ABSTRACT
Bacillus Calmette – Guerin (BCG) is an immune regulator that can enhance hippocampal synaptic plasticity in rats; however, it is unclear whether it can improve synaptic function in a mouse model with Alzheimer’s disease (AD). We hypothesized that BCG plays a protective role in AD mice and investigated its effect on dendritic morphology. The results obtained show that BCG immunization significantly increases dendritic complexity, as indicated by the increased number of dendritic intersections and branch points, as well as the increase in the fractal dimension. Furthermore, the number of primary neurites and dendritic length also increased following BCG immunization, which increased the number of spines and promoted maturation. IFN-γ and IL-4 levels increased, while TNF-α levels decreased following BCG immunization; expression levels of p-JAK2, P-STAT3, SYN, and PSD-95 also increased. Therefore, this study demonstrates that BCG immunization in APP/PS1 mice mitigated hippocampal dendritic spine pathology, especially after the third round of immunization. This effect could possibly be attributed to; changes in dendritic arborization and spine morphology or increases in SYN and PSD-95 expression levels. It could also be related to mechanisms of BCG-induced increases in IFN-γ or IL-4/JAK2/STAT3 levels.
SUMMARY
BCG immunization in a mouse model for Alzheimer’s disease significantly increased dendritic complexity, as indicated by an increase in the number of dendritic intersections and branch points, as well as an increase in the fractal dimension of hippocampal CA1 neurons.
Introduction
While the central nervous system (CNS) is capable of monitoring the peripheral immune status, the latter can also affect the former.Citation1,Citation2 Furthermore, moderate immune enhancement can stimulate the body to produce protective and repair effects, promote neurogenesis, and improve synaptic transmission.Citation3,Citation4 Bacillus Calmette – Guerin (BCG) is a planned vaccine in China. To prevent tuberculosis, babies are inoculated with BCG within 24 h following their birth. Specifically, BCG, a live attenuated vaccine against bacilli, shows strong nonspecific immune stimulation and can induce Th1 cell immunity-dominated immune responses.Citation5,Citation6 Furthermore, BCG is not only associated with tuberculosis prevention, but also offers protection against other diseases, especially in the nervous system. Recent studies have shown that BCG is also an immune modulator and can play a neuroprotective role in animal models for nervous system diseases, such as Parkinson’s syndrome and Japanese encephalitis.Citation7–9 It was reported that BCG vaccination (8–10 weeks old mice vaccinated with live BCG 2 × 107 CFU intraperitoneally) partially preserved markers for striatal dopamine system integrity and prevented an increase in activated microglia in the MPTP mouse model.Citation7 Further study suggested that BCG doses of 1–6 × 106 CFU may have greater beneficial effects than the 2 × 107 CFU dose.Citation8
Alzheimer’s disease (AD) is a neurodegenerative disease, and immunotherapy has been identified as an important direction for clinical treatment. The use of BCG in APP/PS1 mice has been reported;Citation10,Citation11 however, the specific mechanism for the neuroprotective effect of BCG on synaptic damage remains unclear.
Synaptic damage is the basis for memory impairment in patients with AD. Quantitative studies using electron microscopy and immunohistochemical staining have shown that patients with AD are characterized by a significantly decreased dendritic spine density in the hippocampus and related cerebral cortex.Citation12
The hippocampus is important for learning and memory, and its dendritic morphology is related to this function. Moreover, in the learning and memory processes, synaptic transmission is often accompanied by morphological changes, such as the formation, abscission, expansion, and atrophy of dendritic spines, which are important components of the synapse that show great plasticity.Citation13,Citation14 Thus, morphological changes in the dendritic spines are often an integral part of studies on the hippocampal morphology. The implication of synaptic loss or synaptic dysfunction in the etiology of AD has been the subject of intense investigation, mainly using animal models of the disease.Citation15,Citation16 Therefore, in this study, our aim is to investigate the neuroprotective effects of BCG immunization on dendritic morphology in APP/PS1 mice, with respect to dendritic morphology We concentrated on studying the morphology of their dendritic length, dendritic complexity, the density and detailed shapes of their dendritic spines. To visualize dendritic structure, DiOlistic labeling was used to analyze morphology, dendritic branching and dendritic spines in the hippocampus.
Materials and methods
Animals
Three-month-old APP/PS1 male mice were purchased from the Laboratory Animal Center of Guangzhou University of Chinese Medicine (Guangzhou, China) and reared in a specific facility free of pathogens. All mice were housed at 22–25°C, and had free access to food and water during a 12-h light/dark cycle. All experimental procedures were approved by the Experimental Animal Ethics Committee of our university (approval #GD 2019–129). Thirty-six male APP/PS1 mice were used in these procedures.
