ABSTRACT
Continued advances in microarray patch (MAP) technology are starting to make needle-free delivery of a broad range of vaccines an achievable goal. The drivers and potential benefits of a MAP platform for pandemic response and routine vaccination are clear and include dose-sparing, cold-chain elimination, increased safety, and potential self-administration. MAP technology is regarded as a priority innovation to overcome vaccination barriers, ensure equitable access, and improve the effectiveness of vaccines. Vaxxas, a global leader in this technology, has built a strong evidence-base for the commercial application of their high-density (HD) MAP platform, and is rapidly advancing scale-up of the manufacturing process for HD-MAPs. A greater awareness and understanding of the implications of the technology amongst supply-chain participants, regulatory authorities, and global healthcare organizations and foundations is needed to accelerate adoption and, particularly, to prepare for MAP use in pandemics. Key challenges remain in the commercialization of MAP technology and its adoption, including market acceptance, scale-up of production, regulatory approval, and the availability of capital to build advanced manufacturing infrastructure ahead of late-stage clinical trials.
Introduction
Vaxxas is in the process of commercializing its novel needle-free vaccination platform, the high-density microarray patch (HD-MAP), which aims to improve the efficacy, reach, safety, and economics of vaccination (see ).
Figure 1. Vaxxas’ HD-MAP, one of several vaccine MAPs under development worldwide. (a) the circular 1 cm2 MAP; (b) Close-up of the array of ~1,700 micro-projections; (c) scanning electron micrograph of vaccine-coated projections; (d) Vaxxas’ integrated HD-MAP and applicator;. The vaccine-coated HD-MAP is contained within the device, under the foil seal. the units nest together for convenient transport and storage, potentially outside the vaccine cold chain.
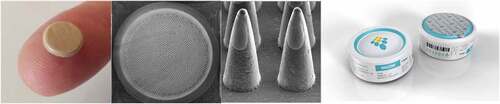
As a platform technology for vaccination aimed at control of endemic, epidemic, or pandemic diseases, MAPs are expected to transform vaccine delivery. They provide a valuable alternative for vaccines that are injected by needles and syringes (N&S), stored and transported in vials in the cold chain and delivered by highly trained healthcare workers. There are many companies and academic groups developing MAPs for vaccine delivery, therapeutic delivery of drugs and for cosmetic applications. MAPs, also termed microneedle patches, are diverse in their presentation and a detailed review of different MAP presentations and developers has been recently published.Citation1 Vaxxas’ HD-MAP comprises an array of thousands of micro-projections, each coated with vaccine and contained within a single-use disposable applicator. The administration process for the HD-MAP is simple: the vaccinator places the device on skin (most likely the upper arm in an adult) and triggers delivery of the vaccine by pressing down on the top of the device (see ).
Figure 2. Application of the HD-MAP, in this case to the forearm, which could be by self-administration.
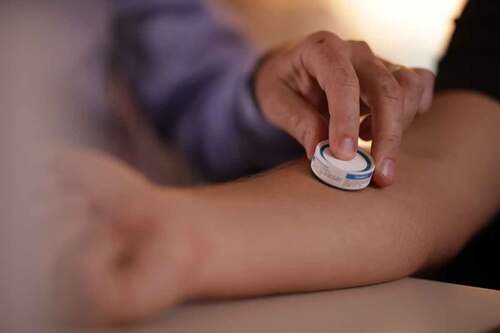
There are extraordinary opportunities for the widespread application of MAPs to significantly boost the capacity and ability of vaccination systems globally to respond effectively to infectious disease threats.
