ABSTRACT
This article describes the results of a preclinical safety and immunogenicity study of QazCovid-in®, the first COVID-19 vaccine developed in Kazakhstan, on BALB/c mice, rats, ferrets, Syrian hamsters and rhesus macaques (Macaca mulatta). The study’s safety data suggests that this immunobiological preparation can be technically considered a Class 5 nontoxic vaccine. The series of injections that were made did not produce any adverse effect or any change in the general condition of the model animals’ health, while macroscopy and histology studies identified no changes in the internal organs of the BALB/c mice and rats. This study has demonstrated that a double immunization enhances the growth of antibody titers as assessed by the microneutralization assay (MNA) and the enzyme-linked immunosorbent assay (ELISA) in a pre-clinical immunogenicity test on animal models. The best GMT results were assessed in MNA and ELISA 7 days after re-vaccination; however, we noted that GMT antibody results in ELISA were lower than in MNA. A comparative GMT assessment after the first immunization and the re-immunization identified significant differences between model animal groups and a growth of GMT antibodies in all of them; also, differences between the gender groups were statistically significant. Moreover, the most marked MNA immune response to the QazCovid-in® vaccine was seen in the Syrian hamsters, while their SARS-CoV-2-specific antibody activity as assessed with ELISA was the lowest.
Introduction
The spread of COVID-19 has become uncontrollable with the overall present-day number of cases at 477,382,359 and deaths at around 6,109,743 globally.Citation1,Citation2 The Republic of Kazakhstan has reported 1,304,983 cases of infection and 13,657 deaths.Citation3 Currently, a number of candidate vaccines have been developed and tested in vitro and in vivo; these are subdivided into six types: the viral vector vaccines,Citation4,Citation5 lipid nanoparticle-based modified mRNA vaccines,Citation6–9 DNA vaccines,Citation10 subunit vaccines,Citation11–13 nanoparticle-based vaccines,Citation14 inactivated whole virus-based vaccinesCitation15,Citation16 and live attenuated virus-based vaccines.Citation17,Citation18 All the vaccines for SARS-CoV, MERS-CoV and SARS-CoV-2 that have been developed are going through pre-clinical or clinical studies;Citation19–27 however, these vaccines are not sufficient to meet the world population’s immunization demand. Given the national significance of having an effective means of specific COVID-19 prophylaxis, Kazakh scientists have developed the country’s first SARS-CoV-2 candidate vaccine. This inactivated vaccine is designed for specific prophylaxis of COVID-19 and will be used in public health efforts to prevent and eliminate infection in Kazakhstan.
The goal of this study is to assess the level of safety, i.e. toxicity, and immunogenicity of the QazCovid-in® inactivated COVID-19 vaccine.
Materials and methods
Ethical approval
All experiments involving animals were conducted in accordance with national and international laboratory animal use regulations and guidelines. The study protocol was approved by the RIBSP’s Animal Experiment Ethics Committee (Permit # 0909/25).
Vaccine
QazCovid-in® is an inactivated vaccine for COVID-19. The object of this study is a cultivated virus vaccine for preventing the COVID-19 disease in the form of a suspension designed for IM injection. The vaccine uses an inactivated SARS-CoV-2/KZ_Almaty/04.2020 strain, that was used in making the vaccine, is not circulating at present. The strain was isolated in March 2020 in Kazakhstan from local clinical samples and was circulating back then. This paper describes pre-clinical safety and immunogenicity studies of QazCovid-in®, which has gone through clinical trials (phase 1, phase 2 and phase 3) and is being used for preventive vaccination in Kazakhstan. One of the vaccine’s unique features is its production technology which allows updating the strain used in the vaccine’s composition. The preparation’s active substance is produced using the Vero cell culture. All vaccination experiments in the study used 5-mg doses of inactivated QazCovid-in® preparation (concentrated viral S-protein); however, these were introduced in different volumes.
We subjected confluent monolayers of Vero cells in 10-tier cell factories (Nalge Nunc International, United States) to multiple inoculations with the SARS-CoV-2/KZ_Almaty/04.2020 vaccine strain at a temperature of 37°C during 48 hours. We treated the virus harvest with freshly prepared formaldehyde (Sigma Aldrich) for 24 hours to inactivate SARS-CoV-2/KZ_Almaty/04.2020 infectivity, followed by the addition of 150 mM sodium bisulfite. We purified and concentrated the inactivated virus using a combination of the tangential flow ultra-filtration and exclusive chromatography methods. We sterilized the purified and concentrated inactivated viral suspension by filtering it through membrane filters with a pore diameter of 0.22 microns. The purified virus, taken at a certain concentration of a specific protein in a phosphate-salt buffer, was mixed with an Al(OH)3 adjuvant (Alhydrogel®, InvivoGen, France) and used as the candidate vaccine.
Toxicity testing
A toxicity study to test low and high-dose vaccine formulations on animal models was conducted by the National Center for Expertise of Medicines and Medical Devices, Committee for Medical and Pharmaceutical Control of the Ministry of Health of the Republic of Kazakhstan (NCEMMD MH RK).
This study tested acute and sub-acute toxicity of QazCovid-in® comparing it to that of PBS. The study used rats and mice randomly assigned to the experimental and the control groups.
Acute toxicity
The QazCovid-in® acute toxicity study involved introducing the maximum allowable vaccine volume IM to BALB/c mice (weighing 20–22 g, 6–8 weeks old) and rats (weighing 190–210 g, 10–16 weeks old).
The dose used in BALB/c mice was equivalent to a single human dose (0.5 ml). For rats we used a 5-ml dose, an equivalent to 10 human doses, which we introduced in 5 injections at 1-hour intervals. The control group animals received a commercial PBS solution in the same volume and using the same administration route as the experimental group.
We performed daily clinical observation of the general state of the animals’ health for 14 days following vaccination. The observation consisted of monitoring the general state of health of the animals, including consumption of feed and water; the general condition of the skin, fur, and mucosa; the behavioral reactions and the motor activity. To analyze changes of the laboratory animals’ body weight over time, we recorded it before vaccination, in 24 hours, at day 7 and day 14 following vaccination. At the experiment’s completion, we decapitated the animals (on the 15th day of the study) in accordance with applicable ethical requirements and performed a visual examination of their internal organs.
Sub-acute toxicity
The sub-acute toxicity study used sixty rats of both sexes weighing 200 ± 10 g that were randomized into 6 groups (n = 10, five♀ and five ♂): a control group (CG) and five experimental groups (EG), ().
The sub-acute toxicity study of QazCovid-in® used commercial PBS as a comparator to the vaccine, both administered IM, with PBS given to the control group (CG#1) at 5.0 ml SID for 7 days. Experimental group 1 received the vaccine in the volume of 0.1 SID for 7 days; experimental group 2 was given 0.2 ml SID for 7 days; experimental group 3 received 0.1 ml five times at 1-hour intervals making a total of 0.5 ml (1 human dose). Experimental group 4 received five 0.75-ml doses of the vaccine at 1-hour intervals making a total of 3.75 ml (7.5 human doses) and experimental group 5 received five 1.0-ml doses at 1-hour intervals which makes a total of 5 ml or 10 human doses.
