ABSTRACT
Vaccinia virus was used to prevent smallpox. After the World Health Organization declared smallpox extinct, vaccinia virus has been explored for the development of vaccines against a variety of infectious diseases. It also finds a new place in oncolytic therapy. Here we provide a brief review of the history, current status, and future prospect of vaccinia virus-based vaccine and oncolytic virus. New advancements, including a single vaccine targeting multiple viruses, strategies of arming vaccinia viruses to enhance anti-tumor activity, the promise and challenge of combining vaccinia-based virotherapy with immunotherapy, are discussed as special focus.
Introduction
Vaccinia virus (VV) was famous for its successful use as smallpox vaccine. Alike smallpox, VV is a poxvirus but less harmful. The smallpox vaccine, first reported by Edward Jenner in 1798, consists of live VVCitation1, and is acclaimed as one of the most successful vaccines ever made. Indeed, smallpox has been the only human disease to be completely eradicated and the WHO recommended in 1980 the global discontinuance of smallpox vaccination except for researchers at special risk of poxvirus infection. To date, VV has been continued to be explored for developing vaccines against other disease-causing human viruses because of its suitability for use as a vaccine vector. With a large genome (~190kb), vaccine virus can readily accommodate 10kb-15kb of foreign genes. Other advantages of vaccinia virus as a vaccine vector include good safety, stable antigen expression and convenient storage.
Most exciting but also controversial uses of VV are efforts to develop recombinant poxviruses as live viral agents (oncolytic virus) for cancer treatment. Oncolytic viruses selectively replicate in tumor cells, resulting in direct lysis of tumor cellsCitation2. During this death process, damage-associated molecular patterns (DAMPs) and tumor-associated antigens are released into the tumor micro-environment, which promotes the recruitment and activation of dendritic cells (DCs). As the activated DCs uptake, process and present the released tumor-associated antigens, tumor-specific effectors T lymphocytes are consequently stimulated to kill the tumor cells, including those uninfected. Thus, the oncolytic virus-induced tumor death is also coined as immunogenic cell death (ICD) because it triggers an inflammatory response capable of changing the tumor micro-environment from “cold” to “warm”. Viral expression of proinflammatory cytokines and/or T cell costimulatory molecules through bioengineering could further accelerate tumor clearance.
Oncolytic viruses (OV) include DNA and RNA viruses. DNA viruses have a large genome and are advantageous in expressing sizable exogenous genes. Compared to other DNA viruses, VV has a number of inherent features to support its use as an oncolytic agent. With a life cycle as short as 8 hours, VV replicates entirety in the cytoplasm, avoiding the risk of integration into host genome. Owing to its own transcription machinery further renders VV less susceptible to the status of host. VV, as distinct from other OVs, does not engage a specific entry receptor, bestowing a broad host and tissue tropism. Finally, and most importantly, VV has an outstanding safety track record tracing its over-a-century use as vaccine. Thus, it is not a surprise, that among the DNA virus-based viral vector (), a majority of the most advanced ones used in virotherapy are derived from VV.
Table 1. Clinical trials of oncolytic viruses of different types
Table 2. Clinical trials of viral vaccine vectors of different types
Choices of vaccinia virus: replicative versus non-replicative
In the process of eliminating smallpox, a variety of replicative vaccinia virus strains, including the Lister, NYCBH (New York City Board of Health), WR (Western Reserve), Wyeth, Copenhagen and Tiantan strains (TTV), appeared around the world. TTV (species number: 752–1) is the strain our laboratory has been using. In 1962, TTV was isolated from a smallpox patient in China; after many passages, TTV exhibited good immunogenicity and lower pathogenicity. TTV had been inoculated hundreds of millions of times in China until 1980, playing a decisive role in the elimination of smallpox. [.]
Figure 1. The history and application of TTV. TTV was isolated from smallpox patients in 1926. TTV passed through three generations in monkeys, five generations in rabbits, three generations in cattle, one to two generations in rabbits and one to three generations in cattle, before becoming the recombinant TTV that is now widely used against many diseases and tumors
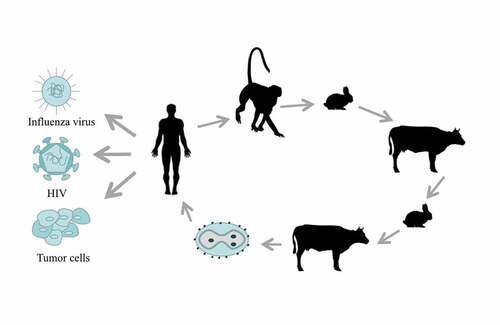
To enhance safety, many strains have an attenuated version generated through successive passages. The modified Ankara vaccinia virus (MVA) is originated from the chorioallantois vaccinia virus strain Ankara by continuous passaging for more than 500 times in chick embryo fibroblasts (CEFs). As a result of losing about 15% of viral genome, MVA is highly attenuated and is not able to replicate in most mammalian cells. Regarded as an efficient viral vector to express vaccinogen with remarkable security, MVA has been widely used in vaccine development. For example, recombinant MVA encoding the full-length MERS-CoV spike glycoprotein demonstrated high safety in an open-label, phase 1 trial, with most common systemic adverse effects presented on vaccine recipientsCitation3. NYVAC (vP866), derived from Copenhagen strain through plaque purification, is another common non-replicative vaccinia virus genetically attenuated by specific deletion of 18 open reading frames.
