ABSTRACT
Animal studies have reported the use of different opioid-vaccine formulations with relative success These studies have suggested that new opioid-vaccine formulations are required, which are capable of triggering a robust humoral response. One strategy that has been used is the co-administration of two or more vaccines with different but complementary properties, which are capable of generating a robust immune response. We have developed two formulations of opioid-vaccine, the M6-TT, and M3-TT, which generate a robust immune response capable of recognizing heroin and morphine. In this work, we evaluate the combination of two vaccine formulations, which we call the M3/6-TT vaccine, to elicit a robust immune response and protection against heroin and morphine. Balb/c mice were immunized simultaneously with M6-TT vaccine and with M3-TT vaccine. A solid-phase antibody-capture ELISA was used for monitoring antibody titer responses after each booster dose in vaccinated animals. The study used tail-flick and hot-plate testing to evaluate the antinociceptive effects induced by heroin or morphine. Immunization with M3-TT and M6-TT vaccine elicits a robust immune response with an antibody titer of 1: 590 000 able to recognize heroin and morphine. These antibodies are capable of reducing the antinociceptive effects induced by doses of up to 40 mg/Kg. of morphine or 10 mg/kg of heroin. This suggests that the combination of two vaccine formulations that generate antibodies with different but complementary characteristics would be a new therapeutic strategy aimed at reducing drug relapses.
Introduction
Several laboratories in the world have developed various pharmacotherapies aimed at reducing opioid drug relapse.Citation1–3 The results of their use were not very successful, due to the severe side effects that these cause.Citation4–6 It was reported that active immunization would be a useful therapy to maintain a long-term drug-abstinence to various drugs of abuse.Citation7–12 These studies have suggested that for an opioid vaccine to be successful, it requires new opioid-vaccine formulations capable of triggering a robust humoral response, wherein the antibodies are capable of recognizing multiple opioid-drugs structurally related.Citation13–15
To date, various vaccine models have been developed aimed to combat opioid drug addiction, including the use of different carrier proteins, haptens, and adjuvants.Citation16–21 Immunization with the morphine-6-monoacetyl-Gly4-KLH vaccine (M6M-KLH), the morphine-6-glutaril-KLH (M6G-KLH), morphine-6-succinyl vaccine (M6S-KLH), and the morphine-6-hemisuccinil vaccine (M6H-KLH) generated antibody titers about 1:160 000; in all cases the antibodies were able to recognize mainly morphine,Citation13,Citation18–22 but they were able to decrease heroin or morphine self-administration, heroin-induced place preference, and heroin- or morphine-induced dopamine release.Citation13,Citation18–22 On the other hand, the morphine-6-acetyl-HAP (6-AcMORHAP-TT) vaccine and the heroin-TT (H-TT) vaccine generated antibody titers of approximately 1:300,000, which decreased heroin-induced antinociception.Citation23–25 In contrast, the 3-acetamide-KLH vaccine produced antibodies with greater specificity for heroin, but the antibody titers were approximate 1:14 000.Citation17
We recently reported the effect of immunization of mice with vaccines 3-0-carboxymethyl-morphine-tetanus toxoid (M3-TT) and morphine-6-hemisuccinate-tetanus toxoid (M6-TT).Citation13,Citation14,Citation26 The antibodies generated by these vaccines were able to recognize with specificity similar to heroin and its metabolites, being capable of reducing heroin or morphine self-administration.Citation13,Citation14 But antibody titers generated by immunization with the M3-TT vaccine were significantly higher, 1: 480 000Citation14,Citation27 than those shown by the M6-TT vaccine.
However, immunization with the combination of two or more formulations capable of generating an immune response against heroin or morphine has not been reported yet. Stowe et al. reported immunization with a 1:1 mixture with the two immunoconjugates that comprise a dynamic vaccine to generate a heterologous immune response for heroin and their metabolites.Citation20,Citation21 However, immunization with the mixture did not improve antibody titers, 1: 39 000, or the ability of antibodies to recognize heroin and their metabolites, nor improved the ability of antibodies to block the antinociceptive effect of 1 mg/kg of heroin, compared to the individual conjugates.Citation20,Citation21
Pioneering studies conducted by Pravetoni et al., demonstrated that co-administration of two or more opioid vaccines concurrently target multiple abusable opioids without compromising the immunogenicity or efficacy of the individual components.Citation27 They reported that the co-administration of the M6M-KLH vaccine and the OXY-KLH vaccine generated antibody titers against heroin and oxycodone, capable of reducing the levels of both drugs in the brain.Citation27 Later, Townsend et al reported the co-administration of the heroin-CRM and fentanyl-CRM vaccines generated titers of high-affinity antibodies to both fentanyl and heroin sufficient to decrease the antinociceptive potency of fentanyl, heroin, and fentanyl/heroin mixture.Citation28 These results suggest that the combination of two vaccines enhances the efficacy of the individual vaccines.
In this sense, in this paper, we evaluate the combination of two vaccine formulations to elicit a robust immune response and protection against heroin and morphine. Balb/c mice were immunized simultaneously with the M6-TT vaccine, which confers highly specific antibodies,Citation13,Citation14 combined with the M3-TT vaccine which triggers a robust immune response to observing a possible synergistic effect on elicited immune response, increasing neutralizing antibody titers and conferring protection against lethal heroin doses.Citation14,Citation26 The results show that immunization with the combination of M3-TT and M6-TT induced a robust immune response capable of protecting against very high doses of heroin or morphine.
Materials and methods
Subjects
Because females typically develop higher antibody responses,Citation29,Citation30 adult female mice 8–10 weeks of age (n = 20–25 g) were used in all experimental procedures. Animals were housed individually and kept in the Vivarium under a temperature-controlled environment (22°C) and a 12 h light/dark cycle (lights on at 7:00 A.M.). In-house animals received water ad libitum and maintained with 25 g/day of standard rat chow (Harlan, USA) during the experiments. All experimental procedures occurred during the light cycle between 9:30 A.M. and 5:00 P.M. Animal studies and standards followed the Principles of Laboratory Animal Care as outlined by the National Institutes of Health USA, and were approved by the Animal Care and Bioethics Committee of the National Institute of Psychiatry in Mexico City.
Drugs
Heroin HCL was kindly provided by the Mexican government under strict regulatory controls (NOM-028-SSA2-2009; COFEPRIS). Morphine sulfate ampoules were purchased from Tecnofarma SA CV. México, under strict regulatory permissions and official surveillance of drug used in animals (NOM = 004–2003). The morphine sulfate and heroin chlorohydrate were diluted in saline solution and filtered through a 25 µm syringe filter (Fisher Scientific, Pittsburgh, PA) before intraperitoneal (i.p.) administration at 100 μl. The solutions were kept at 4°C before use and were freshly prepared before administration into the mice.