Drivers for a new vaccine-delivery paradigm
Well-established global systems exist for the administration of vaccines via N&S, which were first developed ~180 years ago.Citation2 They are a proven vaccine-delivery technology with widespread regulatory approval. There are also established and reliable supply chains and logistics, as well as extensive infrastructure and affirmed operating procedures for their use. Despite this, there are compelling drivers for the industry to invest in novel vaccine delivery platforms, such as the HD-MAP, that address the shortfalls of N&S for vaccination. For example, there are significant potential financial and economic benefits from shifting to more advanced delivery technology including dose sparing, increased reach, and accessibility of vaccines globally. There are costs associated with vaccination other than the price of the vaccine and delivery devices; in low- and middle-income countries (LMICs) the cost of the vaccine and vaccination supplies has been estimated to represent only 38% of the total cost of delivering vaccines,Citation3 and a similar proportion (18.5–33%) has been estimated for Australia.Citation4 With adaptation of existing supply chains to vaccine MAP use, over the longer term, there is the promise of significant potential cost and time savings in logistics and administration.Citation4–6
The speed of vaccine development and deployment in response to the COVID-19 outbreak has been remarkable. It has also laid bare many challenges in the delivery of vaccines to all the populations that need them. Mass vaccination of populations using N&S relies on trained health professionals, who are often in short supply, especially during pandemics. The need for multi-dose COVID vaccination regimens, including booster shots, and for billions of people around the world is predicted to exacerbate shortcomings in vaccination systems and extend to many vaccines beyond those to protect against SARS-CoV-2. The consequences of inadequate global vaccine coverage are already apparent for many diseases and progress in childhood vaccine coverage globally has already stalled, with an estimated 14.5 million ‘zero-dose’ children in 2019, i.e. children who did not receive a single dose of a diphtheria, tetanus, and pertussis-containing vaccine (used as a measure of children not reached by routine vaccination services).Citation7 The emergence of successive variants of concern (VoCs) initiating new waves of SARS-CoV-2 infection is prolonging this pandemic, delaying global post-pandemic recovery and will have a continuing impact on vaccination systems around the world.
HD-MAP technology targets delivery of a vaccine formulation direct to the abundant population of immune cells in the skin. It potentially eliminates the need for cold-chain storage and transport and could enable direct transport to point-of-care use. This could be to an individual at home or to a school setting. HD-MAPs vastly improve the simplicity of vaccine administration, creating an opportunity for self-administration or by a parent or guardian. The case for self-vaccination must balance risk and benefit and consider the safety of vaccination without oversight of a healthcare professional (although self-vaccination by individuals under supervision of a healthcare profession is also possible). Self-vaccination is probably most likely to be used for booster vaccinations where individuals have already received one or more doses of the vaccine in question. Vaccination with HD-MAPs does not require mixing or reconstitution. This removes the rare but significant safety risks associated with reconstitution. Reconstitution errors are believed to have contributed to vaccine hesitancy and the measles epidemic in Samoa.Citation8 HD-MAPs and MAPs in general have the potential to improve immunogenicity—perhaps initiating a faster or longer-lasting, or qualitatively different response.Citation9,Citation10 For some vaccines, an HD-MAP could reduce dosage requirements. MAPs are likely to be more acceptable than N&S and so lessen reasons for vaccine hesitancy.Citation11 Vaccines on MAPs could increase vaccine accessibility and equity, improve vaccination coverage rates, and the capacity of countries to rapidly respond to, prevent, or safeguard populations from both endemic diseases and disease outbreaks and pandemics.
Evidence supporting the shift to vaccine MAPs
There are many MAP technology developers and some have been developing non-vaccine products for over 20 years; however, no vaccine MAP products are currently marketed. The technology around MAPs has advanced significantly in very recent years, and the evidence and support for MAP vaccination systems is growing. As an example, in 2020, the Vaccine Innovation Prioritization Strategy (VIPS) by the VIPS Alliance [Bill and Melinda Gates Foundation (BMGF), Gavi, UNICEF, PATH, World Health Organization] recommended MAPs as one of three priority innovations to help overcome vaccination barriers, ensure equitable access, and improve the effectiveness of vaccines and to contribute to global health security.Citation12
As recognized by the VIPS Alliance, vaccine MAPs as a platform have broad applicability to different product types such as inactivated vaccines, purified/recombinant subunit antigens, live-attenuated vaccines, viral vectors and RNA-based vaccines. Vaxxas is developing MAPs with several of these platform technologies and to address challenges facing several different vaccines, with a number of partners including the BMGF, Merck and the Biomedical Advanced Research and Development Authority (BARDA).