In fact, most, if not all, tumor-selective oncolytic vaccina viruses have been based on the differences between tumor cells and normal cells. For instance, vaccinia virus needs thymidine kinase (TK) to establish a nucleic acid pool for infecting resting cells. This requirement is no longer necessary for tumor cells, which are all proliferating. Consequently, a TK-deleted vaccinia virus is endowed with a capability to preferentially replicate in and kill tumor cells. The same rationale supports the deletion of F4L gene, which encodes small (R2) subunits of ribonucleotide reductases, and the F4L-deleted vaccinia virus indeed shows a selective replication in bladder tumor cells.Citation4
Harnessing the host immune system represents a new promising direction for generating vaccinia virus mutant with improved oncolytic efficacy. During the evolution of vaccinia virus, it develops various strategies to counter the host antiviral response through the action of encoded viral proteins. It is envisioned that disruption of these specialized viral proteins would generate mutant viruses with immunomodulatory profiles more suitable for in vivo killing of tumor cells in its own operation or in cooperation with other anti-tumor therapeutics. Two good examples are provided by C4/C16 and M2 proteins.Citation5,Citation6 The C4 and C16 protein of vaccinia virus antagonize the activity of DNA-PK as pattern recognition receptor (PPR) in recognizing the double-stranded viral genome. Accordingly, both single deletion viruses are attenuated in infected mouse compared to wild-type virus, likely due to increased induction of type I interferon and other cytokines mediating recruitment of inflammatory cells. It awaits further investigation whether the C4/C16-deleted viruses can maintain effective replication in tumors, which are often defective in innate immunity signaling pathways. The M2 viral protein, on the other hand, specializes in countering the host adaptive immunity by interfering with the B7/CD28-CTLA4-PD-L1 interactions. As expected, the M2-deleted vaccinia virus does not show detectable effect on replication in cultured cells. Although further testing of such virus in mouse model shows no change in oncolytic activity, with a more favorable immunomodulatory profile, its potential as a novel platform to develop oncolytic virus is a worthy exploration of the future.
Recombinant vaccinia virus and modification strategy for vaccine development
Homologous recombination can occur between VV genomes and transfected genomic or cloned DNA in infected cells, and this recombination enables effective insertion of foreign DNA sequence into the VV genomeCitation7 []. Currently, there are three main modification strategies of vaccinia virus vectors to improve their use as a vaccine framework: (1) Further controlling virus activity; (2) Enhancing the elicited immune response; (3) Reducing the immunogenicity induced by viral vector itself. During application of live attenuate vaccinia virus as a smallpox vaccine, there are 1–2 deaths and hundreds of complications requiring hospitalization per 1 million vaccine recipientsCitation8. Further improved safety profile would be expected from the next-generation viral vectors. Conventionally, weakened viruses have been obtained by continuous passage in animals until its virulence is attenuated enough to no longer cause disease. With a better understanding of the biology of vaccinia viruses, targeted attenuations have been attained by traditional homologous recombination by inactivating nonessential virulence-related genes and this endeavor is now further facilitated by the advent of CRISR-Cas9 technology. An effective way to enhance the immune response by VV vector is to insert into the viral genome one or more immune-stimulating molecules, exampled by interleukin (IL)-12, interferon (IFN)-γ, IL-2, IL-15, OX40/OX40L, GM-CSFCitation9.
