ABSTRACT
Background
The highly pathogenic avian influenza H5N1 virus poses a serious threat to humans. Due to its antiviral activity, antibody-based therapy is one of the possible effective countermeasures. Here, a combination of intracellular and extracellular human antibodies was investigated and showed an improved protective effect.
Methods
The scFv4F5-based intracellular antibody vectors and IgG1 extracellular antibody were constructed and expressed, respectively, and the sensitivity, specificity, and affinity of these antibodies were determined in vitro. In vivo, the protective effect of IgG1 and the combination of antibodies were tested respectively. Furthermore, the dynamics of viral replication, the related cytokines and apoptosis-related proteins were detected.
Results
In vitro, the expressed intracellular antibody inhibited H5N1 virus propagation and the IgG1 exhibited high specificity, sensitivity, and affinity against the H5N1 virus. In vivo, the extracellular antibody could inhibit viral propagation in a dose-dependent manner. The protective effect of IgG1 was good in a mouse model, and the survival was 100% at a dose of 15 mg/kg under infection with 100 TCID50 virus. When the intracellular antibody was pre-transfected in combination with IgG1, it had a better protective effect. The survival was 16.67% under treatment with IgG1 alone and up to 83.33% under treatment with the combination of antibodies when challenge of 500 TCID 50 virus. Furthermore, the levels of cytokines IFN-γ, IL-6, IL-10 and some apoptosis-related proteins increased.
Conclusions
This antibody combination technique could be used as an appropriate and powerful alternative to antiviral therapy.
1. Introduction
The H5N1 virus has spread from wild birds to domestic poultry and can potentially infect humans with high mortality or morbidity.Citation1,Citation2 The last century witnessed four pandemics of human influenza worldwide, each of which differed from the others in etiology, epidemiology, and severity of disease.Citation3–6 It is essential to develop effective therapies for human populations at risk for H5N1 virus infections as well as those subsequently infection by human-to-human transmission.Citation7,Citation8 The viral surface glycoproteins HA and NA, which are highly susceptible to antigenic variations due to antigenic shift and drift, are the major targets of anti-influenza virus therapies.Citation9–11
In a previous study,Citation12 we obtained a broadly neutralizing single-chain variable fragment 4F5 (scFv4F5) antibody from a human vaccine-immunized phage-display library, and the antibody was shown to be effective against clade 2 and clade 9 of H5N1 viruses. The scFv4F5 antibody could bind to the HA protein and thus interfere with viral entry by steric hindrance. Furthermore, this antibody showed satisfactory protection against H5N1 influenza A virus in pre- and post-challenge studies in an embryonated chicken egg model. The antiviral activity scFv4F5 led to a 100% survival rate and an at least 62.5% survival rate against different clades of H5N1 viruses in pre- and post-treatment studies, respectively.
Intracellular immunizationCitation13 is a promising therapeutic technique that uses various forms of gene transfer to provide specific cellular resistance to viral infection. In addition to antisense oligonucleotides,Citation14 ribozymes,Citation15 and RNA interference,Citation16 another approach for the inhibition of viral protein functions is the use of intracellularly expressed antibodies, which is also known as intrabodies.Citation17 Intracellular antibodies are a new type of genetically engineered antibodies that are expressed in cells and specifically targeted to intracellular antigens to regulate or even block corresponding functions. The most common type of intracellular antibody is scFv antibodies, which can be localized in various organelles, such as the cytoplasm, nucleus, endoplasmic reticulum, mitochondria, cell membrane, and peroxisome, to realize the functional regulation of target antigens. The application of these antibodies is also influenced by early interference in viral replication and tumor growth. An scFv antibody consists of the VH and VL regions of the variable antigen-binding site of an immunoglobulin, connected with a short linker sequence. The scFv is the smallest functional unit of the parental antibody. Intrabodies have much higher specificity against target proteins than oligonucleotides, ribozymes, or RNA interference molecules.
The use of scFvs as intrabodies have several advantages over the use of immunoglobulins: a simple and compact structure, higher stability and solubility, and low immunogenicity. However, scFv antibodies often have some shortcomings, such as low affinity, a single function, poor stability, and rapid elimination in vivo, which restrict their wide application. Compared to scFv antibodies, whole-molecule IgG antibodies have antibody-dependent cellular cytotoxicity (ADCC) and complement-dependent cytotoxicity (CDC) functions due to their Fc fragment, which easily recognizes Fcγ receptors (FcγRs) on immune cells upon binding to tumor or viral antigens. However, whole-molecule IgG antibodies have yet to be introduced into cells.
Each approach has advantages for therapeutic application. In this study, the scFv4F5 gene was cloned into an eukaryotic expression vector and pre-transfected for expression as an intracellular antibody, which improved its antiviral effect in cells. Moreover, the scFv4F5 molecule was remodeled as full-length IgG1 antibody, which enhanced its ADCC and CDC effects on viral infection outside the cells. The combination antibody treatment had a better protective effect when used in challenge studies of mouse models.
