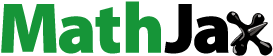
ABSTRACT
Cellular immunity is crucial for an efficient host immune response against rabies virus (RABV) infection. But the T cell receptor (TCR) repertoire in human after RABV vaccine immunization remained unclear. In this study, we conducted high-throughput sequencing of TCR β chain complementarity determining region 3(CDR3) repertoires in 4 healthy volunteers before and after immunization with RABV vaccine. Our data showed that RABV vaccination changed the TCR diversity and the usage of V/J gene segments, as well as V-J pairing. The high-frequency clonotypes that altered after vaccination were identified. These results may provide us with new insights into T cell receptor condition after RABV vaccination.
Introduction
Rabies, caused by the infection of rabies virus (RABV) is still one of the deadliest zoonosis disease.Citation1 It is nearly 100% fatal once clinical symptoms develop.Citation2 According to WHO, rabies ranks as one of the most deadly zoonotic disease that causes as many as 60,000 deaths each year. More than 80% of these deaths come from Asia, while China has the highest burden after India. Notably, children account for about 40% of the deaths. As the start the infection, 99% of the rabies are attributed to bites or scratch of infected animals, such as dogs.Citation3 Otherwise, RABV that transported from bats pose a new challenge to public health in developed countries.Citation4
RABV is a negative-stranded RNA virus belonging to the genus lyssavirus in Rhabdoviridae family, and its genome is approximately 12 kb.Citation5 Most rabies resulted from infected animal bites or scratches, through which RABV particles transport to human muscle cells in the wounds.Citation6 RABV either replicates in muscle cell or infects neurons directly depends on the initial virus amount. RABV spreads to motor neurons by synapses, and subsequently travels to central neural system by retrograde axonal transport.Citation7 Human develops clinical symptoms when actually the RABV have reached the central nervous system (CNS),Citation7 where RABV causes neuronal dysfunction or death.Citation8
Cellular immunity plays an important role in human anti-RABV defense. Human CNS harbors various innate immune cells (i.e., macrophages, astrocytes, and microglia), adaptive immune cells (i.e., T cells, B cells) were indispensable for efficient host immune responses.Citation9 The role of T cells in host anti-RABV can be partly proved by the fact that peripheral inoculation of nude mice with a normally apathogenic RABV resulted in a fatal outcome.Citation10 Other evidences showed that, RABV infection causes activated T cells both in draining lymph nodes and peripheral blood.Citation11,Citation12 Besides, molecules expressed on the surface of activated T cells, i.e. very late antigen-4 (VLA-4) and leukocyte-function associated antigen-1 (LFA-1) equip them the ability to cross the blood-brain barrier (BBB).Citation13 On the contrary, pathogenic RABV mediated decreased T cells in the CNS resulted in an impairment of the lymphocyte-mediated response.Citation11,Citation14 These data indicate that T lymphocytes have a protective role in controlling RABV replication.
T cell receptor (TCR) expressed on the surface of T cells recognizes the antigenic peptides presented by antigen presented cells (APC). Human TCRs were commonly composed of α and β chains.Citation15 The diversity of TCR mostly comes from TCRβ, which were estimated to reach a level of as many as 1106.Citation16 The alteration of TCR repertoire has been observed in many diseases such as malignancy, autoimmune disorders and infectious diseases.Citation17,Citation18 Thus, TCR repertoire is suggested to have great diagnosis value and clinical utility, as its diversity reflects the state of immune system.Citation19 Researchers begin to explore the association between changes in TCR repertoire and diseases, on propose to identify novel biomarkers or prognostic factors.Citation20–22 However, the alteration of TCR repertoire regarding RABV infection remains unclear.
In the present study, we aimed to characterize the TCR profile in subjects vaccinated with RABV vaccine using the high-throughput sequencing, thus determined the role of T cell-mediated response in human immune system against RABV infection. This study may provide us with new insights into the biological role of T cells in protection of host against RABV infection.
Methods and methods
Study subjects
This study was conducted according to protocols approved by the Research Ethics Committee of Yuebei People’s Hospital, Shantou University Medical College, Shaoguan, China. Written informed consents were obtained from all participants. Four healthy volunteers aged from 25 to 35 and haven’t received a RABV vaccine before were enrolled in this study. The clinical information on the four subjects: (V1: Male, 31 years old; V2: Female, 38 years old; V3: Male, 34 years old; V4: Female, 23 years old). All volunteers had vaccination history of rabies virus vaccine. The participants were injected with anti-RABV vaccines (Rabies Vaccine (Vero Cell) for Human Use, Freeze-Dried (Cat # 201703027–1); Changchun Institute of Biological Products Co. Ltd) in day 0, day 7 and day 21 according to the manufacturer’s protocol. The effect of vaccination was evaluated in 7 days after the last injection.
