ABSTRACT
Although COVID-19 vaccines have recently been approved for emergency use, search for new vaccines are still urgent, since the access of the countries, especially the poorest, to the vaccines, has shown to be slower than the necessary to rapidly control the pandemic. We proposed a novel platform for vaccine using recombinant receptor binding domain (rRBD) from Sars-Cov-2 spike protein and Neisseria meningitidis outer membrane vesicles (OMVs). The antigen preparation produced a humoral and cellular immune response. Taken together our findings suggest a good immunostimulatory patter in response to immunization with rRBD plus N. meningitidis OMV.
Introduction
Severe acute respiratory syndrome coronavirus 2 (SARS-CoV-2) is a recently identified coronavirus that causes coronavirus disease 2019 (COVID-19)Citation1 characterized by its severe ‘flu’-like symptoms that can progress to acute respiratory distress (ARDS), pneumonia, renal failure, and death.Citation2 COVID-19 was declared by WHO a public health emergency of international concern on 30 January 2020. Due to the great pathogenicity and rapid spread of SARS-Cov-2, COVID-19 became the deadliest, fastest-moving pandemic since 1918, leading to hundreds of thousands of deaths and huge economic impact.Citation3 Although the first vaccines have recently been approved for emergency use, the access of the countries, especially the poorest, to the vaccines, has shown to be slower than the necessary to rapidly reach the herd immunity and slowdown the pandemic. Thus, researches for new vaccines and adjuvants are urgent.
The spike (S) protein is a structural glycoprotein which plays a key role in the receptor recognition and cell membrane fusion process.Citation4 This protein is composed of two subunits, S1 and S2. The S1 subunit possess the receptor-binding domain (RBD) that binds to the host cell receptor angiotensin-converting enzyme 2 (ACE2) mediating viral cell entry.Citation5 Due to this characteristic, RBD is a critical target for neutralizing antibodies.Citation4
The Outer membrane vesicles (OMVs) are nano-sized spherical blebs shed by Gram-negative bacteria that reflects outer membrane composition. It is composed of bacteria surface-exposed antigens in native conformation and orientation, and immunostimulatory molecules, such as lipopolysaccharide (LPS), lipoproteins or peptidoglycans. These vesicles mimic the outside of bacteria, resembling a pathogen but, as they are non-living, lack the ability to cause associated disease and have been proposed as vaccines since their discovery.Citation6 Vaccines against Neisseria meningitidis serogroup B (MenB) based on OMVs have proved to be very useful against epidemic strains in Cuba,Citation7 NorwayCitation8 and New Zealand.Citation9 On the other hand, the 4CMenB (Bexsero) vaccine that also contains outer membrane vesicles (NZ OMV) and additionally contains three surface-exposed recombinant proteins (fHbp, NadA, and NHBA), shows important cross-reactivity with other MenB strains and it is estimated to provide 66–91% coverage against MenB strains worldwide.Citation10 Besides being approved for use against MenB around the world, the OMV-based vaccines have also been tested against other diseases, such as N. gonorrhea, Mycobacterium tuberculosis, Klebsiella pneumoniae, Salmonella and has proved to be more immunogenic than classical vaccines.Citation6
Here, we propose a preliminary study of a Covid-19 vaccine with rRBD as antigen and N. meningitidis OMV and aluminum hydroxide as adjuvants, with two intramuscular and two intranasal doses.
The critical role of S protein to Sars-Cov-2 cell invasion makes this protein an obvious vaccine candidate.Citation11 Thus, the S protein, including RBD, is actually, the main target to anti-COVID-19 vaccines development,Citation12 and its effectivity as immunogen has proved to be satisfactory even in phase III studies, with different effectiveness, depending on the vaccine platform used.Citation13–15 Besides the choice of the antigenic target, the choice for a good adjuvant is crucial to vaccine development. OMVs from Gram-negative bacteria have shown to have adjuvant properties, since due to its membrane composition, it retains several pathogen-associated molecular pattern (PAMPs), recognized by pattern recognition receptors (PPRs) in antigen presenting cells (APC), what is crucial for a strong adaptive immune response.Citation16 Its application to deliver heterologous vaccine antigens has been demonstrated to bacterial, viral, parasitic, and even cancer antigens.Citation17–20
Aluminum hydroxide was chosen as a compound of our vaccine design, since it has been used as an adjuvant for the licensed vaccines containing OMV, such as Bexsero®Citation21 and its use is allowed in humans. The intramuscular route of administration is the choice for inactivated vaccines that contain aluminum hydroxide in the preparationCitation22 Boost with intranasal antigenCitation23 free of aluminum hydroxide aimed to verify whether the animals would intensify or modulate the response after this administration.