Figure 2. Construction of recombinant TTV. First, the original TTV infects cells and releases the virus genome; then, the plasmid carrying foreign genes will transfect cells and recombine with the TTV genome, producing the recombinant TTV
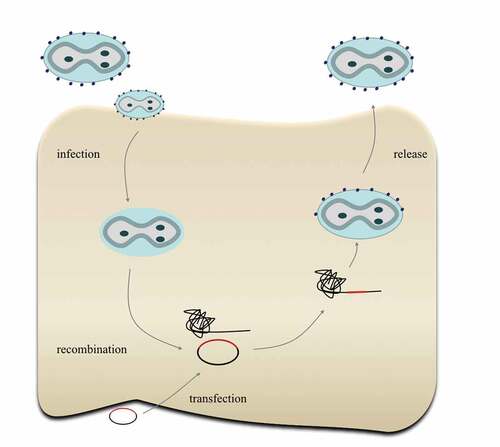
It is a general concern for all viral vector-based vaccines that the immune response against the vector component of vaccine may hamper the induction of immune response specific for the delivered antigen. This is why a vaccination normally does not allow the consecutive systemic administration of two vaccines built from the same viral vector as the first inoculation will risk a systemic immune response nullifying the second vaccine. For vaccinia-based vaccine, an additional challenge came from preexisting anti-vaccinia immunity in the older population receiving smallpox vaccine at a young age. One possible solution to the anti-vector immunity is modifying viral vector to eliminate the neutralizing antibody epitopes on the virus. Recently, Nakatake elegantly applied this solution to vaccinia virus, demonstrating that partial deletion of the host-targeting B5R epitopes from a vaccinia-derived oncolytic virus allows virus to retain oncolytic function irrespective of the presence of antiviral antibodyCitation10.
Vaccinia-based HIV vaccines
Despite the development and use of antiretroviral therapy (ART) greatly reducing the mortality rate, HIV remains a severe threat to human health. In 2018, 770,000 people died from HIV-related causes and 1.7 million became newly infected. The development of an HIV vaccine faces many challenges, including incomplete knowledge of immunization protection, high levels of genetic diversity, extremely high mutation rate accounting for immune escape, no least the complex glycan structures shielding the virus from neutralization. It has become clear that, to overcome these challenges, an effective HIV vaccine, which is not yet developed, need to engage both humoral and cellular arms of human immunity for targeting conserved viral targets.
The RV144 trial, known as the first sign of successful vaccination against HIV, highlighted the potential of poxvirus as a candidate framework for HIV vaccine creation. As a phase 3 randomized placebo-controlled trial, RV144 evaluated a 24-week prime-boost immunization comprised four priming injections of ALVAC-HIV (vCP1521), a recombinant attenuated non-replicating canarypox vector vaccine encoding Gag/Pro and Env antigens, plus two boost injections of AIDSVAX B/E, a bivalent gp120 subunit vaccine derived from a subtype B and subtype E envelope. The study involved 16042 subjects and a modified intention-to-treat analysis at 96 weeks revealed a modest but significant vaccine efficacy of 31.2% against HIV-1 infection compared to the placebo groupCitation11.
Further support of using VV as vector for HIV vaccine came from one most recent study of a therapeutic HIV vaccine regimen. This regimen comprises two doses of two different viral vectors – recombinant adenovirus serotype 26 (Ad26)-based Ad26. Mos. HIV and modified vaccinia Ankara (MVA)-based MVA-Mosaic, both of which are trivalent, expressing in silico-derived HIV ‘mosaic’ antigens. Immunizations were given over 48 weeks with two doses of Ad26.Mos.HIV and two doses of MVA-Mosaic. All 26 participants tolerated well with the vaccine and showed robust immune response together with delayed viral rebound after ARTCitation12. With this encouraging result, researchers plan to launch a large clinical trial to evaluate the protective efficacy of the same regimenCitation13. This mosaic approach can be further advanced by expanding the vector-encoded antigens to novel immunogens and immunomodulators.
Vaccinia-based vector vaccines against influenza and other viruses
To date, seasonal influenza virus infection has caused approximately 250,000 to 500,000 deaths each year. In addition to the spread of seasonal influenza viruses, new pandemic influenza viruses continue to emerge from pigs or birdsCitation14,Citation15. There is a need to develop universal influenza vaccines that are capable of inducing effective cross-group protective immunity. As an effort toward this end, our laboratory recently constructed a recombinant vaccinia-based vector vaccine expressing two bio-informatically identified, composite immunogens that broadly encompass highly conserved T-cell epitopes of six influenza A virus internal genes. When applied with a DNA prime expressing the same immunogens in a prime-boost modality, this vaccine elicited strong influenza-specific T cell response in immunized mice, affording cross-group protection upon lethal influenza challengeCitation16. In another study, Arunkumar et al. evaluated in mouse model an MVA-based vector vaccine encoding both chimeric hemagglutinin and nucleoprotein in addition to matrix protein 1. Their data showed that this vaccine is capable of inducing broad influenza-specific T cell and B cell immune responses, thereby increasing protection against influenza challenge. Progresses have been also made on the development of vaccinia-based vector against other viruses including ZIKACitation17 and HBVCitation18.
