ABSTRACT
Flaviviruses are arthropod-borne viruses (arboviruses) that have been recently considered among the significant public health problems in defined geographical regions. In this line, there have been vaccines approved for some flaviviruses including dengue virus (DENV), Japanese encephalitis virus (JEV), yellow fever virus (YFV), and tick-borne encephalitis virus (TBEV), although the efficiency of such vaccines thought to be questionable. Surprisingly, there are no effective vaccine for many other hazardous flaviviruses, including West Nile and Zika viruses. Furthermore, in spite of approved vaccines for some flaviviruses, for example DENV, alternative prophylactic vaccines seem to be still needed for the protection of a broader population, and it originates from the unsatisfying safety, and the efficacy of vaccines that have been introduced. Thus, adenovirus vector-based vaccine candidates are suggested to be effective, safe, and reliable. Interestingly, recent widespread use of adenovirus vector-based vaccines for the COVID-19 pandemic have highlighted the importance and feasibility of their widespread application. In this review, the applicability of adenovirus vector-based vaccines, as promising approaches to harness the diseases caused by Flaviviruses, is discussed.
Introduction
The genus Flavivirus, from Flaviviridae family, comprises dengue (DENV), Japanese encephalitis (JEV), yellow fever (YFV), tick-borne encephalitis (TBEV), West Nile (WNV), Zika (ZIKV), and many additional viral pathogens that cause encephalitis. As demonstrated in Supplemental Table S1, most of the flaviviruses infect warm-blooded hosts through blood-meals of infected vectors, and hence such flaviviruses are classified as arthropod-borne viruses (arboviruses). Infection by flaviviruses may lead to shock, encephalitis, hemorrhagic fever, and such infections cause substantial mortalities. For instance, it is not surprising that 100 million new cases of DENV, are detected each year in DENV infested regions that harbor more than 2.5 billion people.Citation1 Another point is the fact that some hazardous flaviviruses tend to be zoonotic and it impacts the epidemiology of such pathogens (Supplemental Table S1). Thus, effective vaccines seem to be required to control such arboviruses.
A deno-associated virus, human adenovirus, and retroviruses are examples of viral transgene technology.Citation2 Viral vectors have modified genomic DNA or RNA contents, comprising manipulated genomes that make them noninfectious. Aiming development of anti-flavivirus vaccines, numerous such vectors have been developed for not only DENV,Citation3–17 but also, ZIKV,Citation18–28 WNV,Citation29–32 YFV,Citation33 JEV,Citation34–40 and TBEVCitation41–43 (). Prominent examples of viral vectors can be found in various DNA viruses (Poxviridae, Herpesviridae, Papovaviridae, and Adenoviridae), as well as RNA viruses (Togaviridae, Picornaviridae, Rhabdoviridae, Paramyxoviridae, and Orthomyxoviridae) ().Citation44 Adenovirus vectors (AdV) have been widely used for genetic manipulation of mammalian cells. Some advantages of AdVs include: (a) Versatile applications in the researches on vaccines; (b) A diverse array of dividing or non-dividing cells can be infected with AdVs; (c) Purification of high titers of such viruses is easily feasible; (d) Recombinant adenovirus strains (Ad2, Ad5) are well characterized and (e) Genetic transfers up to 37 kb of multiple foreign genes seems to be feasible in case of some AdVs. Hence, AdVs can be suitable candidates for developing pan-genus, likely pan-Flavivirus vaccines.Citation45 One of the main advantages of AdVs is the fact that the integration of their manipulated genome into the host chromosome tend to be impossible, and this property has made AdVs useful transient gene expression tools. AdV-based vaccine candidates have shown to be effective and safe against viral pathogens.Citation46 It has been highlighted that AdVs elicit suitable levels of protective responses against important flaviviruses. Thus, delivery of protein antigens is simply feasible using such vectors that can induce defined immune reactions in humans. Induction of wide anti-arboval immune system reactions tend to be a characteristic feature of successful and safe AdV-based vaccines, which is an urgent need in case of flaviviral pathogens. In this line, several AdV-based vaccines have been recently developed for SARS-CoV-2 and millions of doses have been applied so far (Supplemental Table S2).
Table 1. Viral vectors used as candidate vaccines against important flaviviruses. Viral vectors, target infectious Flavivirus, and target antigens are summarized.
A denoviruses mimic a natural viral infection that results in a considerable inflammatory, reaction, and it is why these viruses have been recently considered as reliable priming vaccine vectors. Different preclinical and clinical investigations have shown that the recombinant Ad (rAd) induce vigorous and long-lasting cellular and humoral responses against the studied transgene product.Citation45,Citation65 The AdV vaccines have been increasingly used in recent years as efficient platforms primarily due to their high yield in cell culture, excellent safety profiles, and capacity to induce strong inflammatory immune responses.Citation29,Citation66 However, rare incidence of a pathophysiological condition, known as vaccine-induced thrombotic thrombocytopenia (VITT), has been recently reported for some anti-SARS-CoV-2 AdV vaccines, including Johnson & Johnson and AstraZeneca which are approved for emergency use in several countries. In this regard, national authorities of the USA, and some other countries have temporarily paused the administration of Johnson & Johnson vaccine (Ad26.COV2-S) since rare cases of severe thrombosis with thrombocytopenia were reported. Similarly, rare cases of VITT have been reported in case of AstraZeneca vaccine (ChAdOX1-nCoV-19). Hence, health authorities of the USA, including FDA/CDC, have recently conducted a risk/benefit analysis which revealed the fact that despite the risk of developing the VITTS, the risk/benefit strongly encouraged vaccination by those vaccines.Citation67,Citation68
Regarding the aforementioned explanations, we aimed to provide the latest updates of using AdV for the provision of vaccines against important flaviviruses to show the evidence of successful attempts, as well as some common or virus-specific features. In this review, databases in PubMed, Google Scholar, and Scopus were searched using the keywords Adenovirus + Vaccine + Flavivirus + ZIKV/DENV/WNV/YFV/JEV/TBEV, and retrieved 34 papers that used AdV vaccines against ZIKV, DENV, WNV, YFV, JEV, and TBEV. Furthermore, regarding the Covid-19 pandemic, the available situation of SARS-CoV-2 AdV-Based vaccines have been also discussed comparatively. Here, we provide evidence that AdV-based vaccines can be assumed as an efficient strategy to harness Flavivirus infections.
Adenoviruses
Adenovirus genome, structure, and biology
The adenoviral genome normally comprises a molecule of DNA (double-stranded, 34–43 kb). This DNA molecule encodes for about 50 proteins, and it also contains two flanking inverted terminal repeats (ITRs) and a packaging signal. The coding genes in adenoviral genome are described according to their transcriptional order: E1–4 proteins are encoded by the early genes. E proteins govern the replication of viral DNA. Genes L1–5 are indeed late genes encoded for structural proteins, including, fiber, hexon, and penton bases. The translation product of E1 gene is a protein that turns the infected host cell into viral replication factories. E2 gene encodes the protein which is essential for viral DNA polymerization. Moving forward, the E3 gene encodes for host immune modulating protein.Citation69 Finally, the E4 gene products have also roles in viral replication. The rest of the adenovirus genome mainly comprises the late gene (L1–L5). The Ad major late promoter (MLP) governs the synthesis of a single late pre-mRNA. Late mRNAs are produced through splicing of this late pre-mRNA.Citation70,Citation71
Considering the role of adenovirus proteins, it is clear that replicative genes of their vectors are deleted and affect the applicability of the adenovirus as a vector vaccine approach. Hence, deletion of a defined E gene from the adenovirus may have influences on the vector immunogenicity. A benefit of such genetic manipulation is the fact that the immune reaction induced by the remnants of viral proteins can have a role in immunization against the target pathogen.Citation68
Adenoviral vectors
The replication-incompetent type AdV is not only the first AdV introduced, but also is the most broadly investigated type of AdV.Citation72 Deletion of E1 is the common property of such vectors, and so, this protein must be provided in trans to produce recombinant viruses. In addition, the viral E3 region is typically deleted and the viral E4 region in some generations is removed not only aiming improvement of coding capacity, but also reducing the in vivo pro-inflammatory reactions.Citation72 Recombinant adenoviral vector (rAdV) particles can be produced after the antigen-expressing cassette cloned in a shuttle adenoviral plasmid. This rAdV and the backbone structure, comprising E1/E3-deleted AdV, will be transformed into specific bacterial strains such as BJ5183, concomitantly (). Thus, the E1 region of the AdV will harbor the antigen-expressing cassette.Citation72,Citation73 Then, the resulting recombinant plasmid obtained through homologous recombination of the above mentioned two constructs will be transfected into a mammalian host cell encoding AdV E1 gene such as HEK-293 cell line. Hence, the infected host cell will stably express the Ad E1 proteins, and the AdVs virions will be produced ( and ).
Figure 1. Flavivirus structure and antigen presentation. Schematics of Flavivirus particle containing three structural proteins: C, M, and E; A: Genome organization and polyprotein processing: Flavivirus genome is translated as a single polyprotein that is cleaved co- and post-translationally to viral three structural proteins C, M, E, and 7 NS proteins. The 5′ and 3′ un-translated regions (UTR) are indicated as black lines at the end of the viral genome; B: the sequences encoding the prM, E proteins and NS1 of the target Flavivirus; C: in the Adenovector System, a Shuttle vector is cloned with the cDNA of interest. As the next step, following linearization the formed plasmid together with the viral DNA plasmid Adenoviral Backbone ΔE1/E3 transformed into RecA+ bacterial strain (E. coli strain BJ5183). The resulting plasmid lacks E1 and E3. In cells, the complementation of E1 functions can occur. Following homologous recombination, selection of the clones occurs with antibiotic (kanamycin). Then the clones can be screened by restriction enzyme analysis; D: the cleavage of the recombinant adenoviral construct is facilitated using PacI which help expose its Inverted Terminal Repeats (ITR). Now, the transfection of cells to produce viral particles can be performed; E: the final steps are the collection, amplification, and concentration of the recombinant adenoviruses followed with titration of an appropriate dosage for in vivo application and injection into given model animals (e.g. Rhesus monkey).
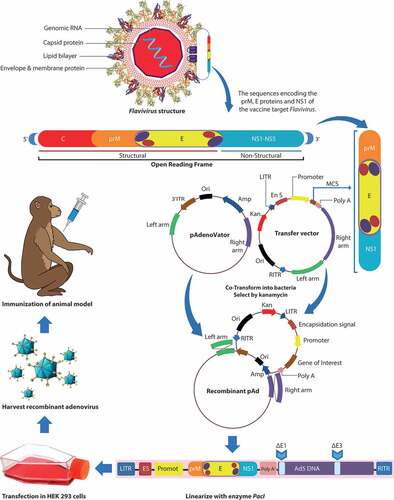
Figure 2. Schematic representation of the adenovirus genome (Ad5) and generations (first, second, and third generation) of adenovirus-based vectors. Map of adenovirus serotype 5 genome and different generations of adenoviral vectors. Early transcripts are represented by E1–E4 regions and late transcripts are represented by L1–L5 regions. MLP: major late promoter; ψ: packaging signal.