Specifically, the APP/PS1 mice were randomly assigned to either the experimental or matched control groups. Mice in the APP+BCG group were administered BCG, while those in the control group were injected with sterile phosphate-buffered saline (PBS) via the same protocol as that used for mice in the APP+BCG group.
Experimental design
APP/PS1 mice were randomly divided into three pairs of mutually matched experimental and control groups. The experimental groups consisted of mice immunized with BCG once, twice, or three times, and were named APP/PS1 + 1BCG, APP/PS1 + 2BCG, and APP/PS1 + 3BCG groups, respectively. The matched control groups were injected with PBS, identical to the protocol in the experimental groups. Each experiment consisted of six groups (i.e., three experimental groups and three matched control groups) and was conducted when the mice were at the age of 20 weeks ().
Figure 1. Experimental procedure. 3-month-old APP/PS1 mice were randomly divided into three pairs of mutually matched experimental and control groups. The experimental groups consisted of mice that were immunized with BCG once, twice, or three times and were named APP/PS1 + 1BCG, APP/PS1 + 2BCG, and APP/PS1 + 3BCG groups, respectively. The matched control groups were injected with PBS according to the same protocol as that for mice in the experimental groups. Mice in the APP/PS1 + 1BCG group were administered a single dose of the treatment at 18 weeks old. Those in the APP/PS1 + 2BCG group were administered a two-dose series of the treatment at 16 and 18 weeks, while those in the APP/PS1 + 3BCG group were administered a three-dose series at 14, 16, and 18 weeks. The control APP/PS1 mice were injected intradermally with 50 μL/mouse sterile PBS following the predetermined protocol. Two weeks after the last injection, the mice were anesthetized, and their tissue was harvested.
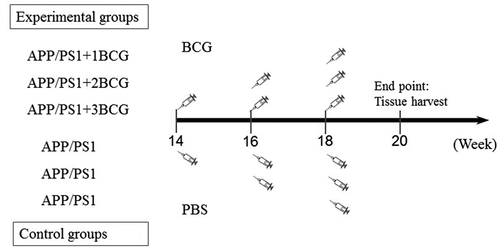
Vaccination
Live freeze-dried BCG (D2-BP302 strain; Biological Institute of Shanghai, Shanghai, China) was suspended in sterile PBS. Thereafter, mice in the APP/PS1 + 1BCG group were administered a single dose of this treatment at 18–weeks-old. Those in the APP/PS1 + 2BCG group were administered a two-dose series of treatment at 16 and 18 weeks, while those in the APP/PS1 + 3BCG group were administered a three-dose series at 14, 16, and 18 weeks ().
The backs of the APP/PS1+BCG and control APP/PS1 mice were each injected intradermally with 50 μL of BCG suspension containing 1 × 106 colony forming units (CFU) and 50 μL/mouse of sterile PBS, respectively, following the predetermined protocol. This dose was initially chosen because BCG doses of 1–6 × 106 CFU may reportedly have greater beneficial effects than the 2 × 107 CFU dose in the mouse model of PD. Additionally, a lower dose of 1 × 105 CFU BCG was not neuroprotective. Thus, a dose of 1–6 × 106 CFU BCG appears to be optimal for our research.Citation8,Citation17
Tissue preparation
Preparation of sections was conducted following a previously described method.Citation18 Briefly, 20% urethane was injected intraperitoneally to anesthetize mice (1.5 g/kg). Thereafter, thoracotomy was performed, and the mice brains were collected and perfused with 2% paraformaldehyde for 2 h. Notably, perfusion speed was high at first, then subsequently reduced. Finally, a vibratome (VT 1000S; Leica, Bannockburn, IL, USA) was used to obtain 200-µm wide coronal slices. These slices were then stored in 0.1 mol/L PBS buffer for DiI staining.