We monitored the animals for 7 days after vaccinating them. We observed the animals’ general condition: mortality; behavioral reactions; appearance changes; injuries and signs of disease once a day for the duration of the experiment. We checked the animals’ body weight after 24 hours, 5 and 7 days from the start of the experiment. At day 8, we conducted biochemistry and hematology studies of blood; histopathology and morphology examination of the organs (heart, lungs, liver, spleen and kidneys) and calculated the organs’ weight coefficients.
Postmortem examination of internal organs and calculation of their weight coefficients
All rats in each experimental group were sacrificed through exposure to carbon dioxide. We performed a postmortem examination immediately after the animals were euthanized. We carried out a macroscopy of all organs and sites of vaccine administration. We measured the weights of organs (heart, liver, spleen, lung, and kidney). For paired organs, we used the combined weight of both organs. We fixed organ tissues with 10% neutral buffered formalin, processed and stained them with hematoxylin and eosin for a histopathology examination.
Blood collection, hematology and biochemistry studies
We collected blood from the ventral aorta and placed it into tubes with or without EDTA for subsequent hematology studies.
We used biochemistry test kits produced by BioSino Bio-Technology & Science Inc. The hematology studies included examination of various parameters such as the red blood cell count (RBC), hemoglobin (HGB), hematocrit (HCT), mean cell volume (MCV), mean cell hemoglobin (color index of blood = 0.03х(МСН)), mean corpuscular hemoglobin concentration (MCHC) and red blood cell distribution width coefficient of variation (RDW-CV)], platelet-related parameters [platelet count (PLT), plateletcrit (PCT), mean platelet volume (MPV), and platelet distribution width (PDW)], as well as immune cell-related parameters [white blood cell count (WBC), lymphocyte count (Lymph), intermediate cell count (Mid), granulocyte count (Gran#), percentage of lymphocytes (lymph%), percentage of intermediate cells (Mid%), and percentage of granulocytes (Gran%)] and erythrocyte sedimentation rate (ESR); we conducted these studies using a Sysmex XP-100 automated hematology analyzer (Sysmex, Kobe, Japan).
We performed the biochemical studies of hepatic function-related parameters [alanine aminotransferase (ALT), aspartate aminotransferase (AST), total protein (TP), albumin (ALB), total bilirubin (TBIL), direct bilirubin (DBIL), indirect bilirubin (IBIL), and alkaline phosphatase (ALP)], renal function-related parameters [urea nitrogen (UREA) and creatinine (CREA)], and other key parameters [blood glucose (GLU)] using an automated biochemistry analyzer (7020, Hitachi, Japan).
Immunogenicity assessment
In assessing the vaccine’s immunogenicity, we used BALB/c mice of both sexes (n = 40), 6–8 weeks old; Syrian hamsters of both sexes (n = 40), 6–8 weeks old; male ferrets (n = 6), 6–7 months old; as well as male rhesus macaques (Macaca mulatta), 2–3 years old (n = 6). All the animals were delivered from specialized animal breeding facilities in Russia and Kazakhstan. We randomized the animals into groups. We used lack of external signs of disease and similar body weight (±20%) as criteria for group randomization.
Our assessment of the vaccine’s immunogenicity involved two immunizations through intramuscular introduction 21 days apart. We used PBS as a comparator. The volume of the injections was 0.5 ml. We collected animals’ blood samples to analyze antibody titers with microneutralization and ELISA assays on day 14 and 21 after the first vaccination and on day 7 after the second vaccination.
Assessment of immunogenicity in ELISA
We determined the ELISA SARS-CoV-2 antibody titer of serum samples collected from immunized animals through an indirect ELISA assay. 96-well microtiter plates were coated with 0.1 μg of purified S protein, M protein, N protein individually at 2-8̊C overnight, and blocked with 2% BSA for 1 h at room temperature. Diluted sera (1:100) were added to each well for 2 h at 37̊C, followed by incubation with goat anti-mouse antibodies conjugated with HRP for 1 h at 37̊C after a 3-time wash with commercial saline. The plate was developed using TMB, following 2 M H2SO4 addition to stopping the reaction, and read at 450/630 nm by ELISA plate reader for final data (Antibodies to SARS-CoV2 (COVID-19) IgG and M (certified Russian test systems of JSC “Vector-Best”).
Assessment of immunogenicity in MNA
Neutralizing antibody level was determined by the inhibition of cytopathic effects (CPE) defined by morphological changes and cellular death mediated by SARS-CoV on Vero cell monolayers as the most commonly used methods for quantifying antibodies. In brief, Vero cells (3 × 105 cells/ml) were cultured in 48-well microtiter plates. Pretreated serum – virus mixture, containing an equivalent volume of diluted serum and SARS-CoV (200 TCID50/ml), was inoculated into microtiter plates (1 ml/well) and incubated for 6 days at 37°C in a 5% CO2 environment. We recorded the CPE of each well every day. The maximum dilution of each serum that completely prevented CPE in 50% of the test-wells was defined as the titer of this serum when the virus control (no serum) showed complete CPE.
Statistical analysis
We performed the statistical analysis of the animal groups’ preclinical data using a Two-way RM ANOVA followed by a multiple comparisons Giesser-Greenhouse correction test.
Differences in protective efficacy between animal groups were compared using Fisher’s one-way exact test, with p < .05 considered significant, and mean values were presented with standard errors (SEM). Statistical analysis of all experimental data was performed using Graph Pad Prizm Software Version 8.0 (Graph Pad Software Inc., La Jolla, CA, USA). The experiments were repeated and the results proved reproducible.
The analysis of GMT using Dunnett’s Multiple Comparisons Test with a confidence interval of 95% after the first and second immunization identified statistically significant differences between the experimental and the control group that was given commercial PBS (** = p < .0002, *** = p < .001).
Outcomes
Acute toxicity
One of the most important indicators of acute toxicity is the death of a vaccinated animal. This study saw no deaths in animals, even those vaccinated with a maximum allowable dose of QazCovid-in® or the PBS comparator, which makes it impossible to establish the LD50.
Throughout the whole experiment we observed key integral indicators, namely: changes in the body weight; feed and water consumption; the state of the skin, fur and mucosa; behavioral reactions and motor activity of the animals. The body weight of experimental group animals did not differ from that of the control group. All animals demonstrated an increase in their body weight as seen from .
Figure 2. An analysis of body weight changes in BALB/c mice and rats before and 14 days after immunization. Body weight change (g) of vaccinated BALB/c mice (a). Body weight change (g) of vaccinated rats (b). The observation period was 14 days; the standard deviations (SD) for the mean body weight values of the groups are presented as error bars. CG: control group, EG: experimental group.
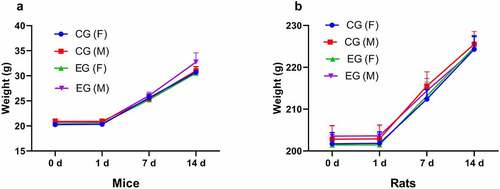
No deviations from normal vital indicators were seen in any experimental group animals on day one of observation. The animals had a healthy appearance and demonstrated proper reaction to tactile, auditory and light stimuli, while their fur was shiny, even and smooth. The rate of body weight increase in experimental group animals on day 6–7 after vaccination was comparable to that of the control group animals. These data are of significant interest because the assessed vaccine does not produce a negative effect on the body weight of laboratory animals.
