ABSTRACT
Severe Acute Respiratory Syndrome Coronavirus 2 (SARS-CoV-2) variants have been reported to be resistant to several neutralizing antibodies (NAbs) targeting Receptor Binding Domain (RBD) and N Terminal Domain (NTD) of spike (S) protein and thus inducing immune escape. However, fewer studies were carried out to investigate the neutralizing ability of S2-specific antibodies. In this research, 10 monoclonal antibodies (mAbs) targeting SARS-CoV-2 S2 subunit were generated from Coronavirus Disease 2019 (COVID-19) convalescent patients by phage display technology and molecular cloning technology. The binding activity of these S2-mAbs toward SARS-CoV-2 S, SARS-CoV-2 S2, SARS-CoV-2 RBD, SARS-CoV-2 NTD, severe acute respiratory syndrome coronavirus (SARS-CoV) S, SARS-CoV S2 and Middle East Respiratory Syndrome Coronavirus (MERS-CoV) S proteins were evaluated by enzyme-linked immunosorbent assay (ELISA). Their neutralizing potency toward SARS-CoV-2 wild-type (WT), B.1.1.7, B.1.351, P.1, B.1.617.2, B.1.1.1 and B.1.621 variants were determined by pseudo-virus-based neutralization assay. Results showed that S2E7-mAb had cross-activity to S or S2 proteins of SARS-CoV-2, SARS-CoV and MERS-CoV, while with limited neutralizing activity to pseudo-viruses of SARS-CoV-2 WT and variants. It is undeniable that the binding and neutralizing activities of the S2-targeting mAbs are significantly weaker than the previously reported antibodies targeting RBD and NTD, but our study may provide some evidences for understanding immune protection and identifying targets for vaccine design based on the conserved S2 subunit.
Introduction
Currently, under the progressive Coronavirus Disease 2019 (COVID-19) pandemic around the world, about 3.9 million mutant strains were found in 7.6 million SARS-CoV-2 sequences as of 1 February 2022,Citation1 SARS-CoV-2 variants have replaced the wild type and become the dominant strains globally. Many neutralizing antibodies (NAbs) elicited via natural infection and immunization of vaccines are gradually decreasing their protective efficacy against SARS-CoV-2 infections. To distinguish and emphasize these variants, World Health Organization (WHO) classified them into Variants of Concern (VOCs), Variants of Interest (VOIs) and Variants under Monitoring (VUM) based on the characteristics and transmission capacity, as well as the resistance to vaccines. Among them, five VOCs (B.1.1.7 (Alpha), B.1.351 (Beta), P.1 (Gamma), B.1.617.2 (Delta) and B.1.1.529 (Omicron)) and 2 VOIs (B.1.1.1 (Lambda) and B.1.621 (Mu)) were noted with increased transmissibility, enhanced infectivity, and elevated immune evasion.Citation2 Out of 1,051,598 genomes sequenced from the virus samples collected from 20 October to 19 December 2021, 1,009,253 (96%) belong to the Delta variant, 176 (<.1%) to Gamma, 53 (<.1%) to Alpha, 16,988 (1.6%) to Omicron, 16 (<.1%) to Beta, and 188 (<.1%) comprised other circulating variants (including VOIs Mu and Lambda).Citation3 However, in the recent 30-day period, among the 488,463 sequences collected from 26 January to 24 February 2022, 486,182 (99.5%) were Omicron, 1,601 (.3%) were Delta, and 6 (<.1%) were Alpha, no Beta, Gamma, Lambda, Mu or any other non-VOC/VOI sequences were reported, the current global epidemiology of SARS-CoV-2 is characterized by the global dominance of the Omicron variant.Citation4 With the continuous emergence of new mutants, developing novel therapeutic antibodies that can neutralize SARS-CoV-2 variants may be a focus for medical countermeasures against COVID-19.
SARS-CoV-2 contains four structural proteins, including spike protein (S), membrane protein (M), envelope protein (E) and nucleocapsid protein (N). In the process of infection, S protein was cleaved into S1 and S2 subunits for binding to human angiotensin-converting enzyme 2 (hACE2) receptors and membrane fusion, respectively.Citation5 Therefore, S protein was identified as the most important target to screen for neutralizing antibodies.Citation6,Citation7 A large number of Receptor Binding Domain (RBD)-targeting and N Terminal Domain (NTD)-specific NAbs with half-maximal inhibitory concentration (IC50) values at nM levels have been reported and characterized.Citation8–14 However, with the accumulation of mutations in variants, especially with D614 G, N501Y, E484K/Q/A, L452 R, T478K, Q493 R, Q498 R, P681 H or some other mutations located at RBD and NTD regions, previously reported neutralizing antibodies targeting RBD and NTD are gradually losing their effectiveness, leading to immune escape of SARS-CoV-2 variants.Citation15 In contrast, only a few mutations that have not been identified as possible neutralizing sites were found in SARS-CoV-2 S2 subunit, and S2 is determined to be markedly more conserved for functionally important sites than S1, such as the heptad repeat regions and fusion loop.Citation16,Citation17 Furthermore, a recent research has confirmed that populations with previous exposure to the common cold coronavirus could have cross-reactive neutralizing antibodies against SARS-CoV-2 S2 domain, suggesting the conservation of S2 across β-coronaviruses.Citation18 Another study also showed that 70% of patients infected with the common coronavirus can develop immune response to SARS-CoV-2 through the CD4 and CD8 pathway,Citation19 possibly activated by S2 epitopes.Citation20 Therefore, the conserved S2 subunit may be worthy of being used to search for neutralizing antibodies to prevent mutational escape.
In our previous work, we have already prepared several NAbs targeting RBD and NTD regions, which have great ability to neutralize authentic virus. However, like many other antibodies that target the neutralizing sites, our previous NAbs lost their neutralizing effect on the variants.Citation21–23 Based on the experience of a few S2-targeting antibodies reported by researchers around the world, considering the conserved S2 subunit may be more capable of recruiting preexisting memory B cells and inducing neutralizing antibodies against mutational escape. In this research, we aim to prepare S2-specific monoclonal antibodies (mAbs) and further evaluate their potential to prevent the immune escape of circulating SARS-CoV-2 variants, especially for these VOC and VOI variants defined by the WHO, finally provide ideas for the development of therapies and vaccines against COVID-19.
Materials and methods
Ethics statement
The informed consent for the use of blood and blood components was obtained from 5 donors in Jiangsu province on 14 February 2020, and then these bloods were taken as biological source materials for this study. The study was initiated after the approval from the Institutional Review Board of the Jiangsu Provincial Center of Disease Control and Prevention.