2. Materials and methods
2.1. Virus, animals, chemical reagents, and antibodies
The virus A/Jiangsu/1/2007 (H5N1) (GenBank: EU434686.1) was isolated from a patient as described previouslyCitation7 and propagated in embryonated chicken eggs. The recombinant scFv4F5 plasmid containing the antibody VH and Vλ chain sequence against the H5N1 virus were screened from a phage display antibody library, and the scFv4F5 and rHA1 proteins were prepared as previously described.Citation12 Specific pathogen-free (SPF) normal male BALB/c mice weighed 20 ± 2 g and aged 6 weeks were purchased from the Experimental Animal Center of Nanjing Medical University. The plasmid pCAG-GFP was purchased from Addgene (plasmid #11150, Cambridge, MA, USA).Citation18 The pCI-neo mammalian expression vector was purchased from Promega Corporation (plasmid #E1841, Madison, USA, 2009).Citation19 pComb3X Lambda (PubMed GenBank AF268281) and pIgG were gifts from Carlos F Barbas.Citation20 Anti-mouse cleaved PARP (#94885) (1:1000), anti-mouse Bim (#2933), and anti-mouse ß-actin (#4970) (1:2000) were purchased from Cell Signaling Technology (Danvers, MA, USA). Mouse anti-influenza A virus nucleoprotein (NP) monoclonal antibody (#MAB3325) was purchased from Abnova Corporation (Taipei, Taiwan). R-PE-conjugated AffiniPureF(ab’)2 fragment goat anti-mouse IgG (115–116-071) (1:2000), R-PE-conjugated AffiniPure F(ab’)2 fragment goat anti-human IgG (109–116-170) (1:2000) and peroxidase AffiniPure goat anti-mouse IgG (H + L) (115–035-003) (1:5000) were purchased from Jackson Immuno Research Inc. (West Grove, PA, USA). HRP-conjugated anti-His (#A00612) (1:5000) was purchased from GenScript (Nanjing, CN). The HiTrap Protein G affinity column (#170404) and Ficoll-Paque (#171440) were purchased from GE Healthcare (Piscataway, NJ, USA). The restriction enzymes EcoRI (#1040) and SmaI (#1085) were purchased from Takara Bio Inc. (Otsu, Shiga, Japan). LipofectamineTM 2000 was purchased from Thermo Fisher Scientific (San Jose, CA, USA). ELISA kits for IFN-α (#42102), IFN-β (#42400), IFN-γ (#MIF00), IL-6 (#M6000B) and IL-10 (#M1000B) were purchased from R&D Systems Inc. (Minneapolis, MN, USA).
2.2. Cell culture
Human lung carcinoma A549 cells were obtained from ATCC (#CRM-CCL-185) (Manassas, VA, USA). Madin-Darby canine kidney (MDCK) cells and the Chinese hamster ovary (CHO) cell-line CHO-DG44 (DHFR-/-), which were originally acquired from ATCC, were kindly provided by Dr. Jiao, CDC Jiangsu, China. A549 and MDCK cells were cultured in Dulbecco’s modified Eagle’s medium (DMEM) supplemented with 10% fetal calf serum (FCS; Atlanta Biologicals, Flowery Branch, GA, USA). CHO-DG44 (DHFR-/-) cells were cultured in CHO DG44 cell medium (Thermo Fisher Scientific, USA). All the cell lines were grown at 37°C in a 5% CO2 atmosphere.
2.3. Construction and transfection of the pCAG-scFv4F5-GFP vector and confirmation of intracellular antibody expression
The phagemid vector pComb3XSS-scFv4F5 was isolated, and the scFv4F5 gene was amplified by PCR with the primers 5’- GGAATTCATGGAGCTGGTGCTGACCCAG-3’ and 5’- CCCCGGGAGCTTGCATAGTCGGGCACGT-3’. The sequence of the 6X His tag in pComb3XSS was also amplified. Moreover, the cleavage site sequences for the restriction enzymes EcoRI and SmaI were added to both sides of the fragment. After double-enzyme digestion, the scFv4F5 gene was inserted into the eukaryotic expression plasmid pCAG-GFP. The recombinant plasmid pCAG-scFv4F5-GFP was constructed and examined by PCR and sequence analysis. For intrabody expression, the recombinant plasmid pCAG-scFv4F5-GFP was transfected into human alveolar epithelial A549 cells mixed with liposomes by using LipofectamineTM 2000 at different ratios. Green fluorescent protein (GFP) was detected with fluorescence microscopy. Furthermore, the transfected A549 cells were collected, and the expression of the scFv4F5 antibody was detected by Western blot after cell lysis in RIPA buffer.
2.4. Measurement of the inhibition of H5N1 virus propagation by the intracellular antibody
pCAG-scFv4F5-GFP was transfected into A549 cells as described above. After 24 hours, the cells were infected with H5N1 virus at a MOI of 0.001 once. The treated cells were detected by immunofluorescence microscopy. The pCAG-GFP plasmid was used as a negative control. The supernatant of each group was collected, and the viral titers (TCID50/ml) were calculated by the Reed and Muench method.Citation21,Citation22
2.5. Remodeling of scFv4F5 as full-length IgG1 and expression and purification of the extracellular antibody
The variable regions Vλ and VH of scFv4F5 were amplified from pComb3XSS-scFv4F5, and the constant regions Cλ and CH1 were amplified from pComb3X Lambda. CH2-3 was amplified from the pIgG vector, and the primers used were as follows, with the cleavage sites for the restriction enzymes EcoRI and SmaI and signal peptides added. VH: 5’-GGAATTCATGTCTGCACTTCTGATCCTAGCTCTTGTTGGAGCTGCAGTTGCTA TGGAGCTGGTGCTGACCCAG-3’ and 5’-ACCAGGCAGCCCAGGGCCGCGCTG GACCTGCTGCTTCCCCC-3’; CH1: 5’-GGGGGAAGCAGCAGGTCCAGCGCGGC CCTGGGCTG CCTGGTC-3’ and 5’-TTTGTCACAAGATTTGGGCTCACAAGATT TGGGCTCTGCTTT-3’; CH2-3: 5’-AAAGCAGAGCCCAAATCTTGTGAGCCCAA ATCTTGTGACAAA-3’ and 5’-CCCCGGGTC AATGGTGGTGATGATGG TG-3’; Vλ: 5’-GGAATTCATGTCTGCACTTCTGATCCTAGCTCTTGTTGGAGCTGCAGT TGCTGAGGTGCAACTGGTGCAATCC-3’ and 5’-GTTACTCTGTTGCAGTAATA ACACGCTGGGGCCCT TAGTGCT-3’; Cλ: 5’-AGCACTAAGGGCCCCAGCGTG TTATTACTGCAACAGAGTAAC-3’ and 5’-CCCCGGGTTATGAACATTCTGTAG GGGC-3’.