For HD-MAPs, penetration of the high-density array of micro-projections through the stratum corneum of the skin is achieved via an integrated applicator. This has been designed to compete on cost and manufacturability with disposable prefilled syringes. The integrated applicator ensures a controlled, consistent skin penetration and delivery of the vaccine product with low reliance on technical expertise of the user. When the applicator is triggered by downward pressure, the HD-MAP accelerates to around 20 m/s before engaging the skin. There are tactile,/audible and visual indicators to confirm use. This dynamic application results in localized cell death at the application site,Citation13 generating ‘alarm signals’ (damage-associated molecular patterns) in combination with proximal delivery of antigen from the high-density array. Significant dose sparing has been observed in preclinical models,Citation13–15 and is most likely due to the co-localization of vaccine, cell death, and alarm signals. Vaxxas has demonstrated this benefit in a phase 1 clinical study comparing seasonal influenza vaccine doses delivered by HD-MAP to the standard dose used for intramuscular (IM) injection.Citation9 In this study, a 2.5 μg hemagglutinin (HA) protein dose (one-sixth of the standard IM dose) delivered using the HD-MAP, induced immune responses comparable with a full dose delivered by N&S (15 μg HA dose injected IM).
The Vaxxas HD-MAP also promises greater thermostability of vaccine products, with the potential to reduce or eliminate the need for cold-chain storage. Seasonal influenza antigens on the Vaxxas HD-MAP, and on other MAPs, have shown no significant decrease in potency over 12 months’ storage at up to 40°C.Citation9,Citation16 Improved thermostability has also been demonstrated with inactivated poliovirus vaccine (IPV) and measles rubella (MR) vaccine.Citation17,Citation18
Challenges in making the shift to vaccine MAPs a commercial reality
The promise of vaccine MAP technology, and its likely impact on global health, is compelling; however, the challenge is now to bridge the gap between early clinical development and commercial reality. There are challenges in several areas including:
To demonstrate breadth of applicability of MAPs to different vaccines in clinical trials. To date, only a limited number of clinical trials have been conducted with vaccine MAPs and these have largely focused on seasonal influenza vaccines,Citation9,Citation16,Citation19 although two clinical development programs with MR vaccine MAPs are ongoing. Demonstration of the safety, tolerability, and immunogenicity of MAPs (including HD-MAPs) with a wider range of vaccines and across a broader range of ages and skin types is needed. All MAP vaccine clinical studies to date have been performed in healthy young adults. The impact of different skin properties and also the properties of the tissues underlying the skin on vaccine delivery needs to be investigated. Additionally, the immunogenicity benefits of MAPs in humans have to date only be shown with protein-based vaccines, such as influenzaCitation9and an inactivated Japanese encephalitis virus vaccine.Citation20 In both of these cases, delivery of the vaccine by MAP resulted in enhanced immune responses compared with N&S injection. However, whether a similar effect will be seen with other vaccine types such as nucleic acids, live attenuated viruses or live virus vectors remains to be seen.
To demonstrate the ability to scale-up the manufacturing of vaccine MAPs to rapidly and reliably supply the quantities of quality-assured product needed in vaccination systems, whether for routine vaccination for endemic, epidemic, or pandemic infections. There are several hurdles to address in meeting this goal:
Scale-up of production
The success of MAP products will require automated manufacturing at scale using advanced microfabrication techniques, reliable dosing-control mechanisms and systems to assess and ensure quality.
Vaccine MAP developers are entering new territory with respect to manufacturing processes, capability, and infrastructure. Advanced pilot manufacturing runs will be required to demonstrate to, and assure, key players in the vaccine industry that a new vaccine MAP platform will be cost-effective to manufacture at scale compared with the existing well-established N&S.
One of the most challenging steps in the Vaxxas HD-MAP manufacturing process is the controlled deposition of the vaccine formulation onto the micro-projection array and associated quality control (QC). Liquid vaccine formulations are accurately and rapidly printed onto the thousands of micro-projections in the array using technology that deposits discrete picoliter-sized drops to the tips of all the 300-micron long projections in a single dispense. This matches the number and geometry of the HD-MAP. Vaxxas has built proof-of-concept machines to demonstrate innovative solutions to these challenges and developed in-house assay methods. The proof-of-concept line includes a rapid quality control (QC) feedback loop that adjusts the dispensing head to ensure continual alignment of the nozzle array to the exact positions of the micro-projections. For QC, to determine the loaded dose on a MAP following manufacture, Vaxxas develops elution methods that extract the dried vaccine formulation into a suitable buffer solution for subsequent analysis—an active potency measure is most often used in combination with verification of complete extraction via a simple protein content assay.