In our study, although immunization with rRBD alone induced a small production of sera with IgG directed toward SARS-CoV-2, we observed an increase in IgG production when rRBD was mixed to OMV plus aluminum hydroxide, in both, prep1 and prep2, 15 and 45 days after the first immunization dose (). As a versatile delivery system, OMVs can be used in a simple mixture with the antigen,Citation24 chemically conjugated to themCitation25 or can be genetically engineered to express the antigen proteins.Citation26 Although there are some evidence that chemical conjugation improves the OMV adjuvant property,Citation25 the simple mixture of the OMV with antigens, as we have done in this work, is capable of inducing serum production of IgG heterologous to OMV, that is, specific to the vaccine antigen.Citation17,Citation18,Citation24,Citation27 We have recently demonstrated that this vaccine schedule induces anti-RBD IgG with intermediary avidity,Citation28 what is not completely surprising, since, even natural infection has shown to fail in inducing high avidity antibody directed toward SARS‐CoV‐2 antigens, probably due to incomplete avidity maturation.Citation29 Since the interaction between SARS-CoV-2 and human ACE2 possesses strong affinity, the induction of high avidity anti- SARS-CoV-2 antibodies are desirable and can be necessary to truly block SARS-CoV-2 adherence and infectionCitation30 and can be a challenge. We also observed IgA production, but only 37 days after the first immunization dose, i.e., only after the first boost with intranasal antigen for both prep1 and prep2 immunization (), what is interesting since IgA seems to dominate the early neutralizing antibody response to SARS-CoV-2Citation31 We also verified the anti-rRBD IgG production in the supernatant of the splenocytes stimulated in vitro with rRBD. In this experiment, IgG production was higher in the groups immunized with prep1 and prep2, compared to the animals immunized with rRBD alone. Although the total amount of antibody in animals immunized with prep1 was slightly higher than in animals immunized with prep2, no statistical significance was observed ().
Figure 1. Immune response after immunization with rRBD from Sars-Cov-2 with aluminum hydroxide plus OMV from Neisseria meningitidis strains B:8:P1.6 (prep1) or C:2a.P1.5 (prep2). The IgG production in sera collected 15 and 45 days after the first immunization dose was measured by ELISA and can be observed in (a). The IgA production was accessed 15, 30, 37 and 45 days after the first immunization dose (b). Additionally, 45 days after the first immunization dose, the animals were euthanized and the splenocytes were cultured under rRBD stimuli. The IgG production in culture supernatant was evaluated by ELISA (c) and the number of cells/106 splenocytes producing IFN-γ (d) or IL-17 (e) was evaluated through ELISpot
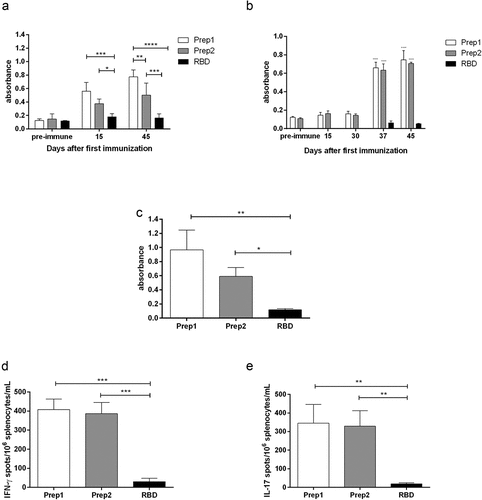
We found an increase in IFN-γ producing cells in splenocytes culture from animals immunized with prep1 and prep2. The animals immunized with preparations containing OMV produced around 13 times more IFN-γ producing cells in splenocytes culture, than animals immunized with rRBD alone (). This finding is in accordance to others, that also find higher number of IFN-γ-producing cellsCitation32 or higher levels of IFN-γ in the seraCitation33 or culture supernatantCitation34 of the animals receiving OMV in their vaccine preparations. We also found an increase in IL-17 producing cells in splenocytes derived from animals immunized with prep1 and prep2 after stimuli with rRBD (). Kim et al.,Citation34 although used OMV as a homologous vaccine, also found an increase in IL-17 production by culture splenocytes. These authors suggest the protection against bacteria-induced lethality occurs via Th1 and Th17 cell responses, since they also found increase in IFN-γ production. Il-17 production was elicited after immunization with SARS-CoV-2 pre-fusion stabilized (S-2P) spike protein adjuvanted with CAF®01 