The acute toxicity experiment has shown that mice and rats of both sexes tolerated the vaccine’s maximum allowable volume (5.0 ml for rats and 0.5 ml for mice) without any visible deviations. The general health condition of animals in the experimental groups, the intensity and nature of their motor activity, their motor coordination, skeletal muscular tone and color of the mucosa remained unchanged throughout the whole experiment. The frequency and depth of respiratory movements or the tail position did not differ from those in control group animals.
Postmortem examination data
The postmortem examination of experimental group animals on day 15 showed no difference in the situation of their internal organs from the anatomic pattern of the control group mice and rats. The heart was covered with the pericardium and had an oval shape cross-sectionally. The lungs were lucent, had an even color and consistency. The liver was smooth and had an even color and shape. The kidneys were of the typical bean-like shape. The other internal organs had the regular color and consistency. The stomach and intestine contained feed. The examination showed no differences in the organs of experimental versus control group animals.
The acute toxicity study of the immunobiological QazCovid-in® preparation, an inactivated COVID-19 vaccine, demonstrated that the vaccine introduced in a maximum allowable volume to laboratory animals – BALB/c mice and rats of both sexes – had no toxic or lethal effect on the animals, the latter evidenced by the absence of deaths among experimental group animals.
Sub-acute toxicity
In our sub-acute toxicity study of various doses as required by the existing GLP standard of the Republic of Kazakhstan,Citation28 we monitored the experimental group animals’ general condition, which we found to be no different from the control group. We observed no deaths in any of the groups.
The intensity and nature of the experimental group animals’ motor activity, their motor coordination, skeletal muscular tone remained unchanged throughout the whole experiment. Experimental group animals’ behavioral reactions, as well as their reactions to tactile, auditory and light stimuli remained unchanged. The condition of the fur and skin remained normal. The body weight of experimental group animals over time is shown in .
Table 1. Model rats’ body weight over time.
The data show an increase in the experimental group animals’ body weight throughout the whole observation period. The study found no statistically significant difference in the body weight increase in the experimental group compared to the control group animals ().
Figure 3. An analysis of change in the percentage deviation of the body weight (g) in the study of sub-acute toxicity of the QazCovid-in® in animals that received the vaccine IM. The observation period totaled 7 days, and the standard deviations (SD) for the mean percent deviation of body weight values of the groups are presented as error bars. CG – control group, EG – experimental group.
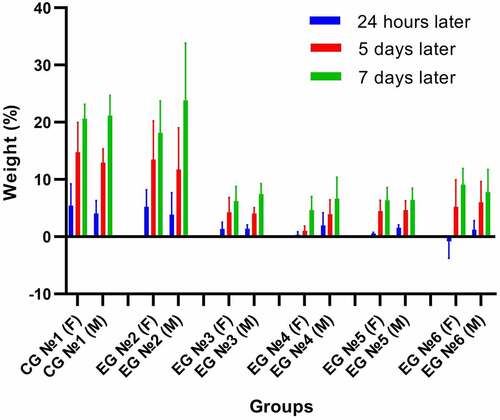
We euthanized all animals, both in the experimental and control groups, 7 days following the end of the experiment. A macroscopic examination of the animals’ internal organs identified no differences between the groups. The animals’ fur had a cleanly and shiny appearance and had no bald spots. An examination of the thoracic and peritoneal cavities did not identify any irregularities in the situation of the internal organs.
We sacrificed all animals on the seventh day following the end of the experiment. Our macroscopy studies of the animals’ internal organs identified no differences between the groups. The animals’ fur had a cleanly and shiny appearance and had no bald spots. An examination of the thoracic and peritoneal cavities did not identify any irregularities in the situation of the internal organs.
We then carried out a comparative biochemical assessment of model rats’ blood samples. As shown in , we identified a slight variation in the total protein, direct bilirubin, alanine aminotransferase (ALT) in experimental group rats; however, this did not constitute a significant difference compared to the control group (p > .05). The biochemical blood parameters in all experimental group animals were within the norm. The glucose level in all of the experimental group animals was virtually the same as that of the control group.
Table 2. Biochemical parameters associated with QazCovid-in® vaccination (n = 10, x±s.D.).
The effect of the vaccine on hematology parameters is shown in below.
Table 3. Hematology parameters associated with QazCovid-in® vaccination QazCovid-in®-COVID-19 (n = 10, x±s.D.).
An analysis of data in shows no significant differences in red blood cell levels of the control versus experimental group. However, HGB and PLT were significantly lower in experimental groups #5 and #6 than in the control group. Moreover, LYMP and GRA counts were significantly lower in rats that received 1.0 ml of the vaccine five times at 1-hour intervals (10 human doses). The HCT, MCV, PCT, MPV, WBC, MID, and ESR levels showed no significant differences between RBC levels in the control and experimental groups.
We performed statistical processing of data on the relative weight of internal organs of animals in the experimental groups comparing them with that of the control group ().
Table 4. Weight coefficient of internal organs.
As seen from , #3 experimental group animals show an increase in the weight coefficient of some internal organs (heart and spleen). It seems likely that the toxic effect is related specifically to the multiple injections of a high dose. The other animal groups demonstrate no significant differences in internal organ weight coefficients, which leads us to assume that they were not exposed to a major toxic effect.
These data are confirmed by the results of postmortem examination of only one experimental group rat.
A study of the histological structure of various organs, such as liver, heart, spleen, lungs and kidneys in the control and experimental groups showed a normal histology () and an absence of any pathological irregularities. Even though we did observe some differences, these occurred only in one or two rats of each experimental group. The other rats showed no change in their histopathology.
Figure 4. Typical histological structure of various organs (HE x 200). Normal histology of vaccinated groups (a). Liver, regular histological structure; (b). Kidney, regular histological structure; (c). Lung, regular histological structure; (d). Heart, regular histological structure; (e). Spleen, regular histological structure. Control group #1 (a). Hepatic tissue of a healthy rat; (b). Renal tissue of a healthy rat; (c). Lung tissue of a healthy rat; (d). Cardiac tissue of a healthy rat; (e). Spleen tissue of a healthy rat.
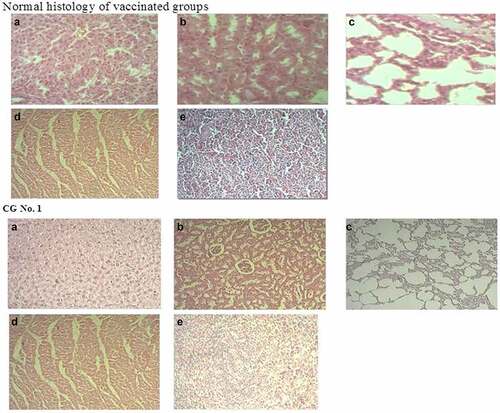
The histological structure of the liver of a control group #1 rat was characterized by lobules with the central vein in their center and hepatocytes radiating away from it. These form hepatic plates. Between these are blood and bile capillaries (). The triade – the artery, vein and biliary vessel – is located on the periphery of a set of three lobules. The histology structure of kidneys, lungs, heart, and spleen of control group #1 has no signs of damage ( (b–)).
A histology examination showed that two rats in experimental group #6 that had signs of impaired blood circulation in the form of vascular congestion and diapedetic hemorrhages additionally had dystrophy and necrosis in their organ tissues. The latter, however, were represented by microfoci. We also observed a proliferation of spleen follicle cells as well as the lungs’ interalveolar septum cells. ( (a–)).