Sample collection
Peripheral venous blood samples of participants were collected before vaccination and 7 days after last injection, respectively. The blood samples were stored in EDTA-coated tubes and processed within 30 min. PBMCs were separated by density gradient centrifugation using Hypaque-Ficoll (GE Healthcare Bio-sciences AB, Sweden). The isolated PBMCs were lysis using TRIzol reagent (Invitrogen, USA) and stored at −80 until used.
RNA extraction and cDNA synthesis
1106 PBMCs from each sample were used for total RNA extraction. Total RNA was extracted using RNeasy Kit (QIAGEN, German) following the manufacture’s protocol. The concentrations and purity of RNA were assessed by NanoDrop-1000 spectrophotometer (Thermo FisherScientifc, Inc. USA) and RNA integrity was analyzed by the Bioanalyzer 2100 system (Agilent Technologies, CA, USA). cDNA libraries for high-throughput sequencing were prepared by 5ʹ-rapid amplification of cDNA ends (RACE) using the SMARTer PCR cDNA synthesis kit (Clontech, USA) as described previously.Citation23 Briefly, 1.5
g of total RNA was mixed with the primer BC1R (CAGTATCTGGAGTCATTGA), which is specific for human TCR
cDNA synthesis. To denature RNA and anneal the priming oligonucleotides, RNA was incubated at 72°C for 3 min and then at 42°C for 2 min. The SMARTer IIA oligonucleotide and SMARTScribe reverse transcriptase were added for 5ʹ-template switching and the cDNA synthesis reaction, which was performed at 42°C for 60 min.
Library construction and sequencing
Libraries were prepared using two-round PCR with specific primers as reported previously.Citation23 Briefly, in the first-run PCR, the reaction system containing cDNA, Advantage 2 polymerase mix (Clontech, USA), universal primer Smart 20 (CACTCTATCCGACAAGCAGTGGTATCAACGCAG), and TCR -specific primer (TGCTTCTGATGGCTCAAACAC) was prepared. TCR-
was amplified using Roche LightCycler 480 (Roche, USA) with the following program: 95°C for 20 s, 65°C for 20 s, 72°C for 50 s, for a total of 18 cyclers. The products were purified using the QIAquick PCR purification kit (QIAquick, German).
In the second-run PCR, the reaction system was prepared containing the purified product, universal primers Step1 (CACTCTATCCGACAAGCAGT), and specific primer hum-bcj (ACACSTTKTTCAGGTCCTC). Amplification was carried out Roche LightCycler 480 with the following program: 95°C for 20 s, 65°C for 20 s, 72°C for 50 s, for a total of 12 cycles. The products were purified as above. Libraries were amplified using Illumina sequencing primers with barcodes. Then, paired-end 150 bp sequencing was performed on the Illumina HiSeq2000 platform in ShenZhen Realomics Inc.
Bioinformatic analysis
The sequencing quality of the library by Illumina Hiseq 2000 was evaluated by the Realomics system formula. Briefly, the adaptor reads and the low-quality reads were filtered from raw data, and the clean data were used in further alignment. Subsequently, the clean data were aligned to human IGH database and analyzed by MiXCR.Citation24 The high-quality reads were further assembled into clonotypes, correcting for PCR and sequencing errors using a heuristic multilayer clustering by VDJ tool.Citation25,Citation26 For statistical analysis, the analyses were conducted with Rscript, and Student’s t test or wilcoxon test was used, P < .05 was considered significant difference.
Results
Summary of the subjects and sequencing
To investigate the changes of TCR repertoire induced by RABV vaccination, 4 healthy volunteers were recruited for the study. The CDR3 profiles of the volunteers before and after vaccination were analyzed by high-throughput sequencing with the Illumina HiSeq2000 platform. A total of 234,744,316 clean reads were obtained after filtering, with an average of 19,562,026 reads for each sample. Detailed information about sequencing data including total clean reads, clones, unique V genes and other sequencing data is presented in .