but not with aluminum hydroxide, nor a squalene-based oil-in-water emulsion system.Citation35 Although IL-17 has been strongly associated with immunopathology, it also has an important role in host defense.Citation36 This cytokine plays a key role in defense from both extracellular bacteria and viruses that infect airway mucous membranes, regulates homeostasis, and contributes to the repair of epithelial cells, damaged previously by an extracellular inflammatory stimulus.Citation37 The exact role of IL-17 against Sars-CoV-2 is not completely elucidated. Its production seems to be enhanced in Sars-CoV-2 infected people with heterogeneous commitment degrees, but its association with disease severity is not clear.Citation38,Citation39
Although our data are preliminary, and more studies are necessary specially to improve avidity of anti-SARS-CoV-2 antibodies, taken together our findings suggest a good immunostimulatory pattern in response to immunization with rRBD plus N. meningitidis OMV, showing a promising platform to anti-COVID-19 vaccine development. Additional studies with animal models as OMV without adjuvants or others are required to assess the potential safety and effectiveness of this vaccine approach in humans.
Methodology
Outer membrane vesicles (OMV) from N. meningitidis strains, serogroup B: (B:8:P1.6) and serogroup C (C:2a.P1.5) were obtained as previously described.Citation40 These two strains have been used for our group to produce OMVs with protein compounds slightly different (data not shown), so they deserved to be tested in separated vaccine preparations. Moreover, they belonged to two different serogroups, B and C. Five Swiss female mice per group were immunized with 3 µg/animal of recombinant receptor-binding domain (rRBD) from SARS-Cov-2 protein S complexed to aluminum hydroxide at 0.1 mM plus 10 μg/ml of OMV from N. meningitidis strains B:8:P1.6 (prep1) or C:2a.P1.5 (prep2) or rRBD alone, as control. The OMVs were detoxified as previously described.Citation41 Animals were immunized with two doses of antigenic preparations intramuscullarlly (IM), 15 days apart and two doses intranasally (IN), 7 days apart.Citation28 The intranasal inoculation was performed with RBD plus OMV, without aluminum hydroxide. Blood samples were obtained by retro-orbital plexus puncture in anesthetized animals, 15 and 45 days after the first immunization dose. Forty-five days after immunization the animals were anesthetized with Dopalen and Anasedan (CEVA, Brazil), sacrificed by cervical dislocation and the spleens were collected for the ELISpot assay. The supernatant from splenocyte culture was collected to IgG measurement. All procedures involving animals were performed following the guidelines of the Brazilian Code for the Use of Laboratory Animals and approved by the ethics committee of CEUA-IAL/Pasteur (protocol number 03/2012 extended to 2022).
Enzyme-Linked Immunosorbent assay (ELISA) from mice sera or from splenocyte supernatant was performed as previously described,Citation40 except for the adsorption step, in which polystyrene plates (Maxisorp Nunc – Thermo Fisher Scientific, Rochester, NY, USA) were adsorbed with 100 μL/well of a solution containing 1 μg/mL of rRBD diluted in 0.05 M carbonate-bicarbonate buffer pH 9.6, and incubated overnight at 4°C, in a humid chamber. The secondary antibodies (dilution) used were anti-mouse IgG (γ) (1:5.000) or IgA(α)(1:2000) (Kirkegaard & Per Laboratories, KPL, Gaithersburg, MD, EUA).
The Enzyme-Linked Immunosorbent Spot (ELISpot) assay was also performed as described by Trzewikoswki de Lima et al.,Citation40 with minor modifications. The splenocytes derived from immunized animals were added to the wells at 1 × 106 cells/mL and the cells were stimulated with 2 µg/mL of rRBD, 10 µg/mL concanavalin A (ConA) (Sigma Chemical Company, St. Louis, MO, USA) or added without stimulus.
The significance of the results was evaluated by ANOVA with Tukey post test. P values were considered significant when p ≤ 0.05.