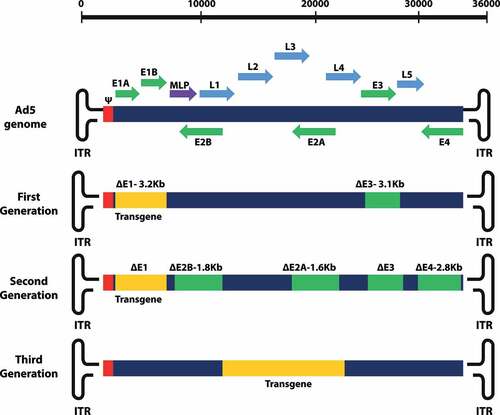
Construction of adenoviral vectors
First-generation adenoviral vectors
The first generation AdVs do not harbor the E1 or less often the E3 region. The products of the E1 region are proteins that govern the order and the level of expression for some other genes, including early and late genes. Multiple genetic deletions in E1 region, up to 3 kb, have been made. Hence, the cloning site of these vectors comprises the E1, and in some recent cases, the E3 regions which can harbor the transgenes ().Citation74 Such vectors have been widely used in cancer and also vaccine research. Such a wide application of these viral biotechnology approaches is mainly associated to acceptable efficiencies in the transfection of cell lines that cannot be transfected through other approaches.Citation74 Adenovirus can further proliferate in E1 complementing cells, such as HEK-293 cells. However, since reverse recombination between the E1 gene of this cell line with recombinant adenovirus 5 (Ad5) viruses is possible, which leads to replication capable adenoviruses (RCA), another cell line, PerC6, is more favorable for the viral amplification.Citation75 In place, the products of E3 region are not necessary for viral growth in vitro, but interfere with host defense mechanisms. Hence, the first-generation AdVs with E3 deletions have a wider cell line specificity compared to those of AdVs with E1 deletions.Citation74 Also, adenoviruses with E1 deletion can harbor genetic insertions of up to 5.1 kb, and it is an disadvantage since insertions of about 8.2 kb can be cloned into the genome of adenoviruses which lost E1 and E3 regions.Citation74
Genetic engineering methods have been used to improve the Ad genome, leading to safety and effectivity of AdVs as vaccines in humans.Citation76 However, a hazardous safety issue in first -generation AdVs is the RCA which is likely to occur mainly due to the similarity of the genome of such adenoviruses to the E1 insertions in the virus-producing host cell, such as HEK293 cell line. Such a phenomenon may occur with detectable multiplicities of infection (MOI), where the E1 region will be unnecessary for replication. The expression of specific genes in host cells leads cells to the proliferative state although it can also cause the production of some proteins with activities similar to E1A. DNA replication of adenovirus will become leaky under such circumstances which lead to interference through the late protein production. Late proteins have some cytotoxic effects and their accumulation impacts the transduced cells, and such cytotoxicity elicits the cellular immune responses in affected cells. The above-mentioned shortcoming can be prevailed by selecting cell lines with minimal genome similarity to the given E1 insertion, for example, PERC.6 cell line.Citation77 Additionally, in vivo studies have shown that the transgene expression observed with E1-deleted vectors is not persistent and such a disadvantage has been made some medical applications questionable.Citation75
Considering de novo biosynthesis of viral proteins in first-generation AdVs, the potent immune response against antigenic transgenes is inducible. In case of E proteins of Flavivirus, not only neutralizing antibodies, but also T cell-mediated reactions have been reported.Citation78 Vaccination practices by first-generation AdVs, likely Ad5-prM-E and Ad4-prM-E, have shown a general development of T-cell immune responses.Citation79 The detectable T-cell-mediated response together with elicitation of antibody response in Ad4-prM/E vaccination have indicated a biased immune response in favor of cellular than humoral reaction. Such a detectable T cell-mediated immune response can have promising protective effects in case of defined Flavivirus pathogens, including DENV,Citation80 JEV,Citation34 WNV,Citation29and ZIKV.Citation48,Citation49 Bullard et al. showed that above-mentioned immunological differences originated from the serotype-specificicity induced by adenovirus vectors. Various studies concerning Flaviviruses have shed light on the fact that the first generation AdV vaccines elicit a detectable anti-epitope E (immunodominant) T-cell-mediated reaction.Citation27 Vaccination with Ad4-prM/E has also been shown to elicit a promising T-ZIKV cell-mediated reaction with an insignificant antibody-mediated response against ZIKV. In place, using Ad5-prM/E as a vaccine causes not only elicitation of anti-ZIKV antibodies, but also detectable T-cell-mediated reaction as revealed in mice.Citation49 Additionally, self-adjuvanting properties have been reported for Ad, and differences have been reported in the cytokine profiles in case of Ad4 and Ad5 vectors. It has been highlighted that the cytokine profile in Ad4 immunized mice is highly T-helper type 1 (Th1) biased while mice immunized by Ad5 have shown to be more Th1/Th2 balanced.Citation81 Recent studies have shown that such a phenomenon originates from the serotype-specific immune profiles elicited by different AdV types.
Second-generation adenoviral vectors
Considering the cytotoxic and immunogenic effects observed in first-generation AdVs, vector optimizations were regarded which resulted in second-generation AdVs. The second-generation AdVs capacity for transgenes has been increased through further omission of E2 and E4 regions. Hence, E1 or E1 and E3 genome regions together with E2 or E2 and E4 regions have been deleted in second-generation AdVs ().Citation82 Consequently, the characteristic leaky viral gene expression profile in host cells cannot be observed in second-generation AdVs, a phenomenon that was a drawback in first-generation AdVs.Citation83 Also, the probability of RCA formation is minimized. In second-generation AdVs, gene inactivations have been performed on genetic regions enrolling in DNA duplication. However, gene deletions in AdVs should be performed in a way that allows insertion of transgenes as large as four separate expression cassettes which can cover up to 14 kb. A higher level of long term transgene expression is seen in second generation AdVs. Collectively, despite the increased transgene capacity, second-generation AdVs have had limited benefits over their first-generation counterparts.Citation45 However, a significantly lower level of virus production yield of adenovirus in host cells is known as the main drawback for the second generation adenoviruses. Furthermore, trans-complementation during the vector proliferation in the host cells can be impacted by cytotoxicity of early gene products, including E2 and E4 products. Such vectors harbor inducible expression systems (IES); however, the virus amplification can be affected, which can have its influences on the vector yields and vector titer.Citation84 Furthermore, the viral proteins can be produced weakly during such reduced transgene expression. Thus, not only the cytotoxicity but also, the immune response induced by these viral vectors are assumed as major drawbacks which affect the applicability of such viral biotechnology approaches.Citation85 Also, further deletion of E2 and E4 regions in second-generation vectors can further decrease immunogenicity. The products of E2, E3, and E4 regions in the adenovirus genome seem to have roles not only in virus replication, but also in modulation of the intracellular immune responses. Hence, deletion of such genomic regions may lead to enhanced packaging capacity of vectors for transgenic sequences. However, second-generation AdVs have still shown degrees of immunogenicity and cytotoxicity.Citation84
Second-generation AdVs may lead to some immune system interferences. Yang et al. (1995) have highlighted the fact that intravenous administration of the aforementioned vectors can cause detectable hepatotoxicity.Citation86 Also, elicitation of a significant immune response against AdVs has been reportedly observed which inhibited repeated treatments.Citation84 As an advantage, such vectors can be successful long-term gene expression platforms.Citation87 Interestingly, COVID-19 vaccine candidates based on second-generation adenoviruses have been designed that encode for multiple SARS-CoV-2 proteins (ClinicalTrials.gov: NCT04732468, NCT04591717 and NCT04710303). Results of experiments performed on HEK293 cells showed the primary role of such proteins for replication of SARS-CoV-2. Hence, the cell can have a critical role for providing them which can elicit a long-term memory T cells-mediated immunity.Citation88
Third generation high-capacity adenoviral vectors (HCAs)
The third class of AdVs is so-called gutless or helper-dependent adenoviruses.Citation89 In third-generation AdVs, the whole adenovirus DNA sequences are deleted, except the cis-acting sequences which are indeed necessary in case of viral DNA duplication and particle packaging. Thus, such vectors seem to be reliable platforms for gene transfer purposes in a varied range of cell types originated from various species (). The above-mentioned deletions of all viral genes might be why vector-associated acute toxicity is minimized in such vectors. However, such cytotoxic effects can only be detected in case of detectable HCAs doses.Citation89 Generation of HCAs is highly complex because separation of sequences encoding for capsid and also packaging the therapeutic DNA is tedious. Following cell transduction, the genome of the third generation AdVs remains episomal in the nuclei. This Ad genome remains associated with histones of the host cells. Then it undergoes repression or may become transcriptionally active depending on the nature of the cloned sequences.Citation89 Additionally, neutralizing antibodies against AdV affect the efficiency of transduction which leads to reduction of adaptive immune response.Citation90 The possibility of in-trans providing of the viral proteins via co-infection is the prominent difference of gutless AdVs which differentiate them from the other AdV generations. Hence, a helper adenovirus is regarded in applications of third-generation AdVs. Hence, an AdV with a first-generation genetic content is provided as a helper virus in this system. This helper virus harbors a packaging signal which is flanked by two loxP-sites. In comparison, the producer cells provide the above-mentioned proteins in trans for the first and also second-generation AdVs. Thus, it is why such high-capacity adenoviral vectors are so-called helper-dependent.Citation91 Another discriminating feature of these viral biotechnology-based products is the fact that multiple transgenes, up to 37 kb length, can be cloned in such AdVs. Cell lines with detectable Cre-recombinase expression, likely modified HEK293, are used for production purposes. During virus proliferation, the producer cell is transduced with a helper virus and the third generation adenovirus genome. Expression of the helper virus genes causes the production of the helper virus proteins and viral capsid assembling. During such assembling step, the helper virus genome won’t become packaged since the packaging signal for the genome of the first generation helper virus is removed through the loxP-sites recombination which is performed via the activity of Cre-recombinase. Hence, the genomes of the HCA vector are packaged, exclusively. However, removing the packaging signal of the first generation helper virus is not always totally accomplished which leads to about 0.1–1% helper virus genome contaminations in HCA vector preparations.Citation92 Also, homologous recombination of genomes of helper virus with E1 gene tends to be inevitable which results in the formation of replication-competent adenoviruses.Citation93
Considering the increased cloning capacity, such gutted AdVs seems to be highly interesting for gene therapy purposes. Also, third generation AdVs have shown a long-term transgene expression potential. Furthermore, they have indicated insignificant cytotoxicity which is highly advantageous. As drawback, the production process for gutless AdVs seems to be highly tedious and has complexities for optimization. However, the significant frequency of contamination with helper viruses has affected the applications of such vector viruses.Citation89
Adenoviral vectors applications
As a conclusion, various generations of AdVs can have different applications depending on the purpose. First-generation AdVs seem to be very useful in cancer and vaccine design. Additionally, these viral biotechnology approaches tend to be applicable in the transfection of cell lines that other methods cannot transfect. In place, second-generation and third-generation AdVs have a characteristic long-term gene expression potential which make them potent gene vectors in the future.