Table 1. Basic sequencing data for 4 volunteers pre-vaccination and post-vaccination
Diversity of TCR β CDR3 repertoires before and after immunization with RABV vaccine
We analyzed the diversity of TCRBV CDR3 repertoires of the 4 healthy volunteers before and after immunization with RABV vaccine (). The frequencies of the top 20 CDR3 clonotypes increased after vaccination. Though the total clonotypes were increased after vaccination, these changes occurred in very low-frequency T cell clones (). We also observed an increase in TCR diversity after vaccination (). However, there were no statistical significance in these changes.
Figure 1. The diversity of repertoires of TCRBV before and after immunization with RABV vaccines in 4 volunteers. The volunteers were injected with anti-RABV vaccines for three times and TCR repertoires were detected by high throughput sequencing. The comparison of most frequent TCRBV before and after RABV vaccination was shown
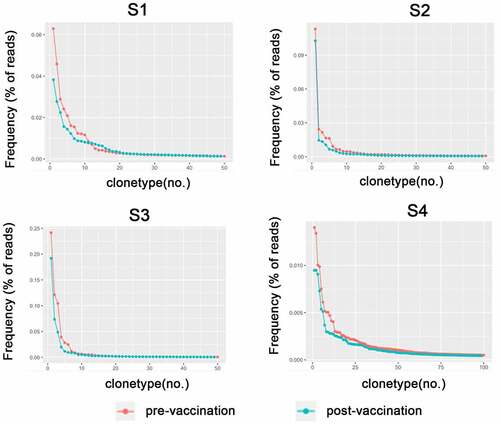
The usage of functional V, J genes and V-J pairing
We identified 47 distinct Vβ gene segments and 14 distinct Jβ gene segments in the healthy volunteers. The frequency of the TRBV genes ranged from 0.01% of TRBV7-4 to 21.25% of TRBV29-1 before vaccination. After vaccination, the usage ratio of 24 gene segments increased and the other 23 gene segments decreased. The TRBV29-1 was the most frequent Vβ gene and its frequency increased after RABV vaccination. Usage ratio of TRBJ genes ranged from 0.03% of TRBJ2-4 to 19.62% of TRBJ2-5 in subjects before vaccination. After vaccination, the frequency of 5 TCRJ gene segments increased and the other 8 gene segments decreased (). However, these changes did not reach statistical significance.
Figure 4. The usage frequencies of TRBV and TRBJ gene segments in 4 volunteers before and after RABV vaccination. (a) Frequency of TRBV segments before and after RABV vaccination. (b) Frequency of TRBJ segments before and after RABV vaccination
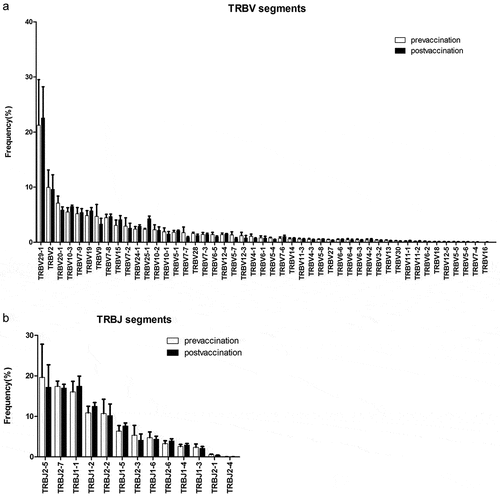
Based on the 47 TRBV and 14 TRBJ genes, there are potential 611 TRBV-TRBJ combinations. Actually, we successfully captured 469 (76.8%) and 461 (75.5%) V-J combinations before and after vaccination immunization, respectively. The data showed that the usage of V-J combinations in each volunteer varied greatly. The frequencies of many V-J combinations increased in S1, while most V-J combinations decreased in S4. In S2 and S3, the V-J combinations were generally expressed at low frequency except for some combinations, i.e. TRBV29-1/TRBJ2-5, TRBV29-1/TRBJ2-3, TRBV2/TRBJ2-2, TRBV9/TRBJ2-5. The frequency of the top V-J combinations changed after RABV vaccination, i.e. TRBV7-2/TRBJ2-2, TRBV2/TRBJ2-7, TRBV29-1/TRBJ2-5, TRBV29-1/TRBJ2-3 ().