Cells
HEK-293F cells were cultured in FreeStyle™ 293 Expression Medium (Gibco, 12,338–018) containing 100 units/mL of Penicillin and .1 mg/mL of Streptomycin (Invitrogen) (Gibco, 15,140–122) at 37°C in an orbital incubator shaker with 8% CO2, which were used for the expression of S2 antibodies. 293T cells were maintained in OPM-293 CD03 Medium (OPM, 81,070–001) supplemented with 10% fetal bovine serum (FBS, Gibco, 12,657–029), 100 units/mL of Penicillin and .1 mg/mL of Streptomycin at 37°C in an orbital incubator shaker with 8% CO2, which were prepared for the SARS-CoV-2 pseudo-virus-based neutralization assay.
Pseudo-Viruses
SARS-CoV-2 pseudo-viruses were obtained from Vazyme Biotech Co., Ltd (China), including wild-type (WT) (Vazyme, DD1402–01), B.1.1.7 (Vazyme, DD1440–01), B.1.351 (Vazyme, DD1441–01), P.1 (Vazyme, DD1446–01), B.1.617.2 (Vazyme, DD1454–01), B.1.1.1 (Vazyme, DD1459–01) and B.1.621 (Vazyme, DD1461–01).
Panning the specific binding Fab pool
Peripheral blood samples (50 mL, 10 mL from each person) were collected from 5 COVID-19 convalescing patients admitted in Huai’an No 4 People’s Hospital of Jiangsu province, China. Peripheral blood mononuclear cells (PBMCs) of the 5 blood samples were collected by using Ficoll-Paque Plus (GE Healthcare) density gradient media according to the manufacturer’s protocol. In brief, blood samples were diluted with the same volume of phosphate buffered saline (PBS) (Gibco, 10,010–023) and then slowly transferred over Ficoll-Paque in SepMate-50 tube, after horizontal centrifugation at 800 g for 20 min at room temperature, PBMCs were collected and transferred into a new centrifuge tube for twice washes with PBS. Next, total RNAs of PBMCs were extracted by using RNeasy Mini Kit (Qiagen, CA) following the manufacturer’s instructions, and then the total RNAs were used as templates for cDNA synthesis by Transcriptor High Fidelity cDNA Synthesis kit (Roche, Germany) according to the manufacturer’s protocol. Full-length light-chain genes and Fd fragments (a variable region and a CH1 domain of a heavy chain) of heavy-chain genes were amplified from the mixed cDNA template by PCR with the primer pairs from 5VK, 7VL, and 8VH gene family.Citation24 Then light-chain genes were cloned into the phagemid vector pComb3 H by Xab I and Sac I and heavy-chain genes were sequentially cloned into the light-chain gene pool Xho I and Spe I following a standard protocol.Citation25 After two-step cloning, the prepared phage antibody library pool was frozen at −80°C for further use.
Recombinant SARS-CoV-2 S2 protein (Sino Biological, 40,590-V08B) was used for panning the phage antibody library pool following the procedure. In brief, SARS-CoV-2 S2 protein was coated in the immune tubes and incubated at 4°C overnight, then discarded the supernatants and washed the tubes with tris buffered saline with Tween® 20 (TBST) for five times. After that, the phage antibody library pool was added and incubated the tubes at 37°C for 2 h, then removed the supernatant and washed the tubes with TBST for five times. Finally, the enriched phage antibody library pool was eluted using .1 M Glycine-HCl (pH 2.2). Following the same steps, 1.0 mL of 50 μg/mL, 20 μg/mL and 10 μg/mL of SARS-CoV-2 S2 protein was used, respectively, for the first, second and third panning for screening specific binding antibodies from the prepared phage antibody library by phage display.Citation25,Citation26
Screening the S2-specific phage-single chain variable fragment (scFv) clones
Enzyme-linked immunosorbent assay (ELISA) was employed to screening for the specific binding phage-scFv clones. Briefly, 96-well ELISA plates (Corning, 42,592) were coated with SARS-CoV-2 S2 protein (Sino Biological, 40,590-V08B) and incubated at 4°C overnight, then plates were blocked with 1% bovine serum albumin (BSA) (Sangon, A600332–0100)/PBS (Gibco, 10,010–023) at 37°C for 2 hours (300 μL/well) and washed with PBST for five times. 50 μL supernatant from the single clone antibodies pool and 50 μL 1% BSA/PBS were added into each sample well and incubated at 37°C for 1 hour, blank control wells were supplemented with 1% BSA/PBS at the same time (100 μL/well). After five washes with PBST, a 1:3000 dilution of Horseradish peroxidase (HRP) conjugated anti-human Fab (Sigma, A0293) was added and incubated at 37°C for 1 hour (100 μL/well). Following five washes with PBST, 3,3',5,5'-Tetramethylbenzidine substrate (Thermo Scientific, 34,029) was added (100 μL/well) and incubated at room temperature for 10 min, finally 50 μL 2 M H2SO4 was added to each well to stop the reaction. Results were monitored at 450 nm, the cut-off value was set as the mean of the blank controls +2 standard deviation. The well with OD 450 value > cut off value was considered as positive well, thus phage-scFv against S2 was separated.
Sequencing and genetic analyses of antibodies
After ELISA, all the phage plasmid samples of single phage specific binding antibody against SARS-CoV-2 S2 subunit were separated for further sequencing the genes (Sangon Biotech, China). Sequences of these phage plasmid samples were blast and aligned with ImMunoGeneTics (IMGT) (www.imgt.org/) database. The full lengths of variable region amino acids were aligned by using the web servers of ESPrit 3.0 (http://espript.ibcp.fr/ESPript/ESPript/). Based on the diversity of variable genes and the OD values of positive wells in indirect ELISA, the specific candidate antibodies plasmids were optimized and constructed into PTT5 vector containing the constant regions of human IgG1 (General Biol, China).
Expression and purification of antibodies
Eukaryotic expression vectors (PTT5) of heavy and light chains were prepared, 500 μg of heavy-chain plasmids and 500 μg of light-chain plasmids were co-transfected with 3.0 mg polyetherimide (PEI, Poly-sciences, 23,966–1) into 1 L HEK-293F cells (1.5 × 106 cells/mL), and then keep shaking at 37°C with 8% CO2 for antibody expression. Seven days later, the cell media were centrifuged at 10,000 g for 10 min and the supernatant was filtered through .22 μm membrane. MabPurix™ Protein A column (Sepax Technologies, USA) was used for antibody purification by AKTA Explorer100 system (Cytiva, Sweden), concentrations were determined by Nanodrop One (Thermo, USA), the purified antibodies were frozen at −80°C for further use.