The full-length whole H and λ chain genes were amplified by overlap PCR and inserted into the mammalian expression vector pCI-neo. The recombinant pCI- neo-λ and pCI-neo-H plasmids were co-transfected into CHO-DG44(DHFR-/-) cells with LipofectamineTM 2000. The positive cell line that could express IgG1 was screened with G418, and positive clones were subcloned 3 times to obtain the stable cell line expressing IgG1. The supernatant of the lysed cells in medium and lysis buffer was purified using a protein G affinity column and probed by SDS-PAGE. The sensitivity, specificity and affinity of the whole-molecular IgG1 antibody were determined by ELISA, flow cytometry and Biacore X100 SPR biosensor technology.
2.6. Microneutralization assay
Neutralizing antibody titers were determined by microneutralization assays performed on MDCK cells following a previously described procedure. The 50% tissue culture infective dose (TCID50) was calculated by the standard Reed and Muench method.Citation21,Citation22 In subsequent microneutralization assays, serially diluted scFv4F5 or IgG1 antibodies were incubated with 100 TCID50 of the virus before being added to MDCK cells. Plates were incubated for 40 hours before fixation of the cells with 4% paraformaldehyde. The presence of the virus was detected with a mouse anti-influenza A virus NP antibody and peroxidase AffiniPure goat anti-mouse IgG. The OD450 was evaluated, and the IC50 was calculated. PBS was used as negative control.
2.7. Therapeutic effect of the intracellular and extracellular antibody combination on mice infected with H5N1 virus
Groups of 6-week-old BALB/c mice were used in all experiments, and the study was approved by the ethics committee of Nanjing Medical University. The mice were randomly divided into five groups, each with six mice. First, 100 TCID50 H5N1 viral suspensions were inoculated by intranasal administration. One day later, the treated groups were administered different concentrations of IgG1 antibody (2 mg/kg, 5 mg/kg, 10 mg/kg, and 15 mg/kg) by intraperitoneal injection. The negative control group was administered only PBS at the same site. The survival of mice was observed and recorded every day for 14 days, and Kaplan–Meier survival curves were constructed. The survival differences among groups were determined using Prism 7. Meanwhile, the weight loss of the mice was also recorded.
Furthermore, different doses of the pCAG-scFv4F5-GFP plasmid (0 μg, 5 μg, 10 μg, 20 μg, and 30 μg) were delivered by multipoint subcutaneous injection in the backs of another 5 groups,Citation23 each with 6 mice. The control groups were administered only PBS, which was also delivered by subcutaneous injection. Two days later, 500 TCID50 H5N1 viral suspensions were inoculated by intranasal administration. The treated groups were administered 15 mg/kg IgG1 by intraperitoneal injection 12, 24 and 48 hours later. The procedures used in this experiment was illustrated in
Figure 1. The eukaryotic expression vector pCAG-scFv4F5-GFP was constructed and then transfected into A549 cells.
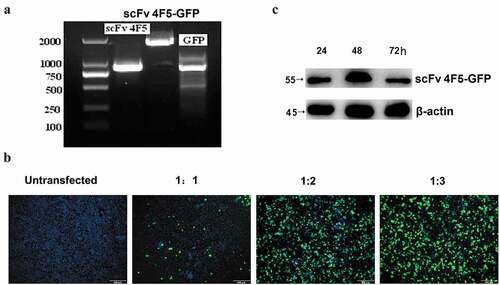
Figure 2. The expressed intracellular antibody can inhibit H5N1 virus propagation in A549 cells.
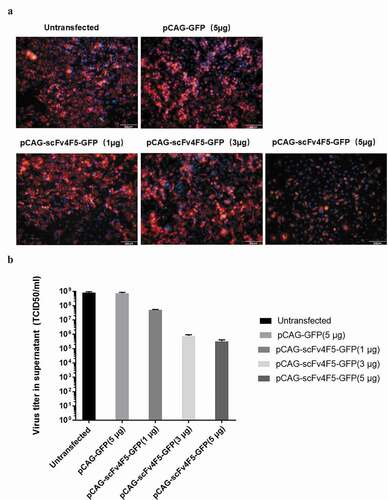
Figure 3. Full-length human anti-H5N1 virus IgG1 antibody-expressing vectors were constructed and then stably transfected into CHO-DG44 cells.

Figure 4. The purified full-length human IgG1 antibody has high specificity, sensitivity and affinity against H5N1 virus.
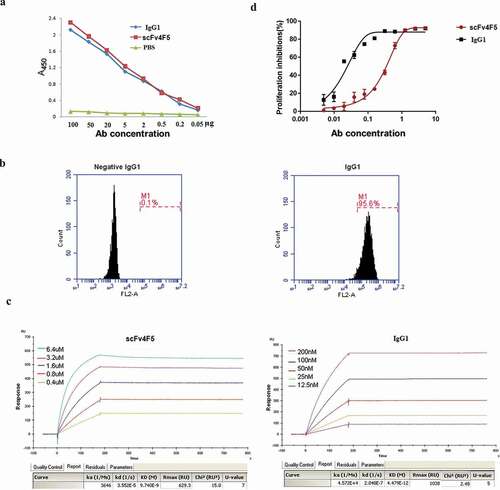
Figure 5. The combination of intracellular and extracellular antibodies has a better protective effect in a mouse model challenged with H5N1 virus.