CDR3 overlapping sequences before and after immunization with recombinant RABV vaccine
We next investigated the overlapping TCR Vβ CDR3 repertoires in the 4 healthy volunteers before and after immunization with RABV vaccine. As shown in , the overlap of unique clonotypes in the same subject before and after vaccination was bigger than that between different subjects. To be noted, the overlap clones between different subjects after RABV vaccination were intensively increased as compared that before RABV vaccination. These may reflect the similar TCR CDR3 repertoire changes induced by RABV vaccination. However, when we normalized these data by calculating the proportion of overlap clones, the differences substantially decreased.
Table 2. The overlap in the repertoires of TCRB CDR3 before and after immunization with RABV vaccines in 4 volunteers
High frequency CDR3aa sequence analysis of TCR CDR3 repertoires after immunization with RABV vaccine
We investigated the top 20 most abundant TRBV β CDR3 clonotypes among 4 healthy volunteers before and after vaccination (). Most of the CDR3 clonotypes remained stable with the similar frequency before and after vaccination. Two CDR3 clonotypes in the prevaccination dataset, i.e. CASSLEVNTEAFF and CSARVGEGTAYPYEQYF, were absent after vaccination. Another three CDR3 clonotypes, i.e. CASSESHKDTGELFF, CASSDTKNTGELFF, and CAISESGQDQHF, were in the top 30 dataset before vaccination. These clonotypes may serve as good candidates for vaccine responding clones. In addition, to further characterize the TCR repertoire, we analyzed the antigen specificity of these CDR3 clonotypes by VDJ tool. However, we were unable to find the reported antigens that were specific to these five CDR3 clonotypes.
Table 3. The top 20 high-frequency clonotypes before and after RABV vaccination
Discussion
In the present study, we investigated the human TCR β immune repertoire before and after RABV vaccination using high throughput sequencing method. Our results showed that RABV vaccination increased the CDR3 diversity, changed the V, J and V-J pairing usage patterns. We also identified 5 top frequent CDR3 clonotypes that would be good candidates for vaccine responding clones.
Previous studies have suggested that T cell-mediated responses were crucial for an efficient host immune response against RABV infection.Citation27–30 Peripheral inoculation of nude mice that defected in T cell response, with a normally apathogenic RABV resulted in a fatal outcome.Citation10 It has been demonstrated that enhancement of BBB permeability played a crucial role in the immune clearance of RABV from the CNS, by allowing the infiltration of immune effectors, such as CD4+ T cells, from the periphery into the CNS to clear viruses.Citation31 The high expand clonotypes are very important in the immunological repertoire, which may reflect the physiological responses to invasive pathogens or antigens. In the present study, to further investigate the T cell-mediated responses in human after RABV infection, we examined the TCR repertoire of PBMCs from RABV vaccine immunized volunteers. We evaluated the frequency of V, J genes and V/J pairing, as well as TCRBV clonotypes before and after RABV vaccination. Our data showed that vaccination changed the frequencies of the high-frequency clonotypes, especially the top 20 clonotypes. Meanwhile, vaccination seemed to induce a large number of low-frequency clonotypes, thus increased the TCRBV diversity. Furthermore, the V and J genes usage showed a trend of alteration after vaccination, but no significant difference was observed. We observed that overlap of TCRBV clonotypes between volunteers increased after vaccination. These results may suggest that RABV immunization induce T-cell mediated reaction.
Limitations associated with the present study warrant mention. First, because the number of samples tested was small and we were unable to compare RABV vaccine responders with non-RABV vaccine responders. thus, it is not possible to determine if these changes are RABV-specific. Second, T-cell interactions with the RABV vaccine were not clarified in this study. Furthermore, some reports suggest that there is a relationship between age and repertoire diversity. However, the participants in the present study were all the same young age, so we were unable to evaluate the age-related response to the RABV vaccine or the relationship between age and repertoire diversity during RABV vaccination. Despite these limitations, our results suggest that TCR diversity and the usage of V/J gene segments, as well as V-J pairing induced by RABV vaccination. It could potentially serve as a surrogate prediction marker for a better response to the RABV vaccine. Further studies with additional subjects are needed to confirm this possibility. In addition, while the sample size of the present work is too small for detecting correlations between anti-RABV responses and specific factors, our data may be useful for futur metadata reviews.
Conclusions
To our knowledge, this is the first report that revealed the TCRβ repertoire in human after RABV vaccine immunization. Our data suggested that TCRBV diversity increased after vaccination and clonal expansion was observed. These findings help us understand the role of T cells in RABV infection and provide a new insight into T cell receptor condition after RABV vaccination.