Enzyme-Linked immunosorbent assay (ELISA)
Binding abilities of 10 representative purified antibodies (S2B2, S2B5, S2B7, S2E6, S2E7, S2F6, S2G1, S2G9, S2H12 and S2110#) toward SARS-CoV-2 S, SARS-CoV-2 S2, SARS-CoV-2 RBD, SARS-CoV-2 NTD, SARS-CoV S, SARS-CoV S2 and MERS-CoV S were analyzed by ELISA. 96-well ELISA plates were coated with SARS-CoV-2 S (Sino biological, 40,589-V08B1), SARS-CoV-2 S2 (Sino biological, 40,590-V08B), SARS-CoV-2 RBD (Sino biological, 40,592-V08B), SARS-CoV-2 NTD (Sino biological, 40,591-V49 H), SARS-CoV S (Sino biological, 40,634-V08B), SARS-CoV S2 (Sino biological, 40,150-V08B3) and MERS-CoV S (Sino biological, 40,069-V088) and incubated at 4°C overnight (100 ng/well), then plates were blocked with 1% BSA/PBS at 37°C for 1 h (300 μL/well). Specific candidate antibodies were threefold serially diluted from 50 μg/mL to 68 ng/mL and incubated at 37°C for 30 min, blank control wells were supplemented with 1% BSA/PBS at the same time (100 μL/well). After washing with PBST for five times, HRP-anti-human IgG was added and incubated at 37°C for 30 min (100 μL/well). After five washes with PBST, 3,3',5,5'-Tetramethylbenzidine substrate was added and incubated at room temperature for 10 min (100 μL/well), then 50 μL 2 M H2SO4 solution was used to stop the reaction and read the absorbance at 450 nm and 630 nm wavelength. Results were evaluated by bringing the antibody concentration and the OD values into GraphPad software, the logarithm of the antibody concentration was taken as the X value, and the difference between OD450 nm and OD630 nm was taken as the Y value, then the half-maximal effective concentrations (EC50) values could be calculated by a four-parameter logistic non-linear regression analysis. Some high-titer antibodies were threefold serially diluted from 1 μg/mL to 1.37 ng/mL, others are consistent with the above method.
SARS-CoV-2 pseudo-virus neutralization assay
293T cells were cultured in OPM-293 CD03 medium (OPM, 81,070–001) containing 10% fetal bovine serum (FBS; Gibco Invitrogen) and incubated at 37°C with 8% CO2. Purified antibodies were diluted by 3‐fold serial dilutions from 200 μg/mL to 91 ng/mL and mixed with SARS‐CoV‐2 pseudo-virus solution containing 500 50% tissue culture infective dose (TCID50), then the mixture was incubated for 1 h at 37°C with 5% CO2. At the same time, blank control wells and virus control wells were added with 10% FBS/OPM and 500TCID50 pseudo-virus/OPM solution, respectively. After incubation, an equal volume of 293T cells (20,000 cells/well) were added to each well and incubated at 37°C with 5% CO2. At day 2 post-infection, the plates were added with Bio-Lite Reporter gene detection reagent (Vazyme, DD1201) and detect chemiluminescence values with microplate reader (Tecan, Switzerland). Bring the antibody concentration and the corresponding RLU into GraphPad software, take the logarithm of the antibody concentration and the RLU as the X value and Y value, respectively, then calculate the IC50 values.
Statistical analysis
The DNA sequences of the S2-mAbs were analyzed by the ImMunoGeneTics (IMGT) information tools, then VDJ sequences and CDR3 sequences were listed. In ELISA and neutralization assay, the half-maximal effective concentration (EC50) and half-maximal inhibitory concentration (IC50) values were determined by a four-parameter logistic non-linear regression analysis in GraphPad Prism 8.0 Software (GraphPad Software Inc.).
Results
A panel of mAbs targeting S2 domain of SARS-CoV-2 S protein were obtained from PBMCs of five COVID-19 convalescent patients by phage display, 10 antibodies with different VDJ sequences and CDR3 sequences in heavy- and light chains among them were selected for in vitro expression and for functional analysis (). Nine mAbs used HCV genes for SARS-CoV-2 mAbs (IGHV3–30 or IGHV3-30-3), while S2E7 mAb used HCV gene (IGHV1–46) (). The H-CDR3 lengths of these antibodies spanned from 12 to 14 amino acids (aa), similar to the lengths of 14 to 16 aa that previously published.Citation27 All of the 10 mAbs used the λ chain, seven of them used genes IGLV1–40, others were IGLV1–51, IGLV2–11, IGLV3–21, or IGLV6–57, which have not been reported in previous S2-mAbs (). The L-CDR3 lengths among these antibodies were ranging from 5 to 11 aa, corresponding to previous researches for SARS-CoV-2 mAbs.Citation27 As can be seen from the gene-type of SARS-CoV-2 S2-targeting antibodies (), the most representative IGHV gene and IGLV gene for mAbs were IGHV3–30 and IGKV3–20, which were slightly different from some gene-types of mAbs used in this manuscript. As public clonotypes specific to the S2 subunit have not been identified and the majority of previous mAbs cannot neutralize SARS-CoV-2, therefore, we prepared the 10 antibodies to explore their binding and neutralizing abilities.
Figure 1. Gene-Type of SARS-CoV-2 S2-targeting antibodies. the antibodies described in this manuscript and public antibodies are shown in orange and blue colors, respectively.
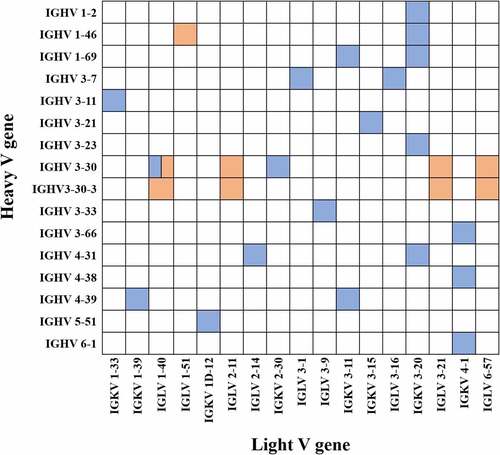
Table 1. Gene family usage of S2-targeting antibodies in this study.
As evaluated by ELISA, all of the 10 antibodies reacted with the SARS-CoV-2 S protein and SARS-CoV-2 S2 domain, and none of them cross binding to SARS-CoV-2 RBD or SARS-CoV-2 NTD subunit, verifying that they were S2-targeting antibodies (). All of them also reacted with the S and S2 subunit of SARS-CoV, but they were less reactive with the MERS-CoV S protein (). Among these antibodies, S2E7 mAb displayed highest reactivity with oversaturated OD values and was selected for ELISA testing in a lower range of concentration (), the results of which showed that S2E7 mAb exhibited EC50 of 54.87, 34.1, 69.24, and 49.13 ng/mL for SARS S, SARS S2, SARS-CoV-2 S and SARS-CoV-2 S2 proteins, respectively ().