Figure 5. Typical pathological results (HE x 200). №6 Experimental group. (a) Vascular congestion; hepatocyte dystrophy and necrocis microfoci; (b). Kidney vascular congestion; microfoci of tubule epithelium dystrophy (c). Focal proliferation of interalveolar septum cells in lungs; (d). Cardiac edema and hemorrhages; (e). Lymphoid follicle proliferation with hemorrhage microfoci.
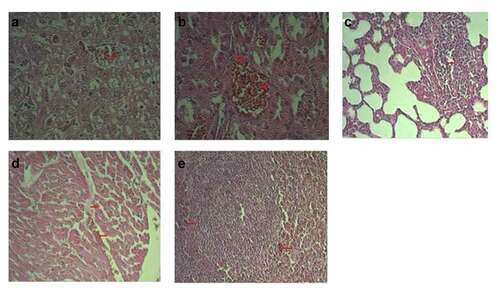
Vaccine’s immunogenicity as seen in mice, Syrian hamsters, ferrets and rhesus macaques (Macaca mulatta)
Based on the results of the QazCovid-in® vaccine’s acute and sub-acute toxicity studies, we examined the safety and immunogenic potential of one dose of the vaccine in mice, Syrian hamsters, ferrets and rhesus macaques (Macaca Mulatta) recommended for clinical studies in the volume of 0.5 ml for a double immunization.
Our immunogenicity study in macaques, mammal models with the highest affinity to humans, demonstrated that a a 5 µg dose did not cause mortality, visible behavioral changes, eating or water consumption changes, clinical infectious symptoms, neurological signs or body weight changes in any of the models.
We observed no deaths, behavioral signs, or food/water consumption differences in the rhesus macaque (Macaca mulatta) models after the vaccination and re-vaccination throughout the whole observation period. Also, none of the vaccinated animals showed clinical symptoms of the infection or any neurological signs and each animal’s body weight on the last day was not lower than on day one of observation.
Over the observation period we did not see increases in the animals’ body temperature () that would exceed the physiological norm (from 37.5°C to 39.5°C).
We studied the immunogenicity of QazCovid-in® in BALB/c mice, ferrets and rhesus macaques (Macaca mulatta) that received two 0.5 cm3 doses intramuscularly at a 21-day interval. In this study, we collected the animals’ serum samples on day 14 and 21 following the first vaccination and on day 7 following re-vaccination.
Thus, we were able to demonstrate that double immunization of animals with the QazCovid-in® vaccine promotes antibody titer growth as assessed by MNA and ELISA in mice, Syrian hamsters, ferrets, and rhesus monkeys (Macaca mulatta) (). We conducted a comparative assessment of GMT after the first immunization and re-vaccination of animals: we discovered significant variances between the experimental groups and noted GMT growth in all of them. The neutralizing and specific antibody titers in MNA and ELISA were different in mice, Syrian hamsters, ferrets, and rhesus monkeys (Macaca mulatta).
Figure 6. Body temperature of macaques immunized with QazCovid-in®, an inactivated COVID-19 vaccine. An analysis of change in body weight and temperature of re-vaccinated rhesus macaques (Macaca mulatta). An analysis of daily readings of body temperatures in macaques (with standard deviation) of the test and control groups for 14 days after the first (a) and second (b) vaccination did not identify any deviations from normal values. The observation period totaled 14 days, and the standard deviations (SD) for mean values of body temperature in groups are provided as error bars.
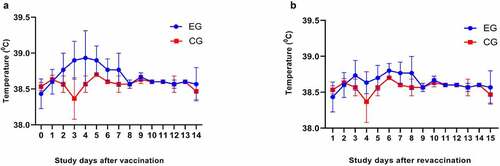
Figure 7. Antibody response in vaccinated BALB/c mice, Syrian hamsters, ferrets and rhesus monkeys (Macaca mulatta). The GMT of antibody against QazCovid-in® determined by MNA (a) and ELISA (b). An analysis of GMT using Dunnett’s Multiple Comparisons Test with a confidence interval of 95% after the first and second immunization identified statistically significant differences between experimental and control group given commercial PBS (** = p < .0002, *** = p < .001). The bars show of increase of antibodies at observation days in both gender groups. At 14, 21 days after first vaccination and 7 days following the re-vaccination no specific antibodies to saline were detected.
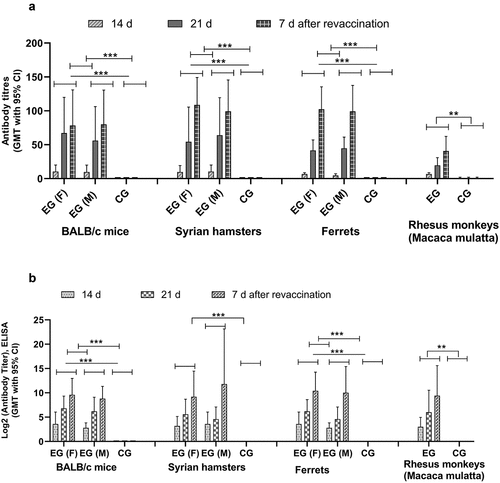
In studying the assessed vaccine’s immunogenicity, we observed a GMT growth both in the MNA and ELISA. Upon comparing the two methods, however, we observed that the MNA yielded a higher antibody GMT.
Also, we noted statistically significant differences between gender groups both in MNA and ELISA. ( and ). Moreover, the most marked immune response to QazCovid-in® was in Syrian hamsters in MNA, however specific antibody activity to SARS-CoV-2 in ELISA was also lower than in MNA ( and ).
Table 5. MNA and ELISA results for GMT in BALB/c mice, Syrian hamsters, ferrets, and rhesus monkeys (Macaca mulatta) with a CI of 95%.
Discussion
Using inactivated virus in making vaccines has been a traditional approach for decades, and COVID-19 vaccines are no exception.Citation25–27−Citation29 Inactivated whole virus vaccines have several advantages over other vaccine approaches.Citation29
Scientists from China, Turkey and Brazil developed an aluminum hydroxide-based inactivated vaccine for SARS-CoV-2 (Sinovac, China). The vaccine, CoronaVac, induces a humoral response to SARS-CoV-2 and has a good tolerability profile, warranting an approval for its emergency use during the pandemic and clinical trials.Citation30
A virus in two different mouse strains, a completely inactivated virus vaccine produced higher neutralizing and hemagglutination inhibition antibody titers than either virosomal or subunit vaccines. Over the recent years, the Research Institute for Biological Safety Problems (RIBSP) of the Ministry of Education and Science of Kazakhstan has conducted research aimed at developing inactivated viral vaccines against pandemic and seasonal influenza for use in Kazakhstan’s disease prevention programs. The quality of RIBSP’s vaccines is not inferior to that of European or American vaccines. According to WHO recommendations, each country should develop its own vaccine(s) and enlarge its production capacities.Citation31,Citation32
Animals have always been an important investigation tool in medical research. The use of animals enables researchers to carry out safety, toxicity and immunogenicity and other testing of vaccines. Si1nce humans cannot be used for most of these experiments, animals make a good substitute. This paper describes our pre-clinical study of QazCovid-in®, an inactivated COVID-19 vaccine. The current study was the first to be conducted with the objective of establishing a new vaccine for COVID-19. Our pre-clinical evaluation of the vaccine’s safety and immunogenicity used BALB/c mice, rats, ferrets, and rhesus macaques (Macaca mulatta).Citation33
Adjuvants are usually needed to enhance a vaccine’s efficacy. Literature sources provide evidence that vaccines containing adjuvants are more immunogenic compared to those that do not.Citation29 QazCovid-in® uses aluminum hydroxide as an adjuvant. The presence of this adjuvant significantly enhances the vaccine’s immunogenicity, allows minimizing the antigen dose and thus reduces associated reactogenicity.