DiOlistic labeling
To analyze the morphology of dendrites and the spines of hippocampal CA1 neurons from 5-month-old mice, the neurons were marked with the fluorescent dye, 1-1’-Dioctadecyl-3,3,3,’3’-tetramethylindocarbocyanine perchlorate (DiI; CM-DiI; Sigma-Aldrich, St. Louis, MO, USA) as previously described.Citation18 Briefly, 2 mg of DiI was fully mixed with 8 mg of 1.0-µm gold particles (BioRad, Hercules, CA, USA). Given that DiI is soluble in organic solvents, after 300 µL of methylene chloride or dimethylformamide was added to it, a solution was formed. Thus, DiI adhered to the surface of the gold particles. Thereafter, the DiI-gold particle warhead was placed in a gene gun, and the particles were scattered on the surface of the brain slices using helium (150–180 p.s.i.). This was followed by rinsing of the brain slices twice with 0.1 M PBS. Finally, the brain slices were stored in 0.1 M PBS and incubated in a 4°C refrigerator for 12–24 h.
Photography and confocal imaging
A blind observer was assigned to acquire and analyze the image. The brain slices were imaged under a laser scanning confocal microscope (LSM 710, Carl Zeiss, Germany). To fully observe single neurons, the 20x objective was selected to adjust optical zoom, and a ray wavelength of 561 nm was selected to excite DiI. After determining branch level and distance, the 63x oil lens was selected, and optical zoom was adjusted to enable the selected dendritic segment to reach maximum pixel distribution. Thereafter, the required dendritic segment was placed at the center of the field of vision. Subsequently, 3–5 secondary dendrites from each neuron were imaged (1024 × 1024 pixels, x – y scaling = 0.0952 μm/pixel); at least three neurons were collected per mouse.Citation19–21 Finally, images of the dendrites and spines were analyzed using Imaris software version 7.0 (Bitplane Inc., St Paul, MN, USA).
Sholl analysis
ImageJ software (National Institute of Health; http://rsb.info.nih.gov/ij/) was used to perform the dendritic morphological analysis. Confocal images of each CA1 neuron were 3D-reconstructed to analyze the dendritic morphological changes. To track the 2D-projection image of the neurons, NIH ImageJ software and ‘NeuronJ’ plugin were used. Thus, Sholl analysis was performed using NIH ImageJ software as previously described.Citation22 Briefly, a series of cell body-centered concentric circles with an increasing radius (10 mm interval) were generated by the ImageJ concentric circle plug-in. Thereafter, the number of dendritic intersections corresponding to each image on each circle was calculated and averaged. The number of dendritic intersections on each circle under each condition was also calculated.
Fractal analysis
Fractal analysis (FD) has proved to be an excellent way to analyze complexity of neuronal dendrites, hence it was used for analysis of the DiI-labeled hippocampal CA1 neuron images. As previously described,Citation23 the box-counting method was used to determine FD of the neurons. Specifically, neurons were projected at the same scale, transformed into binary files, and skeletonized, such that the diameter corresponding to all processes involving the whole neuron profile then corresponded to one pixel. Additionally, we focused on the quantitative analysis of dendritic arborization patterns. Thus, cell bodies and axons were removed from digitized images of the neurons. Thereafter, ImageJ software was used to analyze images using the fractal box counter tool. The calculated FD was found to be in the range of 1 ≤ D ≤ 2. Generally, the higher the FD value, the more complex the dendritic pattern.
Western blot analysis
Hippocampal tissue was collected from the mice at 5-months-old (n = 4) and homogenized in an ice-cold RIPA lysis buffer containing 50.0 mM Tris – HCl, 150 mM NaCl, 5.0 mM CaCl2, 0.02% NaN3, and 1% Triton X-100 in the presence of a protease inhibitor mixture (1 mM PMSF Protease Inhibitor; Sigma-Aldrich). Thereafter, lysates were centrifuged at 12,000 × g for 20 min at 4°C, and the protein concentrations were determined using the bicinchoninic acid (BCA) protein assay kit (Beyotime Biotechnology, Jiangsu, China). In brief, we separated the samples (20 μL in each lane) through 8% sodium dodecyl sulfate – polyacrylamide gel electrophoresis (SDS-PAGE), and electromigration to poly vinylidene fluoride (PVDF) membranes (Bio-Rad) was performed at 70 V for 30 min, then at 110 V for 60 min. The electrophoresis process was terminated once the bromophenol blue was used up. The membranes were then collected and blocked with 5% nonfat milk in tris-buffered saline for 2 h at 20–25°C. Subsequently, the membranes were incubated overnight at 4°C with primary antibodies. Specifically, the primary antibodies were used at the following concentrations: synaptophysin (SYN) (1:500, AB1543P, Sigma-Aldrich), postsynaptic density protein 95 (PSD-95) (1:1000, AB9708, Sigma-Aldrich), Janus kinase 2 (JAK2) (1:1000, 74987S, Cell Signaling Technology, Beverly, MA, USA), STAT3 (1:2000, 4904S, Cell Signaling Technology), phospho-JAK2 (p-JAK2) (1:2000, T56570 M, Abmart, Shanghai, China), phosphorylated signal transducer and activator of transcription 3 (p-STAT3) (1:1000, 13,332, Signalway Antibody, College Park, MD, USA), and β-actin (1:1000; Beyotime Biotechnology). Following incubation, the primary antibodies were diluted with a blocking solution, and PVDF membranes were washed three times with PBST (100 nM phosphate buffer, pH 7.5, containing 150 nM NaCl and 0.1% Tween-20) for 10 min each. Thereafter, the secondary antibody (1:5000, P9375, Sigma-Aldrich) was added, and the mixture was gently shaken on a shaking table at 20–25°C for 2 h. In the next step, the secondary antibody was diluted with BSA blocking solution, and the membranes were rinsed three times with PBST for 10 min each. Immunoblotting was then performed on the films using an enhanced chemiluminescence system (Pierce Chemical Co., Rockford, IL, USA). Thereafter, the films were scanned, and optical density values of the target strips were analyzed using NIH ImageJ software. All assays were performed in triplicates.