Comparatively, the first-generation and also second-generation AdVs are known as successful vaccine vectors. However, the former has shown to be more immunogenic. The simplicity of large-scale manufacturing of first-generation AdVs has currently made them as a prevailed viral genetic tool. In place, third-generation AdVs can be produced with significantly lower efficiency. Furthermore, the helper Ad contamination in the produced virus stocks is the other main drawback in the helper-dependent AdVs production.
The second and third-generation AdVs can harbor very larger insertions when compared to the first-generation. Also, they have indicated a detectable stability together with an insignificant cytotoxicity. Intravenous administration of such vectors would result in robust adaptive immune responses after following the first in vivo immunization.Citation2,Citation89,Citation94
To date, many studies have used AdV to develop vaccines in human phases I-III clinical trials (Supplemental Table S2). AdV vaccines have been broadly administered in early stage clinical trials for an array of infectious diseases including ZIKA (ClinicalTrials.gov: NCT03356561, etc.), HIV (ClinicalTrials.gov: NCT00894114, etc.), HCV (ClinicalTrials.gov: NCT03688061, etc.), influenza (ClinicalTrials.gov: NCT01818362, etc.), and cancers (ClinicalTrials.gov: NCT03225989, NCT02390063, NCT03313596, etc.). Recently, some adenoviral-based vaccines for SARS-CoV-2 have also received an Emergency Authorization license.
Studies on adenoviral vector-based vaccines against flaviviruses
Zika virus (ZIKV)
Zika virus (ZIKV) is an arboviral infection caused by ZIKV. ZIKV, originated from Africa, is mainly transmitted to humans during the blood meal by an infected Aedes aegypti or vertical transmission.Citation95 This pathogen is currently distributed in Central America, South America, the Caribbean, the Pacific region and Southeast Asia.Citation96 The rise of ZIKV infections has shed light on the need for the development of an efficient and protective vaccine. However, introducing such a vaccine against ZIKV is regarded as a major worldwide health concern.
There are currently no specific available treatment or vaccine against ZIKV infection.Citation97 WHO has reported 18 ZIKV vaccine programs with a wide variety of formulations in 2016.Citation98 Live, inactivated, whole-virus, and subunit vaccines are among these vaccine candidates. In addition, messenger RNA (mRNA), DNA-, protein-, and vector-based formulations have also been studied.Citation98
Along the RNA genome of ZIKV, the prM/E region has been mostly selected for designing anti-Zika AdV vaccines ().Citation99 This selection can be highly associated to the fact that an appropriate selection of protein antigen is critically important in designing anti-Zika vaccines that determines the efficiency to induce promising cellular and humoral immunity.
A diverse array of anti-Zika vaccine platforms have been investigated in mouse and primate systems. Some of such vaccine candidates have been proved as highly efficacious in preclinical studies (). However, development of these vaccines encountered challenges for the clinical tests. A diverse array of ZIKV vaccine platforms, including DNA-based vaccines,Citation100 inactivated vaccines,Citation101,Citation102 live-attenuated vaccine (LAV),Citation103 mRNA vaccine,Citation104,Citation105 and vector-based vaccines, such as modified Vaccinia virus Ankara, measles virus, and AdVs, have been investigated ().Citation106
Table 2. Comparative analysis of adenoviral vectors based on the flaviviruses studied, used animal model, dosage, and immunization way.
Several studies have recently used different types of AdVs including Ad26,Citation19,Citation27,Citation50 RhAd52,Citation26,Citation50 Ad2,Citation21 Ad4,Citation48,Citation49 Ad5,Citation25,Citation51 ChAdoX1,Citation20 and GAd, and AdC7 to design vaccines against ZIKV in animal modelsCitation18–21–Citation48–50–Citation52 (). Such investigations have shown protective immune responses; mainly involving a significant elicitation of antibody mediated protection, and also a cellular immune response in miceCitation18–21–Citation25–48–Citation50,Citation51-53 and monkeyCitation26,Citation27,Citation49 models (). Among the array of AdVs that have been studied for anti-Zika Virus vaccine design purposes in human population, ChAdOx1 has entered two phase I clinical trials (ClinicalTrials.gov: NCT04015648 and NCT04440774) (Supplemental Table S2). Furthermore, Lopez-Camacho et al. (2018) evaluated four anti-Zika Virus vaccine candidates which were designed in replication-deficient chimpanzee AdVs (ChAdOx1) platform. Such vaccine candidates involved the prM/E addition or deletion along with the presence or absence of its trans-membrane domain (TM). It has been shown that ChAdOx1 prM/E ∆TM encoding prM and E without TM can have not only promising protective effects, but also long-lasting anti-E-mediated immunity.Citation20 In addition to the chimpanzee platform, GAd-Zvp which is a gorilla AdV-based vaccine candidate, encodes for the prM and E regions. This vaccine candidate seems to be a reliable platform to control some major flavivirus diseases, including congenital ZIKV infections. Interestingly, elicitation of a long-lasting B and T cell immune response has been reported as a reaction to a single dose administration of GAd-Zvp which have had preventive effects against ZIKV maternal-to-fetal transmission in immune-compromised and also immune competent-mice. Therefore, ChAd and GAd can be an effective viral vaccine vector in clinical trials in humans.Citation107
Considering the importance of vertical transmission of ZIKA during pregnancy, the selection of not only appropriate antigens from ZIKV, but also the type of AdV for developing effective AdV vaccines can cause persistent immunity in mothers which lead to child immunization, concomitantly.Citation21,Citation51 In this regard, Liu et al. (2018) developed three AdV vaccines; Ad2-E, Ad2-prM/E, and Ad2-prM/E-NS1 to be assessed in mice model. Ad2-prM/E induced higher titer of neutralizing antibodies to ZIKV compared to Ad2-E, showing that prM/M may have a role in the folding of immunogenic envelope protein. Additionally, Ad2-prM/E-NS1 indicated the most detectable inhibitory effects against the target virus when the ZIKV-infected cells were provided by immune serum. Hence, mice pups were challenged with ZIKV. In ZIKV-challenged newborn mice from immunized dams, Ad2-prM/E-NS1 conferred the highest protection, which led to prevention from weight loss, neurological defects, and inhibition of the virus proliferation. Furthermore, Ad2-prM/E showed higher protective effects when compared to Ad2-prM/E-NS1. In place, Ad2-E reduced the characteristic neurological symptoms, but has had no inhibitory effects against the virus duplication. Liu et al. (2018) suggested that NS1, prM/M and E should be concomitantly considered in the design of ZIKV vaccine.Citation21
Similarly, Kim et al. (2016) developed Zika vaccines with prototype subunit and also AdV that encoded for the extracellular terminal of the envelope protein of ZIKV fused to the T4 fibritin fold on trimerization domain (Efl). Immunization regimen of prime-boost caused the elicitation of the anti-Zika Virus antibodies. Also, pups born to Ad5.ZIKV-Efl-immunized mice have shown immunity which resulted in their survival after the lethal challenge. Furthermore, such pups have shown weight loss with or without neurological symptoms, while a partial protection (50%) have been observed in pups born to dams that received the MNA-ZIKV-rEfl vaccine.Citation51
Another interesting fact on the AdV application as vaccines is that administration of AdV vaccines against various flaviviruses via different roots such as intraperitoneal, intramuscular, subcutaneous and intranasal induces different levels of host immune responses. Steffen et al. (2020) developed a replication-deficient AdV vaccine which expressed the hAd5. This intranasal (hAd5-ZKV) vaccine triggered detectable immune responses against ZIKV prM and E proteins. The vaccine induced cell-mediated as well as humoral immune reactions against ZIKV. Furthermore, it elicited CD8+ T cells with specificity to the dominant T cell epitopes of ZIKV.Citation53 The early innate immune responses to Ad vaccination causes the overwhelming of antigen-presenting cells and/or innate immune cells at the injection site. Such inflammatory reactions may even impact the efficiency of the adaptive immune reactions.Citation108 Such a phenomenon has been also confirmed in Ad vector-based vaccines designed for other viruses, including anti-Hepatitis B vaccine. Interestingly, the intranasal route of infection has shown a much better protection against a challenge with hepatitis B virus (HBV) than intra-peritoneal inoculation. Additionally, the presence of cytotoxic T lymphocytes (CTL) in the draining lymph nodes should have synergistic effects in inducing such mucosal immunity in lungs.Citation109
Dengue virus (DENV)
DENV is a single-stranded sense-positive RNA Arbovirus (). This Flavivirus can be exclusively transmitted to humans through the biting mosquitoes, especially Aedes aegypti. There are four closely related serotypes of DENV: DENV1–4, all of which are capable of causing the same pathogenesis and disease. The risk of disease can be inferred from the fact that about 100 million new cases are detected, annually. Infection by a serotype cannot lead to cross-protection against the other serotypes. Hence, a characteristic protective vaccine is recommended to be tetravalent. The natural conformation of the envelope glycoprotein to induce neutralizing immune responses is the main problem to design efficient anti-dengue vaccines (). Additionally, the other drawback is the necessity of presenting antigens of all four serotypes which hampers the development of protective vaccines. Development of vaccines against DENV was started in early 1920s. Varied vaccine platforms have been introduced so far, including: tetravalent live attenuated candidates, genetically manipulated chimeric DENV and DENV-YFV active viruses, inactivated whole virus, recombinant E protein subunit platforms, DNA-based vaccines, and E or EDIII expressing viral vectors, including AdBVB vaccines.Citation1
Dengvaxia R manufactured by Sanofi Pasteur, Lyon, France, is a chimeric yellow fever virus-tetravalent dengue vaccine (CYD-TDV), which seems to be the only licensed available anti-dengue vaccine. However, additional DENV vaccine candidates, including tetravalent dengue DNA vaccine (TVDV manufactured by Naval Medical Research Center; MD, USA), DENVax (produced by Takeda; Tokyo, Japan), TV003 (produced by NIH; MD, USA), tetravalent dengue vaccine (TDEN) (manufactured by the Walter Reed Army Institute of Research (WRAIR; MD, USA), GlaxoSmithKine (produced by GSK; MD, USA), and V180 (manufactured by Merck; NJ, USA) are still in the process of clinical trials.Citation110,Citation111
It has been suggested that viral vectored vaccines are the best approach to induce cellular immunity, and hopefully to induce strong humoral responses.Citation44
A novel tetravalent adenovirus platform, (cAdVaxD/1–2, cAdVaxD/3–4) capable of expressing multiple antigens have been recently developed as a DENV vaccine by Holman et al. Aforementioned vectors were able to express high levels of prM and E proteins in vitro and also induced potent antibodies against all four DENV serotypes in vivo. The antibody responses were neutralizing and were against all serotypes, as the antigens were naturally expressed and associated with the membrane. In addition, the de novo-synthesized antigens induced reactive cellular immune responses. The data showed an efficient induction of immune responses against different serotypes by cAdVax-based vaccines. Additionally, the vaccine expressed significant levels of the DENV antigens in cells to mimic a natural infection. This vaccine also induced both humoral and cellular immune responses against different serotypes of DENV.Citation16
Furthermore, the development of two bivalent DENV vaccines, using a complex AdV, by incorporating the genes expressing prM and E proteins of DENV1, 2 (CAdVax-Den12) or types 3 and 4 (CAdVax-Den34) were studied on Rhesus macaques. Vaccinated animals produced high-titer of antibodies, that could neutralize of all four serotypes of DENV in vitro. The possibility of the vaccine to induce rapid, sustained, and protective immune responses was tested with two separate live-virus challenges administered at 4 and 24 weeks after the final vaccination. As observed in both challenges, protective responses against viremia were demonstrated for all serotypes of DENV.Citation9
Many studies have shown that multivalent vaccines using live, replicating viruses may have potential problems, presumably stemming from serotype competition and/or dominance.Citation9,Citation112 Interestingly, recent studies have shown that the tetravalent vaccines against four serotypes of DENV elicit an unbalanced immune response, specific to a single serotype. Such a biased immune response seems to be linked to viral interference which is observed when multivalent vaccines against multiple viral serotypes are mixed together and co-injected. Hence, AdV systems may have a role for creation of a single tetravalent DENV vaccine. Furthermore, AdVs seem to be advantageous since such vectors can harbor inserts of up to 35 kb.Citation10,Citation72
West Nile Virus (WNV)
West Nile Virus (WNV), comprising genetic lineages I and II, was first identified in Africa in 1937. Lineage I is associated with viral encephalitis which is a severe disease in human.Citation113 WNV can significantly affect the global society since it infects human and animals.Citation113,Citation114 The risk of WNV can be well understood from the fact that this virus causes infection in a very wide range of hosts, including farm animals and even bird species.Citation113 WNV is transmitted mainly by Culex tarsalis, Cx. quinquefasciatus, and Cx. Pipiens.Citation115,Citation116 This virus is distributed in Africa, southern Asia, northern Australia, and sporadically in more temperate regions of Europe.Citation117 In spite of international efforts to develop WNV vaccines, no approved vaccine for human use is available. On the other hand, several veterinary vaccines have been licensed, such as WNV-INNOVATOR,Citation118 Recombitek Equine WNV,Citation118 and WNV-INNOVATORDNA. Also, several clinical trials have been conducted including ChimeriVAX-WN02.Citation119 It has been shown that AdV vaccines could be promising candidates against WNV. Schepp et al. (2007) showed that multiple antigens synthesized de novo from a complex AdV vaccine (CAdVax) vector were capable of inducing both humoral and cellular immune responses against WNV in mice model.Citation29 Such an Ad vaccine vector (CAdVax) induced both humoral and cell-mediated immune responses.
