significant pathological changes in the spleen of mice in LM4Δhly::E7-1 and LM4Δhly::E7 group (). As far as LM4Δhly infection group, light granular degeneration and vesicular degeneration occurred in hepatocytes, and the structure of acini lienalis increased (). These results indicate that the safety of recombinant LM4Δhly::E7-1 was significantly improved comparing with LM4Δhly::E7.
3.4. Enhanced Th1-type immune response elicited with E7 Codon-optimization
After immunization, LM4Δhly::E7-1 (2627 ± 249.8 pg/mL) could induce 7.6-fold higher IFN-γ secretion in mice compared with the LM4Δhly::E7-immunized group (345.6 ± 31.96 pg/mL) (), suggesting that codon-optimized E7 delivered by LM could induce a stronger antigen-specific response in the host. Moreover, the IFN-γ secretion level of lymphocytes in the spleen was significantly higher than that of IL-4 in the LM4Δhly::E7-1 and LM4Δhly::E7-treated group (), suggesting that recombinant bacteria could induce generation of Th1-type biased immunity.
Figure 4. Enhanced stronger Th1-type immune response elicited with E7 Codon-optimization
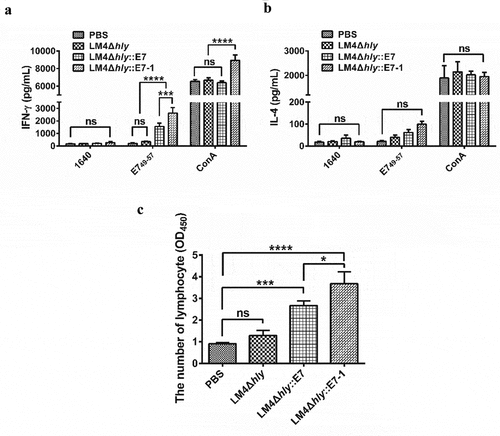
Under stimulation with E749-57 in vitro, proliferation of splenic lymphocytes in mice immunized with LM4Δhly::E7-1 was dramatically promoted compared with the LM4Δhly::E7-immunized mice (), indicating that the LM4Δhly::E7-1-immunized group could elicit stronger proliferation of lymphocytes.
CTLs moderate cell killing by secreting cytokines, playing a vital role in the cellular immune response. The E749-57-specific CTL killing activity induced by recombinant bacteria was measured by the CFSE labeling method. The result illustrated that the labeled CFSEhigh cell peak in LM4Δhly::E7-1-immunized mice was significantly lower than the CFSElow cell peak, suggesting that the cells labeled with CFSE were killed by specific CTLs in the immunized mice, and further eliminated in vivo. However, there was no significant difference between control LM4 and PBS groups, indicating there was no E749-57-specific CTL effect. The E7-specific killing efficiencies of LM4Δhly::E7-1 and LM4Δhly::E7 were 52 ± 7.17% and 48 ± 2.70%, respectively, suggesting the E7-specific CTL effect in the LM4Δhly::E7-1-immunized group was higher than that of LM4Δhly::E7-immunized mice ().
3.5. Immunotherapeutic efficacy by LM4Δhly::E7-1
Tumors gradually grew with time in mice immunized with LM4Δhly and in the PBS control group, all the mice succumbed to their tumor burden at day 36 (). However, no deaths were recorded for any of the mice immunized with the recombinant strains, and the tumors in part of the mice completely regressed after immunotherapy, while the tumors in the remaining mice grew more slowly than control group (Fig. S3). Thus, the vaccines delay the growth of tumors and have better therapeutic effect. This result indicates that Listeria vector vaccines may be a promising strategy by improving the potency of prophylactic and therapeutic HPV vaccines has potential clinical implications.