It is relatively simple to observe and confirm the dose volume filled in a vial or prefilled N&S, but there are technical challenges to achieve this with vaccine MAP platforms. For HD-MAPs, methods are needed to quantify the amount and potency of the vaccine dose coated on to the solid micro-projections. Vaxxas has developed and patented an HD-MAP coating system to ensure that the correct volume has been dispensed. It has further developed high-speed imaging and image software to confirm the targeting of the vaccine dispense and characterize the dose coated. This all takes place within the commercial coating time of 0.1 second per HD-MAP. Similar solutions will need to be found for other vaccine MAP platforms, for example dissolvable MAPS where the formulation is molded into the projection.
These new technologies, methods, mechanical solutions, and control systems must be developed and validated to assure regulators that quality can be consistently and reliably assessed and maintained at scale.
Regulatory requirements
All MAP vaccine clinical studies published to date have been performed using vaccines that are already marketed as an injectable product, and therefore these studies are enabled by existing vaccine product safety information, immunogenicity data, and quality of manufacture. Vaxxas’ first clinical studies were with a registered antigen from a seasonal influenza product. This enabled comparison of immunogenicity based on an established correlate for protection and provided an opportunity to prove the concept of enhanced immunogenicity with a small subject group size. 9 However, the business case for developing an existing vaccine with a novel delivery technology needs to be carefully assessed, given the need to repeat clinical studies; the scope, complexity, and cost of which depends on the vaccine and the expected benefit/label claims of MAP delivery. Developing a MAP delivering a vaccine that is not yet approved reduces or eliminates this repetition but comes with the risk that the vaccine’s efficacy has not yet been demonstrated. Manufacturing HD-MAP product for phase 1 clinical studies can be achieved relatively easily to the required compliance and quality standards. As an HD-MAP product moves into phase 2 and 3 trials, however, the regulatory compliance requirements increase substantially. The regulatory knowledge-base has been built around liquid vaccine filling for delivery by syringe. To qualify and manufacture a new technology, such as dry-coated MAPs, there are several aspects of manufacturing and quality standards that are likely to be unfamiliar to regulatory authorities and require new guidance. Regulators and MAP developers will need to agree acceptable QC methods, for example for the assessment of the deposition of liquid vaccines (as doses) on the micro-scale.
Preemptive investment in infrastructure
Once the proof-of-concept of a vaccine MAP has been demonstrated in phase 1/2 studies, funders and supply chain partners will (hopefully) be motivated to invest in, and accelerate, clinical development projects. The construction and commissioning of a pilot manufacturing facility and bespoke equipment can take 2–3 years. In a commercial environment where time-to-market can make or break a new product, either vaccine MAP developers and/or funding bodies will need to be proactive and prepared to invest ‘at-risk’ to establish pilot-scale facilities as early as possible. Well ahead of anticipated phase 2 studies, Vaxxas (with its partner Harro-Höfliger) is already designing a fully automated aseptic pilot-production line to compete with conventional prefilled syringe liquid-filling technology in terms of scale of output. Raising non-dilutive funding for commercialization and manufacturing infrastructure via government grant programs is an attractive proposition; however, the realities of program compliance and the long timelines to approval make this option restrictive and potentially unviable relative to the opportunity cost of delayed progress. Thus, MAP developers will typically require significant private capital to invest in facilities.