Vaccine of future: a single vector-based vaccine against several pathogens
Given the increasing variety of new and reemerging disease causative viruses, it is ideal to develop vaccination strategy that is capable of simultaneously targeting multiple viral pathogens. Conceivably, such multi-purpose vaccine can take two forms: a single carrier carrying multiple antigens corresponding to the pathogens to be targeted, or a mixture of vaccines each specifically against one pathogen by encoding the cognate antigen. VV is a particular fit for the second form, given its huge capacity for accommodation of foreign genes. In this respect, Prow et al. conducted a pioneering study in constructing a single VV-based vector vaccine to encode structural polyprotein sequences from both ZIKA- and chikungunya virus. Notably, a single administration of this multi-pathogen vaccine-induced neutralizing antibody response to both viruses in mice, generating dual protective immunity.
Vaccinia virus as oncolytic agent
Some of the guidelines for the development of vaccinia virus as oncolytic agent are similar to those employed for vaccine development, but new chapters are added to bolster its battle against tumor cells while limiting damages to normal cells.
Viral pathogenesis control: differentiating normal versus malignant cells
One important feature of oncolytic virus is their capability of preferably killing tumor cells. As aforementioned, vaccinia virus has a large genome containing many genes related to pathogenicity, editing these genes through genetic engineering provides an effective way to limit viral replication to tumor cells. Thymidine kinase (TK) and vaccinia growth factor (VGF) provide two exemplars of such pathogenicity-shaping genes that have been explored to engineer vaccinia virus for creating safer oncolytic agent. TK catalyzes the phosphorylation of thymidine to thymidine monophosphate, which is an important step toward synthesis of thymidine triphosphate, one of the four nucleotide triphosphates that are utilized for DNA synthesis. In normal cells, TK protein is only induced during DNA replication, and becomes rapidly degraded after the mitotic exit. The low level of TK protein in the cytoplasm was not sufficient to support viral replication. However, this limitation is lifted in tumor cells, which continuously synthesize TK proteins. Several studies have shown that the deletion of TK allows viruses to selectively replicate in tumor cells. JX-594, a replication-competent Wyeth strain vaccinia virus armed with human GMCSF and LacZ genes inserted into the TK gene locus, exhibited objective anti-tumor effects in a randomized phase II dose-finding trialCitation19. The vaccinia virus strain Guang9 (VG9) was derived from TTV through traditional single plaque purification, and showed lower neurovirulence and pathogenicity relative to the parental strain. Deng et al. constructed a targeted oncolytic VG9 strain called VG9-EGFP by inserting the EGFP marker gene into the TK gene regionCitation20. VG9-EGFP was attenuated in normal cells while maintaining growth similar to the parental VG9. Notably, VG-9 displayed potent anti-tumor activities in murine melanoma tumor model as well as human hepatoma model. Thus, TK-deleted vaccinia strain such as VG9 is regarded as a constructive virus vector for oncolytic virotherapy.
Vaccinia virus encodes two copies of vaccinia growth factor (VGF), which shares homology with epidermal growth factor (EGF). As a secreted protein released from vaccinia-infected cells at early phase of infection, VGF can activate the EGF receptor (EGFR), thereby functioning as a mitogen to prime surrounding host cells for viral spreading. Consequently, deletion of the VGF gene dramatically diminishes viral replication in resting cells and pathogenicity in vivo. It was reported that the effect of deleting TK and VGF genes was synergisticCitation21. Nude mice systematically injected with 1 × 108 pfu of TK-VGF- virus all lived >100 days, whereas the median survival time for mice receiving WT virus, TK-, or VGF-virus were merely 6, 17 and 29 days, respectivelyCitation22. The same TK-VGF- deleted virus, namely vvDD, was subsequently tested in two phase I studies trials, first with intramural injectionCitation23 followed by the second with intravenous administrationCitation21. In the first trial, vvDD was found to replicate exclusively in injected and non-injected tumors, and is well-tolerated with a maximum feasible dose of 3 × 109 pfu. There was also limited evidence supporting beneficial effect of vvDD on controlling tumor progression. The second trail further highlighted the safety of vvDD. Patients well tolerated the viral administration, with most common adverse effects being grade 1/2 flu-like symptoms and no reported dose-limiting toxicities. Unfortunately, only limited anti-tumor effect was observed for vvDD treatment and this lack of uniform anti-tumor activity might stem from the rapid clearance of vvDD before reaching sites of metastases as the result of an acute inflammatory response induced by systemic exposure to vvDD, or the failure to alter the suppressive status of tumor environment, or both. It was thus proposed that the next step for vvDD is to combine immune checkpoint blockade, or to be armed with an immunomodulatory transgene. It is worth mentioning that, unlike WR or other vaccinia virus strains, the two copies of VGF genes in TTV are not exactly the same. Whether VGF deletion can improve the safety profile of TTV-based oncolytic virus awaits future investigation.