To produce QazCovid-in®, we used a virus that we grew in Vero cells, then inactivated with formaldehyde, purified, concentrated, sterilized (by filtration) and then adsorbed on particles of aluminum hydroxide gel. Our vaccine is a formulation of virus and adjuvant in saline buffer solution. One can see adjuvants, for example SWE (Seppic, France), an equivalent of MF59, being used in research to develop subunit vaccines for SARS-CoV-2.Citation34 There have also been studies of a glycoprotein-based vaccine called NVX-CoV2372 and its effect on the spike protein where low doses of the vaccine containing the saponin-based Matrix-M adjuvant induced a high titer of anti-S IgG which blocks hACE2 receptor binding, neutralizes the virus and prevents SARS-CoV-2 infection.Citation35
A toxicology study using animal models to test low and high-dose vaccine formulations was performed by the National Center for Expertise of Medicines and Medical Devices of the Committee for Medical and Pharmaceutical Control of the Ministry of Health of the Republic of Kazakhstan (NCEMMD MH RK). Clinical and histology findings were compiled by the CRO and reported to NCEMMD MH RK. Official WHO guidelines on pre-clinical safety studies of immunobiological products do not specify particular animal types that should be used as laboratory models.
According to literature, safety studies of biological products often use BALB/c mice, rats, Syrian hamsters, guinea pigs, rabbits and rhesus macaques as models. Safety trials of QazCovid-in® were performed on BALB/c mice, rats, Syrian hamsters and rhesus macaques. Our results demonstrated our vaccine to be absolutely safe for the above animal species. Safety trials of QazCovid-in® on Syrian hamsters were conducted separately by a group of research partners.Citation35,Citation36
Our toxicity studies were based on the Kazakh Minister of Health Order #142 dated 2 April 2018 On Approval of Rules for Medical-Biological Experiments, Pre-clinical (Non-clinical) Studies and Requirements for Clinical and Preclinical Test Facilities.Citation37
No disease, mortality or injection site skin reactions (i.e. no erythema or edema) were observed in any of the animals. Hematology and clinical chemistry parameters remained within the normal range for mice and rats. Animals in the vaccinated groups exhibited no differences from the control groups in terms of group averages of body weight, body weight change over the study period, or food consumption.
The general toxicity study included the study of acute and sub-acute toxicity of QazCovid-in® in comparison with PBS used as a comparator. Our model animals were outbred mice and rats randomized into experimental and control groups.
About 95% of all laboratory animals in medical research are rats and mice bred specifically for research purposes. The reasons for their wide use include their small size, ease of handling and housing, rapid reproduction, short life span and an ability to observe several generations of the animals in a short period of time.Citation38 The Guidelines of CDSCO, India and FDA, USA on preclinical analysis of medications suggest that systemic toxicity of any drug should be compared in two different rodent species (preferably mice and rats).Citation39 Toxicity testing is designed to provide information on the safety of a vaccine before conducting further evaluation of its benefits in clinical trials. Our acute toxicity study of the vaccine that used outbred mice and rats could not establish the LD50 values as there were no deaths observed in experimental animals. The biggest dose was 0.5 ml per mature mouse and 5 ml per mature rat and was limited to the maximum administration volumes for the species. We encountered no signs of intoxication in the form of external side effects in experimental group animals. Daily monitoring of the animals for behavioral reactions showed that the vaccine did not produce any delayed effect on the general state of the animals, their orientation or emotional status. A necropsy 14 days following vaccine administration did not reveal any residual phenomena associated with intoxication.
Sub-acute toxicity studies usually take 9 to 12 months because some substances can manifest their toxicity only after several exposures to it. According to WHO guidelines, the duration of studies in animals should be based on the expected period of clinical use of the tested substance in people. It was proposed that additional peroral use of the tested substance in animals for 9–12 months is comparable to administering the same to humans for over 6 months. The corresponding period of giving the studied product to animals is determined by the period of clinical use in humans. Sub-acute toxicity studies are designed to characterize the long-term and multiple exposure profile of a vaccine on a mammal during a significant portion of this mammal’s average duration of life. The study assesses the effect on the target organs. The results of the study can be used later as confirming data in planning further clinical studies.Citation39,Citation40
The sub-acute toxicity study of the vaccine showed good tolerability in rats as no deaths or toxicity symptoms in either males or females occurred throughout the whole study period.
It is a known fact that the body weight is a sensitive indicator of xenobiotics’ toxicity in a wide range of toxicology studies.Citation41 In this study, the vaccine did not affect the increase in the body weight of animals in the experimental groups except the 6th experimental group, where female rats lost 0.81% of their weight 24 hours after the introduction of a large vaccine dose (5 ml). On day 7 after the vaccination, the body weight of animals stabilized in all groups at a virtually same level. Our sub-acute toxicity results are in line with literature data.Citation42
The parameters of our (sub-acute) toxicology studies are broader than depicted in literature and include the blood’s chemical content; hematology; organ weight analyses; and macroscopy as well as histopathology of a number of tissues including main organs such as liver, kidneys, lungs, heart and spleen.Citation43,Citation44
Hematology test data did not show significant differences between the experimental and the control groups38.39 except for the HGB and PLT levels which were substantially lower in experimental groups #5–6 compared to the control group, which suggests anemia, i.e. suppression of the body by a large dose of the studied vaccine.Citation45 LYMP and GRA counts were significantly lower in rats that received 1.0 ml of vaccine five times at 1-hour intervals (10 human doses). A drop in lymphocytes can be caused by stress; it happens within several hours after a stressful event and can last several days or even weeks.Citation16
The following data were obtained via microscopy and complex morphology studies of organs as part of the sub-acute toxicity assessment. In this study, no death, imminent death or clear clinical signs were observed in any of the experimental or control groups throughout the 7-day period after vaccination. Liver and kidneys were the target organs. The lungs and spleen displayed proliferative processes. The heart was the least affected by changes.Citation40
In summing up our data, we identified that the best tolerated dose used for two IM injections is 5 µg of the active substance (S protein) in 0.5 ml, which is potentially safe for humans. In comparison, the minimum tolerable dose of another inactivated candidate vaccine for SARS-CoV-2 (BBIBP-CorV) was a single-injections 24 µg-dose, an equivalent to 900 times the human dose, which shows a good safety characteristic of the BBIBP-CorVCitation27
The BALB/c mice, Syrian hamsters, ferrets and rhesus macaques (Macaca mulatta) developed an immunogenicity profile on day 14 after the first immunization, a complete immune response after the second immunization, and a further increase in the immune response on the 28th day of the study as assessed by the MNA and ELISA tests. Similar results were seen in other inactivated candidate vaccines for SARS-CoV-2.Citation39,Citation40 Macaques immunized with the candidate PiCoVacc vaccine, for example, displayed partial or complete immune response between the first and third PiCoVacc immunizations.Citation26
Double vaccination of laboratory animals with QazCovid-in® led to a significantly higher level of neutralizing and specific antibodies than a single vaccination. The results shown in indicate that the QazCovid-in® double immunization scheme produces significantly higher immunogenicity in laboratory animals compared to a single immunization. Similar results have been reported for another candidate inactivated vaccine, the BBIBP-CorV, developed by China’s Sinopharm.Citation27
In addition, a group of our research partners performed a study of the protective effectiveness of QazCovid-in® on Syrian hamster models. The study showed that a 5 µg dose of the specific antigen protected the animals from a 104.5 TCID50/ml dose of the wild virus. The candidate vaccine induced the formation of virus-neutralizing antibodies in vaccinated hamsters in titers of 3.3 ± 1.45 log2 to 7.25 ± 0.78 log2 which lasted 6 months (observation period). The candidate vaccine suppressed the wild virus’s replication in hamsters, prevented acute pneumonia and provided 100% survival of the animals. Also, no replicative virus was isolated from the vaccinated animals’ lungs.Citation35
Based on our results, we recommend QazCovid-in®, an inactivated COVID-19 preparation, as a safe and has highly immunogenic vaccine. While this manuscript was in preparation, Chan et al. reported that SARS-CoV-2 caused a severe lung disease in hamsters and suggested that hamsters could serve as a useful mammal model for COVID-19.Citation46–48 Based on the results of this study, we determined that the Syrian hamster would be used in the quality control of the QazCovid-in® vaccine’s immunobiological properties.