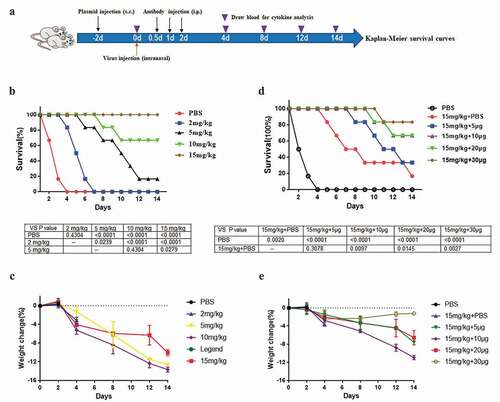
Coagulated blood and anticoagulated blood were collected at 0, 2, 4, 8, 12, and 14 days by tail snipping in another 15 mg/kg IgG1- and 30 μg pCAG-scFv4F5-GFP plasmid-treated groups. Serum was separated from coagulated blood for ELISA to detect cytokines, and uninfected mice were used as negative control. Peripheral blood mononuclear cells (PBMCs) were isolated from anticoagulated blood by Ficoll separation for real-time PCR. All the mice were sacrificed by euthanasia with CO2, and the lung tissues were collected and homogenized in sterile PBS using Potter–Elvehjem tissue grinders. The homogenate was centrifuged, and the viral titers in PBS were determined. The lung viral data were derived from different mice at multiple time points; however, the virus titers were detected simultaneously.
2.8. SDS–PAGE and western blot
Treated tissues were collected and lysed in RIPA lysis buffer containing a protease inhibitor cocktail at a 1/50 dilution (Calbiochem, San Diego, CA, USA). The supernatants of the lysed proteins were mixed with loading buffer and separated by SDS–PAGE. The proteins were transferred onto PVDF membranes and blocked for 1 hour at room temperature with 5% skim milk in PBS. To detect the expression of related proteins, specific diluted primary antibodies were added, and the membranes were incubated for 2 hours at room temperature. Following washed with PBST, an HRP-conjugated secondary antibody was added, and the membranes were incubated for 1 hour at room temperature. After washed with PBST, the membranes were probed with ECL detection solution (GE Healthcare).
2.9. ELISA
For binding activity analysis, H5N1 virus was coated onto ELISA plates. ScFv4F5 or IgG1 was then incubated in the coated wells for 2 hours at 37°C, and the wells were washed 3 times with PBST. Then, HRP-conjugated anti-His secondary antibody was added 1 hour later at 37°C, and the wells were washed 5 times with PBST. TMB solution was added and developed in the dark for 10 min. The enzymatic reaction was stopped by adding 2 M H2SO4, and the OD450 values of the reaction mixtures were determined using a microplate spectrophotometer.
2.10. Real-time PCR
Total RNA from all the PBMC samples was isolated using TRIzol, and 1 μg of total RNA was reversely transcribed using M-MLV reverse transcriptase purchased from Life Technologies (Carlsbad, CA, USA) according to the manufacturer’s instructions. All samples within each experiment were reversely transcribed simultaneously. qRT-PCR using cDNA as a template with SYBR Green for detection was performed using a LightCycler 96 system with FastStart SYBR Green Master Mix purchased from Roche. Appropriate no-RT and nontemplate controls were included in each 96-well PCR, and dissociation analysis was performed at the end of each run to confirm the specificity of the reaction. The expression level of each gene was normalized to the mRNA levels of the housekeeping gene GAPDH. Fold changes in the expression level of each gene were compared to that in the treated 0 day group using the 2−ΔΔCT method.
2.11. Data analysis
All data analyses were performed using Prism 7 (GraphPad, San Diego, CA, USA). The data are expressed as the means ± standard errors of the mean (SEMs). One-way ANOVA was used to determine statistically significant differences among groups followed by a t-test for comparisons.
3. Results
3.1. The eukaryotic expression vector pCAG-scFv4F5-GFP was constructed and then transfected into A549 cells, and the expressing intracellular antibody inhibited H5N1 virus propagation
The scFv4F5 antibody gene with a His-HA-tagg sequence of ~850 bp was amplified from the pComb3XSS-scFv4F5 plasmid. The PCR product was successfully inserted into the pCAG-GFP plasmid. As shown in , the pCAG-scFv4F5-GFP plasmid containing the scFv4F5 gene was approximately 1600 bp, while the blank plasmid pCAG-GFP containing GFP was ~750 bp (). This was further corroborated by sequence analysis. The plasmid pCAG-scFv4F5-GFP was transfected into A549 cells mixed with Lipofectamine 2000 at a ratio of 1:3, the number of GFP-positive cells was the most determined by fluorescence microscopy (). In addition, the expression of the intracellular antibody scFv4F5 was detected by Western blot at 24, 48 and 72 hours, as shown in , and the 55 kD fragment of the scFv4F5 and GFP fusion protein was detected in all the transfected cells, with the highest production observed at 48 hours after transfection. The expression of β-actin was detected, and there was no difference among groups. These results indicated that the antibody was expressed correctly with this eukaryotic expression system.
Furthermore, the relative H5N1 virus quantity in A549 cells, which were pre-transfected with the scFv4F5 vector and then infected with H5N1 virus, was determined. A decrease in the viral titer was observed with increasing transfection doses of pCAG-scFv4F5-GFP from 1 to 5 μg (). The viral titer of each supernatant is shown in , which shows the same trends as immunofluorescence assay. A decrease in the viral titer was observed with increasing doses of pCAG-scFv4F5-GFP. These results indicated that the intracellular antibody could inhibit the propagation of the H5N1 virus in a dose-dependent manner.