In WNV cases, undetectable levels of Ad antigens are expressed. Hence, AdVs only express the WNV antigens and it is the advantage of AdVs versus other viral vectors such as alpha viruses or poxviruses when WNV antigens are to be expressed.Citation29
Yellow fever virus (YFV)
YFV can infect human via the blood-meal of defined species of YFV-positive mosquitoes. This virus spreads among some primates and several mosquito species that act as its vectors.Citation120 According to the statistics, around 200,000 infection cases are recorded annually, which results in around 30,000 deaths per year, with Africa as the hot point of this infection. Considering the risks of this viral infection live attenuated YFV vaccine (YF-17D) was introduced in 1937. WHO statistics concerning this Flavivirus has shown that about 500 million people have been vaccinated since its introduction. The protective efficacy of this vaccine is reported to be around 98% which can have its protective effects for at least 10 years.Citation120 Despite the general safety, there have been recently claims of serious adverse reactions, including hypersensitivity and YF vaccine-associated neurologic disease (YEL-AND). Hence, worldwide movements have recently addressed the development of new vaccines to control YFV.Citation121
To date, two anti-YFV AdV based vaccines have been reported which target the non-structural and structural (Ad-YF C, M, E or Ad-YF NS3) proteins of YFV virus. Bassi et al. (2016) have shown that a single administration of an AdV based vaccine, developed in their laboratory, which encode for the YF-17D non-structural protein 3, can cause a detectable CD8+ T-cell response, which finally lead to a considerable protective reaction as shown in intracranial challenge of vaccinated mice. Bassi et al. have also indicated that a complete protective response can only be observed when the administered AdV encodes for the YF-17D structural proteins. YFV-specific CD8+ T cell-mediated response together with a detectable rise of YFV-neutralizing antibodies has been reported in such immunizations. Interestingly, cumulative data have been in line with the role of both of above-mentioned components for a fully protective response in mouse model ().Citation33
Japanese encephalitis virus (JEV)
Japanese encephalitis virus, also known as “Orient’s plague”, is an Arbovirus that causes the infectious disease JEV in South Asia and the Western Pacific Regions. This Flavivirus, comprising five genotypes, is an enveloped arbovirus which is mainly transmitted by Culex mosquitoes to humans.Citation122 This virus was firstly identified in 1924. JEV Infection statistics has highlighted that about 3 billion people in above-mentioned tropical regions, comprising around 43% of current global population, seem to be at risk, which mirrors the high risk of this Flavivirus, worldwide. Also, it has been shown that approximately 68,000 JEV infection cases lead to around 20,000 deaths, annually.Citation123
Considering the global risk of JEV, effective vaccines have been proposed to be administered for controlling JEV. Inactivated whole virus and also live attenuated vaccines are the platforms developed to harness this disease. Some trials have shown that a vaccine with the inactivated platform has an efficacy of 81–95%.Citation124 This platform has been produced mainly in Japan although was discontinued since 2005. The other platform, live-attenuated vaccine, was developed and then has been produced in China since 1988.Citation125 Meanwhile, developing new platforms has been the target of many researches.
Appaiahgari et al. (2006) have shown that Ad5 vector based JEV vaccine, administered through the IN route, can have promising protection in mouse model. Similarly, Vrati (2010) revealed the same protection in mouse system, which was elicited through the administration of developed recombinant adenovirus. This RAdEs AdV encodes for JEV prM and Es that elicits a detectable immunogenicity in mouse model as reported by Vrati. The later research highlighted that RAdEs vector elicits a considerable titer of JEV neutralizing antibodies which has a complementing effect in protection of the immunized mice against JEV ().Citation34
Tick-Borne encephalitis virus (TBEV)
Tick-borne encephalitis virus (TBEV), is a human-infecting arbovirus that may cause mostly asymptomatic infections in humans. However, symptomatic cases seem to be complicated and typically have neurological manifestations. The infection caused by TBEV is known as Tick-borne encephalitis (TBE), which often leads to permanent problems in neural system which may end to death. This Flavivirus is endemic to Northern Eurasia. Global reports have shown that there are around 10,000 clinical cases of TBEV infections, annually. Infected ticks of the Ixodidae (hard ticks) Family transmit the virus to humans by blood feeding.Citation126
Immunization of the endangered societies against TBEV is thought to be the best strategy to control this disease. Additionally, the importance of TBEV vaccine design and development concomitant with a lack of antiviral treatment has shed more light on the importance of vaccination. In contrast, vaccination coverage against this Flavivirus is relatively insignificant. Furthermore, the used vaccines did not have the needed efficacies which led to low immunogenicity. All licensed vaccines against this virus are based on inactivated whole viruses, containing different strains of the European or Far-Eastern TBEV subtypes.Citation127
Designing TBEV vaccines with protective effects against the subtypes seems to be pivotal which can affect the successfulness of a new TBEV vaccine candidate. Further, such vaccine candidates are expected to result in protective humoral and cellular immunity. Therefore, vector-based vaccines such as AdVs can be considered as effective platforms to induce adequate immunity.
Jacobs et al. (1992) have invented a recombinant adenovirus RAd51 vector based vaccine, an E1-deleted Ad virus harboring the NS1 gene of TBEV. Jacobs et al. studied this adeno virus in mouse model. They reported a detectable antibody-mediated immune response against TBEV NS1 protein. Furthermore, results of the aforementioned research showed a strong immunity against TBEV in challenged mice.Citation43 Elicitation of the immune response in such immunization practices occurred via a replication-defective AdV expressing the TBEV NS1 as shown by Jacobs et al.Citation42 Interestingly, Timofeev et al. (1998) have indicated that a defective rAd51 AdV harboring the TBEV NS1 gene induces a promising immune response which can lead to immunity against several arboviral flaviviruses in animal models ().Citation41
Clinical data analysis of AdV vaccines
An AdV-based vaccine has been recently introduced. This vaccine is designed as Convidicea (Ad5-nCoV), which seems to be the first Chinese AdV-based vaccine against SARS-CoV-2 in a clinical trial.Citation128 Assessment of Immunogenicity and safety of above AdV-based vaccine has been performed in >18 years-old healthy adults. The designed phase II clinical trials comprised 508 eligible participants.Citation129 The data obtained from phase III clinical trials involving thousands of participants and 101 COVID-19 patients indicated that the efficacy of this vaccine was between 65.7% (moderate cases) and 90.98% (severe cases). The above-mentioned vaccine has been approved for public vaccination in China. Similar assessments have been performed on Ad26.COV2.S by Johnson & Johnson.Citation130 The phase III clinical trials for this vaccine involved 44,000 participants. The vaccine provided 85% protection (severe cases) and 100% protection (against hospitalization and death).Citation130 Parallel to these, Sputnik V (Gam-COVID-Vac) vaccine have been developed by Gamaleya research Institute Russia. Two phases III clinical trials have been carried out (NCT04530396, NCT04564716) to assess the efficacy of Sputnik V. Interim reports of the NCT04530396 trial, with 21,977 participants, have shown an efficacy of 91.6% against SARS-CoV-2 infection.Citation131 The aforementioned efficacies reported for Convidicea (Ad5-nCoV), Johnson & Johnson Ad26.COV2.S, and Sputnik V (Gam-COVID-Vac) tend to be in line with those obtained for other AdV-based anti-SARS-CoV-2 vaccines, likely Oxford/AstraZeneca (ChAdOX1-nCoV-19) indicated safety records comparable to other AdV vaccines.Citation68 Such findings regarding the detectable efficacy of AdV-based vaccines have been also proved in case of other viral infectious diseases. In this line, an efficacy of 91% has been revealed for an AdV-based anti-Ebola virus (chAd3-EBOZ) in the studied Malian population.Citation132–134 Similar studies have been performed on an AdV-based anti-malaria vaccine in which Rampling et al. (2016) have developed an AdV-based anti-malaria vaccine (ChAd63 ME-TRAP). The efficacy of this vaccine was assessed in a clinical trial (Clinical Trials Registration: NCT01883609). Rampling et al. showed that no detectable adverse effects were observed after vaccination. Additionally, the efficacy has been reported as 75–82.4% in separated groups. Immunogenicity of both vaccination regimens have been confirmed and protective effects of vaccination have been observed in 83.3–87.5% of subjects.Citation135
Conclusions
AdVs have been widely investigated in many applications. Various vaccine design platforms have shed light on the importance of E, prM, and NS1 proteins of flaviviruses to elicit detectable titers of protective antibodies in various hosts. However, precautions should be taken into account to co-select appropriate structural and non-structural proteins to prevent antibody dependent enhancement (ADE). Interestingly, replication-competent adenoviruses (RCA) in vaccine design against flaviviruses should be investigated since such phenomena have led to considerable consequences in case of adenovirus viral vector vaccine against SARS-CoV-2. Recently, it has been shown that Sputnik V COVID-19 vaccine, which is a heterologous recombinant adenovirus approach and uses Ad26 and Ad5 as vectors for the expression of the acute SARS-CoV-2 spike protein is safe and effective, suggesting that these vectors are at the forefront of production of effective vaccines against different viruses. In this review, we provided evidence that AdV vaccines induce strong anti-flaviviruses humoral and cellular immune reactions which may provide long-lasting protection effects. Hence, we conclude that AdVs could be a promising route against flaviviruses. However, our knowledge of AdV vaccines against flaviviruses is limited. Thus, detailed additional studies are suggested. The present review has some limitations. Despite the fact that ADE, a phenomenon in which binding of some viruses such as flaviviruses to suboptimal antibodies enhances their entry into host cells, is an important issue in flaviviruses pathogenicity, our review on the AdV-based vaccines against flaviviruses could not find information about ADE on this issue. Detailed studies are suggested to be performed. Finally, our review is limited to reports written in English.