Figure 6. Survival curves of tumor-bearing mice
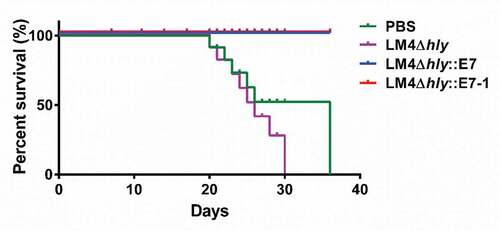
4. Discussion
Cervical cancer is one of the leading causes of cancer death among women and is of huge global concern, particularly in developing countries.Citation29,Citation30 Therefore, it is urgent to develop efficient strategies for reducing the occurrence and improving treatment effect. As far as treatment, great effort has been put into research, therapeutic vaccines are one of the considerable and inspiring strategies, 13 different therapeutic vaccines herein are underway in clinical trials.Citation31 Among varieties of vaccine candidates, the bacterial vector-based strategy is an attractive option, offering superior potency without the need for separate adjuvants, and convenience for large-scale manufacture.Citation32 Recently, two bacterial vector-based vaccines have been tested for safety and efficacy in clinical trials, LM-LLO-E7 (ADXS11-001) in phase I/II/IIIa, and a recombinant Lactobacillus Casei expressing modified version of HPV16-E7 (GLBL101c) in phase I/IIa.Citation31–34 A flaw in both of these two therapeutic vaccines was that HPV E7 gene was delivered in either chloramphenicol resistant (pGG55 in ADXS11-001) or erythromycin-resistant (pKV in GLBL101c) plasmid. Therefore, the vector carrying antibiotic resistance gene is potential risk of spreading antibiotic resistance genes in the human gastrointestinal tract.Citation35–39 Importantly, it was reported that immunization of Listeria vaccine led to bacteremia occurring.Citation40 At present, scientists are struggling for the highly safety and efficient therapeutic measures all over the world.
Our previous studies have reported the integration E7 gene in the bacterial genome replacing plasmid expression, and demonstrated that secretive expression of fusion proteins LLO-HPV16 E7 significantly elicited a strong antigen-specific T cell-mediated immune response.Citation13,Citation41 In the present study, we referred this construction methods and optimized nucleotide sequence of E7 gene according to listerial-biased codon usage, in particularly, fused the E7-1 gene with hly ORF and deleted a 42 bp region from the N-terminal amino acids ProGluSerThr (PEST)-like sequence. Notably, on the condition of same vaccination dosage, eradication speed of bacteria in organs of codon-optimized E7-treated group was quicker than not optimization. It is interesting that such strategy of construction led to codon-optimized HPV16 E7 vaccine strikingly reduced persistence time in vivo, dramatically improved its safety to the host compared to not optimization or LM4Δhly.
How to enhance cell-based immunogenicity and efficacy is the challenge to treat existing HPV infections and HPV-associated cancers, combinatorial approaches are being accepted to response this problem. Codon optimization has been identified as one of effective strategies to enhance immune responses by replacing codons that are rarely used in corresponding microorganisms.Citation42 At present, varieties of DNA vaccines with optimized or modified codons such as antigens from HPV, HIV, Influenza virus, Ebola virus and M. tuberculosis etc. have been shown to be highly immunogenic and induce strong cellular responses in animal models.Citation43–47 It is worth noting that listeria vector has been successfully developed as an attractive vaccine vector, particularly for tumor immunotherapy, while codon optimization of the E7 antigen according the usage bias of Listeria spp. has not been reported in Listeria-based HPV vaccines.Citation48,Citation49 In the present study, considering the serotype of LM4 is 1/2a, and the codon bias is distinct in different serotype strains of L. monocytogenes, we replaced the codons of E7 to serotype 1/2a LM-biased codons. Interestingly, we observed that the codon-optimized E7-containing vector LM4Δhly::E7-1 elicited a stronger E7-specific cellular immune response and caused dramatically enhanced therapeutic effect compared with LM4Δhly::E7. Our data demonstrated that the immune response could be further enhanced by optimization of E7 codon-usage in a listerial vector-based vaccine, thus causing efficient induction of the T cell immune response. Meanwhile, the tumor in the remaining mice immunized with the recombinant strains grew more slowly than control group, and no deaths were recorded. Thereby, the codon-optimized LM4Δhly::E7-1-immunized group showed better therapeutic effect than LM4Δhly::E7 immunized mice.