Disclosure of potential conflicts of interest
No potential conflicts of interest were disclosed.
Acknowledgments
The research was supported with grants by FAPESP (18/04202-0 e 12/15568-0). The receptor-binding domain (RBD) antigen was kindly donated by Dr. Florian Krammer, from Icahn School of Medicine, Mount Sinai, Nova York, NY, EUA.
Additional information
Funding
References
- Sun P, Lu X, Xu C, Sun W, Pan B. Understanding of COVID-19 based on current evidence. J Med Virol. 2020;92(6):548–51. doi:10.1002/jmv.25722.
- Harrison AG, Lin T, Wang P. Mechanisms of SARS-CoV-2 transmission and pathogenesis. Trends Immunol. 2020;41(12):1100–15. doi:10.1016/j.it.2020.10.004.
- Nicola M, Alsafi Z, Sohrabi C, Kerwan A, Al-Jabir A, Iosifidis C, Agha M, Agha R. The socio-economic implications of the coronavirus pandemic (COVID-19): a review. Int J Surg. 2020;78:185–93. doi:10.1016/j.ijsu.2020.04.018.
- Huang Y, Yang C, Xu X-F, Xu W, Liu S-W. Structural and functional properties of SARS-CoV-2 spike protein: potential antivirus drug development for COVID-19. Acta Pharmacol Sin. 2020;41(9):1141–49. doi:10.1038/s41401-020-0485-4.
- Letko M, Marzi A, Munster V. Functional assessment of cell entry and receptor usage for SARS-CoV-2 and other lineage B betacoronaviruses. Nat Microbiol. 2020;5(4):562–69. doi:10.1038/s41564-020-0688-y.
- Micoli F, MacLennan CA. Outer membrane vesicle vaccines. Semin Immunol. 2020;50:101433. doi:10.1016/j.smim.2020.101433.
- Sierra GV, Campa HC, Varcacel NM, Garcia IL, Izquierdo PL, Sotolongo PF, Casanueva GV, Rico CO, Rodriguez CR, Terry MH, et al. Vaccine against group B Neisseria meningitidis: protection trial and mass vaccination results in Cuba. NIPH Ann. 1991;14(2):195–207. discussion 8–10.
- Bjune G, Høiby EA, Grønnesby JK, Arnesen Ø, Fredriksen JH, Lindbak A-K, Nøkleby H, Rosenqvist E, Solberg LK, Closs O, et al. Effect of outer membrane vesicle vaccine against group B meningococcal disease in Norway. Lancet. 1991;338(8775):1093–96. doi:10.1016/0140-6736(91)91961-S.
- Arnold R, Galloway Y, McNicholas A, O’Hallahan J. Effectiveness of a vaccination programme for an epidemic of meningococcal B in New Zealand. Vaccine. 2011;29(40):7100–06. doi:10.1016/j.vaccine.2011.06.120.
- O’Ryan M, Stoddard J, Toneatto D, Wassil J, Dull PM. A multi-component meningococcal serogroup B vaccine (4CMenB): the clinical development program. Drugs. 2014;74(1):15–30. doi:10.1007/s40265-013-0155-7.
- Zhang Y, Kutateladze TG. Molecular structure analyses suggest strategies to therapeutically target SARS-CoV-2. Nat commun. 2020;11(1):2920.
- WHO. Draft landscape and tracker of COVID-19 candidate vaccines: WHO; 2021 [accessed 2021 Aug 2]. https://www.who.int/publications/m/item/draft-landscape-of-covid-19-candidate-vaccines.
- Polack FP, Thomas SJ, Kitchin N, Absalon J, Gurtman A, Lockhart S, Perez JL, Pérez Marc G, Moreira ED, Zerbini C, et al. Safety and efficacy of the BNT162b2 mRNA Covid-19 vaccine. N Engl J Med. 2020;383(27):2603–15. doi:10.1056/NEJMoa2034577.
- Voysey M, Clemens SAC, Madhi SA, Weckx LY, Folegatti PM, Aley PK, Angus B, Baillie VL, Barnabas SL, Bhorat QE, et al. Safety and efficacy of the ChAdOx1 nCoV-19 vaccine (AZD1222) against SARS-CoV-2: an interim analysis of four randomised controlled trials in Brazil, South Africa, and the UK. Lancet. 2021;397(10269):99–111.