Figure 2. Identification of SARS-CoV-2 S2-targeting antibodies by ELISA. (A) Identification of 10 individual mAbs to S protein of SARS-CoV-2 (Shown as mean ± S.D. of values derived from experiments conducted in triplicate). (B) Identification of 10 individual mAbs to S2 protein of SARS-CoV-2. (C) Identification of 10 individual mAbs to RBD protein of SARS-CoV-2. (D) Identification of 10 individual mAbs to NTD protein of SARS-CoV-2. (E) Identification of 10 individual mAbs to S protein of SARS-CoV. (F) Identification of 10 individual mAbs to S2 protein of SARS-CoV. (G) Identification of 10 individual mAbs to S protein of MERS-CoV. (H) Identification of S2E7 mAb to S, S2 and NTD (±) proteins of SARS-CoV-2 and SARS-CoV. “NTD (±)” means SARS-CoV-2 NTD and without SARS-CoV NTD.
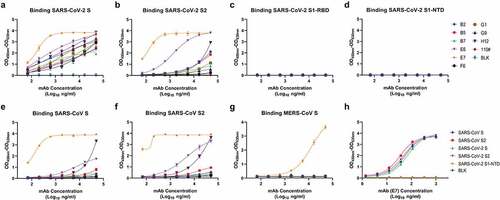
Table 2. EC50 and IC50 values of S2E7-mAb in ELISA and Pseudo-virus neutralization assay.
A pseudo-virus-based single-cycle infection assay was used to evaluate the neutralizing activity of the prepared antibodies on SARS-CoV-2 WT, B.1.1.7, B.1.351, P.1, B.1.617.2, B.1.1.1, and B.1.621 variants. In , when the antibody concentrations were low, the RLU kept plateaus, indicating that these antibodies failed to neutralize the pseudo-viruses. Subsequently, with the increase of antibody concentrations, RLU showed a downward trend. When the antibody concentrations increased to .2 mg/mL, almost all the antibodies were observed to neutralize both the SARS-CoV-2 WT and variants pseudo-viruses. However, the observed low neutralization plateaus and shallow neutralization curves indicated the incomplete neutralization, which were typically less potent than many neutralizing antibodies targeting either RBD or NTD. Among the 10 mAbs, S2E7 mAb exhibited higher activities than other mAbs against six of the seven pseudo-viruses, which could almost reach 100% neutralization at the highest concentration of .2 mg/mL. As for IC50 values, the S2E7-mAb showed limited neutralization potency with IC50 values over 10 μg/mL against the pseudo-viruses (). Considering the fact that these mAbs are too poor neutralizers to make a difference, we do not add an additional evaluation of our mAbs to the Omicron variant, which would be unlikely to contribute to the overall neutralization potency.
Figure 3. Identification of S2-targeting antibodies against SARS-CoV-2 pseudo-viruses by neutralization assay. (A) in vitro neutralization activities of 10 individual mAbs against SARS-CoV-2 WT in 293T-ACE2 cells. (Shown as mean ± S.D. of values derived from experiments conducted in triplicate). (B) in vitro neutralization activities of 10 individual mAbs against B.1.1.7 in 293T-ACE2 cells. (C) in vitro neutralization activities of 10 individual mAbs against B.1.351 in 293T-ACE2 cells. (D) in vitro neutralization activities of 10 individual mAbs against P.1 in 293T-ACE2 cells. (E) in vitro neutralization activities of 10 individual mAbs against B.1.617.2 in 293T-ACE2 cells. (F) in vitro neutralization activities of 10 individual mAbs against B.1.1.1 in 293T-ACE2 cells. (G) in vitro neutralization activities of 10 individual mAbs against B.1.621 in 293T-ACE2 cells.
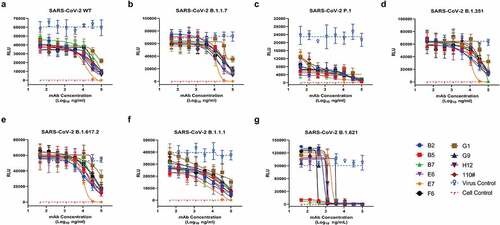
Discussion
In this study, we prepared 10 S2-specific mAbs and further evaluated their potential binding and neutralizing abilities, aimed to prevent the immune escape of circulating SARS-CoV-2 variants and finally provide ideas for the development of therapies and vaccines against COVID-19.
In ELISA, mAbs showed different binding abilities to S and S2 proteins of SARS-CoV-2, SARS-CoV and MERS-CoV, possibly be consistent with the published knowledge, the amino-acid identity between the SARS-CoV and SARS-CoV-2 is much higher in S2 domain (93%) than over the full-length S protein (78%), while the similarity drops sharply (<40%) when considering MERS-CoV.Citation28 Another interesting phenomenon was observed from the ELISA results, data demonstrated the slightly stronger ability of S2E7 in reacting with full-length S protein and S2 subunit of SARS-CoV than that of SARS-CoV-2, leading to the hypothesis that these antibodies may not only originate from the SARS-CoV-2 infection. This hypothesis was consistent with some results in previous reports, 86% of population without SARS-CoV-2 infection were observed with antibodies against SARS-CoV-2 S2 subunit, and most of the convalescent patients had higher levels of anti-S2 antibodies than anti-RBD antibodies, suggesting the provocation of S2 Memory B-cells and the produced IgG protective against infection originated from some old coronaviruses.Citation29 Apart from the preexisting B-cell memory response, the T-cell memory response may also play an important role in inducing strong and long-lasting immune response to antigens. Previous studies have verified that cytotoxic T-lymphocyte (CTL) epitopes at residues 884–891 and 1116–1123 were within the S2 subunit of SARS-CoV, therefore S2 subunit may also induce cell-mediated immunity.Citation30 A study of 5 different cohorts from Germany, Singapore, Netherlands, UK, and the USA confirmed this theory, results indicated that 20 to 50% of individuals who never infected with SARS-CoV-2 had reactive T-cells against SARS-CoV-2.Citation19,Citation31–34 In connection with the results of previous studies that the spike protein of SARS-CoV-2 has a 10 to 20 times greater affinity for ACE2 than that of SARS-CoV, it is speculated that SARS-CoV-2 S may not be recognized and bound by antibodies as easily as SARS-CoV.Citation35
In neutralization assay, different neutralizing capacity of the same mAb to multiple variants may be related to the mutation sites (). Antibodies were observed to have similar reactivity against WT, B.1.1.7, B.1.351, and B.1.617.2, suggesting that they may not recognize antigenically epitopes of A701 V, T716I, S982A, D1118 H and D950N, as these epitopes within S2 domain occurred in B.1.1.7, B.1.351, and B.1.617.2 pseudo-virus particles, respectively. In contrast, these antibodies showed another neutralization trend to B.1.1.1 variants, although they were tested under exactly the same experimental conditions, it is speculated that these differences may be related to the T859N in B.1.1.1. As for P.1 variants, with no mutation sites located in S2 region, the neutralization condition was significantly different from that of WT, may be due to the nature of the pseudo-viruses. Another thing worthy to be noted that these antibodies present different neutralizing abilities against B.1.621 from other variants, although the B.1.621 and B.1.617.2 shared the same mutation site of D950N located in the S2 subunit, verifying the diversity between pseudo-viruses even if they were only different in several amino acid sites. The effects of the mutation sites are only speculation after all, it is valuable to find potent neutralizing antibodies and select live viruses for functional analysis and structural characterization.