Enhancing the lytic activity by transgene expression
Lack of sufficient tumor-infiltrating lymphocytes (TILs) is characteristic of tumor micro-environments (TMEs). Oncolytic virus-mediated lysis of tumor cells releases tumor-associated antigens and DAMPs, which recruit immune effector cells including tumor-specific CD8 + T cells, thereby facilitating the switch of TME from “cold” to “hot”. The anti-tumor activity of oncolytic virus thus closely correlated with its capability to reshape the TME. Accordingly, arming with immunomodulatory molecule(s), proflammatory cytokines in particular, has been long considered as an effective strategy to enhance the effectiveness of oncolytic virus. Along this way came the first FDA-approved oncolytic virus therapy, talimogene laherparepvec (T-VEC, or Imlygic®), which is derived from herpes simplex virus, type 1 (HSV-1) by genetic modifications including the insertion of two copies of human GM-CSF gene. GM-CSF enhanced the TVEC immunogenicity through promoting dendritic cell recruitment and enhancing the function of antigen presenting cells. The GM-CSF strategy has been extended to vaccinia-based oncolytics, creating the above-mentioned JX-594 and more recent VG9-GMCSF of TTV origin. VG9-GMCSF, which was generated from VG9 by inserting a single GM-CSF gene into TK locus, exhibited stronger anti-tumor immunity in mouse tumor model, prolonging survival compared with the parental VG9. The fact that VG9-GMCSF was present at higher level in tumors while at lower level in normal tissues than that in the VG9 group strengthens the note that TK-deleted TTV is much safer than original virus. Looking for more arms in the natural arsenal of immunoregulatory molecules has become a major direction for development of vaccinia-based oncoviral therapy.
Suicide genes represent another type of transgene that can enhance lytic activity of oncolytic virus. Adenoviruses set up an example as bearing suicide transgene, such as bacterial cytosine deaminase (CD) and adenovirus death protein (ADP) afforded an enhanced anti-tumor activity to the modified virusCitation24. Theoretically, tumor suppressor genes, anti-oncogenes, and prodrug-converting enzymes could all serve to arm oncolytic viruses.
Conceivably, oncolytic virus including vaccinia virus can be optimized by being armed with two or more transgenes, especially for those complementing each other in function to synergistically promote anti-tumor response. This is extremely attractive with vaccinia virus, given its large capacity in accommodating foreign genes. The co-expression of multiple transgenes in vaccinia virus can be achieved through a number of ways, including employment of multiple promoters (viral or cellular), usage of internal ribosomal entry sequence (IRES), and adoption of 2A self-cleaving peptide-based multigene expression system (2A – MGES). In 2A-MGES, the genes seeking co-expression are concatenated to a single ORF via 2A peptide-encoding sequence; the resulting artificial polypeptide is processed to simultaneously produce the wanted multiple proteins owing to co-translational cleavage mediated by 2A peptide. As an example, a recent study successfully used 2A-MGES to generate an oncolytic vaccinia virus double armed with GM-CSF and IL-21Citation25.
Stimulating tumor-specific immune response
Epitope spreading is an integral part of the anti-tumor activity of oncolytic virus. In connection, oncolytic virus can be engineered to encode tumor antigen(s). By delivering tumor antigens to the tumor environment, such recombinant virus is advantageous in overcoming the immune escape and facilitating the development of systemic anti-tumor immunity.
Human Epidermal growth factor Receptor 2, encoded by HER2/neu, is a candidate of cancer therapy as its over expression has been associated with approximately 20% of breast cancersCitation26. Using a model of syngeneic HER2/neu- overexpressing mammary carcinoma in FVB/N mice (NBT1), de Vries et al. reported that co-injection of two recombinant vaccinia virus VVneu and VVGMCSF, respectively, encoding HER2/neu and GM-CSF, induces a systemic HER2/neu- specific immunity that is not attainable with intratumoral VVGMCSF. VVneu- VVGMCSF combination also results in prominent reduction in the immune-suppressive myeloid-derived suppressor cells (MDSCs) in tumor as well as spleen. Consequently, the combination treatment shows significantly higher anti-tumor efficacy as compared to single treatment. One caveat of this study is that two oncolytic viruses of the same type are simultaneous applied, raising the question whether their interactions have a role in the observed synergistic effect. This question can be answered by future creation and assessment of a single recombinant vaccinia virus encoding both HER2/neu and GMCSF. Polymorphic epithelial mucin (MUC1), and Carcinoembryonic antigen (CEA) provides additional examples of tumor-associated antigens (TAAs), for cancer immunotherapyCitation27. Gatti-Mays recently reported a phase I clinical trial of the BN-CV301, a poxviral-based vaccine using a recombinant (rec.) Modified vaccinia Ankara (MVA-BN-CV301) as prime and rec. fowlpox (FPV-CV301) as boost. BN-CV301 was constructed by inserting into the viral framework genes encoding two TAAs in MUC1 and CEA and three costimulatory molecules in B7-1, ICAM-1 and LFA-3. The results showed no dose-limiting toxicities, with the majority of adverse effects being temporary and self-limiting. Notably, specific T-cell response to MUC1 and CEA was elicited in most patients.