3.2. Full-length human anti-H5N1 virus IgG1 antibody-expressing vectors were constructed and then stably transfected into CHO-DG44 cells
The IgG1 genes VH, CH1, CH2-3, Vλ, and Cλ were amplified from different plasmids, and whole H and λ chain genes were amplified by overlap PCR and inserted into the mammalian expression vector pCI-neo. As shown in , Vλ, Cλ, VH, and CH1 were ~ 350 bp, the λ and CH2-3 gene fragments were ~ 750 bp, and the whole H gene was ~ 1500 bp. All the gene fragment sizes were as expected. The stable cell-line CHO-DG44, which can express the IgG1 antibody, was screened with 500 μg/ml G418, and a large number of cells that were not successfully transfected with the pCI-λ and pCI-H plasmids died 2 days later. Clone formation was visible by 5–10 days (). The expression of the whole-molecular IgG1 antibody protein was detected by Western blot. As shown in , a 25 kD λ chain and a 55 kD H chain were detected in both the supernatant and cell pellet. The collected supernatant was purified using a protein G affinity column and detected by SDS-PAGE. The 25 kD and 55 kD bands can be observed clearly in . The protein was obtained at high purity and high yield, with 5 mg of recombinant protein obtained from 100 ml of supernatant, which was sufficient for in vivo therapeutic assays.
3.3. The purified full-length human IgG1 antibody exhibited high specificity, sensitivity, and affinity against the H5N1 virus
The function of IgG1 was determined by ELISA, flow cytometry, the Biacore SPR biosensor technology, and a microneutralization assay, and the assays measured the following four parameters: i) the binding reactivity and sensitivity of whole-molecular IgG1 and the scFv4F5 protein were determined using H5N1 virus as the antigen. As shown in , the OD450 values of full-length IgG1 and scFv4F5 were almost the same at the same concentrations with no statistically significant differences, which illustrated that the binding reactivity of the IgG1 antibody was similar to that of scFv4F5. ii) The specificity of the IgG1 antibody was also determined by flow cytometry. IgG1 could bind up to 95% of virus-infected cells, compared to 0.1% for a commercial human IgG1 antibody (), which demonstrated that IgG1 had a specific binding reactivity against the H5N1 virus. iii) The affinities of IgG1 and scFv4F5 for the H5N1 virus glycoprotein rHA1 were measured and are expressed as the dissociation constant (Kd value). The Kd of IgG1 was 4.47 × 10−12, while that of scFv4F5 was 9.74 × 10−9 (), indicating that IgG1 had a better advantage against the virus than scFv4F5. iv) Finally, a microneutralization assay was used to determine the IC50 values of the antibodies. The TCID50 of the H5N1 virus was 10−5.615/0.1 mL when the virus was co-incubated at 100 TCID50 with the antibodies. The IC50 of IgG1 was 0.01521 μg/ml, which was lower than the value of 0.3451 μg/ml for scFv4F5. shows the inhibitory effect of the antibodies against H5N1 virus proliferation in MDCK cells. All these results indicated that the remodeled IgG1 antibody had high specificity, sensitivity, and affinity.
3.4. A combination of intracellular and extracellular antibodies has a better protective effect in a mouse model challenged with H5N1 virus
In vivo, mice pre-infected with 100 TCID50 H5N1 virus were treated with IgG1 at concentrations from 2 mg/kg to 15 mg/kg, the survival increased with increasing IgG1 concentration. Fourteen days later, the mice in the control group that were injected with PBS and those in the 2 mg/kg group all died. Mouse survival was 16.67% and 66.67% when the IgG1 concentration was 5 mg/kg and 10 mg/kg, respectively. It increased to 100% at a dose of 15 mg/kg (). The protective effect of IgG1 against the virus was dose-dependent. The weight loss of the mice was shown in , the mice received 15 mg/kg IgG1 showed the least weight loss at 14th day among the antibody treated groups.
In the subsequent combination therapeutic study, due to its good protective effect of IgG1, we chose the 500 TCID50 challenge, and the protective effect of the intrabody plasmid vector could be distinguished from that of IgG1. When the antibody combination was used, IgG1 was administered at a constant dose of 15 mg/kg, and the pCAG-scFv4F5-GFP vector was administered at 5 μg, 10 μg, 20 μg, or 30 μg. The protective effect against 500 TCID50 H5N1 virus increased with increasing pre-transfected scFv4F5 vector dose. The survival rates at vector doses of 5 μg, 10 μg, 20 μg, and 30 μg were 33.33%, 66.67%, 66.67%, and 83.33%, respectively. However, the survival rate was only 16.67% after treatment with IgG1 alone, and that after treatment with PBS alone was 0% (). The weight loss of the mice was shown in , the mice received 15 mg/kg IgG1 antibody with 30 µg plasmid group showed the least weight loss at 14th day among the combination antibody treated groups.