Synthesis of the M6-TT vaccine
The method to synthesize the M6-TT vaccine and the M-6-H BSA conjugate was similar to that previously reported.Citation13,Citation14,Citation31–34
Synthesis of the M3-TT vaccine
Synthetic protocols and procedures previously reported in the literature were used for the synthesis of the M3TT and M-3-H-BSA conjugate.Citation13,Citation14,Citation35,Citation36
Immunization schedule
The immunization schedule was based on that previously reported.Citation13,Citation14,Citation31 In total, 264 mice were immunized with the M3-TT vaccine, 264 mice were immunized with the M6-TT vaccine and 264 mice were immunized with the M3/6-TT vaccine adsorbed to an aluminum hydroxide gel adjuvant (Pierce, Rockford, IL, USA) and 264 mice were immunized with TT vaccine adsorbed to an aluminum hydroxide gel adjuvant. Each animal was injected subcutaneously (s.c.) at four sites over the shoulders bilaterally (two inoculations/side), for a total dose of M3-TT, or M6-TT vaccine/adjuvant of 100 μg/1 mg aluminum hydroxide (≈100 μg/inoculation/animal/booster). The M3/6-TT vaccine was generated by the simultaneous immunization of the M3-TT, and M6-TT, vaccines in a 1: 1 ratio. Subsequently, 6 booster injections were administered using the same unit dose and adjuvant, over 14–16 weeks (once every 14 days). Control mice received TT injections at the same time as vaccinated mice. Fourteen days after each booster, the mice were invariably bled and sera were collected and frozen at −20 ◦C until use.
Determination of serum antibody titers via ELISA
The ELISA test was performed as reported on that previously reported.Citation13,Citation14,Citation31 Briefly, a solid-phase antibody capture ELISA was used to monitor antibody titer responses after each booster in vaccinated animals. ELISA plates were coated with morphine-BSA conjugate, and a range of serial dilutions of antisera was added in triplicate. Biotin-labeled anti-mouse secondary antibody (Jackson Immunoresearch, West Grove, PA, USA), coupled to an OPD system (Sigma–Aldrich, St. Louis, MO, USA) as chromogenic substrate, was used to detect immunopositive signals. Antibody titers were initially defined as the inverse of the serum dilution that gave 50% of the maximal response.
Behavioral procedure
Tail-Flick Procedure
A tail-flick test was performed according to a method described elsewhere,Citation37,Citation38 using a tail-flick apparatus (Model 37360; Ugo Basile, Comerio VA, Italy). The mice were gently held by the tail and placed on the apparatus to measure the latency of the tail-flick response.
Each animal was placed in a restrainer with its tail outside. The tail (distal third) was exposed to a source of infrared radiation. The animal flicked its tail away from the source of heat on feeling pain. The tail-flick response was elicited by applying radiant heat (50°C) on the dorsal surface of the tail. Baseline latency for the tail-flick was recorded at the beginning of the experiment. To avoid tissue damage, exposure to the thermal stimulus was limited to 10 s (cutoff time). All behavioral testing was conducted by the same experimenter. After each experimental session, the mice were placed back in their stay boxes and the tail-flick apparatus was cleaned.
Hot Plate- Procedure
The experimental apparatus consisted of a solid aluminum plate (Ugo Basile 7280 Varese, Italy) that was heated and maintained at a constant temperature of 50 ± 0.5°C and continuously monitored by an onboard module with a digital display and by an additional digital thermometer. Additionally, the device is connected to a manually operated timer that records the amount of time the mouse spends on the heated surface before showing signs of nociception (e.g. jumping, paw licks). The heated surface was confined by a transparent, removable Perspex cylinder (diameter ¼ 20 cm; height ¼ 40 cm).
The hot-plate test was carried out according to the method described by Chrubasik et al., 1993, with some modifications. To minimize possible transfer effects and avoid potential visual or olfactory influences, mice were moved to the testing room in their home cages for three days and allowed to acclimate for 30 min at hot-plate apparatus. Mice were placed separately on the heated surface, and the latency of nociceptive responses, such as licking of the hind paws, was recorded with a stopwatch.
The cutoff time of 30 s was chosen to avoid tissue injury. Latency was recorded for 30 min following administration of the treatments. The behavioral measures were scored by trained observers blind to the experimental conditions. After each observation, the cylinder and plate surface were carefully cleaned with ethyl alcohol (70%). All efforts were made to minimize the number of animals used and their suffering.
Experimental Design: Experiment 1
Experiment 1 sought to characterize the anti-heroin/morphine antibody titers produced by the M3/6-TT vaccine. For this, we used 32 female Balb-c mice, which were divided into 4 experimental groups (n = 8). Each group received a different pharmacological treatment ()). The experiment consisted of the immunization phase with a duration of 84 days.
Figure 1. Antibody titer responses in mice immunized with the M3-TT, the M6-TT, and the M3/6-TT vaccines. (a) Experiment timeline. (b) Serum samples were collected 14 days after each immunization. Mean titers (± S.E.M.). *P < .01 Significant effects from the second booster compared to the 1st booster, &P < .01 Significant effects entre M3/6-TT vaccine compared M3-TT and M6-TT vaccine. #P < .01 Significant effects entre M3-TT vaccine compared M6-TT vaccine as determined by repeated-measures two-way ANOVA followed by Tukey’s test
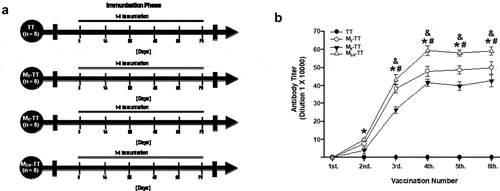
After three days of habituation to the laboratory, the mice (n = 32) were randomly assigned to the TT (n = 8), M3-TT (n = 8), M6-TT (n = 8), and M3/6-TT (n = 8) groups. During the immunization phase, the mice of the TT groups received immunization with the TT vaccine. In contrast, the animals in the M3-TT, M6-TT, and M3/6-TT group received vaccination with the M3-TT, M6-TT, and M3/6-TT vaccine, respectively. 14 days after each immunization serum samples were taken to assess antibody titers. Both groups received 6 immunizations, one immunization every 14 days.
Experimental Design: Experiment 2
Experiment 2 sought to determine the efficacy of the antibodies generated by the M3/6-TT vaccine in preventing the antinociception induced by different doses of morphine. In this experiment, 512 female Balb-c mice were used, which were divided into two groups; one group of mice was used in the tail-flick studies (n = 256) and another group was used in the hot-plate test (n = 256). The 256 Balb-c mice used in the tail-flick test or the hot-plate test were randomly subdivided into 32 experimental groups (n = 8). Each group received a different pharmacological treatment.
For the tail-flick test, the experiment consisted of two phases. These were phase I, referred to as the baseline recording phase, which included 3 assessments; and phase II referred to as the evaluation phase of the morphine-induced antinociceptive effects, which included 1 assessment ()).