We further probed into the mechanism of enhancing therapeutic efficacy of E7 codon usage optimization. Previous reports have verified that codon bias correlates with the abundance of cognate tRNAs available in certain cells and so contributes to optimal translation.Citation18 Ikemura et al. observed that the tRNA Arg 4 that reads the infrequently used AGG and AGA codons for Arg is present in E. coli.Citation50 Interestingly, we found that amino acids were usually encoded between two to six types of codons for HPV16 E7, i.e., leucine was encoded by six codons (UUA, UUG, CUU, CUC, CUA, CUG), while only UUA was the biased codon encoded leucine in serotype 1/2a L. monocytogenes strains, so it was clearly showed the codon usage bias in different microorganisms were obviously diverse. Heretofore, we replaced 55 codons (56.7%) of E7 to serotype 1/2a LM-biased codons during construction of LM4Δhly::E7-1, and tempted to understand the functional effect of codon optimization. Interestingly, qRT-PCR results verified that the transcript level of E7-1 is far higher than that of E7. Western blot analysis showed that listeria-biased codon dramatically promoted the expression level of E7. Correspondingly, we observed that the expression of pro-inflammatory IFN-γ and E7-specific lymphocyte proliferation were elicited to a significantly greater extent, and the CD8+-specific lymphocyte reaction obviously increased in the codon-optimized E7-treated group compared with LM4Δhly::E7. Our results suggested that the immunogenic expression of E7 was enhanced following codon usage optimization, thus causing efficient induction of the cellular immune response. Therefore, our research supports the opinion that codon biases have a profound impact on the expression of heterologous antigens in prokaryotes.Citation13
The frequencies of codons are used vary significantly between different organisms; therefore, optimization of codon usage when delivering heterologous antigens is one approach worth thoroughly exploring. Taken together, our results suggest that optimization of codon usage in LM vector-based vaccines is an important consideration in constructing live bacterial vaccines and may be required for generation of effective T cell responses. Our research provides new ideas for obtaining a practical balance between safety and efficacy in an HPV therapeutic vaccine.
Optimization of codon usage in LM vector-based vaccine induced significantly higher cellular responses, and better therapeutic effect than no optimization, whereas there is still a long way from tumor-bearing mice evaluation to entering clinical trial. It is valuable to further optimize the immunization procedure, deeply probe into the CD8+ T cell immune response, and evaluate the therapeutic effect of codon optimized HPV16 E7 vaccine in nude mice model as well as primates.
Disclosure of potential conflicts of interest
The authors declared that they have no conflicts of interest to this work.
We declare that we do not have any commercial or associative interest that represents a conflict of interest in connection with the work submitted.
Competing interest
The authors have declared no competing interests.
Author contributions
The project was designed by Y.Y., X.J. and Z.P., Y.Y., F.D., J. C., H.Y., Y.W., Y.J., Z.L. and Y.F. performed experiments and data analysis. Y.Y. and F.D. prepared the draft of the manuscript.
Supplemental Material
Download Zip (797.1 KB)Supplementary material
Supplemental data for this article can be accessed on the publisher’s website
Additional information
Funding
References
- Wakeham K, Kavanagh K. The burden of HPV-associated anogenital cancers. Curr Oncol Rep. 2014;16(9):402. doi:10.1007/s11912-014-0402-4.
- Small W, Bacon MA Jr, Bajaj A, Chuang LT, Fisher BJ, Harkenrider MM, Jhingran A, Kitchener HC, Mileshkin LR, Viswanathan AN, et al. Cervical cancer: A global health crisis. Cancer. 2017;123(13):2404–12. doi:10.1002/cncr.30667.
- Luo X, Donnelly CR, Gong W, Heath BR, Hao Y, Donnelly LA, Moghbeli T, Tan YS, Lin X, Bellile E, et al. HPV16 drives cancer immune escape via NLRX1-mediated degradation of STING. J Clin Invest. 2020;130(4):1635–52. doi:10.1172/JCI129497.
- Zhu B, Xiao Y, Yeager M, Clifford G, Wentzensen N, Cullen M, Boland JF, Bass S, Steinberg MK, Raine-Bennett T, et al. Mutations in the HPV16 genome induced by APOBEC3 are associated with viral clearance. Nat Commun. 2020;11(1):886. doi:10.1038/s41467-020-14730-1.
- Kim HJ, Kim HJ. Current status and future prospects for human papillomavirus vaccines. Arch Pharm Res. 2017;40(9):1050–63. doi:10.1007/s12272-017-0952-8.
- Kash N, MA L, Kollipara R, Downing C, Guidry J, Tyring SK. Safety and efficacy data on vaccines and immunization to human papillomavirus. J Clin Med. 2015;4(4):614–33. doi:10.3390/jcm4040614.