Selecting the right administration route
Like other oncolytic viruses, it is generally accepted that VV should be administrated through intratumoral injection (i.t). This is largely because that systemic administration is expected to face challenge from preexisting anti-vaccinia immunity as neutralizing antibodies (nAb) would block viruses from reaching tumor cells. However, intratumoral injection is only suitable for visible tumors, and carries the risk of triggering metastasis. Recent research on reovirus revealed the antibody-neutralized virus could remain infective with aid from monocyte. When loaded on monocytes, the preformed reovirus-nAb complex can be delivered to and subsequently kill the tumor cells. A working mechanism for this special delivery pathway has been proposed that the antibody receptor, FcgRIII in particular, promotes the binding of virus/antibody complexes to monocyte and subsequent internalization. With the antibody degraded inside the monocyte, the virus is released to restore its infectivity. Although the generality of this mechanism needs further investigation, it provides new opportunity for delivery of vaccinia virus via systemic routeCitation28.
Combine with other therapy for a better treatment
As one of the tumor immunotherapies, oncolytic viruses target multiple steps within the cancer–immunity cycle. When combined with other therapies, oncolytic viruses can help establish long-lasting stable anti-tumor immunity. Immune checkpoint inhibitors have emerged as one of the most promising agents in immunotherapy. A recent phase 1b clinical trial tested a combination treatment of talimogene laherparepvec, the first-in-class oncolytic virus derived from herpes simplex virus, with the anti-PD-1 antibody pembrolizumab, and reported unprecedented therapeutic efficacy paralleled by remarkable improvement in cytotoxic T cell infiltrationCitation29. However, there are no reports about the combination of TTV and immune checkpoint inhibitors.
Recently, the combination of recombinant vaccinia virus VG9 and the STAT3 inhibitor Stattic was proven to have a preferable anti-tumor effect compared to either agent alone when tested on immunodeficient nude mice bearing HeLa cellCitation30. STAT3, which can be activated by many viral infections, is a well-known transcription factor that promotes the proliferation, differentiation, and anti-apoptosis of tumor cells. The synergetic effect between VG9 and Stattic in tumor suppression might arise from distinct mechanisms used by VG9 and Stattic to kill tumor cells: apoptosis by VG9 and autophagy by Stattic. By the same reasoning, it is conceivable that vaccinia-based oncolytic viruses can be combined with radiotherapy or chemotherapy to achieve anti-tumor effects not attainable by either therapy alone.
Conclusion
As one of the oldest viral vectors used for vaccine development, the exploration of vaccina virus for disease prevention and treatment has entered a new era. A better understanding of biology of vaccinia virus, aided by the advent of new gene-editing technology, is likely to drive a paradigm shift of vaccinia-based vaccine from specializing in a single pathogen to simultaneously targeting multiple pathogens. This will bring the benefit of reducing the cost of vaccine development. Given the preexisting anti-vaccinia human immunity, how to achieve systemic administration of vaccinia virus-based vaccine remains a challenge and requires the development of new delivery method. The solution to the problem is equally important for vaccinia-based oncolytic viruses, which has seen extensive efforts to improve their safety and anti-tumor efficacy. There is growing consensus that the tumor-killing efficacy of oncolytic virus is dictated by a balance between engaged anti-viral and anti-tumor immunity. Accordingly, arming oncolytic virus with immune-regulatory molecule to enhance its ability to reshape the tumor environment has become a main strategy to improve virotherapy. Analogous to the encoding of multiple antigens by a single vaccinia-based vaccine, the vaccinia-based oncolytic virus can be engineered to co-express multiple immune-stimulating molecules to deliver a synergistic anti-tumor activity. It will be also exciting to further pursue the combination strategy where the power of virotherapy and other therapy, especially different forms of immunotherapy, are united. With all these efforts, we would expect to see a new growth of vaccinia virus as a constructive agent against infectious diseases and cancer.
Disclosure of potential conflicts of interest
No potential conflicts of interest were disclosed.
Additional information
Funding
References
- Liu MA. Vaccine developments. Nat Med. 1998;4:515–19. doi:10.1038/nm0598supp-515.