Figure 2. The morphine-treated groups had a dose-dependent increase in tail-flick and hot-plate latency. (a) Experiment timeline. Vaccinated mice administered with 1, 10, 20, or 40 mg/kg morphine had an attenuation in morphine-induced tail-flick (b) or hot-plate (c) latency. Mean tail-flick or hot-plate latency (± S.E.M.). *P < .01 significant effects on tail-flick or hot-plate latency in the TT + MOR, the M3-TT-MOR, the M6-TT-MOR, and M3/6-TT-MOR groups compared to saline-treated groups. ** P < .01 Significant effects on tail-flick or hot-plate latency in the TT-MOR groups dosed with 1, 10, or 20 mg/kg morphine compared to the M3-TT-MOR, the M6-TT-MOR, and M3/6-TT-MOR groups. & P < .01 Significant effects on tail-flick or hot-plate latency in the TT-MOR−10mg M3-TT-MOR−10mg, M6-TT-MOR−10mg, and M3/6-TT-MOR−10mg, groups compared to the TT-MOR-1mg, M3-TT-MOR−1mg, M6-TT-MOR−1mg, and M3/6-TT-MOR−1mg groups. # P < .01 Significant effects on tail-flick or hot-plate latency TT-MOR−20mg, M3-TT-MOR−20mg, M6-TT-MOR−20mg, and M3/6-TT-MOR−20mg groups compared to the TT-MOR-10mg, M3-TT-MOR−10mg, M6-TT-MOR−10mg and, M3/6-TT-MOR−10mg groups. ¤ P < .01 Significant effects on tail-flick or hot-plate latency in the TT-MOR−40mg, M3-TT-MOR−40mg, M6-TT-MOR−40mg, and M3/6-TT-MOR−40mg groups compared to the TT-MOR-20mg, M3-TT-MOR−20mg, M6-TT-MOR−20mg, and, M3/6-TT-MOR−20mg groups, as determined by two-way ANOVA followed by Tukey’s test
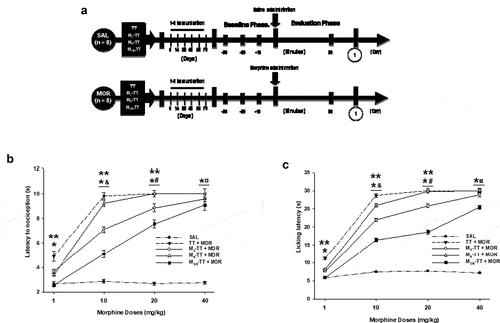
After three days of habituation in the room where the tail-flick test would be performed, baseline latency was recorded for mice from all the experimental groups. The recording took place at three moments: 30, 20, and 10 minutes before the administration of the drug.
During phase II of the experiment, the mice of the saline groups (TT + SAL-1mg, TT + SAL-10mg, TT + SAL-20mg, TT + SAL-40mg, M3-TT + SAL-1mg, M3-TT + SAL-10mg, M3-TT + SAL-20mg, M3-TT + SAL-40mg, M6-TT + SAL-1mg, M6-TT + SAL-10mg, M6-TT + SAL-20mg, M6-TT + SAL-40mg, M3/6-TT + SAL-1mg, M3/6-TT + SAL-10mg, M3/6-TT + SAL-20mg, and M3/6-TT + SAL-40mg) received IP sterile saline solution (9% NaCl). In contrast, the animals of the morphine groups (TT + MOR-1mg, TT + MOR-10mg, TT + MOR-20mg, TT + MOR-40mg, M3-TT + MOR-1mg, M3-TT + MOR-10mg, M3-TT + MOR-20mg, M3-TT + MOR-40mg, M6-TT + MOR-1mg, M6-TT + MOR-10mg, M6-TT + MOR-20mg, M6-TT + MOR-40mg, M3/6-TT + MOR-1mg, M3/6-TT + MOR-10mg, M3/6-TT + MOR-20mg, and M3/6-TT + MOR-40mg) were administered with different doses of morphine (1, 10, 20, and 40 mg/kg, salt, respectively). And 30 minutes after administration of each treatment, tail-flick latency was recorded ()).
For the hot-plate test, the experiment consisted of one phase, the evaluation phase of the morphine-induced antinociceptive effects which included 1 assessment. After three days of habituation in the room where the hot-plate test would be performed, the animals received the treatments described for the tail-flick test. 30 minutes after administration of each treatment, licking latency was recorded.
Experimental design: Experiment 3
Experiment 3 sought to determine the efficacy of the antibodies generated by the M3-TT vaccine in preventing the antinociception induced by different doses of heroin. In this experiment, 512 female Balb-c mice were used, which were divided into two groups; one group of mice was used in the tail-flick studies (n = 256) and another group was used in the hot-plate test (n = 256). The 256 Balb-c mice used in the tail-flick test or the hot-plate test were randomly subdivided into 32 experimental groups (n = 8). Each group received a different pharmacological treatment.
The tail-flick and hot-plate experiment were carried out according to what was described in experiment 2.
Statistical analysis
Data were expressed as mean ± SEM. For Experiments 2 and 3, tail-flick and hot-plate latency were analyzed using two-way ANOVA, with treatments (TT and M-TT) and drug doses (1, 2, 5, 10, 20, and 40 mg/kg) as the between-subjects factor. Results of antibody titers were analyzed using two-way repeated-measures ANOVA, where vaccination number was the repeated measures, and treatments (TT or M-TT), as the between-subjects factors; followed by a Tukey test for post hoc comparisons. Correlations between antibody titers and tail-flick or hot-plate latency mean in the different experiments were analyzed by Pearson correlations. SPSS software was used to perform all statistical analyses. The level of statistical significance was set at p < .05.
Results
Experiment 1
As shown in ), the TT group immunized with the TT vaccine did not show an increase in anti-heroin antibody titers. In contrast, the animals immunized with the M3-TT, M6-TT, and M3/6-TT vaccine showed a progressive increase (F = (5,135) 9636.407, p < .001) in anti-heroin/morphine antibody titer measured by ELISA ()).
Tukey test found significant differences in the antibody titers shown by the M3-TT, M6-TT, and M3/6-TT groups compared to the TT (p < .001) group, from the second immunization. Additionally, the Tukey test found that the maximum anti-heroin antibody titer for the M3-TT, M6-TT, and M3/6-TT groups was reached after the fourth booster (p < .001).
Additionally, the post-hoc test found differences in the specific antibody titers shown by the M3-TT and M3/6-TT groups compared to those shown by the M6-TT group (p < .002) from the third immunization. Furthermore, from the fourth vaccination, the statistical test found differences in antibody titers between the M3-TT and M3/6-TT (p < .002) groups.