- Yang A, Jeang J, Cheng K, Cheng T, Yang B, Wu TC, Hung CF. Current state in the development of candidate therapeutic HPV vaccines. Expert Rev Vaccines. 2016;15(8):989–1007. doi:10.1586/14760584.2016.1157477.
- Nomura T, Kawamura I, Tsuchiya K, Kohda C, Baba H, Ito Y, Kimoto T, Watanabe I, Mitsuyama M. Essential role of interleukin-12 (IL-12) and IL-18 for gamma interferon production induced by listeriolysin O in mouse spleen cells. Infect Immun. 2002;70(3):1049–55. doi:10.1128/IAI.70.3.1049-1055.2002.
- Stockinger S, Kastner R, Kernbauer E, Pilz A, Westermaye S, Reutterer B, Soulat D, Stengl G, Vogl C, Frenz T, et al. Characterization of the interferon-producing cell in mice infected with Listeria monocytogenes. PLoS Pathog. 2009;5(3):e1000355. doi:10.1371/journal.ppat.1000355.
- Pamer EG. Immune responses to Listeria monocytogenes. Nat Rev Immunol. 2004;4(10):812–23. doi:10.1038/nri1461.
- Pillich H, Chakraborty T, Mraheil MA. Cell-autonomous responses in Listeria monocytogenes infection. Future Microbiol. 2015;10(4):583–97. doi:10.2217/fmb.15.4.
- Maciag PC, Radulovic S, Rothman J. The first clinical use of a live-attenuated Listeria monocytogenes vaccine: a Phase I safety study of Lm-LLO-E7 in patients with advanced carcinoma of the cervix. Vaccine. 2009;27(30):3975–83. doi:10.1016/j.vaccine.2009.04.041.
- Jia YY, Tan WJ, Duan FF, Pan ZM, Chen X, Yin YL, Jiao XA. A genetically modified attenuated Listeria vaccine expressing HPV16 E7 kill tumor cells in direct and antigen-specific manner. Front Cell Infect Microbiol. 2017;7:279. doi:10.3389/fcimb.2017.00279.
- Yin Y, Lian K, Zhao D, Tao C, Chen X, Tan W, Wang X, Xu Z, Hu M, Rao Y, et al. A promising Listeria-vectored vaccine induces Th1-type immune responses and confers protection against tuberculosis. Front Cell Infect Microbiol. 2017;7:407. doi:10.3389/fcimb.2017.00407.
- Basu P, Mehta A, Jain M, Gupta S, Nagarkar RV, John S, Petit R. A randomized phase 2 study of ADXS11-001 Listeria monocytogenes-listeriolysin O immunotherapy with or without cisplatin in treatment of advanced cervical cancer. Int J Gynecol Cancer. 2018;28(4):764–72. doi:10.1097/IGC.0000000000001235.
- Ginaldi L, Loreto MF, Corsi MP, Modesti M, De Martinis M. Immunosenescence and infectious diseases. Microbes Infect. 2001;3(10):851–57. doi:10.1016/S1286-4579(01)01443-5.
- Lithwick G, Margalit H. Hierarchy of sequence-dependent features associated with prokaryotic translation. Genome Res. 2003;13(12):2665–73. doi:10.1101/gr.1485203.
- Gustafsson C, Govindarajan S, Minshull J. Codon bias and heterologous protein expression. Trends Biotechnol. 2004;22(7):346–53. doi:10.1016/j.tibtech.2004.04.006.
- Maertens B, Spriestersbach A, von Groll U, Roth U, Kubicek J, Gerrits M, Graf M, Liss M, Daubert D, Wagner R, et al. Gene optimization mechanisms: a multi-gene study reveals a high success rate of full-length human proteins expressed in Escherichia coli. Protein Sci. 2010;19(7):1312–26. doi:10.1002/pro.408.
- Xiang L, Wang Q, Zhou Y, Yin L, Zhang G, Ma Y. High-level expression of a ZEN-detoxifying gene by codon optimization and biobrick in Pichia pastoris. Microbiol Res. 2016;193:48–56. doi:10.1016/j.micres.2016.09.004.
- Zhao KN, Chen J. Codon usage roles in human papillomavirus. Rev Med Virol. 2011;21(6):397–411. doi:10.1002/rmv.707.
- Takakura Y, Katayama S, Nagata Y. High-level expression of tamavidin 2 in human cells by codon-usage optimization. Biosci Biotechnol Biochem. 2015;79(4):610–16. doi:10.1080/09168451.2014.991690.