Authors’ contributions
MS: designed and represented the concept and co-wrote the manuscript. KA, FR, MS, AA and HB: co-wrote the manuscript and supervised the research. Authors checked and approved the manuscript, finally.
Ethical approval
This research had no human participants or animals.
Supplemental Material
Download MS Word (48.8 KB)Acknowledgements
This research covers a part of a PhD program. The kind collaborations by the departments: Molecular Virology, Arboviruses and Viral Hemorrhagic Fevers, at Pasteur Institute of Iran are appreciated. Great Thanks to Moslem Papizadeh and Adel Eta for helping us to improve the manuscript.
Disclosure statement
No potential conflict of interest was reported by the author(s).
Supplementary material
Supplemental data for this article can be accessed on the publisher’s website at https://doi.org/10.1080/21645515.2022.2079323.
Additional information
Funding
References
- Deng S-Q, Yang X, Wei Y, Chen J-T, Wang X-J, Peng H-J. A review on dengue vaccine development. Vaccines. 2020;8(1):63. doi:10.3390/vaccines8010063.
- Lee CS, Bishop ES, Zhang R, Yu X, Farina EM, Yan S, Zhao C, Zeng Z, Shu Y, Wu X, et al. Adenovirus-Mediated gene delivery: potential applications for gene and cell-based therapies in the new era of personalized medicine. Genes Dis. 2017;4(2):43–16. doi:10.1016/j.gendis.2017.04.001.
- Khalil SM, Tonkin DR, Mattocks MD, Snead AT, Johnston RE, White LJ. A tetravalent alphavirus-vector based dengue vaccine provides effective immunity in an early life mouse model. Vaccine. 2014;32(32):4068–74. doi:10.1016/j.vaccine.2014.05.053.
- White LJ, Parsons MM, Whitmore AC, Williams BM, de Silva A, Johnston RE. An immunogenic and protective alphavirus replicon particle-based dengue vaccine overcomes maternal antibody interference in weanling mice. Virol J. 2007;81(19):10329–39. doi:10.1128/JVI.00512-07.
- White LJ, Sariol CA, Mattocks MD, Wahala MPBW, Yingsiwaphat V, Collier ML, Whitley J, Mikkelsen R, Rodriguez IV, Martinez MI, et al. An alphavirus vector-based tetravalent dengue vaccine induces a rapid and protective immune response in macaques that differs qualitatively from immunity induced by live virus infection. Virol J. 2013;87(6):3409–24. doi:10.1128/JVI.02298-12.
- Brandler S, Ruffie C, Najburg V, Frenkiel M-P, Bedouelle H, Desprès P, Tangy F. Pediatric measles vaccine expressing a dengue tetravalent antigen elicits neutralizing antibodies against all four dengue viruses. Vaccine. 2010;28(41):6730–39. doi:10.1016/j.vaccine.2010.07.073.
- Brandler S, Lucas-Hourani M, Moris A, Frenkiel M-P, Combredet C, Février M, Bedouelle H, Schwartz O, Desprès P, Tangy F, et al. Pediatric measles vaccine expressing a dengue antigen induces durable serotype-specific neutralizing antibodies to dengue virus. PLoS Negl Trop Dis. 2007;1(3):e96. doi:10.1371/journal.pntd.0000096.
- Ramsauer K, Schwameis M, Firbas C, Müllner M, Putnak RJ, Thomas SJ, Desprès P, Tauber E, Jilma B, Tangy F, et al. Immunogenicity, safety, and tolerability of a recombinant measles-virus-based chikungunya vaccine: a randomised, double-blind, placebo-controlled, active-comparator, first-in-man trial. Lancet Infect Dis. 2015;15(5):519–27. doi:10.1016/S1473-3099(15)70043-5.
- Raviprakash K, Wang D, Ewing D, Holman DH, Block K, Woraratanadharm J, Chen L, Hayes C, Dong JY, Porter K, et al. A tetravalent dengue vaccine based on a complex adenovirus vector provides significant protection in rhesus monkeys against all four serotypes of dengue virus. Virol J. 2008;82(14):6927–34. doi:10.1128/JVI.02724-07.
- Jaiswal S, Khanna N, Swaminathan S. Replication-Defective adenoviral vaccine vector for the induction of immune responses to dengue virus type 2. Virol J. 2003;77(23):12907–13. doi:10.1128/JVI.77.23.12907-12913.2003.
- Men R, Wyatt L, Tokimatsu I, Arakaki S, Shameem G, Elkins R, Chanock R, Moss B, Lai C-J. Immunization of rhesus monkeys with a recombinant of modified vaccinia virus Ankara expressing a truncated envelope glycoprotein of dengue type 2 virus induced resistance to dengue type 2 virus challenge. Vaccine. 2000;18(27):3113–22. doi:10.1016/S0264-410X(00)00121-3.
- Khanam S, Pilankatta R, Khanna N, Swaminathan S. An adenovirus type 5 (AdV5) vector encoding an envelope domain III-based tetravalent antigen elicits immune responses against all four dengue viruses in the presence of prior AdV5 immunity. Vaccine. 2009;27(43):6011–21. doi:10.1016/j.vaccine.2009.07.073.
- Drexler I, Staib C, Sutter G. Modified vaccinia virus Ankara as antigen delivery system: how can we best use its potential? Curr Opin Biotechnol. 2004;15(6):506–12. doi:10.1016/j.copbio.2004.09.001.
- Zhao B, Prince G, Horswood R, Eckels K, Summers P, Chanock R, Lai CJ. Expression of dengue virus structural proteins and nonstructural protein NS1 by a recombinant vaccinia virus. Virol J. 1987;61(12):4019–22. doi:10.1128/jvi.61.12.4019-4022.1987.
- Khanam S, Rajendra P, Khanna N, Swaminathan S. An adenovirus prime/plasmid boost strategy for induction of equipotent immune responses to two dengue virus serotypes. BMC Biotechnol. 2007;7(1):1–11. doi:10.1186/1472-6750-7-10.
- Holman DH, Wang D, Raviprakash K, Raja NU, Luo M, Zhang J, Porter KR, Dong JY. Two complex, adenovirus-based vaccines that together induce immune responses to all four dengue virus serotypes. Clin Vaccine Immunol. 2007;14(2):182–89. doi:10.1128/CVI.00330-06.
- Chen L, Ewing D, Subramanian H, Block K, Rayner J, Alterson KD, Sedegah M, Hayes C, Porter K, Raviprakash K, et al. A heterologous DNA prime-Venezuelan equine encephalitis virus replicon particle boost dengue vaccine regimen affords complete protection from virus challenge in cynomolgus macaques. Virol J. 2007;81(21):11634–39. doi:10.1128/JVI.00996-07.
- Xu K, Song Y, Dai L, Zhang Y, Lu X, Xie Y, Zhang H, Cheng T, Wang Q, Huang Q, et al. Recombinant chimpanzee adenovirus vaccine AdC7-M/E protects against Zika virus infection and testis damage. Virol J. 2018;92(6): e01722-17. doi:10.1128/JVI.01722-17.
- Cox F, van der Fits L, Abbink P, Larocca RA, van Huizen E, Saeland E, Verhagen J, Peterson R, Tolboom J, Kaufmann B, et al. Adenoviral vector type 26 encoding Zika virus (ZIKV) M-Env antigen induces humoral and cellular immune responses and protects mice and nonhuman primates against ZIKV challenge. PloS One. 2018;13(8):e0202820. doi:10.1371/journal.pone.0202820.
- López-Camacho C, Abbink P, Larocca RA, Dejnirattisai W, Boyd M, Badamchi-Zadeh A, Wallace ZR, Doig J, Velazquez RS, Neto RDL, et al. Rational Zika vaccine design via the modulation of antigen membrane anchors in chimpanzee adenoviral vectors. Nat Commun. 2018;9(1):1–11. doi:10.1038/s41467-018-04859-5.
- Liu X, Qu L, Ye X, Yi C, Zheng X, Hao M, Su W, Yao Z, Chen P, Zhang S, et al. Incorporation of NS1 and prM/M are important to confer effective protection of adenovirus-vectored Zika virus vaccine carrying E protein. Npj Vaccines. 2018;3(1):1–8. doi:10.1038/s41541-018-0072-6.
- Prow NA, Liu L, Nakayama E, Cooper TH, Yan K, Eldi P, Hazlewood JE, Tang B, Le TT, Setoh YX, et al. A vaccinia-based single vector construct multi-pathogen vaccine protects against both Zika and chikungunya viruses. Nat Commun. 2018;9(1):1–12. doi:10.1038/s41467-018-03662-6.
- Betancourt D, De Queiroz NM, Xia T, Ahn J, Barber GN. Cutting edge: innate immune augmenting vesicular stomatitis virus expressing Zika virus proteins confers protective immunity. J Immunol. 2017;198(8):3023–28. doi:10.4049/jimmunol.1602180.
- Nürnberger C, Bodmer BS, Fiedler AH, Gabriel G, Mühlebach MD, Heise MT. A measles virus-based vaccine candidate mediates protection against Zika virus in an allogeneic mouse pregnancy model. Virol J. 2019;93(3): e01485-18. doi:10.1128/JVI.01485-18.
- Guo Q, Chan J-W, Poon V-M, Wu S, Chan C-S, Hou L, Yip CCY, Ren C, Cai J-P, Zhao M, et al. Immunization with a novel human type 5 adenovirus-vectored vaccine expressing the premembrane and envelope proteins of Zika virus provides consistent and sterilizing protection in multiple immunocompetent and immunocompromised animal models. J Infect Dis. 2018;218(3):365–77. doi:10.1093/infdis/jiy187.
- Abbink P, Larocca RA, Visitsunthorn K, Boyd M, Rafael A, Gromowski GD, Kirilova M, Peterson R, Li Z, Nanayakkara O, et al. Durability and correlates of vaccine protection against Zika virus in rhesus monkeys. Sci Transl Med. 2017;9(420). doi:10.1126/scitranslmed.aao4163.
- Abbink P, Larocca RA, Rafael A, Bricault CA, Moseley ET, Boyd M, Kirilova M, Li Z, Ng’-Ang’a D, Nanayakkara O, et al. Protective efficacy of multiple vaccine platforms against Zika virus challenge in rhesus monkeys. Science. 2016;353(6304):1129–32. doi:10.1126/science.aah6157.