Experiment 2
To determine the ability of specific morphine antibodies, produced by the M3-TT, M6-TT, and M3/6-TT vaccines, to decrease the antinociception induced by different doses of morphine; we measured the antinociceptive responses induced by 1, 10, 20, and 40 mg/kg of morphine intraperitoneally with the tail-flick and hot-plate test ()). Statistical analysis found no difference in tail-flick and licking latency shown by all saline groups evaluated in this study.
Tail-flick
As shown in ) and , (morphine at 1, 10, 20, and 40 mg/kg significantly increased (F = (4, 160) 1612.734 p < .001) antinociception to thermal pain in the TT + MOR groups compared to the tail-flick latency in the TT + SAL, M3-TT + SAL, M6-TT + SAL, and M3/6-TT + SAL groups.
Table 1. Comparison between the different groups of animals immunized with TT, M3-TT, M6-TT, and M3/6-TT vaccines on antinociception (tail-flick latency) induced by different doses of morphine, using a Tukey test. Groups administered with A) 1 mg/Kg, B) 10 mg/Kg, C) 20 mg/Kg, and D) 40 mg/Kg of morphine are shown in the different panels. *indicates significant p-values (p < .05)
In contrast, the mice immunized with the M3-TT vaccine and treated with 1, 10, or 20 mg/kg of morphine showed a significant decrease in the morphine-induced antinociceptive effect compared to the TT + MOR groups (); ). As shown in , the post-hoc test found no significant differences in tail-flick latency in the M3-TT + MOR-40mg group compared to the TT + MOR-40mg group On the other hand, table A-B shows that the animals vaccinated with the M6-TT vaccine showed a decrease in tail-flick latency only when they received 1 or 10 mg/Kg of morphine; the post-hoc test found no differences in the antinociceptive effect between the TT + MOR20mg and TT + MOR40mg groups compared to the M6-TT + MOR20mg and M6-TT + MOR40mg groups, respectively. Regarding the M3/6-TT vaccine, the statistical analysis found significant differences in the tail-flick latency shown by the mice of the M3/6-TT + MOR groups treated with 1, 10, 20, and 40 mg/Kg morphine compared to the respective TT + MOR groups (); ).
Additionally, shows that the post-hoc analysis found differences in the morphine-induced tail-flick latency in the M3-TT + MOR, M6-TT + MOR, and M3/6-TT + MOR groups versus the TT + SAL groups.
Additionally, the post-hoc test found significant differences in the morphine tail-flick latency in the groups immunized with the TT and M-TT vaccine, treated with 1 mg/Kg of morphine compared to the groups treated with 10 (p < .001), 20 (p < .001) or 40 (p < .001) mg/Kg. In addition, the analysis found differences between the groups treated with 10 mg/Kg compared to the groups treated with 20 (p < .002) or 40 (p < .002) mg/Kg. But, the same analysis found no differences between the groups treated with 20 mg/Kg compared to the groups treated with 40 (p = .97) mg/Kg ()).
Hot-plate
Morphine in different doses significantly increased (F = (4, 160) 850.121 p < .001) antinociception in the TT + MOR compared to the licking latency in the TT + SAL, M3-TT + SAL, M6-TT + SAL, and M3/6-TT + SAL groups (); ).
Table 2. Comparison between the different groups of animals immunized with TT, M3-TT, M6-TT, and M3/6-TT vaccines on antinociception (hot-plate latency) induced by different doses of morphine, using a Tukey test. Groups administered with A) 1 mg/Kg, B) 10 mg/Kg, C) 20 mg/Kg, and D) 40 mg/Kg of morphine are shown in the different panels. *indicates significant p-values (p < .05)
As shown in , the Tukey test found differences in the hot-pain latency shown by the animals of the M3-TT group compared to the TT + MOR at 1, 10, and 20 mg/Kg of morphine. But, found no difference at 40 mg/Kg of morphine between the two groups () and ). Besides, shows that the post-hoc test found differences in hot-plate latency induced by 1, and 10 mg/Kg shown by the M6-TT + MOR groups versus that shown by the TT + MOR1mg and TT + MOR10mg groups. However, As shown in the statistical analysis did not find differences between the latency shown by the M6-TT group treated with 20 or 40 mg/Kg of morphine compared to that shown by the TT + MOR20mg and TT + MOR40mg groups () and ). In contrast, the morphine licking latency shown by the M3/6-TT + MOR groups treated with 1, 10, 20, and 40 mg/Kg of morphine was significantly different from that shown by the TT + MOR groups treated with the respective doses of morphine. As well as, found differences in the hot-plate latency shown by the groups vaccinated with the M3-TT, M6-TT, and M3/6-TT vaccines compared to the TT + SAL groups, in doses of 10, 20, and 40 mg/Kg of morphine () and ).
Finally, statistical analysis found differences in the licking latency shown by the groups treated with 1 mg/kg of morphine and the animals treated with 10 (p < .001), 20 (p < .003), and 40 (p < .002) mg/Kg of morphine. As well as found differences between the animals treated with 10 or 20 mg/Kg of morphine versus the animals treated with 40 (p < .002) mg/Kg of morphine ()).
As observed in Pearson correlation coefficient analysis indicates that in the groups immunized with the M3-TT, M6-TT, and M3/6-TT vaccine at the different doses of morphine there is a significant correlation between specific antibody titers and morphine-induced tail-flick () or hot-plate () latency. Pearson correlation coefficient suggests that approximately 90% of the animals of groups immunized with the M3-TT or M6-TT vaccine, the morphine-induced tail-flick or hot plate latency depends on specific antibody titers. In contrast, about 95% of the animals in the group immunized with the M3/6-TT vaccine showed a significant correlation between morphine-induced tail-flick or hot plate latency and specific antibody titers.
Table 3. Comparison between the different groups of animals immunized with TT, M3-TT, M6-TT, and M3/6-TT vaccines on antinociception (tail-flick latency) induced by different doses of morphine, using a Tukey test. Groups administered with A) 1 mg/Kg, B) 2 mg/Kg, C) 5 mg/Kg, and D) 10 mg/Kg of heroin are shown in the different panels. *indicates significant p-values (p < .05)
Table 4. Comparison between the different groups of animals immunized with TT, M3-TT, M6-TT, and M3/6-TT vaccines on antinociception (hot-plate latency) induced by different doses of morphine, using a Tukey test. Groups administered with A) 1 mg/Kg, B) 2 mg/Kg, C) 5 mg/Kg, and D) 10 mg/Kg of heroin are shown in the different panels. *indicates significant p-values (p < .05)
Table 5. Comparison between the different groups of animals immunized with TT, M3-TT, M6-TT, and M3/6-TT vaccines on antinociception (tail-flick or hot-plate latency) induced by different doses of morphine or heroin, using the Pearson correlation coefficient. Panel A shows the Pearson correlation coefficient between morphine-induced tail flick latency and antibody titers. In B) the correlation coefficient between morphine-induced hotplate latency and antibody titers is shown. C) shows the correlation coefficient between heroin-induced tail-flick latency and antibody titers and D) shows the correlation coefficient between heroin-induced hotplate latency and antibody titers. *indicates significant p-values (p < .05)
Experiment 3
To determine the ability of specific heroin antibodies, produced by the M3-TT, M6-TT, and M3/6-TT vaccines, to decrease the antinociception induced by different doses of heroin; we measured the antinociceptive responses induced by 1, 2, 5, and 10 mg/kg, heroin intraperitoneally with the tail-flick and hot-plate test ()). Statistical analysis found no difference in tail-flick and licking latency shown by all saline groups evaluated in this study.