Market acceptance
Commercial applications of novel MAP technology to new and existing vaccines will require approval or support from reimbursement authorities and possibly from insurers. Assuming equivalency, such decisions are typically driven by cost (price of the vaccine dose) rather than vaccinee/guardian preference. There are other clinical challenges to user and industry acceptance of vaccine MAPs. For example, for vaccine MAPs, the anatomical site at which the vaccine stimulates the immune response, rather than being hidden in subcutaneous or muscle tissue, is superficial just under the skin. The inflammation that accompanies the initiation (or boosting) of immunity is expected to produce a visible, transient response on the skin. This could be viewed in some vaccination systems as a positive affirmation of vaccine delivery, similar to the purple ink on the finger of a polio-vaccinated child,Citation21 but some vaccine recipients (vaccine developers or regulatory authorities) may deem it unacceptable. Introduction of a new technology also requires a clear plan and data set to test usability/human factors in a real-world context. Several usability studies have been published indicating the general ease of use of Vaxxas MAP technology in both low/middle income and high-income countries, but both studies also highlighted the need for significant user education around technology introduction.Citation22,Citation23
Future opportunities for vaccine MAP technology
The pipeline of HD-MAP products in development at Vaxxas, and other MAP developers, has been growing in recent years. In 2021, there are new opportunities for the development of COVID-19 and mRNA vaccines on HD-MAPs, as two recent studies have indicated:
Preclinical studies with the SARS-CoV-2 HexaPro modified spike proteinCitation24 have shown that the protein can be formulated, dried, and stabilized on the HD-MAP.Citation21 In a mouse model, delivery of HexaPro on the HD-MAP was superior to intradermal (ID) delivery with N&S, inducing high titers of antibodies capable of neutralizing a range of SARS-CoV-2 VoCs, including Alpha and Beta.Citation25 In a transgenic mouse model, vaccination with a single dose of HexaPro plus the adjuvant QS21 on HD-MAPs elicited 100% protection from a lethal SARS-CoV-2 virus challenge. Without the adjuvant, two doses of HexaPro were sufficient for protection.Citation25
Since their use in the COVID-19 pandemic, there is growing global (including commercial) interest in use of RNA-based vaccines for other diseases including seasonal influenza.Citation26,Citation27 RNA-based vaccines have been shown to be suitable for rapid development and deployment in the early stages of a pandemic response. They can be used as booster doses and can be re-engineered with SARS-CoV-2 VoC sequences. Vaxxas, like other vaccine MAP developers, believes there are exciting opportunities to couple these RNA-based vaccines with MAP technologies—with the benefits of the RNA-based vaccines synergizing with the advantages of MAP delivery. This could be especially useful in pandemic preparedness, response, and recovery. For at least some of the RNA-based vaccine platforms, there remain challenges in vaccine formulation for use with MAPs, including if lipid nanoparticles are necessary for the formulation. Progress is, however, already being made by vaccine MAP developers in the dispensing and stabilization of RNA-based formulations.
Concluding statement
Demonstration of dose-sparing, and the safety and acceptability of HD-MAPs in human populations, combined with demonstration of the scalability of manufacturing and potentially lower cost of goods compared with prefilled N&S, should accelerate the adoption of HD-MAP technology by vaccine manufacturers and funding by global health partners.
In an environment where the threat presented by COVID-19 persists with the emergence of VoCs such as Omicron, in 2021, the Coalition for Epidemic Preparedness Innovations (CEPI) has announced an aspiration for the world to be able to respond to the next Disease X with a new vaccine in just 100 days.Citation28 While technical, production, and regulatory challenges remain, new technologies, such as the Vaxxas HD-MAP, will be critical in enabling vaccination systems to respond rapidly to deliver new vaccines and vaccine boosters, both for pandemics and routine vaccination. They could play an important part in protecting the most vulnerable populations across the world from devastating infections.
Acknowledgments
The authors would like to thank Prof Trent Munro, Prof Paul Young from The University of Queensland, Dr Patrick Silvey from VenturePro and Dr Julian Hickling and Rebecca Jones from Working in Tandem for their contributions to the manuscript.
Disclosure statement
No potential conflict of interest was reported by the author(s).
Additional information
Funding
References
- Prausnitz M. Engineering microneedle patches for vaccination and drug delivery to skin. Annu Rev Chem Biomol Eng. 2017;8:177–6. doi:10.1146/annurev-chembioeng-060816-101514.
- Lawrence G. The hypodermic syringe. Lancet. 2002;359:1074. doi:10.1016/S0140-6736(02)08101-1.