Enzyme-linked immunosorbent assay (ELISA)
The TNF-α, IL-4, and IFN-γ in serum levels and hippocampus samples from the 5-month-old mice were measured using an ELISA kit in accordance with the manufacturer’s protocol. Briefly, we used a buffer (pH 7.6) containing 150 mM NaCl, 50.0 mM Tris – HCl, 5.0 mM CaCl2, 1% Triton X-100, and 0.02% NaN3 to homogenize the samples on ice. Thereafter, the samples were centrifuged at 17,000 × g for 30 min at 4°C. In accordance with a previous study,Citation24 we used an enhanced BCA Protein Assay Kit (Beyotime Biotechnology) to tune the total hippocampal homogenate concentration to 4.5 mg/mL. Finally, the prepared plates were analyzed at 450 nm using a microplate reader (ELx800, BioTek, Winooski, VT, USA).
Statistical analysis
Statistical analysis was conducted using SPSS version 13.0 (SPSS for Windows, Armonk, NY, USA) and GraphPad Prism 5.0 (GraphPad Software, San Diego, CA, USA). Statistical significance was set at p < .05. The results obtained are presented as the mean ± SEM.
Results
Changes in dendritic morphology following APP/PS1 mice immunization with BCG
shows a representative image of the typical pyramidal cell used for analysis. It also indicates different compartments of the dendritic cell. The primary neurite numbers (p < .01, ), total dendritic length (p < .05, ), apical dendritic length (p < .05, ), and average dendritic length (p < .05, ) were significantly increased following BCG treatment (three immunizations). Additionally, the number of primary neurites and total dendritic length corresponding to the APP/PS1 + 2BCG mice group were significantly higher (p < .05, ) than those corresponding to the APP/PS1 mice group. The data indicates that both the number of primary neurites and neurite stretching increased in APP/PS1 mice following BCG application.
Figure 2. Regulatory effects of Bacillus Calmette – Guerin (BCG) treatment on dendritic morphology in the hippocampal CA1 area of APP/PS1 mice. (a) Representative image of a typical pyramidal cell used for analysis. The different compartments of the dendritic tree are indicated. (b) Histogram showing a comparison of the number of primary neurites in the hippocampal pyramidal cells, which increased following BCG treatment two and three times, relative to the number corresponding to the APP/PS1 mice; 1, 2, and 3 represents APP/PS1 mice injected with BCG once, twice, and three times, respectively. (c–e) APP/PS1 + 3BCG mice showing significant increases in total dendritic length, apical dendritic length, and average dendritic length, respectively, compared with APP/PS1 mice. APP/PS1 + 2BCG showed an increased apical dendritic length at two weeks following treatment. Data were analyzed by performing Student’s t-test, and the results obtained are presented as the mean ± SEM. n = 9 neurons (three neurons each from the three mice). *p <.05 and **p <.01 vs. vehicle control.