3.5. The secretion of IFN-γ and the related cytokines IL-6 and IL-10 increased after administration of the antibody combination
Serum and PBMCs were collected from the 15 mg/kg IgG1- and 30 μg pCAG-scFv4F5-GFP plasmid-treated groups, and 3 mice from each group were examined. First, the levels of cytokines, i.e., IFN-α, IFN-β, IFN-γ, IL-6, and IL-10, were determined by ELISA. shows that the IFN-α and IFN-β levels were not significantly different at any time point. The IFN-γ, IL-6 and IL-10 levels first increased on the 2nd day and then decreased after peaking on the 8th day. This indicated that the antibody combination could significantly affect the secretion of the cytokines IFN-γ, IL-6 and IL-10. Uninfected mice were used as negative control, and the levels of these cytokines is almost tending to zero in these mice. Real-time PCR, which was used to test the expression of the corresponding genes, corroborated this result. shows that the IFN-α and IFN-β expression levels were not significantly different at any time point. IFN-γ expression first increased on the 2nd day and then decreased after peaking on the 4th day. IL-6 and IL-10 expression first increased on the 4th day and then decreased after peaking on the 8th day.
Figure 6. The secretion of cytokines and the apoptosis-related proteins increased after administration of the antibody combination.
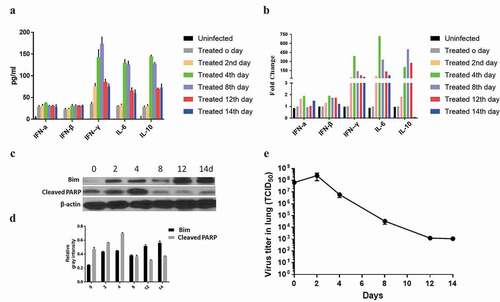
The apoptosis-related proteins Bim and cleaved PARP were detected by Western blot. As shown in and , the protein expression of Bim remained at a comparably high level on the 12th and 14th days after infection, while the expression of cleaved PARP peaked on the 4th day but showed no significant increase 8 days later. These results may indicate that the antibody combination could induce cell apoptosis.
The dynamics of viral replication were also determined. The viral titer (TCID50/lung) in each lung was calculated by the Reed and Muench method.Citation21,Citation22 As shown in , 24 hours after antibody injection, viral replication peaked at 2.5 × 108. Viral titers decreased after repeated administration, falling to 1.1 × 103 14 days later. All the results revealed that the antibody combination exerted a positive effect in the inhibition of viral replication.
4. Discussion
Antibody-based therapeutics have received significant interest in recent years. An increasing number of antibody drugs that target viruses or carcinoma cells have been successful in the treatment of diseases. Different antibody structures from various sources have been developed for anti-H5N1 virus therapies, with the viral surface HA and NA as the major targets.Citation24–27 In this paper, the protective effect of a combination of intracellular and extracellular antibodies against human influenza virus H5N1 was investigated. This is the first report of combination antibody-mediated inhibition of H5N1 virus propagation and secretion.
The cytoplasm is an important environment for viral replication and amplification. However, the use of most of the full-length IgG and some of the Fab or scFv antibodies have been limited because they cannot penetrate cells. These antibodies fail to enter cells and show inhibitory activities inside cells. Recently, some cell-penetrating antibodies that can be internalized into cells have been developed by conjugation with a peptide.Citation28 Compared to other intracellular therapies, i.e., antisense oligonucleotides, RNAi, shRNA, and siRNA, intracellular immunization with scFvs is worthy of investigation as they have several advantages, such as high specificity and long half-lives. Kaku et al. developed a scFv-based intrabody against RABV phosphoprotein (RABV-P), and when the scFv was transiently expressed in mouse neuronal cell lines before RABV infection, it severely inhibited the propagation and secretion of the virus and the spread of infection.Citation29 In addition, intrabody scFvs possess several advantages over full-length IgG, including a simple and compact structure, higher stability, and increasing solubility. Intrabodies based on scFvs have already been developed against human immunodeficiency virus-1,Citation30 hepatitis B virus,Citation31 hepatitis C virus,Citation32 rotavirus,Citation33 herpes virusCitation33,Citation34 and influenza virus.Citation35 The intrabody-based strategy can be applied to the development of therapeutic agents against viruses in the future because the cytoplasm is one of the most important environments for viral replication and amplification.
The antibody used in this study was scFv4F5, which was screened from vaccine-immunized human scFv libraries and could neutralize different clades of H5N1 viruses and bind to a conserved peptide in the hemagglutinin protein. The pre-expressed intrabody, which was pre-transfected on the eukaryotic expression vector, had a significant effect in eradicating H5N1 viral propagation in H5N1 virus-infected A549 cells. This indicates that scFv4F5 could be a prospective candidate intrabody; however, this inhibition might have been achieved partially due to an unexpectedly higher transfection efficiency compared to that of other expression systems. As such, the establishment of an appropriate delivery or application system is very important for further studies.
Furthermore, the Fc segment, which is the binding site on human IgG for FcγRI, FcγRII, RIII, C1q and FcRn, determines the effector function of the antibody, including the ADCC and CDC. To maintain all of these advantages, scFv4F5 was converted to a full-length IgG1 molecule for use as an extracellular antibody. Our results showed that the IgG1 molecule affinity and IC50 were obviously better than those of scFv4F5. This was corroborated by the fact that IgG1 could inhibit H5N1 virus propagation and reduce the replication of the virus in mouse challenge models.
In conclusion, we demonstrated that scFv4F5, when pre-expressed as an intrabody in A549 cells, substantially inhibited the propagation of the H5N1 virus. In addition, full-length human IgG1 was constructed expressed and used in combination with the intrabody in challenge studies in mouse models, which are among the best animal models for studying the effects of drugs on the H5N1 virus. In the in vivo experiment, mouse survival increased to 100% when IgG1 was used at 15 mg/kg. For the subsequent combination therapeutic study, due to its good protective effect of IgG1, we chose 500 TCID50 for challenge, and the protective effect of the intrabody plasmid vector could be distinguished from that of IgG1. The antibody combination obviously improved mouse survival. The survival rate was only 16.67% under treatment with IgG1 alone, and it reached 83.33% when antibodies were administered in combination. This scFv-based intracellular immunization could be a candidate therapeutic tool for use against H5N1 virus infection in the future if combined with an appropriate delivery and application system, such as a viral vector. Here, we describe a powerful tool for the establishment of novel therapeutic agents against H5N1 virus infection.