WHO has approved QazCovid-in® as a prospective candidate vaccine for coronavirus during the pandemic. Based on pre-clinical trial results, the vaccine was recommended for clinical trials on volunteers. To date, QazCovid-in® has gone through its clinical trials (phase 1, phase 2 and phase 3) (clinical trial numbers NCT04530357 and NCT04691908) and is being used in Kazakhstan for preventive vaccination.Citation36
The value of this study is the evidence it brings about the absolute safety of QazCovid-in® as tested on laboratory models; our results also demonstrate that QazCovid-in® is highly immunogenic against SARS-CoV-2 and induces specific immune response to the virus in various animal species. WHO has approved QazCovid-in® as a prospective candidate vaccine for use during the pandemic. The unique feature of the vaccine’s production technology lies in the fact that it allows using any circulating strain. At present, the Research Institute for Biological Safety Problems is working to update the QazCovid-in® by adding the Omicron variant.
Conclusion
Our study has demonstrated that the immunobiological QazCovid-in® preparation, an inactivated vaccine for COVID-19 (produced by RSE RIBSP, Ministry of Education and Science of the Republic of Kazakhstan), does not affect the general health status of laboratory animals, does not produce a negative effect on the hematological and biochemical blood parameters and main physiological functions in BALB/c mice and rats, and does not cause pathomorphological changes. None of the animals who received QazCovid-in® became sick or died.
This vaccine is prepared according to the generally accepted technology of inactivated vaccines, and is similar in design to other inactivated CoV-2 vaccines that have been approved. However, the vaccine has a number of differences from its foreign analogues in terms of the biological properties of the local of Kazakhstan strain, virus purification and concentration technology as well as dosage used. This monovalent inactivated vaccine QazCovid-in® against coronavirus was produced using a technology of whole-virion candidates adsorbed on aluminum hydroxide. Aluminum hydroxide is included in the vaccine as the adjuvant. The presence of aluminum hydroxide enhances the vaccine’s effectiveness to a great extent and makes it possible to minimize the antigen dose thus reducing the vaccine’s reactogenicity (i.e. ability to cause adverse reactions) and increasing its safety. Antigens and the adjuvant form a combination that stimulates the humoral and cellular aspects of immunity. This leads to the generation of protective protein-specific antibodies responsible for protection from coronavirus.
The development and production of this vaccine has established domestic large-scale production, thereby ensuring independence from foreign analogues, the purchase of which was scarce during the pandemic, and ensured local biological safety.
QazCovid-in® provides a steady buildup of neutralization and specific antibodies in BALB/c mice, Syrian hamsters, ferrets, and rhesus macaques (Macaca mulatta).
The study’s results indicate that QazCovid-in® is safe and has a high immunogenic potential. The results of this and other studies of the vaccine warrant recommending it for clinical research, large-scale production, and as a viable candidate vaccine in the fight against SARS-CoV-2.
Ethics approval and consent to participate
The authors affirm that human research participants were not provided in this study. All experiments involving animals were conducted in accordance with national and international laboratory animal use regulations and guidelines. The study protocol was approved by the RIBSP’s Animal Experiment Ethics Committee (Permit #0909/25).
Acknowledgments
The authors express their gratitude to associate professor Valentina Shnaukshta, CBS, Pharmacology Trial Laboratory supervisor; as well as to category 1 specialists Fariza Bazilova and Ansaya Nogaibayeva of the Pharmacology Trial Laboratory at the Almaty Branch of the National Center for Expertise of Medicines and Medical Products, Ministry of Health of Kazakhstan for their kind support in this study.
Disclosure statement
No potential conflict of interest was reported by the author(s).
Additional information
Funding
References
- COVID-19 Map—Johns Hopkins Coronavirus Resource Center. Johns Hopkins coronavirus resource center; 2020 [accessed 2022 Mar 25]. https://coronavirus.jhu.edu/map.html.
- World Health Organization. Coronavirus disease (COVID-19). Situation Report – 136; 2020 Jun. https://www.who.int/docs/default-source/coronaviruse/situation-reports/20200604-covid-19-sitrep-136.pdf?sfvrsn=fd36550b_2.
- The situation with the coronavirus; [accessed 2022 Mar 25]. https://www.coronavirus2020.kz/.
- Ahn DG, Shin HJ, Kim MH, Lee S, Kim HS, Myoung J, Kim BT, Kim SJ. Current status of epidemiology, diagnosis, therapeutics, and vaccines for novel coronavirus disease 2019 (COVID-19). J Microbiol Biotechnol. 2020;30(3):1–14. doi: 10.4014/jmb.2003.03011.
- Li H, Liu SM, Yu ZH, Tang SL, Tang CK. Coronavirus disease 2019 (COVID-19): current status and future perspectives. Int J Antimicrob Agents. 2020;55(5):105951. doi: 10.1016/j.ijantimicag.2020.105951.
- Lamb YN. Bnt162b2 mRNA COVID-19 vaccine: first approval. Drugs. 2021;81(4):495–501. doi: 10.1007/s40265-021-01480-7.
- Huang Q, Zeng J, Yan J. COVID-19 mRNA vaccines. J Genet Genomics. 2021;48(2):107–114. doi: 10.1016/j.jgg.2021.02.006.
- Park JW, Lagniton PNP, Liu Y, Xu RH. mRNA vaccines for COVID-19: what, why and how. Int J Biol Sci. 2021;17(6):1446–1460. doi: 10.7150/ijbs.59233.
- Sagili ADP, Sivakumar K, Venugopal P, Sriram DK, George M. Can mRNA vaccines turn the tables during the COVID-19 pandemic? Current status and challenges. Clin Drug Investig. 2021;41(6):499–509. doi: 10.1007/s40261-021-01022-9.