Table 3. Mutation sites of SARS-CoV-2 pseudo-virus particles.
In the real world, since November 2021, Omicron strains have been found in many countries. As of 24 February 2022, WHO determined that Omicron accounted for more than 99% of the strains sequenced in the past 30 days, so Omicron has become a major global epidemic strain.Citation4 At present, there are three hypotheses about the origins and evolution of Omicron, Omicron may circulate and evolve in a hidden population, in some immunosuppressed patients, or originate from adaptation in animal reservoirs and been transmitted back to human.Citation36 Compared with SARS-CoV-2 WT, Omicron has 53 more mutations, of which 34 mutations occurred in the S protein region, 13 mutations cluster within three functionally important regions of the S-gene seem cooperatively interact to mitigate their individual fitness costs and adaptively alter the function of SARS-CoV-2 S protein.Citation37 A recent study confirmed that Omicron S protein binds more strongly to hACE2 than other variants, and it mediates enhanced entry into cells expressing several different animal ACE2s, including various domestic avian species, horseshoe bats and mice, suggesting it has an increased propensity for reverse zoonosis and is more likely to establish an animal reservoir of SARS-CoV-2 than previous variants.Citation38 Another study also found that the N501Y and Q498 R in Omicron could enable the variant to bind rat ACE2 with increased affinity to almost 20-fold, which would be expected to drive increased human-to-human and cross-species transmission.Citation39 Numerous studies have shown that Omicron triggers immune escape more easily than other variants, neutralization titers of Omicron by many potent monoclonal antibodies and sera from COVID-19 vaccinees and SARS-CoV-2 convalescent subjects are substantially reduced, or these antibodies and sera failed to neutralize.Citation40–43 Therefore, finding potent neutralizing antibodies targeting conserved epitopes may inhibit the immune escape caused by these variants, such as the S2-specific NAbs.
Currently, there are fewer studies on S2-targeting antibodies (). Emanuele Andreano et al. isolated S2-specific mAb (L19) from COVID-19 convalescent patients, which showed neutralization potency with 19.8, 12.5 and 9.9 μg/mL for the authentic WT, the D614 G and the B.1.1.7 variant.Citation27 Another S2-binding mAb (CV3–25) reported by Madeleine F. Jennewein and colleagues was isolated from COVID-19+ subjects, which could neutralize both SARS-CoV-1 (IC50 2.1 μg/mL), Wuhan strain of SARS-CoV-2 (IC50.34 μg/mL) and the B.1.351 mutant strain, and can bind the S proteins of HKU1 and OC43 strongly, indicating that CV3–25 recognizes a conserved epitope among diverse coronaviruses.Citation44 Other two S2-mAbs (1.6C7 and 28D9) with remarkable cross-reactivity were isolated from immunized humanized mice by Chunyan Wang et al., the ELISA-based EC50 titers of mAbs 1.6C7 and 28D9 were 7 and .05 μg/mL, while no neutralization of them was seen against authentic SARS-CoV-2, only low levels of neutralizing activity by 28D9 were detected by VSV pseudoviral system (IC50 45.3 μg/mL).Citation45 Elaine C. Chen et al. identified four S2-specific antibodies (COV2–2002, COV2–2333, COV2–2164 and CnC2t1p1_B10) from convalescent survivors of SARS-CoV-2 and individuals vaccinated with mRNA vaccines, which bound to S2 with EC50 values of .67, 1.00, .61 and .63 μg/mL, respectively. However, the IC50 values from the real-time cell analysis (RTCA) assay were unavailable, suggesting the lack of neutralizing capacity for authentic SARS-CoV-2 and VSV-SARS-CoV-2.Citation46 Six S2-mAbs originated from COVID-19 patients showed moderately neutralizing ability to SARS-CoV-2 WT in the PRNT assay (EC50 36–133.33 nM) in Kuan-Ying A. Huang and colleagues’ study.Citation47 Some other S2-mAbs (46,472–1, 46,472–2, 46,472–3 and 46,472–4) prepared from recovered SARS-CoV donor sample had cross-reactivity with SARS-CoV-2 S but without neutralization in vitro were characterized by Andrea R. Shiakolas et al.Citation48 Fatima Amanat et al. also found that SARS-CoV-2 mRNA vaccination could induce functionally diverse antibodies to NTD, RBD, and S2, while all the S2-targeting mAbs did not have neutralizing activity.Citation49 In our study, the S2E7 mAb with inferior binding and neutralizing abilities than the RBD/NTD-NAbs, which may be classified as weak neutralizing antibody just like these S2-mAbs summarized in .
Table 4. Summary of S2-targeting antibodies in published researches and in this study.
Although the S2-mAbs in published researches and in this study did not have strong neutralizing abilities, the cross-reactivity of them between SARS-CoV-2 and other coronaviruses suggested the high sequence conservation of S2 subunit. These mAbs may not be affected by the mutations and has the potential to become a broad-spectrum therapeutic antibody. Rather than neutralizing antibodies with potent specificity for one antigen, we hope to screen neutralizing antibodies with cross-reactivity to multiple antigens to prevent immune escape caused by mutations in the real world. In addition, study on the S2 subunit may not only should be limited to the level of neutralizing antibodies it induces, but also in its role in distinguishing case types and preventing future infections. As asymptomatic infections usually elicited weaker antibody responses, the higher sensitivity of S2-mAbs (IgG) than RBD-mAbs (IgG) could supplement nucleic acid testing in identifying asymptomatic cases at early period.Citation50 Another study also proved that S2-peptides can be used as potential biomarkers for assessing the effectiveness of vaccination and differentiating COVID-19 patients from vaccinated individuals.Citation51 Furthermore, to understand the immune response to SARS-CoV-2, analyzing T-cell responses would be as important as B-cell responses, especially when T cell epitopes mainly present in S2 subunit.Citation52
There are several limitations in this study. First of all, limited by the expensive cost and experimental conditions of Cryo-Electron Microscopy (Cryo-EM), and for the reason that our screened antibodies did not have potent neutralizing abilities and had no further practical therapeutic value in the real world, so we did not use Cryo-EM to characterize the actual epitopes of mAbs binding to antigens. In addition, in the neutralization assay of pseudo-viruses, the performance of pseudo-viruses may be unstable during freeze-thaw and use, and fluorescence attenuation and quenching may occur during detection, which may affect the results.