- Kaufman HL, Kohlhapp FJ, Zloza A. Oncolytic viruses: a new class of immunotherapy drugs. Nat Rev Drug Discov. 2015;14:642–62. doi:10.1038/nrd4663.
- Koch T, Dahlke C, Fathi A, Kupke A, Krähling V, Okba N, Halwe S, Rohde C, Eickmann M, Volz A, et al. Safety and immunogenicity of a modified vaccinia virus Ankara vector vaccine candidate for Middle East respiratory syndrome: an open-label, phase 1 trial. Lancet Infect Dis. 2020;20:827–38. doi:10.1016/S1473-3099(20)30248-6.
- Potts KG, Irwin CR, Favis NA, Pink DB, Vincent KM, Lewis JD, Moore RB, Hitt MM, Evans DH. Deletion of F4L (ribonucleotide reductase) in vaccinia virus produces a selective oncolytic virus and promotes anti-tumor immunity with superior safety in bladder cancer models. EMBO Mol Med. 2017;9:638–54. doi:10.15252/emmm.201607296.
- Scutts SR, Ember SW, Ren H, Ye C, Lovejoy CA, Mazzon M, Veyer DL, Sumner RP, Smith GL. DNA-PK is targeted by multiple vaccinia virus proteins to inhibit DNA sensing. Cell Rep. 2018;25:1953–65. doi:10.1016/j.celrep.2018.10.034.
- Kleinpeter P, Remy-Ziller C, Winter E, Gantzer M, Nourtier V, Kempf J, Hortelano J, Schmitt D, Schultz H, Geist M, et al. By binding CD80 and CD86, the vaccinia virus M2 protein blocks their interactions with both CD28 and CTLA4 and potentiates CD80 Binding to PD-L1. J Virol. 2019;93. doi:10.1128/JVI.00207-19.
- Mackett M, Smith GL, Moss B. Vaccinia virus: a selectable eukaryotic cloning and expression vector. Proc Natl Acad Sci U S A. 1982;79:7415–19. doi:10.1073/pnas.79.23.7415.
- Kennedy RB, Ovsyannikova I, Poland GA. Smallpox vaccines for biodefense. Vaccine. 2009;27(Suppl 4):D73–D79. doi:10.1016/j.vaccine.2009.07.103.
- Garcia-Arriaza J, Esteban M. Enhancing poxvirus vectors vaccine immunogenicity. Hum Vaccin Immunother. 2014;10:2235–44. doi:10.4161/hv.28974.
- Nakatake M, Kurosaki H, Kuwano N, Horita K, Ito M, Kono H, Okamura T, Hasegawa K, Yasutomi Y, Nakamura T. Partial deletion of glycoprotein B5R enhances vaccinia virus neutralization escape while preserving oncolytic function. Mol Ther Oncolytics. 2019;14:159–71. doi:10.1016/j.omto.2019.05.003.
- Gray GE, Huang Y, Grunenberg N, Laher F, Roux S, Andersen-Nissen E, De Rosa SC, Flach B, Randhawa AK, Jensen R, et al. Immune correlates of the Thai RV144 HIV vaccine regimen in South Africa. Sci Transl Med. 2019;11. doi:10.1126/scitranslmed.aax1880.
- Colby DJ, Sarnecki M, Barouch DH, Tipsuk S, Stieh DJ, Kroon E, Schuetz A, Intasan J, Sacdalan C, Pinyakorn S, et al. Safety and immunogenicity of Ad26 and MVA vaccines in acutely treated HIV and effect on viral rebound after antiretroviral therapy interruption. Nat Med. 2020;26:498–501. doi:10.1038/s41591-020-0774-y.
- Mega ER. ‘Mosaic’ HIV vaccine to be tested in thousands of people across the world. Nature. 2019;572:165–66. doi:10.1038/d41586-019-02319-8.
- Herfst S, Schrauwen EJ, Linster M, Chutinimitkul S, de Wit E, Munster VJ, Sorrell EM, Bestebroer TM, Burke DF, Smith DJ, et al. Airborne transmission of influenza A/H5N1 virus between ferrets. Science. 2012;336:1534–41. doi:10.1126/science.1213362.
- Fouchier RA, Garcia-Sastre A, Kawaoka Y. H5N1 virus: transmission studies resume for avian flu. Nature. 2013;493:609. doi:10.1038/nature11858.
- Xie X, Zhao C, He Q, Qiu T, Yuan S, Ding L, Liu L, Jiang L, Wang J, Zhang L, et al. Influenza vaccine with consensus internal antigens as immunogens provides cross-group protection against influenza A viruses. Front Microbiol. 2019;10:1630. doi:10.3389/fmicb.2019.01630.