Figure 3. The heroin-treated groups had a dose-dependent increase in tail-flick and hot-plate latency. (a) Experiment timeline. Vaccinated mice administered with 1, 2, 5, or 10 mg/kg heroin had an attenuation in heroin-induced tail-flick or hot-plate latency. Mean tail-flick (b) or hot-plate (c) latency (± S.E.M.). *P < .01 significant effects on tail-flick or hot-plate latency in the TT + HER, the M3-TT-HER, the M6-TT-HER, and M3/6-TT-HER groups compared to saline-treated groups. ** P < .01 Significant effects on tail-flick or hot-plate latency in the TT-HER groups dosed with 1, 2, 5, or 10 mg/kg heroin compared to the M3-TT-HER, the M6-TT-HER, and M3/6-TT-HER groups. & P < .01 Significant effects on tail-flick or hot-plate latency in the TT-HER-2mg M3-TT-HER−2mg, M6-TT-HER−2mg, and M3/6-TT-HER−2mg compared to the TT-HER-1mg, M3-TT-HER−1mg, M6-TT-HER−1mg, and M3/6-TT-HER−1mg groups. # P < .01 Significant effects on tail-flick or hot-plate latency TT-HER−5mg, M3-TT-HER−5mg, M6-TT-HER−5mg, and M3/6-TT-HER−5mg groups compared to the TT-HER-2mg, M3-TT-HER−2mg, M6-TT-HER−2mg and, M3/6-TT-HER−2mg groups. ¤ P < .01 Significant effects on tail-flick or hot-plate latency in the TT-HER−10mg, M3-TT-HER−10mg, M6-TT-HER−10mg, and M3/6-TT-HER−10mg groups compared to the TT-HER−5mg M3-TT-HER−5mg, M6-TT-HER−5mg, and, M3/6-TT-HER−5mg groups, as determined by two-way ANOVA followed by Tukey’s test
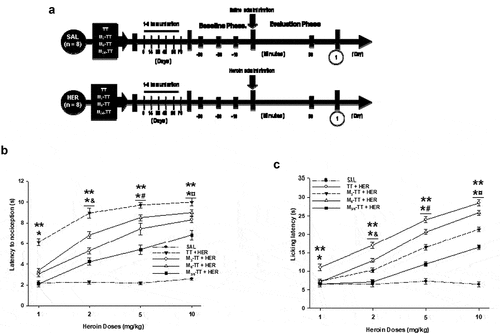
Tail-flick
As shown in ) and , heroin at 1, 2, 5, and 10 mg/kg significantly increased (F = (4, 160) 1446.649 p < .001) antinociception to thermal pain in the TT + HER compared to the tail-flick latency in the TT + SAL, M3-TT + SAL, M6-TT + SAL and M3/6-TT + SAL groups.
In contrast, as shown in , the mice immunized with the M3-TT, M6-TT, and M3/6-TT vaccines and treated with 1, 2, 5, or 10 mg/kg of heroin, showed a significant decrease in the heroin-induced antinociceptive effect compared to the respective TT + HER groups ()).
Additionally, as shown in , the post-hoc analysis found differences in the heroin-induced tail-flick latency in the M3-TT + HER, M6-TT + HER, and M3/6-TT + HER groups versus the TT + SAL, M3-TT + SAL, M6-TT + SAL, and M3/6-TT + SAL groups () and ). On the other hand, shows that the Tukey test found differences in the tail-flick latency shown by the M3-TT + HER and M6-TT + HER groups treated with 1 or 10 mg/Kg heroin compared to the M3/6-TT + HER group. However, the same table shows that the analysis did not find differences between the M3-TT + HER and M6-TT + HER groups. Furthermore, the post-hoc analysis shown in found a difference in tail-flick latency between the M3-TT + HER, M6-TT + HER, and M3/6-TT + HER groups when treated with 2 or 5 mg/Kg of heroin ()).
On the other hand, the post-hoc test found significant differences in the heroin tail-flick latency in the groups immunized with the TT and M-TT vaccine, treated with 1 mg/Kg of heroin compared to the groups treated with 2 (p < .001), 5 (p < .001) or 10 (p < .001) mg/Kg. In addition, the analysis found differences between the groups treated with 2 mg/Kg compared to the groups treated with 5 (p < .002) or 10 (p < .002) mg/Kg. Additionally, the statistical analysis found differences between the groups treated with 5 mg/Kg compared to the groups treated with 1 (p < .002) mg/Kg of heroin ()).
Hot-plate
As shown in ) and , heroin in different doses significantly increased (F = (4, 160) 950.294 p < .001) antinociception in the TT + HER compared to the licking latency in the TT + SAL, M3-TT + SAL, M6-TT + SAL and M3/6-TT + SAL groups.
Furthermore, shows that the Tukey test found differences in the latency shown by the animals of the M3-TT, M6TT, and M3/6-TT groups compared to the respective TT + HER groups. As well as found differences in the hot-plate latency shown by the groups vaccinated with M3-TT, M6TT, and M3/6-TT vaccines compared to the respective TT + SAL groups, in doses of 2, 5, or 10 mg/Kg of heroin () and ).
Finally, the statistical analysis found differences in the licking latency shown by the groups treated with 1 mg/kg of heroin and the animals treated with 2 (p < .003), 5 (p < .001), and 10 (p < .002) mg/Kg of heroin. As well as found differences between the animals treated with 2 or 5 mg/Kg of heroin versus the animals treated with 10 mg/Kg of heroin (p < .001).
shows the Pearson correlation coefficient analysis, which shows that in the groups immunized with the M3-TT, M6-TT, and M3/6-TT vaccine at the different doses of heroin there is a significant correlation between specific antibody titers and heroin-induced tail-flick () or hot-plate () latency. Pearson correlation coefficient suggests that the groups immunized with the M3-TT or M6-TT vaccine showed a similar correlation coefficient, approximately 93% of the animals, the heroin-induced tail-flick or hot plate latency depends on specific antibody titers. In contrast, about 97% of the animals in the group immunized with the M3/6-TT vaccine showed a significant correlation between heroin-induced tail-flick or hot plate latency and specific antibody titers.