- Lafay B, Lloyd AT, McLean MJ, Devine KM, Sharp PM, Wolfe KH. Proteome composition and codon usage in spirochaetes: species-specific and DNA strand-specific mutational biases. Nucleic Acids Res. 1999;27(7):1642–49. doi:10.1093/nar/27.7.1642.
- Sharp PM, Bailes E, Grocock RJ, Peden JF, Sockett RE. Variation in the strength of selected codon usage bias among bacteria. Nucleic Acids Res. 2005;33(4):1141–53. doi:10.1093/nar/gki242.
- Yin Y, Zhang C, Dong H, Niu Z, Pan Z, Huang J, Jiao X. Protective immunity induced by a LLO-deficient Listeria monocytogenes. Microbiol Immunol. 2010;54(4):175–83. doi:10.1111/j.1348-0421.2010.00211.x.
- Park SF, Stewart GS. High-efficiency transformation of Listeria monocytogenes by electroporation of penicillin-treated cells. Gene. 1990;94(1):129–32. doi:10.1016/0378-1119(90)90479-B.
- Zhou J, Liu WJ, Peng SW, Sun XY, Frazer I. Papillomavirus capsid protein expression level depends on the match between codon usage and tRNA availability. J Virol. 1999;73(6):4972–82. doi:10.1128/JVI.73.6.4972-4982.1999.
- Jia Y, Yin Y, Tan W, Duan F, Pan Z, Chen X, Jiao XA. Construction and characterization of an attenuated recombinant Listeria monocytogenes vector vaccine delivering HPV16 E7. Chinese J Biotechnol. 2016;32:683–92.
- Forman D, de Martel C, Lacey CJ, Soerjomataram I, Lortet-Tieulent J, Bruni L, Vignat J, Ferlay J, Bray F, Plummer M, et al. Global burden of human papillomavirus and related diseases. Vaccine. 2012;30:F12–F23. doi:10.1016/j.vaccine.2012.07.055.
- Gupta SM, Mania-Pramanik J. Molecular mechanisms in progression of HPV-associated cervical carcinogenesis. J Biomed Sci. 2019;26:28.
- Miles BA, Monk BJ, Safran HP. Mechanistic insights into ADXS11-001 human papillomavirus-associated cancer immunotherapy. Gynecol Oncol Res Pract. 2017;4:9. doi:10.1186/s40661-017-0046-9.
- Petit RG, Mehta A, Jain M, Gupta S, Nagarkar R, Kumar V, Premkumar S, Neve R, John S, Basu P. ADXS11-001 immunotherapy targeting HPV-E7: final results from a Phase II study in Indian women with recurrent cervical cancer. J ImmunoTher Cancer. 2014;2(S3):1–2. doi:10.1186/2051-1426-2-S3-P92.
- Kawana K, Adachi K, Kojima S, Taguchi A, Tomio K, Yamashita A, Nishida H, Nagasaka K, Arimoto T, Yokoyama T, et al. Oral vaccination against HPV E7 for treatment of cervical intraepithelial neoplasia grade 3 (CIN3) elicits E7-specific mucosal immunity in the cervix of CIN3 patients. Vaccine. 2014;32(47):6233–39. doi:10.1016/j.vaccine.2014.09.020.
- Komatsu A, Igimi S, Kawana K. Optimization of human papillomavirus (HPV) type 16 E7-expressing lactobacillus-based vaccine for induction of mucosal E7-specific IFNγ-producing cells. Vaccine. 2018;36(24):3423–26. doi:10.1016/j.vaccine.2018.05.009.
- Bosch FX, de Sanjosé S. Chapter 1: human papillomavirus and cervical cancer–burden and assessment of causality. J Natl Cancer Inst Monogr. 2003;2003(31):3–13. doi:10.1093/oxfordjournals.jncimonographs.a003479.
- Adachi K, Kawana K, Yokoyama T, Fujii T, Tomio A, Miura S, Tomio K, Kojima S, Oda K, Sewaki T, et al. Oral immunization with a Lactobacillus casei vaccine expressing human papillomavirus (HPV) type 16 E7 is an effective strategy to induce mucosal cytotoxic lymphocytes against HPV16 E7. Vaccine. 2010;28(16):2810–17. doi:10.1016/j.vaccine.2010.02.005.