- Perez P, Lazaro-Frias A, Jimenez DON, Blazquez AB, Escribano-Romero E, Ortego J, Saiz JC, Esteban M, et al. A vaccine based on a modified vaccinia virus ankara vector expressing zika virus structural proteins controls zika virus replication in mice. Sci Rep. 2018;8:17385. doi:10.1038/s41598-018-35724-6.
- Deng Y, Chuai X, Chen P, Chen H, Wang W, Ruan L, Li W, Tan W. Recombinant vaccinia vector-based vaccine (Tiantan) boosting a novel HBV subunit vaccine induced more robust and lasting immunity in rhesus macaques. Vaccine. 2017;35:3347–53. doi:10.1016/j.vaccine.2017.04.059.
- Heo J, Reid T, Ruo L, Breitbach CJ, Rose S, Bloomston M, Cho M, Lim HY, Chung HC, Kim CW, et al. Randomized dose-finding clinical trial of oncolytic immunotherapeutic vaccinia JX-594 in liver cancer. Nat Med. 2013;19:329–36. doi:10.1038/nm.3089.
- Deng L, Fan J, Guo M, Huang B. Oncolytic and immunologic cancer therapy with GM-CSF-armed vaccinia virus of Tian Tan strain Guang9. Cancer Lett. 2016;372:251–57. doi:10.1016/j.canlet.2016.01.025.
- Downs-Canner S, Guo ZS, Ravindranathan R, Breitbach CJ, O’Malley ME, Jones HL, Moon A, McCart JA, Shuai Y, Zeh HJ, et al. Phase 1 study of intravenous oncolytic poxvirus (vvDD) in patients with advanced solid cancers. Mol Ther. 2016;24:1492–501. doi:10.1038/mt.2016.101.
- McCart JA, Ward JM, Lee J, Hu Y, Alexander HR, Libutti SK, Moss B, Bartlett DL. Systemic cancer therapy with a tumor-selective vaccinia virus mutant lacking thymidine kinase and vaccinia growth factor genes. Cancer Res. 2001;61:8751–57.
- Zeh HJ, Downs-Canner S, McCart JA, Guo ZS, Rao UN, Ramalingam L, Thorne SH, Jones HL, Kalinski P, Wieckowski E, et al. First-in-man study of western reserve strain oncolytic vaccinia virus: safety, systemic spread, and antitumor activity. Mol Ther. 2015;23:202–14. doi:10.1038/mt.2014.194.
- Hermiston TW, Kuhn I. Armed therapeutic viruses: strategies and challenges to arming oncolytic viruses with therapeutic genes. Cancer Gene Ther. 2002;9:1022–35. doi:10.1038/sj.cgt.7700542.
- Deng L, Yang X, Fan J, Ding Y, Peng Y, Xu D, Huang B, Hu Z. An oncolytic vaccinia virus armed with GM-CSF and IL-24 double genes for cancer targeted therapy. Onco Targets Ther. 2020;13:3535–44. doi:10.2147/OTT.S249816.
- Pernas S, Tolaney SM. HER2-positive breast cancer: new therapeutic frontiers and overcoming resistance. Ther Adv Med Oncol. 2019;11:432518737. doi:10.1177/1758835919833519.
- Jochems C, Tucker JA, Vergati M, Boyerinas B, Gulley JL, Schlom J, Tsang KY. Identification and characterization of agonist epitopes of the MUC1-C oncoprotein. Cancer Immunol Immunother. 2014;63:161–74. doi:10.1007/s00262-013-1494-7.
- Berkeley RA, Steele LP, Mulder AA, van den Wollenberg D, Kottke TJ, Thompson J, Coffey M, Hoeben RC, Vile RG, Melcher A, et al. Antibody-neutralized reovirus is effective in oncolytic virotherapy. Cancer Immunol Res. 2018;6:1161–73. doi:10.1158/2326-6066.CIR-18-0309.
- Ribas A, Dummer R, Puzanov I, VanderWalde A, Andtbacka R, Michielin O, Olszanski AJ, Malvehy J, Cebon J, Fernandez E, et al. Oncolytic Virotherapy Promotes Intratumoral T Cell Infiltration and Improves Anti-PD-1 Immunotherapy. Cell. 2018;174:1031–32. doi:10.1016/j.cell.2018.07.035.
- Yang R, Wang L, Sheng J, Huang Q, Pan D, Xu Y, Yan J, Wang X, Dong Z, Yang M. Combinatory effects of vaccinia virus VG9 and the STAT3 inhibitor Stattic on cancer therapy. Arch Virol. 2019;164:1805–14. doi:10.1007/s00705-019-04257-2.