Discussion
Heroin is an opioid drug with strong addictive properties.Citation19,Citation20 Clinical studies have mentioned that, for now, there is no effective therapy, capable of significantly reducing relapses.Citation1,Citation2 A therapy that recently has been generating good results, both preclinical and clinical level, in decrease drug relapses at different addictive drugs, is active vaccination.Citation7–12 For opioid drugs have been reported different vaccine formulations against the heroin- or morphine-induced reinforcing effects.Citation13,Citation14,Citation17–22 These studies, in general, have suggested that an opioid vaccine formulation is required that is capable of trigger a very strong immune response and that antibodies capable of recognizing with extraordinary specificity to heroin, and morphine.Citation13,Citation14,Citation17–22 One strategy that has been used is the co-administration of two or more vaccines with different but complementary properties, which are capable of generating an immune response with the ideal properties needed to prevent the drug-relapses.Citation27,Citation28
We recently reported the development of two vaccines. The M6-TT and the M3-TT, which was capable of triggering a robust immune response.Citation13,Citation14 In the case of the M6-TT vaccine, previous reports have described that Immunization with the M6-TT elicited antibody titers of 1: 250 000 to the fourth immunization. These reports mentioned that the antibodies generated by the M6-TT vaccine were able to recognize morphine and heroinCitation13,Citation14 and reduce the self-administration of heroin or morphine, as well as to attenuate the antinociceptive effect of morphine and heroin.Citation13,Citation14,Citation31
Other studies have shown that immunization with the M3-TT vaccine produced antibody titers of 1: 480 000 for the four immunization.Citation14 These studies indicated that the antibodies generated by the M3-TT vaccine were able to recognize with a specificity similar to morphine and heroin, which were able to decrease the self-administration of heroin or morphine and attenuate the antinociception induced by different doses of heroin.Citation14
These studies are in line with the results of this study where immunization with the M6-TT vaccine or with the M3-TT vaccine generated antibody titers of about 1: 400,000 or 1: 480,000, respectively. Additionally, we found that mice immunized with the M6-TT vaccine showed a decrease in antinociception induced by different doses of morphine and heroin, which is consistent with that previously described in other studies, where animals vaccinated with the M6-TT vaccine showed a decrease in the thermal effect induced by doses of up to 5 mg/Kg of morphine.Citation31 In contrast, another study showed that animals vaccinated with the M3-TT vaccine showed a decrease in antinociception induced by doses of up to 20 mg/Kg of morphine or 10 mg/Kg of heroin; which is in line with what was found in this study where animals immunized with the M3-TT vaccine showed a decrease in tail-flick and hot-plate latency induced by doses of 20 mg/Kg of morphine or 10 mg/kg. Kg of heroin,
It is recognized that simultaneous administration or combination of vaccines in animals or humans for different pathologies is an effective therapeutic strategy because it minimizes the impact of some diseases, vaccination coverage improvement, since it does not interfere with the development of the immune response to different antigens, does not significantly increase the reactogenicity and does not compromise the safety of vaccination. Additionally combined immunization schedule greatly reduces the rate of infection, morbidity, and mortality and especially minimizing socioeconomic disruptions associated with the disease.Citation39–41
However, to the current date, the effect of simultaneous administration on the immune response is still under debate.Citation42 Some studies have shown that the combination vaccine did not decrease the immunogenicity of individual vaccines, conversely, increase the immune response, as in the case of the administration of the mixture of the HibT vaccine with DtaP vaccine, in which is increased potency anti-tetanus to greater than the sum of the potencies of the individual components (Hibt and DTaP vaccines).Citation43 However, other studies have suggested that the application of a combined vaccination program could compromise the immune response, not produce an optimal response for each antigen. Furthermore, the immune response may be different for single and combined vaccines.Citation42,Citation44
We in this work reported that the combination of M6-TT and M3-TT vaccine enhance the immunogenic response of individual vaccines. Immunization with M3/6-TT produces an antibody titer of 1: 590 000 for the third immunization. This suggests that the combination of two opioid vaccine formulations enhanced the immune response, induced by the individual vaccines.
These results are in line with studies reported by PravetoniCitation27 where the co-administration of the M6M-KLH vaccine and the OXY-KLH vaccine increased the immunogenicity induced by individual vaccines.Citation27 Townsend et alCitation28 found similar results, where the co-administration of the heroin-CRM and fentanyl-CRM vaccines generated titers of antibodies higher than those found for the individual vaccines.Citation28 This suggests that the combination of two vaccines enhances the efficacy of the individual vaccines.
However, Stowe et al.,Citation20,Citation21 reported different results where the combination of two immunoconjugates, Heroin-KLH and Morphine-KLH, not improve antibody titers, 1: 39 000, or the ability of antibodies to recognize heroin or morphine to that exhibited by the individual vaccines.Citation20,Citation21
Concerning the ability of the antibodies to recognize and decrease the antinociception induced by heroin or morphine, the animals vaccinated with the M3/6-TT vaccine showed a significant improvement in the decrease induced by the individual vaccine in the thermal antinociceptive effects. Animals vaccinated with the M3/6-TT vaccine were able to reduce the thermal antinociception induced up to 40 mg/Kg of morphine or 10 mg/Kg of heroin, evaluated in tail-flick and hot-plate tests.
These results are consistent with previous reports, where the combination of the M6M-KLH vaccine and the OXY-KLH vaccines significantly increased morphine and oxycodone retention in serum and reduced the distribution of 6-MAM and oxycodone to the brain.Citation27 Besides, the combination of the heroin-CRM and fentanyl-CRM vaccines generated titers of antibodies capable of decreasing the antinociceptive potency of fentanyl, heroin, and fentanyl/heroin mixture,Citation28 which is in line with the results of this study.
Additionally, Pearson’s correlation coefficient analyzes support that the combination of M6-TT and M3-TT vaccine enhances the immunogenic and behavioral responses of individual vaccines. This analysis indicates that the M3/6-TT vaccine is superior to the individual vaccines, M3-TT and M6-TT, given that a higher percentage of animals, the tail-flick or hot-plate latency induced by morphine (95%) or heroin (97%) depends on the specific antibody titers.
Thus, our results suggest that the combination of M3-TT and M6-TT vaccines are capable of generating antibody titers higher than those generated by monovalent vaccines and these antibodies are capable of reducing the antinociceptive effects induced by doses of up to 40 mg/Kg. of morphine or 10 mg/kg of heroin.