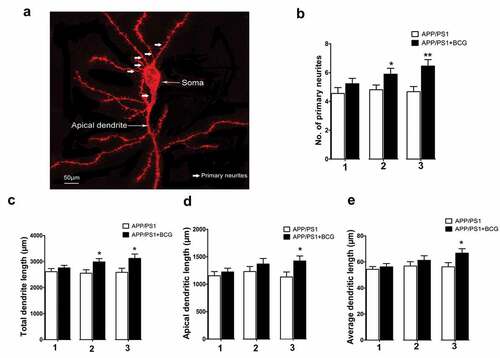
Changes in hippocampal dendritic complexity following APP/PS1 mice immunization with BCG
BCG plays an important role in dendritic embranchment and promotes dendritic complexity.Citation25 To research whether BCG immunization brought about changes in dendritic complexity in the hippocampal CA1 neurons, we performed a Sholl analysis that involved placing concentric rings at fixed intervals from the cell body, then determining the intersection between each ring and dendrite.Citation23,Citation26 The number of intersections, number of branch points, and fractal dimension were used as indicators for morphological complexity. Thus, it was observed that the three-time BCG immunization resulted in an obvious increase in dendritic complexity as compared with that of the control APP/PS1 mice (p < .05, p < .01, ). Moreover, there was a significant increase in the number of intersections in APP/PS1+BCG mice at a distance of 60–110 mm from the soma as compared with the controls (p < .05, p < .01, ).
Figure 3. Regulatory effect of Bacillus Calmette – Guerin (BCG) treatment on dendritic complexity in the hippocampal CA1 area of the APP/PS1 mice. (a) Graph showing the variation of dendritic complexity as the function of the distance to the cell body of the hippocampal neurons in the CA1 area under control conditions or BCG vaccination. The dendritic complexity of the neurons expressing the indicated constructs was measured via the Sholl analysis. Intersections at various radial distances (10–200 mm) from the center of the cell soma were significantly improved following three-time BCG immunizations. (b) Fractal dimension (FD) of CA1 neurons in the different experimental groups. APP/PS1 + 3BCG mice showed a significant increase in FD. (c) APP +3BCG group showing a significant increase in the number of branch points. Data were analyzed by performing the Student’s t-test and the results obtained are presented as the mean ± SEM. n = 9 neurons (three neurons each from the three mice). *p <.05 and **p <.01 vs. vehicle control.
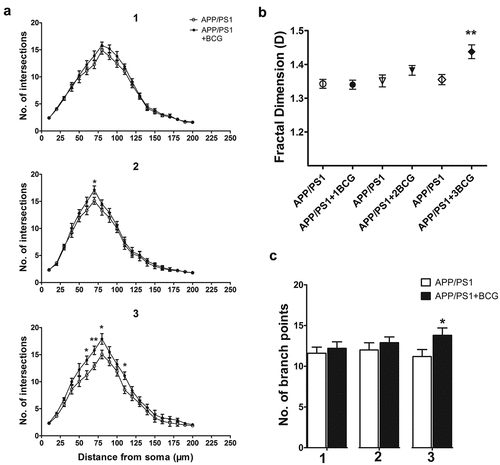
Changes in hippocampal spine morphology following APP/PS1 mice immunization with BCG
Hippocampal spines are associated with cognition, and reportedly play an important role in information transmission.Citation27,Citation28 Additionally, to some extent, changes in spine morphology are responsible for neuronal circuit remodeling.Citation29 Therefore, we assessed the hippocampal spine morphology via DiOlistic labeling. The pyramidal cell body, apical dendrites, several lateral, basilar dendrites, and dendritic spines could be recognized. Assessment of the dendritic spine number per unit length, spine area, spine head width, and spine length was performed from the lateral dendrites (). Thus, we observed significant increases in the spine density (p < .05, ), area (p < .01, ), and head width (p < .01, ) in APP/PS1 + 3BCG mice as compared with that of the control mice. However, there was no significant difference in the spine morphology of mice with only one BCG immunization. The increase in the spine area was also significantly higher for APP/PS1 + 2BCG mice (p < .05, ) than for the APP/PS1 mice.
Figure 4. Regulatory effect of Bacillus Calmette – Guerin (BCG) treatment on spine morphology in the hippocampal CA1 area. (a) Use of DiOlistic assay to visualize dendritic spines. Individual hippocampal neurons corresponding to APP/PS1 and APP/PS1+BCG mice; representative sections of lateral dendrites from APP/PS1 and APP/PS1+BCG groups are also shown. Three-time immunization with BCG increased spine density (b), spine area (c), and spine head width (e) relative to APP/PS1 mice. Spine length in APP/PS1+BCG mice show no significant alterations relative to the control mice (d). Data were analyzed by performing Student’s t-test and the results obtained are presented as the mean ± SEM. n = 9 neurons (three dendritic segments per neuron, and three neurons each from the three mice). *p <.05 and **p <.01 vs. vehicle control.