We also found that the secretion of cytokines, i.e., IFN-γ, IL-6, and IL-10, and the expression of apoptosis-related proteins, i.e., Bim and cleaved PARP, were increasing. All of these proteins could protect against viral infection within a certain range. As a double-edged sword, not all increased cytokine levels are beneficial; they could be indicators of severe infection rather than protection and may cause a lethal cytokine storm, leading to ARDS. The possible induction of IL-6 elevation in patients could lead to lung damage and cytokine storms. Furthermore, drug metabolism and pharmacokinetic parameters, i.e., the length of protection and antibody half-life, in the mouse model need to be investigated in preclinical research. For the reasons above, it may remain a major challenge to use this method in the clinic.
On the other hand, in intracellular and extracellular antibody combination therapy, 500 TCID50 is a relatively high dose that is not reflective of real-world infections. The dosage of scFv production and circulation after DNA vaccination is difficult to detect. Furthermore, with the given experiment results, it is difficult to tell whether the increased levels are a result of the virus challenge or ip-injected IgG1 or sc-injected scFv4F5 gene, or combinations of the above mentioned three. Thus, all of these points needed to be clearly shown in future studies.
Author Contributions
Conceptualization: X. Zhang; Methodology: X. Zhang and Q. Jin; Software: X. Zhang, and Z. Yao; Validation: X. Zhang, F. Liu, and J. Gao; Investigation: Q. Jin and Y. Di; Resources: X. Zhang; Data curation: X. Zhang and Z. Yao; Writing – Original Draft Preparation: Q. Jin and Z. Yao; Writing – Review & Editing: X. Zhang; Visualization: Q. Jin and J. Gao; Supervision: F. Liu and Y. Di; Project Administration: X. Zhang; Funding Acquisition: X. Zhang.
Animal rights statement
All institutional and national guidelines for the use of animals were followed.
Disclosure statement
The authors declare that they have no conflicts of interest.
Additional information
Funding
References
- Andrade CR, Ibiapina Cda C, Champs NS, Toledo Junior AC, Picinin IF. Avian influenza: the threat of the 21st century. J Bras Pneumol. 2009;35:5.
- Kilbourne ED. Influenza pandemics of the 20th century. Emerg Infect Dis. 2006;12(1):1. doi:10.3201/eid1201.051254.
- Uyeki TM. Human infection with highly pathogenic avian influenza A (H5N1) virus: review of clinical issues. Clin Infect Dis. 2009;49(2):2. doi:10.1086/600035.
- Nicholls H. Pandemic influenza: the inside story. PLoS Biol. 2006;4(2):2. doi:10.1371/journal.pbio.0040050.
- Gregg MB, Hinman AR, Craven RB. The Russian flu. Its history and implications for this year’s influenza season. JAMA. 1978;240:21. doi:10.1001/jama.1978.03290210042022.
- Gao R, Cao B, Hu Y, Feng Z, Wang D, Hu W, Chen J, Jie Z, Qiu H, Xu K, et al. Human infection with a novel avian-origin influenza A (H7N9) virus. N Engl J Med. 2013;368:20. doi:10.1056/NEJMoa1304459.
- Wang H, Feng Z, Shu Y, Yu H, Zhou L, Zu R, Huai Y, Dong J, Bao C, Wen L, et al. Probable limited person-to-person transmission of highly pathogenic avian influenza A (H5N1) virus in China. Lancet. 2008;371:9622. doi:10.1016/S0140-6736(08)60493-6.
- Doerr HW, Varwig D, Allwinn R, Cinatl J. Will the next human influenza pandemic be caused by the virus of the avian flu A/H5N1? Arguments pro and counter. Med Microbiol Immunol. 2006;195:2. doi:10.1007/s00430-005-0008-4.
- De Clercq E, Neyts J. Avian influenza A (H5N1) infection: targets and strategies for chemotherapeutic intervention. Trends Pharmacol Sci. 2007;28:6.
- Russell CJ, Webster RG. The genesis of a pandemic influenza virus. Cell. 2005;123:3. doi:10.1016/j.cell.2005.10.019.
- Subbarao K, Murphy BR, Fauci AS. Development of effective vaccines against pandemic influenza. Immunity. 2006;24(1):1. doi:10.1016/j.immuni.2005.12.005.
- Zhang X, Qi X, Zhang Q, Zeng X, Shi Z, Jin Q, Zhan F, Xu Y, Liu Z, Feng Z, et al. Human 4F5 single-chain Fv antibody recognizing a conserved HA1 epitope has broad neutralizing potency against H5N1 influenza A viruses of different clades. Antiviral Res. 2013;99:2.
- Baltimore D. Gene therapy. Intracellular immunization. Nature. 1988;335(6189):6189. doi:10.1038/335395a0.
- Zhang T, Wang TC, Zhao PS, Liang M, Gao YW, Yang ST, Qin C, Wang CY, Xia XZ. Antisense oligonucleotides targeting the RNA binding region of the NP gene inhibit replication of highly pathogenic avian influenza virus H5N1. Int Immunopharmacol. 2011;11:12. doi:10.1016/j.intimp.2011.08.019.
- Nawtaisong P, Keith J, Fraser T, Balaraman V, Kolokoltsov A, Davey RA, Higgs S, Mohammed A, Rongsriyam Y, Komalamisra N, et al. Effective suppression of Dengue fever virus in mosquito cell cultures using retroviral transduction of hammerhead ribozymes targeting the viral genome. Virol J. 2009;6(1). doi:10.1186/1743-422X-6-73.