Disclosure of potential conflicts of interest
No potential conflicts of interest were disclosed.
Additional information
Funding
References
- Davis BM, Rall GF, Schnell MJ. Everything you always wanted to know about rabies virus (but were afraid to ask). Ann Rev Virol. 2015;2(1):451–71. doi:10.1146/annurev-virology-100114-055157.
- Rupprecht CE, Hanlon CA, Hemachudha T. Rabies re-examined. Lancet Infect Dis. 2002;2(6):327–43. doi:10.1016/S1473-3099(02)00287-6.
- Fooks AR, Banyard AC, Horton DL, Johnson N, McElhinney LM, Jackson AC. Current status of rabies and prospects for elimination. Lancet. 2014;384(9951):1389–99. doi:10.1016/S0140-6736(13)62707-5.
- Dato VM, Campagnolo ER, Long J, Rupprecht CE. A systematic review of human bat rabies virus variant cases: evaluating unprotected physical contact with claws and teeth in support of accurate risk assessments. PLoS One. 2016;11(7):e0159443. doi:10.1371/journal.pone.0159443.
- Wunner WH, Larson JK, Dietzschold B, Smith CL. The molecular biology of rabies viruses. Rev Infect Dis. 1988;10(Suppl 4):S771–784. doi:10.1093/clinids/10.Supplement_4.S771.
- Lafon M. Rabies virus receptors. J Neurovirol. 2005;11(1):82–87. doi:10.1080/13550280590900427.
- Kelly R, Strick P. Rabies as a transneuronal tracer of circuits in the central nervous system. J Neurosci Methods. 2000;103(1):63–71. doi:10.1016/S0165-0270(00)00296-X.
- Lafon M. Evasive strategies in rabies virus infection. Adv Virus Res. 2011;79:33–53.
- Katz ISS, Guedes F, Fernandes ER, Silva SDR. Immunological aspects of rabies: a literature review. Arch Virol. 2017;162(6):1–18. doi:10.1007/s00705-017-3484-0.
- Galelli A, Baloul L, Lafon M. Abortive rabies virus central nervous infection is controlled by T lymphocyte local recruitment and induction of apoptosis. J Neurovirol. 2000;6(5):359–72. doi:10.3109/13550280009018300.
- Lafon M. Modulation of the immune response in the nervous system by rabies virus. Curr Top Microbiol Immunol. 2005;289:239–58. doi:10.1007/3-540-27320-4_11.
- Roy A, Hooper DC. Lethal silver-haired bat rabies virus infection can be prevented by opening the blood-brain barrier. J Virol. 2007;81(15):7993–98. doi:10.1128/JVI.00710-07.
- Bailey DM, Roukens R, Knauth M, Kallenberg K, Christ S, Mohr A, Genius J, Storch-Hagenlocher B, Meisel F, McEneny J, et al. Free radical-mediated damage to barrier function is not associated with altered brain morphology in high-altitude headache. J Cereb Blood Flow Metab. 2006;26(1):99–111. doi:10.1038/sj.jcbfm.9600169.
- Baloul L, Camelo S, Lafon M. Up-regulation of Fas ligand (FasL) in the central nervous system: a mechanism of immune evasion by rabies virus. J Neurovirol. 2004;10(6):372–82. doi:10.1080/13550280490521122.
- Hogquist KA, Jameson SC, Heath WR, Howard JL, Bevan MJ, Carbone FR. T cell receptor antagonist peptides induce positive selection. Cell. 1994;76(1):17–27. doi:10.1016/0092-8674(94)90169-4.
- Warren RL, Freeman JD, Zeng T, Choe G, Munro S, Moore R, Webb JR, Holt RA. Exhaustive T-cell repertoire sequencing of human peripheral blood samples reveals signatures of antigen selection and a directly measured repertoire size of at least 1 million clonotypes. Genome Res. 2011;21(5):790–97. doi:10.1101/gr.115428.110.
- Van Heijst JW, Ceberio I, Lipuma LB, Samilo DW, Wasilewski GD, Gonzales AM, Nieves JL, Mr VDB, Perales MA, Pamer EG. Quantitative assessment of T cell repertoire recovery after hematopoietic stem cell transplantation. Nat Med. 2013;19(3):372–77. doi:10.1038/nm.3100.