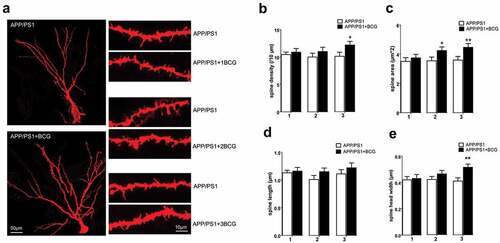
Changes in cytokine levels in APP/PS1 mice serum and hippocampus following BCG immunization
After observing the changes in dendritic and spine morphology, we then considered the potential effects of the immune-related diffusible mediators in modulating neural network functioning. Previous studies have focused on several pro-inflammatory cytokines, including IFN-γ, IL-4, and TNF-α, which have detrimental effects on the neuronal function and dendritic morphology.Citation4,Citation30 Therefore, we investigated the potential changes in the levels of these immune activation and dendritic morphology related-mediators. Our results revealed that the APP+BCG groups, especially the APP +3BCG group, displayed increased serum IFN-γ and IL-4, and decreased serum TNF-α levels (). We then examined cytokine levels in the hippocampus to determine whether changes in the peripheral cytokine levels affected cytokine levels in the CNS. As expected, there were significant increases in the IFN-γ and IL-4 levels, while the level of TNF-α showed a significant decrease in the hippocampus of mice in the APP+BCG groups, especially APP +3BCG (). Thus, it is evident that BCG immunization alters cytokine expression in both the serum and hippocampus. Moreover, alteration trends corresponding to the serum and hippocampus were similar, suggesting that an internal link exists between them.
Figure 5. Alteration of the cytokines levels in the hippocampus and serum following Bacillus Calmette – Guerin (BCG) treatment. The normalized and analyzed levels of TNF-α, IL-4, and IFN-γ in serum (a) and hippocampus (b); 1, 2, and 3 represents APP/PS1 mice injected with BCG once, twice, and three times, respectively. In serum, BCG vaccination up-regulated IL-4 and IFN-γ expression, and down-regulated TNF-α expression, and changes in the levels of these cytokines in the hippocampus were almost consistent with those in serum. Data were analyzed by performing Student’s t-test and the results obtained are presented as the mean ± SEM normalized to the controls. n = 6 for each group. *p <.05, **p <.01 and #p <.001 vs. vehicle control.
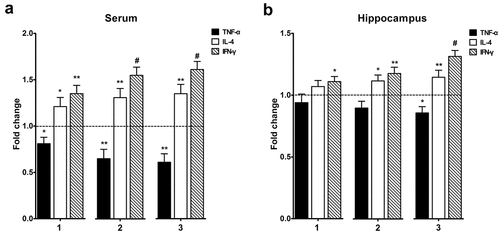
Changes in JAK2/STAT3 expression and synaptic proteins in the hippocampus of APP/PS1 mice following immunization with BCG
Changes in the levels of peripheral and CNS inflammatory factors owing to the BCG immunization may be primarily responsible for the BCG neuroprotective effect. The specific mechanism by which inflammatory factors affect neural circuits has not yet been explored. Furthermore, JAK2 and STAT3, which are expressed in glial cells and hippocampal neurons, are related to the development of Parkinson’s disease (PD) and AD;Citation31–33 hence, their signaling pathways are involved in neuroimmunity regulation. Therefore, we investigated JAK2/STAT3 expression in the hippocampus of mice. As shown in ), BCG immunization upregulated p-JAK2 and p-STAT3 expression (p < .05, p < .01), suggesting that the cytokines may play a neuroprotective role by activating this pathway. We also detected synaptophysin and PSD-95, further verifying the neuroprotective effect of the cytokines. Specifically, synaptophysin is a major integral membrane protein in the synaptic vesicles,Citation34 and its main functions include docking, fusion, and endocytosis, otherwise known as membrane trafficking.Citation35 Furthermore, PSD-95, i.e., the major scaffolding protein that contributes to excitatory postsynaptic density (PSD), is a potent regulator of synaptic strength and maturation, and has been considered a key synaptic protein that promotes synapse stability.Citation28,Citation29,Citation36,Citation37 The APP/PS1 + 3BCG group displayed an increase in SYN (p < .01, ) and PSD-95 (p < .05, ) expression, indicating that changes in the synaptic proteins are associated with spine morphology.