- Portnoy A, Ozawa S, Grewal S, Norman BA, Rajgopal J, Gorham KM, Haidari LA, Brown ST, Lee BY. Costs of vaccine programs across 94 low- and middle-income countries. Vaccine. 2015;33(Suppl 1):A99–108. doi:10.1016/j.vaccine.2014.12.037.
- Bozorgi A, Fahimnia B. Transforming the vaccine supply chain in Australia: opportunities and challenges. Vaccine. 2021;39:6157–65. doi:10.1016/j.vaccine.2021.08.033.
- Bozorgi A, Fahimnia B. Micro array patch (MAP) for the delivery of thermostable vaccines in Australia: a cost/benefit analysis. Vaccine. 2021;39:6166–73. doi:10.1016/j.vaccine.2021.08.016.
- Lee BY, Bartsch SM, Mvundura M, Jarrahian C, Zapf KM, Marinan K, Wateska AR, Snyder B, Swaminathan S, Jacoby E, et al. An economic model assessing the value of microneedle patch delivery of the seasonal influenza vaccine. Vaccine. 2015;33:4727–36. doi:10.1016/j.vaccine.2015.02.076.
- Galles NC, Liu PY, Updike RL, Fullman N, Nguyen J, Rolfe S, Sbarra AN, Schipp MF, Marks A, Abady GG . GBD 2020, release 1, vaccine coverage collaborators. measuring routine childhood vaccination coverage in 204 countries and territories, 1980-2019: a systematic analysis for the Global Burden of Disease Study 2020, release 1. Lancet. 2021;398:503–21. doi:10.1016/S0140-6736(21)00984-3.
- Craig AT, Heywood AE, Worth H. Measles epidemic in Samoa and other Pacific islands. Lancet Infect Dis. 2020;20:273–75. doi:10.1016/S1473-3099(20)30053-0.
- Forster AH, Witham K, Depelsenaire ACI, Veitch M, Wells JW, Wheatley A, Pryor M, Lickliter JD, Francis B, Rockman S, et al. Safety, tolerability, and immunogenicity of influenza vaccination with a high-density microarray patch: results from a randomized, controlled phase I clinical trial. PLoS Med. 2020;17:e1003024. doi:10.1371/journal.pmed.1003024.
- Rouphael NG, Lai L, Tandon S, McCullough MP, Kong Y, Kabbani S, Natrajan MS, Xu Y, Zhu Y, Wang D, et al. Immunologic mechanisms of seasonal influenza vaccination administered by microneedle patch from a randomized phase I trial. NPJ Vaccines. 2021;6:89. doi:10.1038/s41541-021-00353-0.
- Frew PM, Paine MB, Rouphael N, Schamel J, Chung Y, Mulligan MJ, Prausnitz MR. Acceptability of an inactivated influenza vaccine delivered by microneedle patch: results from a phase I clinical trial of safety, reactogenicity, and immunogenicity. Vaccine. 2020;38:7175–81. doi:10.1016/j.vaccine.2020.07.064.
- The Vaccine Innovation Prioritisation Strategy. [Internet]. [accessed 2021 Dec 14]. https://www.gavi.org/our-alliance/market-shaping/vaccine-innovation-prioritisation-strategy
- Depelsenaire ACI, Meliga SC, McNeilly CL, Pearson FE, Coffey JW, Haigh OL, Flaim CJ, Frazer IH, Kendall MAF. Colocalization of cell death with antigen deposition in skin enhances vaccine immunogenicity. J Invest Dermatol. 2014;134:2361–70. doi:10.1038/jid.2014.174.
- Muller DA, Fernando GJP, Owens NS, Agyei-Yeboah C, Wei JCJ, Depelsenaire ACI, Forster A, Fahey P, Weldon WC, Oberste MS, et al. High-Density microprojection array delivery to rat skin of low doses of trivalent inactivated poliovirus vaccine elicits potent neutralising antibody responses. Sci Rep. 2017;7:12644. doi:10.1038/s41598-017-13011-0.
- Fernando GJP, Chen X, Prow TW, Crichton ML, Fairmaid EJ, Roberts MS, Frazer IH, Brown LE, Kendall MAF. Potent immunity to low doses of influenza vaccine by probabilistic guided micro-targeted skin delivery in a mouse model. PLoS ONE. 2010;5:e10266. doi:10.1371/journal.pone.0010266.