- Jia Q, Zhou J, Chen G, Shi Y, Yu H, Guan P, Lin R, Jiang N, Yu P, Li QJ. Diversity index of mucosal resident T lymphocyte repertoire predicts clinical prognosis in gastric cancer. Oncoimmunology. 2015;4(4):e1001230. doi:10.1080/2162402X.2014.1001230.
- Woodsworth, Castellarin, and Holt. Sequence analysis of T-cell repertoires in health and disease. Genome Med. 2013;5(10):1–13. doi:10.1186/gm405.
- Chen Y, Xu Y, Zhao M, Liu Y, Gong M, Xie C, Wu H, Wang Z. High-throughput T cell receptor sequencing reveals distinct repertoires between tumor and adjacent non-tumor tissues in HBV-associated HCC. Oncoimmunology. 2016;5(10):e1219010. doi:10.1080/2162402X.2016.1219010.
- Liaskou E, Klemsdal Henriksen EK, Holm K, Kaveh F, Hamm D, Fear J, Viken MK, Hov JR, Melum E, Robins H. High-throughput T-cell receptor sequencing across chronic liver diseases reveals distinct disease-associated repertoires. Hepatology. 2016;63(5):1608–19. doi:10.1002/hep.28116.
- Chen XM, Zhang T, Qiu D, Feng JY, Jin ZY, Luo Q, Wang XY, Wu XL. Gene expression pattern of TCR repertoire and alteration expression of IL-17A gene of γδ T cells in patients with acute myocardial infarction. J Transl Med. 2018;16(1):189. doi:10.1186/s12967-018-1567-7.
- Mamedov IZ, Britanova OV, Zvyagin IV, Turchaninova MA, Bolotin DA, Putintseva EV, Lebedev YB, Chudakov DM. Preparing unbiased T-cell receptor and antibody cDNA libraries for the deep next generation sequencing profiling. Front Immunol. 2013;4:456. doi:10.3389/fimmu.2013.00456.
- Bolotin DA, Poslavsky S, Mitrophanov I, Shugay M, Mamedov IZ, Putintseva EV, Chudakov DM. MiXCR: software for comprehensive adaptive immunity profiling. Nat Methods. 2015;12(5):380–81. doi:10.1038/nmeth.3364.
- Liu X, Zhang W, Zeng X, Zhang R, Du Y, Hong X, Cao H, Su Z, Wang C, Wu J. Systematic comparative evaluation of methods for investigating the TCRÎ2 repertoire. PLoS One. 2016;11:e0152464.
- Bai X, Zhang Q, Wu S, Zhang X, Wang M, He F, Wei T, Yang J, Lou Y, Cai Z. Characteristics of tumor infiltrating lymphocyte and circulating lymphocyte repertoires in pancreatic cancer by the sequencing of T cell receptors. Sci Rep. 2015;5:13664. doi:10.1038/srep13664.
- Dorfmeier CL, Lytle AG, Dunkel AL, Gatt A, McGettigan JP. Protective vaccine-induced CD4(+) T cell-independent B cell responses against rabies infection. J Virol. 2012;86(21):11533–40. doi:10.1128/JVI.00615-12.
- Celis E, Larson J, Otvos L Jr., Wunner WH. Identification of a rabies virus T cell epitope on the basis of its similarity with a hepatitis B surface antigen peptide presented to T cells by the same MHC molecule (HLA-DPw4). J Immunol. 1990;145:305–10.
- Celis E, Miller RW, Wiktor TJ, Dietzschold B, Koprowski H. Isolation and characterization of human T cell lines and clones reactive to rabies virus: antigen specificity and production of interferon-gamma. J Immunol. 1986;136:692–97.
- Fujii H, Mannen K, Takita-Sonoda Y, Hirai K, Cruz-Abrenica MS, Kawano Y, Nishizono A, Mifune K. Target cells of cytotoxic T lymphocytes directed to the individual structural proteins of rabies virus. Microbiol Immunol. 1994;38(9):721–26. doi:10.1111/j.1348-0421.1994.tb01847.x.
- Roy A, Phares TW, Koprowski H, Hooper DC. Failure to open the blood-brain barrier and deliver immune effectors to central nervous system tissues leads to the lethal outcome of silver-haired bat rabies virus infection. J Virol. 2007;81(3):1110–18. doi:10.1128/JVI.01964-06.