In conclusion, ten antibodies targeting the SARS-CoV-2 S2 domain were prepared in this study. S2E7-mAb showed cross-reactivity to SARS-CoV-2 S, SARS-CoV S, MERS-CoV S, SARS-CoV-2 S2 and SARS-CoV S2 proteins, but with weak neutralizing abilities to SARS-CoV-2 WT and variants. It seems to indicate that S2-targeting neutralizing antibodies were not present in the 5 convalescent individuals included in our study. However, the cross- recognize activity is worthy of recognition, the verified conserved S2 domain may have important implications for recruiting preexisting memory B cells and T cells responses for diverse coronaviruses. Perhaps at some point in the future, S2-specific antibodies that are equivalent to neutralizing antibodies targeting RBD or NTD can be screened out, then vaccine design based on S2 neutralizing epitopes will effectively solve the current problem of mutational escape in multiple SARS-CoV-2 variants all over the world.
Acknowledgements
We thank Jinpeng Bi, Yidan Zhang, Minya Zhou and Zhiying Sun for their technical assistance. We thank the Funding of Jiangsu Provincial Key Research and Development Program BE2021738.
Disclosure statement
No potential conflict of interest was reported by the author(s).
Additional information
Funding
References
- National Genomics Data Center, China National Center for Bioinformation / Beijing Institute of Genomics, Chinese Academy of Sciences. Released Genome Sequences. 2021.
- WHO. Tracking SARS-CoV-2 variants. WHO. 2021. https://wwwwhoint/en/activities/tracking-SARS-CoV-2-variants/ .
- WHO. Weekly epidemiological update on COVID-19 - 21 December 2021. WHO. 2021. https://wwwwhoint/publications/m/item/weekly-epidemiological-update-on-covid-19—21-december-2021 .
- WHO. Weekly epidemiological update on COVID-19 - 1 March 2022. 2022. WHO. https://wwwwhoint/publications/m/item/weekly-epidemiological-update-on-covid-19—1-march-2022 .
- Walls AC, Park YJ, Tortorici MA, Wall A, McGuire AT, Veesler D. Structure, function, and antigenicity of the SARS-CoV-2 Spike Glycoprotein. Cell. 2020;181(2):281–92.e286. doi:10.1016/j.cell.2020.02.058.
- Hoffmann M, Kleine-Weber H, Schroeder S, Krüger N, Herrler T, Erichsen S, Schiergens TS, Herrler G, Wu NH, Nitsche A, et al. SARS-CoV-2 cell entry depends on ACE2 and TMPRSS2 and is blocked by a clinically proven protease inhibitor. Cell. 2020;181(2):271–80.e278. doi:10.1016/j.cell.2020.02.052.
- Papageorgiou AC, Mohsin I. The SARS-CoV-2 Spike Glycoprotein as a drug and vaccine target: structural insights into its complexes with ACE2 and antibodies. Cells. 2020;9(11). doi:10.3390/cells9112343.
- Barnes CO, West AP Jr., Huey-Tubman KE, Hoffmann MAG, Sharaf NG, Hoffman PR, Koranda N, Gristick HB, Gaebler C, Muecksch F, et al. Structures of human antibodies bound to SARS-CoV-2 Spike reveal common epitopes and recurrent features of antibodies. Cell. 2020;182(4):828–42.e816. doi:10.1016/j.cell.2020.06.025.
- Cao Y, Su B, Guo X, Sun W, Deng Y, Bao L, Zhu Q, Zhang X, Zheng Y, Geng C, et al. Potent neutralizing antibodies against SARS-CoV-2 identified by high-throughput single-cell sequencing of convalescent patients’ B cells. Cell. 2020;182(1):73–84.e16. doi:10.1016/j.cell.2020.05.025.
- Du S, Cao Y, Zhu Q, Yu P, Qi F, Wang G, Du X, Bao L, Deng W, Zhu H, et al. Structurally resolved SARS-CoV-2 antibody shows high efficacy in severely infected hamsters and provides a potent cocktail pairing strategy. Cell. 2020;183(4):1013–23.e13. doi:10.1016/j.cell.2020.09.035.
- Piccoli L, Park YJ, Tortorici MA, Czudnochowski N, Walls AC, Beltramello M, Silacci-Fregni C, Pinto D, Rosen LE, Bowen JE, et al. Mapping neutralizing and immunodominant sites on the SARS-CoV-2 Spike receptor-binding domain by structure-guided high-resolution serology. Cell. 2020;183(4):1024–42.e21. doi:10.1016/j.cell.2020.09.037.
- Shi R, Shan C, Duan X, Chen Z, Liu P, Song J, Song T, Bi X, Han C, Wu L, et al. A human neutralizing antibody targets the receptor-binding site of SARS-CoV-2. Nature. 2020;584(7819):120–11. doi:10.1038/s41586-020-2381-y.
- Liu L, Wang P, Nair MS, Yu J, Rapp M, Wang Q, Luo Y, Chan JF, Sahi V, Figueroa A, et al. Potent neutralizing antibodies against multiple epitopes on SARS-CoV-2 spike. Nature. 2020;584(7821):450–56. doi:10.1038/s41586-020-2571-7.
- Chi X, Yan R, Zhang J, Zhang G, Zhang Y, Hao M, Zhang Z, Fan P, Dong Y, Yang Y, et al. A neutralizing human antibody binds to the N-terminal domain of the Spike protein of SARS-CoV-2. Science. 2020;369(6504):650–55. doi:10.1126/science.abc6952.
- Baum A, Fulton BO, Wloga E, Copin R, Pascal KE, Russo V, Giordano S, Lanza K, Negron N, Ni M, et al. Antibody cocktail to SARS-CoV-2 spike protein prevents rapid mutational escape seen with individual antibodies. Science. 2020;369(6506):1014–18. doi:10.1126/science.abd0831.
- Premkumar L, Segovia-Chumbez B, Jadi R, Martinez DR, Raut R, Markmann A, Cornaby C, Bartelt L, Weiss S, Park Y, et al. The receptor binding domain of the viral spike protein is an immunodominant and highly specific target of antibodies in SARS-CoV-2 patients. Sci Immunol. 2020;5(48). doi:10.1126/sciimmunol.abc8413.
- Anderson EM, Goodwin EC, Verma A, Arevalo CP, Bolton MJ, Weirick ME, Gouma S, McAllister CM, Christensen SR, Weaver J, et al. Seasonal human coronavirus antibodies are boosted upon SARS-CoV-2 infection but not associated with protection. Cell. 2021;184(7):1858–64.e1810. doi:10.1016/j.cell.2021.02.010.