- Muthumani K, Falzarano D, Reuschel EL, Tingey C, Flingai S, Villarreal DO, Wise M, Patel A, Izmirly A, Aljuaid A, et al. A synthetic consensus anti–spike protein DNA vaccine induces protective immunity against middle east respiratory syndrome coronavirus in nonhuman primates. Sci Transl Med. 2015;7(301): 301-132. doi: 10.1126/scitranslmed.aac7462.
- Yang S, Li Y, Dai L, Wang J, He P, Li C, Fang X, Wang C, Zhao X, Huang E, et al. Safety and immunogenicity of a recombinant tandem-repeat dimeric RBD-based protein subunit vaccine (ZF2001) against COVID-19 in adults: two randomised, double-blind, placebo-controlled, phase 1 and 2 trials. Lancet Infect Dis. 2021;21(8):1107–1119. doi: 10.1016/S1473-3099(21)00127-4.
- Liang JG, Su D, Song TZ, Zeng Y, Huang W, Wu J, Xu R, Luo P, Yang X, Zhang X, et al. S-Trimer, a COVID-19 subunit vaccine candidate, induces protective immunity in nonhuman primates. Nat Commun. 2021;12(1):1346. doi: 10.1038/s41467-021-21634-1.
- Arashkia A, Jalilvand S, Mohajel N, Afchangi A, Azadmanesh K, Salehi-Vaziri M, Fazlalipour M, Pouriayevali MH, Jalali T, Mousavi Nasab SD, et al. Severe acute respiratory syndrome-coronavirus-2 spike (S) protein based vaccine candidates: state of the art and future prospects. Rev Med Virol. 2021;31(3):e2183. doi: 10.1002/rmv.2183.
- Royal JM, Simpson CA, McCormick AA, Phillips A, Hume S, Morton J, Shepherd J, Oh Y, Swope K, DeBeauchamp JL, et al. Development of a SARS-CoV-2 vaccine candidate using plant-based manufacturing and a Tobacco mosaic virus-like nano-particle. Vaccines (Basel). 2021;9(11):1347. doi: 10.3390/vaccines9111347.
- Akova M, Unal S. A randomized, double-blind, placebo-controlled phase III clinical trial to evaluate the efficacy and safety of SARS-CoV-2 vaccine (inactivated, Vero cell): a structured summary of a study protocol for a randomised controlled trial. Trials. 2021;22(1):276. doi: 10.1186/s13063-021-05180-1.
- Mohandas S, Yadav PD, Shete-Aich A, Abraham P, Vadrevu KM, Sapkal G, Mote C, Nyayanit D, Gupta N, Srinivas VK, et al. Immunogenicity and protective efficacy of BBV152, whole virion inactivated SARS- CoV-2 vaccine candidates in the Syrian hamster model. iScience. 2021;24(2):102054. doi: 10.1016/j.isci.2021.102054.
- Wang Y, Yang C, Song Y, Coleman JR, Stawowczyk M, Tafrova J, Tasker S, Boltz D, Baker R, Garcia L, et al. Scalable live-attenuated SARS-CoV-2 vaccine candidate demonstrates preclinical safety and efficacy. Proc Natl Acad Sci USA. 2021;118(29):e2102775118. doi: 10.1073/pnas.2102775118.
- Sanchez-Felipe L, Vercruysse T, Sharma S, Ma J, Lemmens V, Van Looveren D, Arkalagud Javarappa MP, Boudewijns R, Malengier-Devlies B, Liesenborghs L, et al. A single-dose live-attenuated YF17D-vectored SARS-CoV-2 vaccine candidate. Nature. 2021;590(7845):320–325. doi: 10.1038/s41586-020-3035-9.
- Munster VJ, Wells D, Lambe T, Wright D, Fischer RJ, Bushmaker T, Saturday G, van Doremalen N, Gilbert SC, de Wit E, et al. Protective efficacy of a novel simian adenovirus vaccine against lethal MERS-CoV challenge in a transgenic human DPP4 mouse model. NPJ Vaccines. 2017;2(1):28. doi: 10.1038/s41541-017-0029-1.
- Bisht H, Roberts A, Vogel L, Bukreyev A, Collins PL, Murphy BR, Subbarao K, Moss B. Severe acute respiratory syndrome coronavirus spike protein expressed by attenuated vaccinia virus protectively immunizes mice. Proc Natl Acad Sci U S a. 2004;101(17):6641–6646. doi: 10.1073/pnas.0401939101.
- Zhang H, Wang G, Li J, Nie Y, Shi X, Lian G, Wang W, Yin X, Zhao Y, Qu X, et al. Identification of an antigenic determinant on the S2 domain of the severe acute respiratory syndrome coronavirus spike glycoprotein capable of inducing neutralizing antibodies. J Virol. 2004;78(13):6938–6945. doi: 10.1128/JVI.78.13.6938-6945.2004.
- Kim TW, Lee JH, Hung CF, Peng S, Roden R, Wang M-C, Viscidi R, Tsai Y-C, He L, Chen P-J, et al. Generation and characterization of DNA vaccines targeting the nucleocapsid protein of severe acute respiratory syndrome coronavirus. J Virol. 2004;78(9):4638–4645. doi: 10.1128/JVI.78.9.4638-4645.2004.
- Mou H, Raj VS, van Kuppeveld FJ, Rottier PJ, Haagmans BL, Bosch BJ, van Kuppeveld FJM. The receptor binding domain of the new middle east respiratory syndrome coronavirus maps to a 231-residue region in the spike protein that efficiently elicits neutralizing antibodies. J Virol. 2013;87(16):9379–9383. doi: 10.1128/JVI.01277-13.
- Nyon MP, Du L, Tseng CK, Seid CA, Pollet J, Naceanceno KS, Agrawal A, Algaissi A, Peng BH, Tai W, et al. Engineering a stable CHO cell line for the expression of a MERS-coronavirus vaccine antigen. Vaccine. 2018;36(14):1853–1862. doi: 10.1016/j.vaccine.2018.02.065.
- Tang L, Zhu Q, Qin E, Yu M, Ding Z, Shi H, Cheng X, Wang C, Chang G, Zhu Q, et al. Inactivated SARS-CoV vaccine prepared from whole virus induces a high level of neutralizing antibodies in BALB/c mice. DNA Cell Biol. 2004;23(6):391–394. doi: 10.1089/104454904323145272.
- Gao Q, Bao L, Mao H, Wang L, Xu K, Yang M, Li Y, Zhu L, Wang N, Lv Z, et al. Development of an inactivated vaccine candidate for SARS-CoV-2. Science. 2020;369:77–81. doi: 10.1126/science.abc1932.
- Wang H, Zhang Y, Huang B, Deng W, Quan Y, Wang W, Xu W, Zhao Y, Li N, Zhang J, et al. Development of an inactivated vaccine candidate, BBIBP-CorV, with potent protection against SARS-CoV-2. Cell. 2020;182(3):713–721. doi: 10.1016/j.cell.2020.06.008.