- Huddleston JR. Horizontal gene transfer in the human gastrointestinal tract: potential spread of antibiotic resistance genes. Infect Drug Resist. 2014;7:167–76. doi:10.2147/IDR.S48820.
- Sacco JJ, Evans M, Harrington KJ, Man S, Powell N, Shaw RJ, Jones TM. Systemic listeriosis following vaccination with the attenuated Listeria monocytogenes therapeutic vaccine, ADXS11-001. Hum Vaccin Immunother. 2016;12(4):1085–86. doi:10.1080/21645515.2015.1121338.
- Yang A, Farmer E, Wu TC, Hung CF. Perspectives for therapeutic HPV vaccine development. J Biomed Sci. 2016;23(1):75. doi:10.1186/s12929-016-0293-9.
- Fares E, McCloskey CB, Gutierrez A, Princiotta M, Salinas LJ, Drevets DA. Vaccine strain Listeria monocytogenes bacteremia occurring 31 months after immunization. Infection. 2019;47(3):489–92. doi:10.1007/s15010-018-1249-7.
- Wang S, Huang W, Li K, Yao Y, Yang X, Bai H, Sun W, Liu C, Ma Y. Engineered outer membrane vesicle is potent to elicit HPV16E7-specific cellular immunity in a mouse model of TC-1 graft tumor. Int J Nanomedicine. 2017;12:6813–25. doi:10.2147/IJN.S143264.
- Ang KS, Kyriakopoulos S, Li W, Lee DY. Multi-omics data driven analysis establishes reference codon biases for synthetic gene design in microbial and mammalian cells. Methods. 2016;102:26–35. doi:10.1016/j.ymeth.2016.01.016.
- Wu J, Ma H, Qu Q, Zhou WJ, Luo YP, Thangaraj H, Lowrie DB, XY F. Incorporation of immunostimulatory motifs in the transcribed region of a plasmid DNA vaccine enhances Th1 immune responses and therapeutic effect against Mycobacterium tuberculosis in mice. Vaccine. 2011;29(44):7624–30. doi:10.1016/j.vaccine.2011.08.020.
- Fan RL, Valkenburg SA, Wong CK, Li OT, Nicholls JM, Rabadan R, Peiris JS, Poon LL. Generation of live attenuated influenza virus by using codon usage bias. J Virol. 2015;89(21):10762–73. doi:10.1128/JVI.01443-15.
- Soleimanjahi H, Razavinikoo H, Fotouhi F, Ardebili A. Antitumor response to a codon-optimized HPV-16 E7/HSP70 fusion antigen DNA vaccine. Iran J Immunol. 2017;14:180–91.
- Latanova AA, Petkov S, Kilpelainen A, Jansons J, Latyshev OE, Kuzmenko YV, Hinkula J, Abakumov MA, Valuev-Elliston VT, Gomelsky M, et al. Codon optimization and improved delivery/immunization regimen enhance the immune response against wild-type and drug-resistant HIV-1 reverse transcriptase, preserving its Th2-polarity. Sci Rep. 2018;8(1):8078. doi:10.1038/s41598-018-26281-z.
- Suschak JJ, Dupuy LC, Shoemaker CJ, Six C, Kwilas SA, Spik KW, Williams JA, Schmaljohn CS. Nanoplasmid vectors co-expressing innate immune agonists enhance DNA vaccines for Venezuelan Equine Encephalitis virus and Ebola virus. Molecular therapy. Mol Ther Methods Clin Dev. 2020;17:810–21. doi:10.1016/j.omtm.2020.04.009.
- Shahabi V, Reyes-Reyes M, Wallecha A, Rivera S, Paterson Y, Maciag P. Development of a Listeria monocytogenes based vaccine against prostate cancer. Cancer Immunol Immunother. 2008;57(9):1301–13. doi:10.1007/s00262-008-0463-z.
- Wood LM, Attenuated PY. Listeria monocytogenes: a powerful and versatile vector for the future of tumor immunotherapy. Front Cell Infect Microbiol. 2014;4:51. doi:10.3389/fcimb.2014.00051.
- Ikemura T. Correlation between the abundance of Escherichia coli transfer RNAs and the occurrence of the respective codons in its protein genes: a proposal for a synonymous codon choice that is optimal for the E. coli translational system. J Mol Biol. 1981;151(3):389–409. doi:10.1016/0022-2836(81)90003-6.