- López-Camacho C, De Lorenzo G, Slon-Campos JL, Dowall S, Abbink P, Larocca RA, Kim YC, Poggianella M, Graham V, Findlay-Wilson S, et al. Immunogenicity and efficacy of Zika virus envelope domain III in DNA, protein, and ChAdox1 adenoviral-vectored vaccines. Vaccines. 2020;8(2):307. doi:10.3390/vaccines8020307.
- Schepp-Berglind J, Luo M, Wang D, Wicker JA, Raja NU, Hoel BD, Holman DH, Barrett ADT, Dong JY. Complex adenovirus-mediated expression of West Nile virus C, PreM, E, and NS1 proteins induces both humoral and cellular immune responses. Clin Vaccine Immunol. 2007;14(9):1117–26. doi:10.1128/CVI.00070-07.
- Brandler S, Marianneau P, Loth P, Lacôte S, Combredet C, Frenkiel M-P, Desprès P, Contamin H, Tangy F. Measles vaccine expressing the secreted form of West Nile virus envelope glycoprotein induces protective immunity in squirrel monkeys, a new model of West Nile virus infection. J Infect Dis. 2012;206(2):212–19. doi:10.1093/infdis/jis328.
- Despres P, Combredet C, Frenkiel M-P, Lorin C, Brahic M, Tangy F. Live measles vaccine expressing the secreted form of the West Nile virus envelope glycoprotein protects against West Nile virus encephalitis. J Infect Dis. 2005;191(2):207–14. doi:10.1086/426824.
- Iyer AV, Pahar B, Boudreaux MJ, Wakamatsu N, Roy AF, Chouljenko VN, Baghian A, Apetrei C, Marx PA, Kousoulas KG, et al. Recombinant vesicular stomatitis virus-based west Nile vaccine elicits strong humoral and cellular immune responses and protects mice against lethal challenge with the virulent west Nile virus strain LSU-AR01. Vaccine. 2009;27(6):893–903. doi:10.1016/j.vaccine.2008.11.087.
- Bassi MR, Larsen MA, Kongsgaard M, Rasmussen M, Buus S, Stryhn A, Thomsen AR, Christensen JP. Vaccination with replication deficient adenovectors encoding YF-17D antigens induces long-lasting protection from severe yellow fever virus infection in mice. PLoS Negle Trop Dis. 2016;10(2):e0004464. doi:10.1371/journal.pntd.0004464.
- Appaiahgari MB, Saini M, Rauthan M, Vrati S. Immunization with recombinant adenovirus synthesizing the secretory form of Japanese encephalitis virus envelope protein protects adenovirus-exposed mice against lethal encephalitis. Microbes Infect. 2006;8(1):92–104. doi:10.1016/j.micinf.2005.05.023.
- Konishi E, Kurane I, Mason PW, Shope RE, Kanesa-Thasan N, Smucny JJ, Hoke CH, Ennis FA. Induction of Japanese encephalitis virus-specific cytotoxic T lymphocytes in humans by poxvirus-based JE vaccine candidates. Vaccine. 1998;16(8):842–49. doi:10.1016/S0264-410X(97)00265-X.
- Kanesa-Thasan N, Smucny JJ, Hoke Jr CH, Marks DH, Konishi E, Kurane I, Tang DB, Vaughn DW, Mason PW, Shope RE, et al. Safety and immunogenicity of NYVAC-JEV and ALVAC-JEV attenuated recombinant Japanese encephalitis virus — poxvirus vaccines in vaccinia-nonimmune and vaccinia-immune humans. Vaccine. 2000;19(4–5):483–91. doi:10.1016/S0264-410X(00)00191-2.
- Konishi E, Pincus S, Paoletti E, Shope R, Mason P. A vipox virus-vectored Japanese encephalitis virus vaccines: use as vaccine candidates in combination with purified subunit immunogens. Vaccine. 1994;12(7):633–38. doi:10.1016/0264-410X(94)90269-0.
- Lobigs M, Pavy M, Hall RA, Lobigs P, Cooper P, Komiya T, Toriniwa H, Petrovsky N. An inactivated vero cell-grown Japanese encephalitis vaccine formulated with Advax, a novel inulin-based adjuvant, induces protective neutralizing antibody against homologous and heterologous flaviviruses. J Gen Virol. 2010;91(6):1407. doi:10.1099/vir.0.019190-0.
- Nam JH, Bang HS, Cho HW, Chung YH. Different contribution of co-stimulatory molecules B7.1 and B7.2 to the immune response to recombinant modified vaccinia virus ankara vaccine expressing prM/E proteins of Japanese encephalitis virus and two hepatitis B virus vaccines. Acta Virol. 2007;51:125–30.
- Konishi E, Kurane I, Mason PW, Shope RE, Ennis FA. Poxvirus-Based Japanese encephalitis vaccine candidates induce JE virus-specific CD8+ cytotoxic T lymphocytes in mice. Virology. 1997;227(2):353–60. doi:10.1006/viro.1996.8331.
- Elbert LB, Karganova GG, Stephenson JR, Deeva A, Ozherelkov SV, Timofeev AV, Pronin AV. Immunological basis for protection in a murine model of tick-borne encephalitis by a recombinant adenovirus carrying the gene encoding the NS1 non-structural protein. J Gen Virol. 1998;79(4):689–95. doi:10.1099/0022-1317-79-4-689.
- Jacobs SC, Stephenson JR, Wilkinson GW. Protection elicited by a replication-defective adenovirus vector expressing the tick-borne encephalitis virus non-structural glycoprotein NS1. J Gen Virol. 1994;75(9):2399–402. doi:10.1099/0022-1317-75-9-2399.
- Jacobs S, Stephenson J, Wilkinson G. High-Level expression of the tick-borne encephalitis virus NS1 protein by using an adenovirus-based vector: protection elicited in a murine model. Virol J. 1992;66(4):2086–95. doi:10.1128/jvi.66.4.2086-2095.1992.
- Ura T, Okuda K, Shimada M. Developments in viral vector-based vaccines. Vaccines. 2014;2(3):624–41. doi:10.3390/vaccines2030624.
- Chang J. Adenovirus vectors: excellent tools for vaccine development. Immune Netw. 2021;21(1). doi:10.4110/in.2021.21.e6.
- Harui A, Suzuki S, Kochanek S, Mitani K. Frequency and stability of chromosomal integration of adenovirus vectors. Virol J. 1999;73(7):6141–46. doi:10.1128/JVI.73.7.6141-6146.1999.
- Khanam S, Khanna N, Swaminathan S. Induction of neutralizing antibodies and T cell responses by dengue virus type 2 envelope domain III encoded by plasmid and adenoviral vectors. Vaccine. 2006;24:6513–25. doi:10.1016/j.vaccine.2006.06.031.
- Bullard BL, Corder BN, Gorman MJ, Diamond MS, Weaver EA. Efficacy of a T cell-biased adenovirus vector as a Zika virus vaccine. Sci Rep. 2018;8(1):1–10. doi:10.1038/s41598-018-35755-z.
- Bullard BL, Corder BN, Gordon DN, Pierson TC, Weaver EA. Characterization of a species E adenovirus vector as a Zika virus vaccine. Sci Rep. 2020;10(1):1–10. doi:10.1038/s41598-020-60238-5.
- Larocca RA, Mendes EA, Abbink P, Peterson RL, Martinot AJ, Iampietro MJ, Kang ZH, Aid M, Kirilova M, Jacob-Dolan C, et al. Adenovirus vector-based vaccines confer maternal-fetal protection against Zika virus challenge in pregnant IFN-αβR−/− mice. Cell Host Microbe. 2019;26(5):591–600. e4. doi:10.1016/j.chom.2019.10.001.
- Kim E, Erdos G, Huang S, Kenniston T, Falo LD Jr, Gambotto A. Preventative vaccines for Zika virus outbreak: preliminary evaluation. EBioMedicine. 2016;13:315–20. doi:10.1016/j.ebiom.2016.09.028.
- Hassan AO, Dmitriev IP, Kashentseva EA, Zhao H, Brough DE, Fremont DH, Curiel DT, Diamond MS. A gorilla adenovirus-based vaccine against Zika virus induces durable immunity and confers protection in pregnancy. Cell Rep. 2019;28(10):2634–46. e4. doi:10.1016/j.celrep.2019.08.005.
- Steffen T, Hassert M, Hoft SG, Stone ET, Zhang J, Geerling E, Grimberg BT, Roberts MS, Pinto AK, Brien JD, et al. Immunogenicity and efficacy of a recombinant human adenovirus type 5 vaccine against Zika virus. Vaccines. 2020;8(2):170. doi:10.3390/vaccines8020170.
- Vrati S. Recombinant vaccine against japanese encephalitis virus [jev] infection and a method thereof. Google Patents. 2010.
- Timofeev A, Ozherelkov S, Pronin A, Deeva A, Elbert L, Stefenson J. [A recombinant adenovirus expressing the NS1 nonstructural protein of tick-borne encephalitis virus: some characteristics of the immunologic basis of antiviral action]. Vopr Virusol. 1997;42:219–22.
- Pugachev KV, Mason PW, Shope RE, Frey TK. Double-Subgenomic Sindbis virus recombinants expressing immunogenic proteins of Japanese encephalitis virus induce significant protection in mice against lethal JEV infection. Virology. 1995;212(2):587–94. doi:10.1006/viro.1995.1516.
- Lauretti F, Chattopadhyay A, de Oliveira França RF, Castro-Jorge L, Rose J, Fonseca B. Recombinant vesicular stomatitis virus-based dengue-2 vaccine candidate induces humoral response and protects mice against lethal infection. Hum Vaccines Immunother. 2016;12:2327–33. doi:10.1080/21645515.2016.1183857.
- Quinan BR, Flesch IE, Pinho TM, Coelho FM, Tscharke DC, da Fonseca FG. An intact signal peptide on dengue virus E protein enhances immunogenicity for CD8+ T cells and antibody when expressed from modified vaccinia Ankara. Vaccine. 2014;32(25):2972–79. doi:10.1016/j.vaccine.2014.03.093.
- Yasuda A, Kimura-Kuroda J, Ogimoto M, Miyamoto M, Sata T, Sato T, Takamura C, Kurata T, Kojima A, Yasui K, et al. Induction of protective immunity in animals vaccinated with recombinant vaccinia viruses that express PreM and E glycoproteins of Japanese encephalitis virus. Virol J. 1990;64(6):2788–95. doi:10.1128/jvi.64.6.2788-2795.1990.
- Nam J-H, Wyatt LS, Chae S-L, Cho H-W, Park Y-K, Moss B. Protection against lethal Japanese encephalitis virus infection of mice by immunization with the highly attenuated MVA strain of vaccinia virus expressing JEV prM and E genes. Vaccine. 1999;17(3):261–68. doi:10.1016/S0264-410X(98)00156-X.
- Nam J-H, Chae S-L, Cho H-W. Immunogenicity of a recombinant MVA and a DNA vaccine for Japanese encephalitis virus in swine. Microbiol Immunol. 2002;46(1):23–28. doi:10.1111/j.1348-0421.2002.tb02672.x.