- Logunov DY, Dolzhikova IV, Shcheblyakov DV, Tukhvatulin AI, Zubkova OV, Dzharullaeva AS, Kovyrshina AV, Lubenets NL, Grousova DM, Erokhova AS, et al. Safety and efficacy of an rAd26 and rAd5 vector-based heterologous prime-boost COVID-19 vaccine: an interim analysis of a randomised controlled phase 3 trial in Russia. Lancet. 2021;397(10275):671–81. doi:10.1016/S0140-6736(21)00234-8.
- Mancini F, Rossi O, Necchi F, Micoli F. OMV vaccines and the role of TLR agonists in immune response. Int J Mol Sci. 2020;21(12):4416. doi:10.3390/ijms21124416.
- Koeberling O, Giuntini S, Seubert A, Granoff DM. Meningococcal outer membrane vesicle vaccines derived from mutant strains engineered to express factor H binding proteins from antigenic variant groups 1 and 2. Clin Vaccine Immunol: CVI. 2009;16(2):156–62. doi:10.1128/CVI.00403-08.
- Watkins HC, Rappazzo CG, Higgins JS, Sun X, Brock N, Chau A, Misra A, Cannizzo JPB, King MR, Maines TR, et al. Safe recombinant outer membrane vesicles that display M2e elicit heterologous influenza protection. Mol Therapy: J Am Soc Gene Therapy. 2017;25(4):989–1002. doi:10.1016/j.ymthe.2017.01.010.
- Scaria PV, Rowe CG, Chen BB, Muratova OV, Fischer ER, Barnafo EK, Anderson CF, Zaidi IU, Lambert LE, Lucas BJ, et al. Outer membrane protein complex as a carrier for malaria transmission blocking antigen Pfs230. NPJ Vaccines. 2019;4(1):24. doi:10.1038/s41541-019-0121-9.
- Grandi A, Tomasi M, Zanella I, Ganfini L, Caproni E, Fantappiè L, Irene C, Frattini L, Isaac SJ, König E, et al. Synergistic protective activity of tumor-specific epitopes engineered in bacterial outer membrane vesicles. Front Oncol. 2017;7:253. doi:10.3389/fonc.2017.00253.
- FDA. BEXSERO® US prescribing information [internet]. FDA; 2015. [uploaded 2015; cited 2021 Mar 23]. Available from https://www.fda.gov/media/90996/download.
- Cook IF. Evidence based route of administration of vaccines. Hum Vaccin. 2008;4(1):67–73. doi:10.4161/hv.4.1.4747.
- Guy B, Fourage S, Hessler C, Sanchez V, Millet MJ. Effects of the nature of adjuvant and site of parenteral immunization on the serum and mucosal immune responses induced by a nasal boost with a vaccine alone. Clin Diagn Lab Immunol. 1998;5(5):732–36. doi:10.1128/CDLI.5.5.732-736.1998.
- Sardiñas G, Reddin K, Pajon R, Gorringe A. Outer membrane vesicles of Neisseria lactamica as a potential mucosal adjuvant. Vaccine. 2006;24(2):206–14. doi:10.1016/j.vaccine.2005.07.064.
- Micoli F, Alfini R, Di Benedetto R, Necchi F, Schiavo F, Mancini F, Carducci M, Palmieri E, Balocchi C, Gasperini G, et al. GMMA is a versatile platform to design effective multivalent combination vaccines. Vaccine. 2020;8(3):540. doi:10.3390/vaccines8030540.
- Chen DJ, Osterrieder N, Metzger SM, Buckles E, Doody AM, DeLisa MP, Putnam D. Delivery of foreign antigens by engineered outer membrane vesicle vaccines. Proc Natl Acad Sci. 2010;107(7):3099–104. doi:10.1073/pnas.0805532107.
- Lee DH, Kim S-H, Kang W, Choi YS, Lee S-H, Lee S-R, You S, Lee HK, Chang K-T, Shin E-C, et al. Adjuvant effect of bacterial outer membrane vesicles with penta-acylated lipopolysaccharide on antigen-specific T cell priming. Vaccine. 2011;29(46):8293–301. doi:10.1016/j.vaccine.2011.08.102.
- Gaspar EB, De Gaspari E. Avidity assay to test functionality of anti-SARS-Cov-2 antibodies. Vaccine. 2021;39(10):1473–75. doi:10.1016/j.vaccine.2021.02.003.