Figure 6. Regulatory effect of the Bacillus Calmette – Guerin (BCG) treatment on the expression of JAK2/STAT3 and synaptic proteins in the hippocampus. APP/PS1 + 2BCG and APP/PS1 + 3BCG groups showed significant increases in the expression levels of p-JAK2 (c) and p-STAT3 (e) compared with their matched controls. The APP/PS1 + 3BCG group showed increases in the expression levels of SYN (f) and PSD-95 (g). Data were analyzed by performing Student’s t-test and the results obtained are presented as the mean ± SEM normalized to the matched control. n = 4 per group. *p <.05 and **p <.01 vs. The control group.
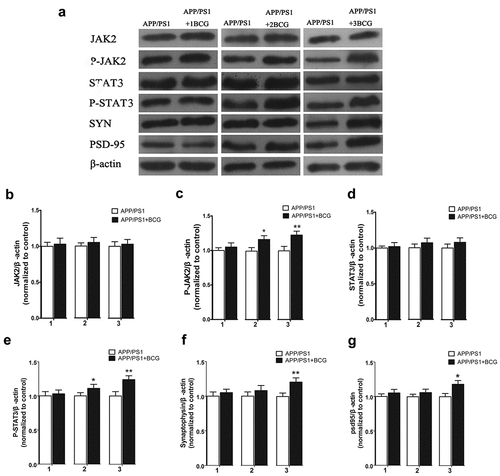
Discussion
It has been proposed that BCG plays a neuroprotective role in nervous system diseases, such as Parkinson’s syndrome and Japanese encephalitis, as an immunomodulator.Citation7–9 Our research group previously observed that BCG improves cognitive impairment in APP/PS1 mice and reduces the neuroinflammatory responses, suggesting the existence of an altered cytokine dynamic that possibly impacts the dendritic morphology in the hippocampus.Citation10,Citation11 Hence, in this study, we analyzed the dendrites complexity of hippocampal pyramidal neurons following immunization of APP/PS1 mice with BCG. It is worth noting that, APP/PS1 mice showed cognitive decline from the age of 6 months, however, hippocampal mossy fiber synapses display morphological and functional presynaptic deficits in middle-aged mice (3 to 6 months).Citation38 Accordingly, APP/PS1mice over 6 months old are usually selected for studies on cognitive deficits, so they chose 9-month-old mice in Ref 10 and 11. Our manuscript focused on the changes of synaptic morphology, so we choose 14 weeks old mice (middle age) to inject BCG to study whether BCG can prevent the changes of synaptic morphology.
A decreased number of neurons, synapse loss, and the resulting brain atrophy are significant pathological features of AD. Previous studies have shown that there is a synaptic transmission dysfunction, as well as synaptic plasticity in the brains of patients with AD. This has also been confirmed through in vivo and in vitro animal experiments.Citation12–41 Additionally, hippocampus changes represents one of the most obvious pathological changes and synaptic damage is the basis for memory impairment in patients with AD. Additionally, quantitative studies using electron microscopy and immunohistochemical staining have shown that the hippocampal spine density is significantly decreased in patients with AD,Citation12 and this brings about a corresponding decrease in the presynaptic and postsynaptic components. However, in this study, BCG immunization prevented this subsequent synaptic damage in APP/PS1 mice. Our results also indicate that dendritic complexity in APP/PS1 mice increased after BCG immunization, resulting in an increase in the available surface area for synaptic contact and enhanced neuronal connection capability. The number of intersections and branch points, as well as FD of the dendrites collectively represents dendritic complexity. These changes in dendritic complexity in APP/PS1+BCG mice could be attributed to: (1) alteration of the neural activity of hippocampal circuits or (2) compensatory mechanisms for preventing the neural pathway imbalance. Here, we observed that APP/PS1 mice immunized with BCG displayed an increased number of primary neurites as well as an increased dendritic length. It has been demonstrated that a greater number of primary neurites and dendritic length enhance synaptic transmission.Citation42 The changes in dendritic morphology observed in this study indicate that BCG prevents subsequent synaptic damage in the APP/PS1 mice.
Studies have shown that cognitive impairment in AD is related to dendritic spine loss, especially in the hippocampus.Citation43,Citation44 Specifically, dendritic spines are polymorphic structures that play a key role in the acquisition, integration, and transmission of nerve signals. Therefore, changes to their density and morphology may affect synaptic transmission. Thus, we observed that BCG immunization increased the spine density, area, and head width, indicating that the effects of BCG on the hippocampal spine pathology observed in APP/PS1+BCG mice might be related to the eradication of synaptic degeneration.