- Rouphael NG, Paine M, Mosley R, Henry S, McAllister DV, Kalluri H, Pewin W, Frew PM, Yu T, Thornburg NJ, et al. The safety, immunogenicity, and acceptability of inactivated influenza vaccine delivered by microneedle patch (TIV-MNP 2015): a randomised, partly blinded, placebo-controlled, phase 1 trial. Lancet. 2017;390:649–58. doi:10.1016/S0140-6736(17)30575-5.
- Wan Y, Hickey JM, Bird C, Witham K, Fahey P, Forster A, Joshi SB, Volkin DB. Development of stabilizing formulations of a trivalent inactivated poliovirus vaccine in a dried state for delivery in the nanopatchTM microprojection array. J Pharm Sci. 2018;107:1540–51. doi:10.1016/j.xphs.2018.01.027.
- Wan Y, Gupta V, Bird C, Pullagurla SR, Fahey P, Forster A, Volkin DB, Joshi SB. Formulation development and improved stability of a combination measles and rubella live-viral vaccine dried for use in the NanopatchTM microneedle delivery system. Hum Vaccines Immunother. 2021;17:2501–16.
- Hirobe S, Azukizawa H, Hanafusa T, Matsuo K, Quan Y-S, Kamiyama F, Katayama I, Okada N, Nakagawa S. Clinical study and stability assessment of a novel transcutaneous influenza vaccination using a dissolving microneedle patch. Biomaterials. 2015;57:50–58. doi:10.1016/j.biomaterials.2015.04.007.
- Iwata H, Kakita K, Imakuku K, Takashima S, Haga N, Yamaguchi Y, Taguchi K, Oyamada T. Safety and dose-sparing effect of japanese encephalitis vaccine administered by microneedle patch in uninfected, healthy adults (MNA-J): a randomised, partly blinded, active-controlled, phase I trial. Lancet Microbe. 2021. online. doi:10.1016/S2666-5247(21)00269-X.
- GPEI-The final frontier in polio eradication. [Internet]. [accessed 2021 Dec 14]. https://polioeradication.org/news-post/the-final-frontier-in-polio-eradication/
- Guillermet E, Alfa DA, Mai LTP, Subedi M, Demolis R, Giersing B, Jaillard P. End-User acceptability study of the nanopatchTM; a microarray patch (MAP) for child immunization in low and middle-income countries. Vaccine. 2019;37:4435–43. doi:10.1016/j.vaccine.2019.02.079.
- Davies C, Taba M, Deng L, Karatas C, Bag S, Ross C, Forster A, Booy R, Skinner R. Usability, acceptability, and feasibility of a high-density microarray patch (HD-MAP) applicator as a delivery method for vaccination in clinical settings. Hum Vaccines Immunother. 2022; online. doi:10.1080/21645515.2021.2018863.
- Hsieh C-L, Goldsmith JA, Schaub JM, DiVenere AM, Kuo H-C, Javanmardi K, Le KC, Wrapp D, Lee AG, Liu Y, et al. Structure-Based design of prefusion-stabilized SARS-CoV-2 spikes. Science. 2020;369:1501–05. doi:10.1126/science.abd0826.
- McMillan CLD, Choo JJY, Idris A, Supramaniam A, Modhiran N, Amarilla AA, Isaacs A, Cheung STM, Liang B, Bielefeldt-Ohmann H, et al. Complete protection by a single-dose skin patch-delivered SARS-CoV-2 spike vaccine. Sci Adv. 2021;7:eabj8065. doi:10.1126/sciadv.abj8065.
- Dolgin E. mRNA flu shots move into trials. Nat Rev Drug Discov. 2021;20:801–03. doi:10.1038/d41573-021-00176-7.
- Maruggi G, Zhang C, Li J, Ulmer JB. Yu D. mRNA as a transformative technology for vaccine development to control infectious diseases. Mol Ther. 2019;27:757–72. doi:10.1016/j.ymthe.2019.01.020.
- Developing pandemic-busting vaccines in 100 days [Internet]. CEPI; 2021 [accessed 2021 Dec 14]. https://100days.cepi.net/100-days/