However, this study has limitations, it is certainly demonstrated that the combination of M6-TT and M3-TT vaccine enhances the immunological and behavioral responses of individual vaccines, however, this study only evaluated one facet of the effects of an opioid drug such as heroin or morphine, antinociception, to our knowledge we need to evaluate the effect of the M3/6-TT vaccine on behavioral models such as self-administration, locomotor activity or place-preference since these pharmacological-behavioral paradigms are part of important processes (e.g. behavioral sensitization or compulsive seeking) of opioid use disorder in humans. On the other hand, another limitation consists in the form of immunization, a combination of two vaccines, it is necessary to improve the final formulation. For this, it is necessary to carry out studies in which an attempt is made to couple the two haptens (morphine-6-hemisuccinate and morphine-3-0-carboxymethyl) into the same carrier protein. This formulation could decrease the number of immunizations necessary to achieve maximum specific antibody titers.
In any case, these results support the strategy where the co-administration of two or more vaccines directed at one or multiple opioid targets could be useful to aid in the treatment of patients with opioid use disorder.
Authorship contributions
Participated in research design: Alberto Salazar-Juárez.
Conducted experiments: Alberto Salazar-Juárez, Maura Matus-Ortega, Ricardo Hernández Miramontes, and Susana Barbosa Méndez.
Performed data analysis: Alberto Salazar-Juárez and Susana Barbosa Méndez. Wrote or contributed to the writing of the manuscript: Alberto Salazar-Juárez and Susana Barbosa Méndez.
Disclosure of potential conflicts of interest
No potential conflicts of interest were disclosed.
Additional information
Funding
References
- Tetrault JM, Fiellin DA. Current and potential pharmacological treatment options for maintenance therapy in opioid-dependent individuals. Drugs. 2012;72(2):217–28. doi:10.2165/11597520-000000000-00000.
- Rahimi-Movaghar A, Amin-Esmaeili M, Hefazi M, Yousefi-Nooraie R. Pharmacological therapies for maintenance treatments of opium dependence. Cochrane Database Syst Rev. 2013. doi:10.1002/14651858.CD007775.
- Amato L, Minozzi S, Davoli M, Vecchi S, Ferri MM, Mayet S. Heroin anticraving medications: a systematic review. Am J Drug Alcohol Abuse. 2010;36:332–41. doi:10.3109/00952990.2010.505991.
- Kreek MJ, Borg L, Ducat E, Ray B. Pharmacotherapy in the treatment of addiction: methadone. J Addict Dis. 2010;29:200–16. doi:10.1080/10550881003684798.
- Fareed A, Vayalapalli S, Casarella J, Amar R, Drexler K. Psychosocial and pharmacological treatments versus pharmacological treatments for opioid detoxification. Cochrane Database Syst Rev. 2008;8:CD005031.
- Pasternak GW. Preclinical pharmacology and opioid combinations. Pain Med. 2012;13(Suppl 1):S4–11. doi:10.1111/j.1526-4637.2012.01335.x.
- Carrera MR, Ashley JA, Parsons LH, Wirsching P, Koob GF, Janda KD. Suppression of psychoactive effects of cocaine by active immunization. Nature. 1995;378(6558):727–30. doi:10.1038/378727a0.
- Cerny EH, Cerny T. Vaccines against nicotine. Hum Vaccine. 2009;5(4):200–05. doi:10.4161/hv.5.4.7310.
- Fahim RE, Kessler PD, Fuller SA, Kalnik MW. Nicotine vaccines. CNS Neurol Disord Drug Targets. 2011;10(8):905–15. doi:10.2174/187152711799219343.
- Fox BS, Kantak KM, Edwards MA, Black KM, Bollinger BK, Botka AJ, French TL, Thompson TL, Schad VC, Greenstein JL, et al. Efficacy of a therapeutic cocaine vaccine in rodent models. Nat Med. 1996;2(10):1129–32. doi:10.1038/nm1096-1129.
- Kosten TR, Rosen M, Bond J, Settles M, Roberts JS, Shields J, Jack L, Fox B. Human therapeutic cocaine vaccine: safety and immunogenicity. Vaccine. 2002;20:1196–204. doi:10.1016/S0264-410X(01)00425-X.
- Martell BA, Mitchell E, Poling J, Gonsai K, Kosten TR. Vaccine pharmacotherapy for the treatment of cocaine dependence. Biol Psychiatry. 2005;58:158–64. doi:10.1016/j.biopsych.2005.04.032.
- Anton B, Leff P. A novel bivalent morphine/heroin vaccine that prevents relapse to heroin addiction in rodents. Vaccine. 2006;24:3232–40. doi:10.1016/j.vaccine.2006.01.047.
- Anton B, Salazar A, Flores A, Matus M, Marin R, Hernandez JA, Leff P. Vaccines against morphine/heroin and its use as effective medication for preventing relapse to opiate addictive behaviors. Hum Vaccine. 2009;5:214–29. doi:10.4161/hv.5.4.7556.
- Schlosburg JE, Vendruscolo LF, Bremer PT, Lockner JW, Wade CL, Nunes AA, Stowe GN, Edwards S, Janda KD, Koob GF. Dynamic vaccine blocks relapse to compulsive intake of heroin. Proc Natl Acad Sci U S A. 2013;(22):9036–41. doi:10.1073/pnas.1219159110.
- Bonese KF, Wainer BH, Fitch FW, Rothberg RM, Schuster CR. Changes in heroin self-administration by a rhesus monkey after morphine immunization. Nature. 1974;252:708–10. doi:10.1038/252708a0.
- Bremer PT, Janda KD. Investigating the effects of a hydrolytically stable hapten and a Th1 adjuvant on heroin vaccine performance. J Med Chem. 2012;55:10776–80. doi:10.1021/jm301262z.
- Li QQ, Luo YX, Sun CY, Xue YX, Zhu WL, Shi HS, Zhai HF, Shi J, Lu L. A morphine/heroin vaccine with new hapten design attenuates behavioral effects in rats. J Neurochem. 2011;119:1271–81. doi:10.1111/j.1471-4159.2011.07502.x.
- Raleigh MD, Pravetoni M, Harris AC, Birnbaum AK, Pentel PR. Selective effects of a morphine conjugate vaccine on heroin and metabolite distribution and heroin-induced behaviors in rats. J Pharmacol Exp Ther. 2013;344:397–406. doi:10.1124/jpet.112.201194.
- Stowe GN, Schlosburg JE, Vendruscolo LF, Edwards S, Misra KK, Schulteis G, Zakhari JS, Koob GF, Janda KD. Developing a vaccine against multiple psychoactive targets: a case study of heroin. CNS Neurol Disord Drug Targets. 2011a;10:865–75. doi:10.2174/187152711799219316.
- Stowe GN, Vendruscolo LF, Edwards S, Schlosburg JE, Misra KK, Schulteis G, Mayorov AV, Zakhari JS, Koob GF, Janda KD. A vaccine strategy that induces protective immunity against heroin. J Med Chem. 2011b;54:5195–204. doi:10.1021/jm200461m.