- Wang F, Feng X, Zheng Q, Hou H, Cao R, Zhou B, Liu Q, Liu X, Pang R, Zhao J, et al. Multiple linear epitopes (B-cell, CTL and Th) of JEV expressed in recombinant MVA as multiple epitope vaccine induces a protective immune response. Virol J. 2012;9(1):1–10. doi:10.1186/1743-422X-9-204.
- Pérez P, Marín MQ, Lázaro-Frías A, de Oya NJ, Blázquez A-B, Escribano-Romero E, Sorzano CÓ, Ortego J, Saiz J-C, Esteban M, et al. A vaccine based on a modified vaccinia virus ankara vector expressing Zika virus structural proteins controls Zika virus replication in mice. Sci Rep. 2018;8(1):1–15. doi:10.1038/s41598-018-35724-6.
- Brault AC, Domi A, McDonald EM, Talmi-Frank D, McCurley N, Basu R, Robinson HL, Hellerstein M, Duggal NK, Bowen RA, et al. A Zika vaccine targeting NS1 protein protects immunocompetent adult mice in a lethal challenge model. Sci Rep. 2017;7(1):1–11. doi:10.1038/s41598-017-15039-8.
- Olive M, Eisenlohr L, Flomenberg N, Hsu S, Flomenberg P. The adenovirus capsid protein hexon contains a highly conserved human CD4 + T-cell epitope. Hum Gene Ther. 2002;13(10):1167–78. doi:10.1089/104303402320138952.
- Sharma PK, Dmitriev IP, Kashentseva EA, Raes G, Li L, Kim SW, Lu Z-H, Arbeit JM, Fleming TP, Kaliberov SA, et al. Development of an adenovirus vector vaccine platform for targeting dendritic cells. Cancer Gene Ther. 2018;25(1–2):27–38. doi:10.1038/s41417-017-0002-1.
- Klok FA, Pai M, Huisman MV, Makris M. Vaccine-Induced immune thrombotic thrombocytopenia. Lancet Haematol. 2021;9(1):73–80.
- Mendonça SA, Lorincz R, Boucher P, Curiel DT. Adenoviral vector vaccine platforms in the SARS-CoV-2 pandemic. Npj Vaccines. 2021;6(1):1–14. doi:10.1038/s41541-021-00356-x.
- Russell W. Update on adenovirus and its vectors. J Gen Virol. 2000;81(11):2573–604. doi:10.1099/0022-1317-81-11-2573.
- Parks RJ. Adenovirus protein IX: a new look at an old protein. Mol Ther. 2005;11(1):19–25. doi:10.1016/j.ymthe.2004.09.018.
- Goradel NH, Mohajel N, Malekshahi ZV, Jahangiri S, Najafi M, Farhood B, Mortezaee K, Negahdari B, Arashkia A. Oncolytic adenovirus: a tool for cancer therapy in combination with other therapeutic approaches. J Cell Physiol. 2019;234(6):8636–46. doi:10.1002/jcp.27850.
- Hitt MM, Graham FL. Adenovirus vectors for human gene therapy. Adv Virus Res. 2000;55:479–505. doi:10.1016/s0065-3527(00)55014-3.
- Polo JM, Dubensky TW Jr. Virus-Based vectors for human vaccine applications. Drug Discov. 2002;7:719–27.
- Danthinne X, Imperiale M. Production of first generation adenovirus vectors: a review. Gene Ther. 2000;7(20):1707–14. doi:10.1038/sj.gt.3301301.
- Fallaux FJ, Bout A, van der Velde I, van den Wollenberg DJ, Hehir KM, Keegan J, Auger C, Cramer SJ, van Ormondt H, van der Eb AJ, et al. New helper cells and matched early region 1-deleted adenovirus vectors prevent generation of replication-competent adenoviruses. Hum Gene Ther. 1998;9(13):1909–17. doi:10.1089/hum.1998.9.13-1909.
- Bett AJ, Haddara W, Prevec L, Graham FL. An efficient and flexible system for construction of adenovirus vectors with insertions or deletions in early regions 1 and 3. Proc Natl Acad Sci. 1994;91(19):8802–06. doi:10.1073/pnas.91.19.8802.
- Kovesdi I, Hedley SJ. Adenoviral producer cells. Viruses. 2010;2(8):1681–703. doi:10.3390/v2081681.
- Dussupt V, Sankhala RS, Gromowski GD, Donofrio G, Rafael A, Larocca RA, Zaky W, Mendez-Rivera L, Choe M, Davidson E, et al. Potent Zika and dengue cross-neutralizing antibodies induced by Zika vaccination in a dengue-experienced donor. Nat Med. 2020;26(2):228–35. doi:10.1038/s41591-019-0746-2.
- Vrba SM, Kirk NM, Brisse ME, Liang Y, Ly H. Development and applications of viral vectored vaccines to combat zoonotic and emerging public health threats. Vaccines. 2020;8(4):680. doi:10.3390/vaccines8040680.
- Raja NU, Holman DH, Wang D, Raviprakash K, Juompan LY, Deitz SB, et al. Induction of bivalent immune responses by expression of dengue virus type 1 and type 2 antigens from a single complex adenoviral vector. Am J Trop Med. 2007;76:743–51. doi:10.4269/ajtmh.2007.76.743.
- Molinier-Frenkel V, Lengagne R, Gaden F, Hong S-S, Choppin J, Gahery-Ségard H, Boulanger P, Guillet J-G. Adenovirus hexon protein is a potent adjuvant for activation of a cellular immune response. Virol J. 2002;76(1):127–35. doi:10.1128/JVI.76.1.127-135.2002.
- Carina Silva A, Peixoto C, Lucas T, Kuppers C, Cruz P E, Alves P M, Kochanek S. Adenovirus vector production and purification. Curr Gene Ther. 2010;10(6):437–55. doi:10.2174/156652310793797694.
- Havenga M, Holterman L, Melis I, Smits S, Kaspers J, Heemskerk E, Vlugt RVD, Koldijk M, Schouten GJ, Hateboer G, et al. Serum‐free transient protein production system based on adenoviral vector and PER. C6 technology: high yield and preserved bioactivity. Biotechnol Bioeng. 2008;100(2):273–83. doi:10.1002/bit.21757.
- Lusky M, Christ M, Rittner K, Dieterle A, Dreyer D, Mourot B, Schultz H, Stoeckel F, Pavirani A, Mehtali M, et al. In vitro and in vivo biology of recombinant adenovirus vectors with E1, E1/E2A, or E1/E4 deleted. Virol J. 1998;72(3):2022–32. doi:10.1128/JVI.72.3.2022-2032.1998.
- Fang B, Wang H, Gordon G, Bellinger D, Read M, Brinkhous K, Woo SL, Eisensmith RC. Lack of persistence of E1- recombinant adenoviral vectors containing a temperature-sensitive E2A mutation in immunocompetent mice and hemophilia B dogs. Gene Ther. 1996;3:217–22.
- Yang Y, Li Q, Ertl H, Wilson JM. Cellular and humoral immune responses to viral antigens create barriers to lung-directed gene therapy with recombinant adenoviruses. Virol J. 1995;69(4):2004–15. doi:10.1128/jvi.69.4.2004-2015.1995.
- Brunetti-Pierri N, Ng T, Iannitti D, Cioffi W, Stapleton G, Law M, Breinholt J, Palmer D, Grove N, Rice K, et al. Transgene expression up to 7 years in nonhuman primates following hepatic transduction with helper-dependent adenoviral vectors. Hum Gene Ther. 2013;24(8):761–65. doi:10.1089/hum.2013.071.
- He Q, Mao Q, Zhang J, Bian L, Gao F, Wang J, et al. COVID-19 vaccines: current understanding on immunogenicity, safety, and further considerations. Front Immunol. 2021;12:669339.
- Ricobaraza A, Gonzalez-Aparicio M, Mora-Jimenez L, Lumbreras S, Hernandez-Alcoceba R. High-Capacity adenoviral vectors: expanding the scope of gene therapy. Int J Mol Sci. 2020;21(10):3643. doi:10.3390/ijms21103643.
- Suzuki M, Cerullo V, Bertin TK, Cela R, Clarke C, Guenther M, Brunetti-Pierri N, Lee B. MyD88-Dependent silencing of transgene expression during the innate and adaptive immune response to helper-dependent adenovirus. Hum Gene Ther. 2010;21(3):325–36. doi:10.1089/hum.2009.155.
- Hartigan-O’-Connor D, Amalfitano A, Chamberlain JS. Improved production of gutted adenovirus in cells expressing adenovirus preterminal protein and DNA polymerase. Virol J. 1999;73(9):7835–41. doi:10.1128/JVI.73.9.7835-7841.1999.
- Palmer D, Ng P. Improved system for helper-dependent adenoviral vector production. Mol Ther. 2003;8(5):846–52. doi:10.1016/j.ymthe.2003.08.014.
- Hehir KM, Armentano D, Cardoza LM, Choquette TL, Berthelette PB, White GA, Couture LA, Everton MB, Keegan J, Martin JM, et al. Molecular characterization of replication-competent variants of adenovirus vectors and genome modifications to prevent their occurrence. Virol J. 1996;70(12):8459–67. doi:10.1128/jvi.70.12.8459-8467.1996.
- Ramezanpour B, Haan I, Osterhaus A, Claassen E. Vector-Based genetically modified vaccines: exploiting Jenner’s legacy. Vaccine. 2016;34(50):6436–48. doi:10.1016/j.vaccine.2016.06.059.
- Boyer S, Calvez E, Chouin-Carneiro T, Diallo D, Failloux A-B. An overview of mosquito vectors of Zika virus. Microbes Infect. 2018;20(11–12):646–60. doi:10.1016/j.micinf.2018.01.006.
- Mumtaz N, van Kampen JJ, Reusken CB, Boucher CA, Koopmans MP. Zika virus: where is the treatment? Curr Treat Options Infect Dis. 2016;8:208–11. doi:10.1007/s40506-016-0083-7.
- Zhou K, Li C, Shi W, Hu X, Nandakumar KS, Jiang S, Zhang N. Current progress in the development of Zika virus vaccines. Vaccines. 2021;9(9):1004. doi:10.3390/vaccines9091004.
- Poland GA, Ovsyannikova IG, Kennedy RB. Zika vaccine development: current status. Mayo Clin Proc. 2019;94(12):2572–86. doi:10.1016/j.mayocp.2019.05.016.
- Estévez-Herrera J, Pérez-Yanes S, Cabrera-Rodríguez R, Márquez-Arce D, Trujillo-González R, Machado J-D, Madrid R, Valenzuela-Fernández A. Zika virus pathogenesis: a battle for immune evasion. Vaccines. 2021;9(3):294. doi:10.3390/vaccines9030294.
- Wang R, Liao X, Fan D, Wang L, Song J, Feng K, Li M, Wang P, Chen H, An J, et al. Maternal immunization with a DNA vaccine candidate elicits specific passive protection against post-natal Zika virus infection in immunocompetent BALB/c mice. Vaccine. 2018;36(24):3522–32. doi:10.1016/j.vaccine.2018.04.051.
- Baldwin WR, Livengood JA, Giebler HA, Stovall JL, Boroughs KL, Sonnberg S, Bohning KJ, Dietrich EA, Ong YT, Danh HK, et al. Purified inactivated Zika vaccine candidates afford protection against lethal challenge in mice. Sci Rep. 2018;8(1):1–13. doi:10.1038/s41598-018-34735-7.