- Ng KW, Faulkner N, Cornish GH, Rosa A, Harvey R, Hussain S, Ulferts R, Earl C, Wrobel AG, Benton DJ, et al. Preexisting and de novo humoral immunity to SARS-CoV-2 in humans. Science. 2020;370(6522):1339–43. doi:10.1126/science.abe1107.
- Grifoni A, Weiskopf D, Ramirez SI, Mateus J, Dan JM, Moderbacher CR, Rawlings SA, Sutherland A, Premkumar L, Jadi RS, et al. Targets of T cell responses to SARS-CoV-2 Coronavirus in humans with COVID-19 disease and unexposed individuals. Cell. 2020;181(7):1489–501.e1415. doi:10.1016/j.cell.2020.05.015.
- Ladner JT, Henson SN, Boyle AS, Engelbrektson AL, Fink ZW, Rahee F, D’-Ambrozio J, Schaecher KE, Stone M, Dong W, et al. Epitope-Resolved profiling of the SARS-CoV-2 antibody response identifies cross-reactivity with endemic human coronaviruses. Cell Rep Med. 2021;2(1):100189. doi:10.1016/j.xcrm.2020.100189.
- Zhang L, Cao L, Gao XS, Zheng BY, Deng YQ, Li JX, Feng R, Bian Q, Guo XL, Wang N, et al. A proof of concept for neutralizing antibody-guided vaccine design against SARS-CoV-2. Natl Sci Rev. 2021;8(8):nwab053. doi:10.1093/nsr/nwab053.
- Liu C, Ginn HM, Dejnirattisai W, Supasa P, Wang B, Tuekprakhon A, Nutalai R, Zhou D, Mentzer AJ, Zhao Y, et al. Reduced neutralization of SARS-CoV-2 B.1.617 by vaccine and convalescent serum. Cell. 2021;184(16): 4220–4236.e13. doi:10.1016/j.cell.2021.06.020.
- Wang L, Zhou T, Zhang Y, Yang ES, Schramm CA, Shi W, Pegu A, Oloniniyi OK, Henry AR, Darko S, et al. Ultrapotent antibodies against diverse and highly transmissible SARS-CoV-2 variants. Science. 2021;373(6556):6556. doi:10.1126/science.abh1766.
- Barbas CF 3rd, Kang AS, Lerner RA, Benkovic SJ. Assembly of combinatorial antibody libraries on phage surfaces: the gene III site. Proc Natl Acad Sci U S a. 1991;88(18):7978–82. doi:10.1073/pnas.88.18.7978.
- Chen Z, Ren X, Yang J, Dong J, Xue Y, Sun L, Zhu Y, Jin Q. An elaborate landscape of the human antibody repertoire against enterovirus 71 infection is revealed by phage display screening and deep sequencing. mAbs. 2017;9(2):342–49. doi:10.1080/19420862.2016.1267086.
- Somasundaram R, Choraria A, Antonysamy M. An approach towards development of monoclonal IgY antibodies against SARS CoV-2 spike protein (S) using phage display method: a review. Int Immunopharmacol. 2020;85:106654. doi:10.1016/j.intimp.2020.106654.
- Andreano E, Nicastri E, Paciello I, Pileri P, Manganaro N, Piccini G, Manenti A, Pantano E, Kabanova A, Troisi M, et al. Extremely potent human monoclonal antibodies from COVID-19 convalescent patients. Cell. 2021;184(7):1821–35.e1816. doi:10.1016/j.cell.2021.02.035.
- Zheng Z, Monteil VM, Maurer-Stroh S, Yew CW, Leong C, Mohd-Ismail NK, Cheyyatraivendran Arularasu S, Chow VTK, Lin RTP, Mirazimi A, et al. Monoclonal antibodies for the S2 subunit of spike of SARS-CoV-1 cross-react with the newly-emerged SARS-CoV-2. Euro Surveill. 2020;25(28). doi:10.2807/1560-7917.ES.2020.25.28.2000291.
- Nguyen-Contant P, Embong AK, Kanagaiah P, Chaves FA, Yang H, Branche AR, Topham DJ, Sangster MY, Ellebedy A, Schultz-Cherry S. S protein-reactive IgG and memory B cell production after human SARS-CoV-2 infection includes broad reactivity to the S2 subunit. mBio. 2020;11(5). doi:10.1128/mBio.01991-20.
- Poh WP, Narasaraju T, Pereira NA, Zhong F, Phoon MC, Macary PA, Wong SH, Lu J, Koh DR, Chow VT. Characterization of cytotoxic T-lymphocyte epitopes and immune responses to SARS coronavirus spike DNA vaccine expressing the RGD-integrin-binding motif. J Med Virol. 2009;81(7):1131–39. doi:10.1002/jmv.21571.
- Braun J, Loyal L, Frentsch M, Wendisch D, Georg P, Kurth F, Hippenstiel S, Dingeldey M, Kruse B, Fauchere F, et al. SARS-CoV-2-Reactive T cells in healthy donors and patients with COVID-19. Nature. 2020;587(7833):270–74. doi:10.1038/s41586-020-2598-9.
- Meckiff BJ, Ramírez-Suástegui C, Fajardo V, Chee SJ, Kusnadi A, Simon H, Eschweiler S, Grifoni A, Pelosi E, Weiskopf D, et al. Imbalance of regulatory and cytotoxic SARS-CoV-2-reactive CD4(+) T Cells in COVID-19. Cell. 2020;183(5):1340–53.e1316. doi:10.1016/j.cell.2020.10.001.
- Le Bert N, Tan AT, Kunasegaran K, Tham CYL, Hafezi M, Chia A, Chng MHY, Lin M, Tan N, Linster M, et al. SARS-CoV-2-Specific T cell immunity in cases of COVID-19 and SARS, and uninfected controls. Nature. 2020;584(7821):457–62. doi:10.1038/s41586-020-2550-z.
- Weiskopf D, Schmitz KS, Raadsen MP, Grifoni A, Okba NMA, Endeman H, van den Akker JPC, Molenkamp R, Koopmans MPG, van Gorp ECM, et al. Phenotype and kinetics of SARS-CoV-2-specific T cells in COVID-19 patients with acute respiratory distress syndrome. Sci Immunol. 2020;5(48). doi:10.1126/sciimmunol.abd2071.
- Zheng J. SARS-CoV-2: an emerging Coronavirus that causes a global threat. Int J Biol Sci. 2020;16(10):1678–85. doi:10.7150/ijbs.45053.
- Du P, Gao GF, Wang Q. The mysterious origins of the Omicron variant of SARS-CoV-2. Innovation (New York, NY). 2022;3:100206. doi:10.1016/j.xinn.2022.100206.