- Kosten TA, Shen XY, O’Malley PW, Kinsey BM, Lykissa ED, Orson FM, Kosten TR. A morphine conjugate vaccine attenuates the behavioral effects of morphine in rats. Prog Neuropsychopharmacol Biol Psychiatry. 2013;45:223–29. doi:10.1016/j.pnpbp.2013.05.012.
- Torres OB, Matyas GR, Rao M, Peachman KK, Jalah R, Beck Z, Michael NL, Rice KC, Jacobson AE, Alving CR. Heroin-HIV-1 (H2) vaccine: induction of dual immunologic effects with a heroin hapten-conjugate and an HIV-1 envelope V2 peptide with liposomal lipid A as an adjuvant. NPJ Vaccines. 2017;2:13. doi:10.1038/s41541-017-0013-9.
- Sulima A, Jalah R, Antoline JFG, Torres OB, Imler GH, Deschamps JR, Beck Z, Alving CR, Jacobson AE, Rice KC, et al. Analogue that can serve as a vaccine hapten to induce antibodies that block the effects of heroin and its metabolites in rodents and that cross-react immunologically with related drugs of abuse. J Med Chem. 2018;61(1):329–43. doi:10.1021/acs.jmedchem.7b01427.
- Jalah R, Torres OB, Mayorov AV, Li F, Antoline JF, Jacobson AE, Rice KC, Deschamps JR, Beck Z, Alving CR, et al. Efficacy, but not antibody titer or affinity, of a heroin hapten conjugate vaccine correlates with increasing hapten densities on tetanus toxoid, but not on CRM197 carriers. Bioconjug Chem. 2015;26(6):1041–53. doi:10.1021/acs.bioconjchem.5b00085.
- Salazar-Juárez A, Barbosa-Méndez S, Feregrino-Alonso MI, Hernández-Miramontes R, Ramos-Ochoa E, Bonilla-Reyes E, Jacinto-Gutierrez S, Pentel P, Antón B. Nuevas Vacunas contra la morfina/heroína. Salud Mental. 2013;36:219–27. doi:10.17711/SM.0185-3325.2013.028.
- Pravetoni M, Raleigh MD, Le Naour M, Tucker AM, Harmon TM, Jones JM, Birnbaum AK, Portoghese PS, Pentel PR. Co-administration of morphine and oxycodone vaccines reduces the distribution of 6-monoacetylmorphine and oxycodone to brain in rats. Vaccine. 2012;30(31):4617–24. doi:10.1016/j.vaccine.2012.04.101.
- Townsend EA, Bremer PT, Faunce KE, Negus SS, Jaster AM, Robinson HL, Janda KD, Banks ML. Evaluation of a dual fentanyl/heroin vaccine on the antinociceptive and reinforcing effects of a fentanyl/heroin mixture in male and female rats. ACS Chem Neurosci. 2020;11(9):1300–10. doi:10.1021/acschemneuro.0c00064.
- Fink AL, Engle K, Ursin RL, Tang WY, Klein SL. Biological sex affects vaccine efficacy and protection against influenza in mice. Proc Natl Acad Sci U S A. 2018;115:12477‐12482. doi:10.1073/pnas.1805268115.
- Klein SL, Marriott I, Fish EN. Sex-based differences in immune function and responses to vaccination. Trans R Soc Trop Med Hyg. 2015;109:9‐15. doi:10.1093/trstmh/tru167.
- Méndez SB, Matus-Ortega M, Miramontes RH, Salazar-Juárez A. Effect of the morphine/heroin vaccine on opioid and non-opioid drug-induced antinociception in mice. Eur J Pharmacol. 2020. doi:10.1016/j.ejphar.2020.173718.
- Simon EJ, Dole WP, Hiller JM. Coupling of a new, active morphine derivative to sepharose for affinity chromatography. Proc Natl Acad Sci U S A. 1972;69:1835–37. doi:10.1073/pnas.69.7.1835.
- Wainer BH, Fitch FW, Fried J, Rothberg RM. A measurement of the specificities of antibodies to morphine-6-succinyl-BSA by competitive inhibition of 14 C-morphine binding. J Immunol. 1973a;110:667–73.
- Wainer BH, Fitch FW, Rothberg RM, Schuster CR. In vitro morphine antagonism by antibodies. Nature. 1973b;241:537–38. doi:10.1038/241537a0.
- Spector S, Berkowitz B, Flynn EJ, Peskar B. Antibodies to morphine,barbiturates, and serotonin. Pharmacol Rev. 1973;25:281–91.
- Spector S, Parker CW. Morphine: radioimmunoassay. Science. 1970;168:1347–48. doi:10.1126/science.168.3937.1347.
- D’Amour FE, Smith DL. A method for determining loss of pain sensation. J Pharmacol Exp Ther. 1941;72:74–78.
- Dewey WL, Harris LS, Howes JF, Nuite JA. The effect of various neurohumoral modulators on the activity of morphine and the narcotic antagonists in the tail-flick and phenylquinone tests. J Pharmacol Exp Ther. 1970;175:435–42.
- Tchilian EZ, Ronan EO, De Lara C, Lee LN, Franken KL, Vordermeier MH, Ottenhoff TH, Beverley PC. Simultaneous immunization against tuberculosis. PLoS One. 2011;6:e27477. doi:10.1371/journal.pone.0027477.
- Rieger MA, Hofmann F, Michaelis M. Simultaneous vaccination against hepatitis A and B: results of an open, randomized study from the occupational health point of view. Int J Occup Med Environ Health. 2004;17:379–91.
- Fukuda T, Iwaki M, Komiya T, Shibayama K, Takahashi M, Nakashima H. Effects of simultaneous immunization of Haemophilus influenzae type b conjugate vaccine and diphtheria-tetanus-acellular pertussis vaccine on anti-tetanus potencies in mice, guinea pigs, and rats. Jpn J Infect Dis. 2013;66:41–45. doi:10.7883/yoken.66.41.
- Boot HJ, Schipper CM. Simultaneous vaccination with Prevenar and multicomponent vaccines for children: interference or no interference? Hum Vaccine. 2009;5:15–17. doi:10.4161/hv.5.1.6520.
- Vesikari T, Forstén A, Desole MG, Ferrera G, Caubet M, Mesaros N, Boutriau D. A combined haemophilus influenzae Type b-Neisseria meningitidis Serogroup C-Tetanus toxoid conjugate vaccine (Hib-MenC-TT) is immunogenic and well-tolerated when co-administered with DTPa-HBV-IPV at 3, 5, and 11 months of age: results of an open, randomized, controlled study. Pediatr Infect Dis J. 2013;32:521–9. doi:10.1097/INF.0b013e31827e22e3.
- Madan RA, Heery CR, Gulley JL. Combination of vaccine and immune checkpoint inhibitor is safe with encouraging clinical activity. Oncoimmunology. 2012;1:1167–68. doi:10.4161/onci.20591.