- Zhou K, He H, Wu Y, Duan M. RNA interference of avian influenza virus H5N1 by inhibiting viral mRNA with siRNA expression plasmids. J Biotechnol. 2008;135(2):2. doi:10.1016/j.jbiotec.2008.03.007.
- Marasco WA. Intrabodies: turning the humoral immune system outside in for intracellular immunization. Gene Ther. 1997;4:1. doi:10.1038/sj.gt.3300346.
- Niwa H, Yamamura K, Miyazaki J. Efficient selection for high-expression transfectants with a novel eukaryotic vector. Gene. 1991;108:2.
- Brondyk B. pCI and pSI Mammalian expression vectors. Promega Note. 1994;49:7-11.
- Rader C, Popkov M, Neves JA, Barbas CF 3rd. Integrin alpha(v)beta3 targeted therapy for Kaposi’s sarcoma with an in vitro evolved antibody. FASEB J. 2002;16:14. doi:10.1096/fj.02-0281fje.
- Reed LJ, Muench HA. A simple method of estimating fifty percent endpoints. Am J Hyg. 1938;27:493–12.
- Krah DL. A simplified multiwell plate assay for the measurement of hepatitis A virus infectivity. Biologicals. 1991;19:3. doi:10.1016/1045-1056(91)90039-M.
- Prausnitz MR, Mikszta JA, Cormier M, and Andrianov AK. Microneedle-based vaccines. Curr Top Microbiol Immunol. 2009;333:369-93.
- Sui J, Hwang WC, Perez S, Wei G, Aird D, Chen LM, Santelli E, Stec B, Cadwell G, Ali M, et al. Structural and functional bases for broad-spectrum neutralization of avian and human influenza A viruses. Nat Struct Mol Biol. 2009;16:3. doi:10.1038/nsmb.1566.
- Oh HL, Akerstrom S, Shen S, Bereczky S, Karlberg H, Klingstrom J, Lal SK, Mirazimi A, Tan YJ. An antibody against a novel and conserved epitope in the hemagglutinin 1 subunit neutralizes numerous H5N1 influenza viruses. J Virol. 2010;84:16. doi:10.1128/JVI.02593-09.
- Ekiert DC, Bhabha G, Elsliger MA, Friesen RH, Jongeneelen M, Throsby M, Goudsmit J, Wilson IA. Antibody recognition of a highly conserved influenza virus epitope. Science. 2009;324:5924. doi:10.1126/science.1171491.
- Sakabe S, Iwatsuki-Horimoto K, Horimoto T, Nidom CA, Le M, Takano R, Kubota-Koketsu R, Okuno Y, Ozawa M, Kawaoka Y. A cross-reactive neutralizing monoclonal antibody protects mice from H5N1 and pandemic (H1N1) 2009 virus infection. Antiviral Res. 2010;88(3):3. doi:10.1016/j.antiviral.2010.09.007.
- Richard S, Agnes MH, And James PB. Cell penetrating antibodies for intracellular targets: expanding the antibody-based treatments for cancer. Proceedings of the 103rd Annual Meeting of the American Association for Cancer Research. Chicago, IL. Philadelphia (PA) 2012 Mar 31-Apr 4. p. 4637
- Kaku Y, Noguchi A, Hotta K, Yamada A, Inoue S. Inhibition of rabies virus propagation in mouse neuroblastoma cells by an intrabody against the viral phosphoprotein. Antiviral Res. 2011;91(1):1. doi:10.1016/j.antiviral.2011.04.016.
- Goncalves J, Silva F, Freitas-Vieira A, Santa-Marta M, Malho R, Yang X, Gabuzda D, Barbas C 3rd. Functional neutralization of HIV-1 Vif protein by intracellular immunization inhibits reverse transcription and viral replication. J Biol Chem. 2002;277(35):35. doi:10.1074/jbc.M201906200.
- Xun Y, Pan Q, Tang Z, Chen X, Yu Y, Xi M, Zang G. Intracellular-delivery of a single-chain antibody against hepatitis B core protein via cell-penetrating peptide inhibits hepatitis B virus replication in vitro. Int J Mol Med. 2013;31(2):2. doi:10.3892/ijmm.2012.1210.
- Karthe J, Tessmann K, Li J, Machida R, Daleman M, Haussinger D, Heintges T. Specific targeting of hepatitis C virus core protein by an intracellular single-chain antibody of human origin. Hepatology. 2008;48(3):3. doi:10.1002/hep.22366.
- Vascotto F, Campagna M, Visintin M, Cattaneo A, Burrone OR. Effects of intrabodies specific for rotavirus NSP5 during the virus replicative cycle. J Gen Virol. 2004;85(11):3285–90. Pt 11. doi:10.1099/vir.0.80075-0.
- Corte-Real S, Collins C, Aires da Silva F, Simas JP, Barbas CF 3rd, Chang Y, Moore P, Goncalves J. Intrabodies targeting the Kaposi sarcoma-associated herpesvirus latency antigen inhibit viral persistence in lymphoma cells. Blood. 2005;106:12. doi:10.1182/blood-2005-04-1627.
- Ashour J, Schmidt FI, Hanke L, Cragnolini J, Cavallari M, Altenburg A, Brewer R, Ingram J, Shoemaker C, Ploegh HL. Intracellular expression of camelid single-domain antibodies specific for influenza virus nucleoprotein uncovers distinct features of its nuclear localization. J Virol. 2015;89(5):5. doi:10.1128/JVI.02693-14.