- Lecouturier V, Pavot V, Berry C, Donadieu A, de Montfort A, Boudet F, Rokbi B, Jackson N, Heinrichs J. An optimized purified inactivated Zika vaccine provides sustained immunogenicity and protection in cynomolgus macaques. Npj Vaccines. 2020;5(1):1–10. doi:10.1038/s41541-020-0167-8.
- Shan C, Muruato AE, Nunes BT, Luo H, Xie X, Medeiros DB, Wakamiya M, Tesh RB, Barrett AD, Wang T, et al. A live-attenuated Zika virus vaccine candidate induces sterilizing immunity in mouse models. Nat Med. 2017;23(6):763–67. doi:10.1038/nm.4322.
- Richner JM, Himansu S, Dowd KA, Butler SL, Salazar V, Fox JM, Julander JG, Tang WW, Shresta S, Pierson TC, et al. Modified mRNA vaccines protect against Zika virus infection. Cell. 2017;168(6):1114–25. e10. doi:10.1016/j.cell.2017.02.017.
- Pardi N, Hogan MJ, Pelc RS, Muramatsu H, Andersen H, DeMaso CR, Dowd KA, Sutherland LL, Scearce RM, Parks R, et al. Zika virus protection by a single low-dose nucleoside-modified mRNA vaccination. Nature. 2017;543(7644):248–51. doi:10.1038/nature21428.
- Abbink P, Stephenson KE, Barouch DH. Zika virus vaccines. Nat Rev Microbiol. 2018;16(10):594–600. doi:10.1038/s41579-018-0039-7.
- Dicks MD, Spencer AJ, Edwards NJ, Wadell G, Bojang K, Gilbert SC, Hill AVS, Cottingham MG. A novel chimpanzee adenovirus vector with low human seroprevalence: improved systems for vector derivation and comparative immunogenicity. PloS One. 2012;7(7):e40385. doi:10.1371/journal.pone.0040385.
- Coughlan L. Factors which contribute to the immunogenicity of non-replicating adenoviral vectored vaccines. Front Immunol. 2020;11:909. doi:10.3389/fimmu.2020.00909.
- Imler J-L. Adenovirus vectors as recombinant viral vaccines. Vaccine. 1995;13(13):1143–51. doi:10.1016/0264-410X(95)00032-V.
- Shrivastava A, Tripathi NK, Dash PK, Parida M. Working towards dengue as a vaccine-preventable disease: challenges and opportunities. Expert Opin Biol Ther. 2017;17(10):1193–99. doi:10.1080/14712598.2017.1356284.
- Zahid K, Shakoor S, Sajid HA, Afzal S, Ali L, Amin I, Shahid M, Idrees M. Advancements in developing an effective and preventive dengue vaccine. Future Virol. 2020;15(2):127–38. doi:10.2217/fvl-2019-0080.
- Edelman R, Wasserman SS, Bodison SA, Putnak RJ, Eckels KH, Tang D, et al. Phase I trial of 16 formulations of a tetravalent live-attenuated dengue vaccine. Am J Trop Med Hyg. 2003;69:48–60. doi:10.4269/ajtmh.2003.69.48.
- Fall G, Di Paola N, Faye M, Dia M, Freire C, Loucoubar C, Zanotto PMDA, Faye O, Sall AA, et al. Biological and phylogenetic characteristics of West African lineages of West Nile virus. PLoS Negle Trop Dis. 2017;11(11):e0006078. doi:10.1371/journal.pntd.0006078.
- Bakhshi H, Failloux A-B, Zakeri S, Raz A, Djadid ND. Mosquito-Borne viral diseases and potential transmission blocking vaccine candidates. Infect Genet Evol. 2018;63:195–203. doi:10.1016/j.meegid.2018.05.023.
- Colpitts TM, Conway MJ, Montgomery RR, Fikrig E. West Nile Virus: biology, transmission, and human infection. Clin Microbiol Rev. 2012;25(4):635–48. doi:10.1128/CMR.00045-12.
- Leonova GN, Pavlenko EV. Characterization of neutralizing antibodies to Far Eastern of tick-borne encephalitis virus subtype and the antibody avidity for four tick-borne encephalitis vaccines in human. Vaccine. 2009;27:2899–904. doi:10.1016/j.vaccine.2009.02.069.
- Petersen LR, Roehrig JT, Sejvar JJ. West Nile virus in the Americas. New and Evolving Infections of the 21st Century. 2007;3–56.
- Dauphin G, Zientara S. West Nile virus: recent trends in diagnosis and vaccine development. Vaccine. 2007;25(30):5563–76. doi:10.1016/j.vaccine.2006.12.005.
- Guy B, Guirakhoo F, Barban V, Higgs S, Monath TP, Lang J. Preclinical and clinical development of YFV 17D-based chimeric vaccines against dengue, West Nile and Japanese encephalitis viruses. Vaccine. 2010;28(3):632–49. doi:10.1016/j.vaccine.2009.09.098.
- Mokaya J, Kimathi D, Lambe T, Warimwe GM. What constitutes protective immunity Following yellow fever vaccination? Vaccines. 2021;9:671. doi:10.3390/vaccines9060671.
- Thomas RE, Lorenzetti DL, Spragins W, Jackson D, Williamson T. Active and passive surveillance of yellow fever vaccine 17D or 17DD-associated serious adverse events: systematic review. Vaccine. 2011;29(28):4544–55. doi:10.1016/j.vaccine.2011.04.055.
- Erlanger TE, Weiss S, Keiser J, Utzinger J, Wiedenmayer K. Past, present, and future of Japanese encephalitis. Emerg Infect Dis. 2009;15(1):1. doi:10.3201/eid1501.080311.
- Araujo SC, Pereira LR, Alves RP, Andreata-Santos R, Kanno AI, Ferreira LCS, Gonçalves VM. Anti-Flavivirus vaccines: review of the present situation and perspectives of subunit vaccines produced in Escherichia coli. Vaccines. 2020;8(3):492. doi:10.3390/vaccines8030492.
- Hoke CH, Nisalak A, Sangawhipa N, Jatanasen S, Laorakapongse T, Innis BL, Kotchasenee S-O, Gingrich JB, Latendresse J, Fukai K, et al. Protection against Japanese encephalitis by inactivated vaccines. N Engl J Med. 1988;319(10):608–14. doi:10.1056/NEJM198809083191004.
- Kim DS, Houillon G, Jang GC, Cha S-H, Choi S-H, Lee J, Kim HM, Kim JH, Kang JH, Kim J-H, et al. A randomized study of the immunogenicity and safety of Japanese encephalitis chimeric virus vaccine (JE-CV) in comparison with SA14-14-2 vaccine in children in the Republic of Korea. Hum Vaccines Immunother. 2014;10(9):2656–63. doi:10.4161/hv.29743.
- Taba P, Schmutzhard E, Forsberg P, Lutsar I, Ljøstad U, Mygland Å, Levchenko I, Strle F, Steiner I. EAN consensus review on prevention, diagnosis and management of tick‐borne encephalitis. Eur J Neurol. 2017;24(10):1214–e61. doi:10.1111/ene.13356.
- Kubinski M, Beicht J, Gerlach T, Volz A, Sutter G, Rimmelzwaan GF. Tick-Borne encephalitis virus: a quest for better vaccines against a virus on the rise. Vaccines. 2020;8(3):451. doi:10.3390/vaccines8030451.
- Zhu F-C, Li Y-H, Guan X-H, Hou L-H, Wang W-J, Li J-X, Wu S-P, Wang B-S, Wang Z, Wang L, et al. Safety, tolerability, and immunogenicity of a recombinant adenovirus type-5 vectored COVID-19 vaccine: a dose-escalation, open-label, non-randomised, first-in-human trial. Lancet. 2020;395(10240):1845–54. doi:10.1016/S0140-6736(20)31208-3.
- Zhu F-C, Guan X-H, Li Y-H, Huang J-Y, Jiang T, Hou L-H, Li J-X, Yang B-F, Wang L, Wang W-J, et al. Immunogenicity and safety of a recombinant adenovirus type-5-vectored COVID-19 vaccine in healthy adults aged 18 years or older: a randomised, double-blind, placebo-controlled, phase 2 trial. Lancet. 2020;396(10249):479–88. doi:10.1016/S0140-6736(20)31605-6.
- Sadoff J, Gray G, Vandebosch A, Cárdenas V, Shukarev G, Grinsztejn B, Goepfert PA, Truyers C, Fennema H, Spiessens B, et al. Safety and efficacy of single-dose Ad26. COV2. S vaccine against Covid-19. N Engl J Med. 2021;384(23):2187–201. doi:10.1056/NEJMoa2101544.
- Logunov DY, Dolzhikova IV, Shcheblyakov DV, Tukhvatulin AI, Zubkova OV, Dzharullaeva AS, Kovyrshina AV, Lubenets NL, Grousova DM, Erokhova AS, et al. Safety and efficacy of an rAd26 and rAd5 vector-based heterologous prime-boost COVID-19 vaccine: an interim analysis of a randomised controlled phase 3 trial in Russia. Lancet. 2021;397(10275):671–81. doi:10.1016/S0140-6736(21)00234-8.
- Ewer K, Rampling T, Venkatraman N, Bowyer G, Wright D, Lambe T, Imoukhuede EB, Payne R, Fehling SK, Strecker T, et al. A monovalent chimpanzee adenovirus Ebola vaccine boosted with MVA. N Engl J Med. 2016;374(17):1635–46. doi:10.1056/NEJMoa1411627.
- Tapia MD, Sow SO, Lyke KE, Haidara FC, Diallo F, Doumbia M, Traore A, Coulibaly F, Kodio M, Onwuchekwa U, et al. Use of ChAd3-EBO-Z Ebola virus vaccine in Malian and US adults, and boosting of Malian adults with MVA-BN-Filo: a phase 1, single-blind, randomised trial, a phase 1b, open-label and double-blind, dose-escalation trial, and a nested, randomised, double-blind, placebo-controlled trial. Lancet Infect Dis. 2016;16(1):31–42. doi:10.1016/S1473-3099(15)00362-X.
- Zhu F-C, Wurie AH, Hou L-H, Liang Q, Li Y-H, Russell JB, Wu S-P, Li J-X, Hu Y-M, Guo Q, et al. Safety and immunogenicity of a recombinant adenovirus type-5 vector-based Ebola vaccine in healthy adults in Sierra Leone: a single-centre, randomised, double-blind, placebo-controlled, phase 2 trial. Lancet. 2017;389(10069):621–28. doi:10.1016/S0140-6736(16)32617-4.
- Rampling T, Ewer KJ, Bowyer G, Bliss CM, Edwards NJ, Wright D, Payne RO, Venkatraman N, de Barra E, Snudden CM, et al. Safety and high level efficacy of the combination malaria vaccine Regimen of RTS,S/AS01 B with chimpanzee adenovirus 63 and modified vaccinia Ankara vectored vaccines expressing ME-TRAP. J Infect Dis. 2016;214(5):772–81. doi:10.1093/infdis/jiw244.