- Bauer G, Struck F, Schreiner P, Staschik E, Soutschek E, Motz M. The challenge of avidity determination in SARS-CoV-2 serology. J Med Virol. 2021;93(5):3092–3104. doi:10.1002/jmv.26863.
- Khatri I, Staal FJT, Van Dongen JJM. Blocking of the high-affinity interaction-synapse between SARS-CoV-2 spike and human ACE2 proteins likely requires multiple high-affinity antibodies: an immune perspective. Front Immunol. 2020;11(2258). doi:10.3389/fimmu.2020.570018.
- Sterlin D, Mathian A, Miyara M, Mohr A, Anna F, Claër L, Quentric P, Fadlallah J, Devilliers H, Ghillani P. IgA dominates the early neutralizing antibody response to SARS-CoV-2. Sci Trans Med. 2021;13(577). doi:10.1126/scitranslmed.abd2223.
- Watkins HC, Pagan CL, Childs HR, Posada S, Chau A, Rios J, Guarino C, DeLisa MP, Whittaker GR, Putnam D, et al. A single dose and long lasting vaccine against pandemic influenza through the controlled release of a heterospecies tandem M2 sequence embedded within detoxified bacterial outer membrane vesicles. Vaccine. 2017;35(40):5373–80. doi:10.1016/j.vaccine.2017.08.013.
- Xu K, Zhao Q, Wen X, Wu R, Wen Y, Huang X, Huang Y, Yan Q, Han X, Ma X, et al. A trivalent Apx-fusion protein delivered by E. coli outer membrane vesicles induce protection against Actinobacillus pleuropneumoniae of serotype 1 and 7 challenge in a murine model. PLoS One. 2018;13(1):e0191286. doi:10.1371/journal.pone.0191286. PMID: 29373591; PMCID: PMC5786296
- Kim OY, Hong BS, Park K-S, Yoon YJ, Choi SJ, Lee WH, Roh T-Y, Lötvall J, Kim Y-K, Gho YS, et al. Immunization with escherichia coli outer membrane vesicles protects bacteria-induced lethality via Th1 and Th17 cell responses. J Immunol. 2013;190(8):4092–102. doi:10.4049/jimmunol.1200742.
- Wørzner K, Sheward DJ, Schmidt ST, Hanke L, Zimmermann J, McInerney G, Karlsson Hedestam GB, Murrell B, Christensen D, Pedersen GK, et al. Adjuvanted SARS-CoV-2 spike protein elicits neutralizing antibodies and CD4 T cell responses after a single immunization in mice. EBioMed. 2021;63:103197. doi:10.1016/j.ebiom.2020.103197.
- Veldhoen M. Interleukin 17 is a chief orchestrator of immunity. Nat Immunol. 2017;18(6):612–21. doi:10.1038/ni.3742.
- Casillo GM, Mansour AA, Raucci F, Saviano A, Mascolo N, Iqbal AJ, Maione F. Could IL-17 represent a new therapeutic target for the treatment and/or management of COVID-19-related respiratory syndrome? Pharmacol Res. 2020;156:104791. doi:10.1016/j.phrs.2020.104791.
- Ghazavi A, Ganji A, Keshavarzian N, Rabiemajd S, Mosayebi G. Cytokine profile and disease severity in patients with COVID-19. Cytokine. 2021;137:155323. doi:10.1016/j.cyto.2020.155323.
- Liu Y, Zhang C, Huang F, Yang Y, Wang F, Yuan J, Zhang Z, Qin Y, Li X, Zhao D, et al. Elevated plasma levels of selective cytokines in COVID-19 patients reflect viral load and lung injury. Natl Sci Rev. 2020;7(6):1003–11. doi:10.1093/nsr/nwaa037.
- Trzewikoswki De Lima G, Rodrigues TS, Portilho AI, Correa VA, Gaspar EB, De Gaspari E. Immune responses of meningococcal B outer membrane vesicles in middle-aged mice. Pathog Dis. 2020;78(5). doi:10.1093/femspd/ftaa028.
- De Oliveira Santos FA, Lincopan N, De Gaspari E. Evaluation of intranasal and subcutaneous route of immunization in neonatal mice using DODAB-BF as adjuvant with outer membrane vesicles of Neisseria meningitis B. Immunobiol. 2018;223(12):750–60. doi:10.1016/j.imbio.2018.07.021.