- The Minister of Healthcare of the Republic of Kazakhstan. Good Laboratory Practice (GLP) requirements of the republic of Kazakhstan; 2020 [accessed 2022 May 20]. https://adilet.zan.kz/eng/docs/V2000021596
- Roberts A, Lamirande EW, Vogel L, Baras B, Goossens G, Knott I, Chen J, Ward JM, Vassilev V, Subbarao K. Immunogenicity and protective efficacy in mice and hamsters of a β-propiolactone inactivated whole virus SARS-CoV vaccine. Viral Immunol. 2010;23(5):509–519. doi: 10.1089/vim.2010.0028.
- Ricardo P, Elizabeth GP, de Roberta OP, Monica TRPC, Ana PB, Gang Z, Qianqian X, Esper GK, Jorge F, Christian FO, et al. Double-blind, randomized, placebo-controlled phase III clinical trial to evaluate the efficacy and safety of treating healthcare professionals with the adsorbed COVID-19 (inactivated) vaccine manufactured by Sinovac – PROFISCOV: a structured summary of a study protocol for a randomised controlled trial. Trials. 2020;21:853.
- Tabynov K, Kydyrbayev Z, Sansyzbay A, Khairullin B, Ryskeldinova S, Assanzhanova N, Kozhamkulov Y, Inkarbekov D. Immunogenic and protective properties of the first Kazakhstan vaccine against pandemic influenza a (H1N1) pdm09 in Ferrets. Virol Sin. 2012;27(6):344–351. doi: 10.1007/s12250-012-3272-7.
- Nurpeisova A, Kassenov M, Rametov N, Tabynov K, Renukaradhya GJ, Volgin Y, Sagymbay A, Makbuz A, Sansyzbay A, Khairullin B. Analysis of the efficacy of an adjuvant-based inactivated pandemic H5N1 influenza virus vaccine. Arch Virol. 2019;164(4):1027–1036. doi: 10.1007/s00705-019-04147-7.
- Kumar R, Lee MH, Mickael C, Kassa B, Pasha Q, Tuder R, Graham B. Pathophysiology and potential future therapeutic targets using preclinical models of COVID-19. ERJ Open Res. 2020;6(4):00405–2020. doi: 10.1183/23120541.00405-2020.
- Tabynov K, Turebekov N, Babayeva M, Fomin G, Yerubayev T, Yespolov T, Li L, Gourapura JR, Petrovsky N, Tabynov K. An adjuvanted subunit SARS-CoV-2 spike protein vaccine provides protection against Covid-19 infection and transmission. NPJ Vaccines. 2022;7(1):24. doi: 10.1038/s41541-022-00450-8.
- Zhugunissov K, Zakarya K, Khairullin B, Orynbayev M, Abduraimov Y, Kassenov M, Sultankulova K, Kerimbayev A, Nurabayev S, Myrzakhmetova B, et al. Development of the inactivated QazCovid-in vaccine: protective efficacy of the vaccine in Syrian hamsters. Front Microbiol. 2021;12:720437. doi: 10.3389/fmicb.2021.720437.
- Zakarya K, Kutumbetov L, Orynbayev M, Ye A, Sultankulova K, Kassenov M, Sarsenbayeva G, Kulmagambetov I, Davlyatshin T, Sergeeva M, et al. Safety and immunogenicity of a QazCovid-in® inactivated whole-virion vaccine against COVID-19 in healthy adults: a single-centre, randomised, single-blind, placebo-controlled phase 1 and an open-label phase 2 clinical trials with a 6 months follow-up in Kazakhstan. EClinicalMedicine. 2021;39:101078. doi: 10.1016/j.eclinm.2021.101078.
- The Minister of Healthcare of the Republic of Kazakhstan. Rules for conducting preclinical (non-clinical) studies, clinical trials; 2018 [accessed 2022 May 20]. https://adilet.zan.kz/rus/docs/V1800016768
- Delwatta SL, Gunatilake M, Baumans V, Seneviratne MD, Dissanayaka MLB, Batagoda SS, Udagedara AH, Prasad BW. Reference values for selected hematological, biochemical and physiological parameters of Sprague-Dawley rats at the animal house, faculty of medicine, University of Colombo, Sri Lanka. Animal Model Exp Med. 2018;1(4):250–254. doi: 10.1002/ame2.12041.
- Sharma S, Varsha KK, Kumari S, Gopalakrishnan V, Jose AE, Choudhary B, Mantelingu K, Raghavan SC. Acute toxicity analysis of Disarib, an inhibitor of BCL2. Sci Rep. 2020;10(1):15188. doi: 10.1038/s41598-020-72058-8.
- Sireeratawong S, Jaijoy K, Khonsung P, Lertprasertsuk N, Ingkaninan K. Acute and chronic toxicities of Bacopa monnieri extract in Sprague-Dawley rats. BMC Complement Altern Med. 2016;16(1):249. doi: 10.1186/s12906-016-1236-4.
- López Y, Infante JF, Sifontes S, Díaz D, Pérez V, Año G, Hernández T, Fernández S, Castaño JL, Cedré B, et al. Pharmacology and toxicology of an oral tablet whole cells inactivated cholera vaccine in Sprague Dawley rats. Vaccine. 2011;29(19):3596–3599. doi: 10.1016/j.vaccine.2011.02.074.
- Reynaldo O, Fraleigh NL, Lewicky JD, Fariñas M, Hernández T, Martel AL, Navarro I, Dagmar G-R, Acevedo R, Hoang-Thanh L. Repeat-Dose toxicity study using the AFPL1-conjugate nicotine vaccine in male Sprague Dawley rats. Pharmaceutics. 2020;11:626.
- Forster F. Study designs for the nonclinical safety testing of new vaccine products. J Pharmacol Toxicol. 2012;66(1):1–7. doi: 10.1016/j.vascn.2012.04.003.
- Al-Humadi N. Pre-Clinical toxicology considerations for vaccine development. Vaccine. 2017;35(43):5762–5767. doi: 10.1016/j.vaccine.2017.09.021.
- Kandeil A, Mostafa A, Hegazy RR, El-Shesheny R, Taweel EA, Gomaa MR, Shehata M, Elbaset MA, Kayed AE, Mahmoud SH, et al. Immunogenicity and safety of an inactivated SARS-CoV-2 vaccine: preclinical studies. Vaccines (Basel). 2021;9(3):214. doi: 10.3390/vaccines9030214.
- Chan J-W, Zhang AJ, Yuan S, Poon V-M, Chan C-S, Lee A-Y, W-M C, Fan Z, H-W T, Wen L, et al. Simulation of the clinical and pathological manifestations of coronavirus disease 2019 (COVID-19) in golden Syrian hamster model: implications for disease pathogenesis and transmissibility. Clin Infect Dis. 2020;71(16):2428–2446. doi: 10.1093/cid/ciaa644.
- Sia SF, Yan LM, Chin AWH, Fung K, Choy KT, Wong AYL, Kaewpreedee P, Perera RAPM, Poon LLM, Nicholls JM, et al. Pathogenesis and transmission of SARS-CoV-2 in golden hamsters. Nature. 2020;583(7818):834–838. doi: 10.1038/s41586-020-2342-5.
- Imai M, Iwatsuki-Horimoto K, Hatta M, Loeber S, Halfmann PJ, Nakajima N, Watanabe T, Ujie M, Takahashi K, Ito M, et al. Syrian hamsters as a small animal model for SARS-CoV-2 infection and countermeasure development. Proc Natl Acad Sci U S A. 2020;117(28):16587–16595. doi: 10.1073/pnas.2009799117.