- Martin DP, Lytras S, Lucaci AG, Maier W, Grüning B, Shank SD, Weaver S, MacLean OA, Orton RJ, Lemey P, et al. Selection analysis identifies unusual clustered mutational changes in Omicron lineage BA.1 that likely impact Spike function. Mol Biol Evol. 2022. doi:10.1093/molbev/msac061.
- Peacock TP, Brown JC, Zhou J, Thakur N, Newman J, Kugathasan R, Sukhova K, Kaforou M, Bailey D, Barclay WS. The SARS-CoV-2 variant, Omicron, shows rapid replication in human primary nasal epithelial cultures and efficiently uses the endosomal route of entry. 2022. bioRxiv. doi:10.1101/2021.12.31.474653.
- Bate N, Savva CG, Moody PC, Brown EA, Ball JK, Schwabe JW, Sale JE, Brindle NP. In vitro evolution predicts emerging CoV-2 mutations with high affinity for ACE2 and cross-species binding. 2021.2012.2023.473975. 2021.
- Dejnirattisai W, Huo J, Zhou D, Zahradník J, Supasa P, Liu C, Duyvesteyn HME, Ginn HM, Mentzer AJ, Tuekprakhon A, et al. SARS-CoV-2 Omicron-B.1.1.529 leads to widespread escape from neutralizing antibody responses. Cell. 2022;185(3):467–84.e415. doi:10.1016/j.cell.2021.12.046.
- Garcia-Beltran WF, St Denis KJ, Hoelzemer A, Lam EC, Nitido AD, Sheehan ML, Berrios C, Ofoman O, Chang CC, Hauser BM, et al. mRNA-Based COVID-19 vaccine boosters induce neutralizing immunity against SARS-CoV-2 Omicron variant. Cell. 2022;185(3):457–66.e454. doi:10.1016/j.cell.2021.12.033.
- Planas D, Saunders N, Maes P, Guivel-Benhassine F, Planchais C, Buchrieser J, Bolland WH, Porrot F, Staropoli I, Lemoine F, et al. Considerable escape of SARS-CoV-2 Omicron to antibody neutralization. Nature. 2022;602(7898):671–75. doi:10.1038/s41586-021-04389-z.
- Cao Y, Wang J, Jian F, Xiao T, Song W, Yisimayi A, Huang W, Li Q, Wang P, An R, et al. Omicron escapes the majority of existing SARS-CoV-2 neutralizing antibodies. Nature. 2022;602(7898):657–63. doi:10.1038/s41586-021-04385-3.
- Jennewein MF, MacCamy AJ, Akins NR, Feng J, Homad LJ, Hurlburt NK, Seydoux E, Wan YH, Stuart AB, Edara VV, et al. Isolation and characterization of cross-neutralizing coronavirus antibodies from COVID-19+ subjects. Cell Rep. 2021;36(2):109353. doi:10.1016/j.celrep.2021.109353.
- Wang C, van Haperen R, Gutiérrez-Álvarez J, Li W, Okba NMA, Albulescu I, Widjaja I, van Dieren B, Fernandez-Delgado R, Sola I, et al. A conserved immunogenic and vulnerable site on the coronavirus spike protein delineated by cross-reactive monoclonal antibodies. Nat Commun. 2021;12(1):1715. doi:10.1038/s41467-021-21968-w.
- Chen EC, Gilchuk P, Zost SJ, Suryadevara N, Winkler ES, Cabel CR, Binshtein E, Chen RE, Sutton RE, Rodriguez J, et al. Convergent antibody responses to the SARS-CoV-2 spike protein in convalescent and vaccinated individuals. Cell Rep. 2021;36(8):109604. doi:10.1016/j.celrep.2021.109604.
- Huang KA, Tan TK, Chen TH, Huang CG, Harvey R, Hussain S, Chen CP, Harding A, Gilbert-Jaramillo J, Liu X, et al. Breadth and function of antibody response to acute SARS-CoV-2 infection in humans. PLoS Pathog. 2021;17(2):e1009352. doi:10.1371/journal.ppat.1009352.
- Shiakolas AR, Kramer KJ, Wrapp D, Richardson SI, Schäfer A, Wall S, Wang N, Janowska K, Pilewski KA, Venkat R, et al. Cross-Reactive coronavirus antibodies with diverse epitope specificities and Fc effector functions. Cell Rep Med. 2021;2(6):100313. doi:10.1016/j.xcrm.2021.100313.
- Amanat F, Thapa M, Lei T, Ahmed SMS, Adelsberg DC, Carreño JM, Strohmeier S, Schmitz AJ, Zafar S, Zhou JQ, et al. SARS-CoV-2 mRNA vaccination induces functionally diverse antibodies to NTD, RBD, and S2. Cell. 2021;184(15):3936–48.e3910. doi:10.1016/j.cell.2021.06.005.
- Liao B, Chen Z, Zheng P, Li L, Zhuo J, Li F, Li S, Chen D, Wen C, Cai W, et al. Detection of anti-SARS-CoV-2-S2 IgG is more sensitive than anti-RBD IgG in identifying asymptomatic COVID-19 patients. Front Immunol. 2021;12:724763. doi:10.3389/fimmu.2021.724763.
- Ma ML, Shi DW, Li Y, Hong W, Lai DY, Xue JB, Jiang HW, Zhang HN, Qi H, Meng QF, et al. Systematic profiling of SARS-CoV-2-specific IgG responses elicited by an inactivated virus vaccine identifies peptides and proteins for predicting vaccination efficacy. Cell Discovery. 2021;7(1):67. doi:10.1038/s41421-021-00309-7.
- Dawood RM, El-Meguid MA, Salum GM, El-Wakeel K, Shemis M, El Awady MK. Bioinformatics prediction of B and T cell epitopes within the spike and nucleocapsid proteins of SARS-CoV2. J Infect Public Health. 2021;14(2):169–78. doi:10.1016/j.jiph.2020.12.006.
- Gillot C, Douxfils J, Cadrobbi J, Laffineur K, Dogné JM, Elsen M, Eucher C, Melchionda S, Modaffarri É, Tré-Hardy M, et al. An original ELISA-based multiplex method for the simultaneous detection of 5 SARS-CoV-2 IgG antibodies directed against different antigens. J Clin Med. 2020;9(11):3752. doi:10.3390/jcm9113752.
- Demers-Mathieu V, DaPra C, Mathijssen G, Sela DA, Jarvinen KM, Seppo A, Fels S, Medo E. Human milk antibodies against S1 and S2 subunits from SARS-CoV-2, HCoV-OC43, and HCoV-229E in Mothers with a confirmed COVID-19 PCR, viral SYMPTOMS, and unexposed mothers. Int J Mol Sci. 2021;22(4):1749. doi:10.3390/ijms22041749.