Additionally, a considerable number of studies have confirmed that changes in the peripheral immune response can regulate the CNS’s environment.Citation3,Citation4 Specifically, Schwartz et al.Citation3,Citation45 reported that CNS-specific T cells can protect neurons from secondary injury, and the effect of T cells on the CNS is determined by time and intensity of the immunization. An appropriate immune enhancement can also induce body tissue repair, promote neurogenesis, and improve synaptic plasticity; however, an excessive immune response does not favor the survival of neurons, and it affects their morphology as a large number of inflammatory factors are released. The immune responses induced by BCG have been widely studied, and the results have shown that these responses are activators for antigen-presenting cells (APCs) and strong inducers of IFN-γ-secreting Th1-type T cells.Citation46,Citation47 Furthermore, an increasing number of researchers have started using BCG to induce the T cell response to ameliorate symptoms of neurodegenerative diseases.Citation7,Citation8 BCG vaccination inhibits systemic inflammation, which is associated with inflammation-mediated neurodegeneration through glia-neurons interaction, in several neurological disorders of the CNS including Multiple Sclerosis (MS), AD, and Parkinson’s disease (PD), as well as in neurocognitive disorders. Based on the reports for BCG and neurodegenerative diseases, its strain and dose-dependent mechanism of action, related to modulation of peripheral and CNS immune components, are primarily responsible for its neuroprotective effect.Citation48 Accordingly, in this study, we investigated changes in the levels of the immune factors as well as their effects on dendritic morphology in APP/PS1 mice after BCG immunization. Thus, we observed an increase in the IFN-γ and IL-4 levels, regarded as neurotrophic factors, and a decrease in the TNF-α level in both the serum and hippocampus of APP+BCG mice, relative to the control mice; changes were more pronounced in the APP +3BCG group. These changes in the cytokines levels in the hippocampus were similar to that in serum, thus indicating that there is a close connection between the brain and peripheral immune system. Reportedly, cytokines in peripheral blood can enter the CNS and directly affect information transmission between neurons.Citation49 However, the specific mechanism underlying this protective effect remains unclear. Therefore, we further investigated the effect of cytokines on the dendritic morphology.
The JAK/STAT signaling pathway plays an important role in the CNS. Specifically, JAK2 and STAT3, which are related to neurodegenerative disease progress, including AD and PD, are expressed in the glial cells and hippocampal neurons,Citation31–33 and their pathway is also involved in neuroimmunity regulation. Our results revealed that BCG upregulated p-JAK2 and p-STAT3 expression, suggesting that cytokines may play a neuroprotective role through activation of the JAK2/STAT3 signaling pathway. Several proteins involved in regulation of dendrites and spine morphology are present on the presynaptic and postsynaptic membranes. To further verify the molecular mechanism by which BCG exerts the neuroprotective effect, we analyzed SYN and PSD-95 levels. Thus, we observed that changes in the SYN and PSD-95 levels were almost parallel to changes in the p-JAK2 and p-STAT3 levels, besides dendritic complexity and spine density. There is reportedly a correlation between spine density, PSD-95, and the immune response.Citation50,Citation51 Although immune factors participate in the physiological and pathological events, depending on the PSD-95 level,Citation52 it is still unclear how BCG-related cytokines regulate synaptic protein expression.
Alterations in the dendritic structure and spine morphology occurred after two or three BCG immunizations. These changes paralleled the increase in the IFN- γ, IL-4, p-JAK2, p-STAT3, SYN, and PSD-95 levels in the hippocampus. Consistent with results from previous studies, this study revealed that BCG immunization induced a Th1 cytokine response (increased IFN-γ and IL-4) and had a beneficial effect on the synaptic transmission. Furthermore, BCG has a dual function. It is both an antigen and adjuvant. Thus, it enhances immune responses. Repeated BCG administration reportedly induces an increased humoral response in mice; however, if only a single dose of BCG is administered, this humoral response gradually diminishes within a few weeks.Citation53 Moreover, repeated BCG immunization can enhance IFN-γ and IL-4 levels as compared to a single BCG dose. Accordingly, in this study, repeated BCG stimulation in APP/PS1 mice showed an intensive immune activation in terms of the increased IL-4 and IFN-γ levels, with a concomitant increase in the p-JAK2 and p-STAT3 levels in the hippocampus. Similar changes were observed for SYN and PSD-95.
It was previously reported that BCG exerts neuro-beneficial effects in terms of the physiological status following BCG immunization at a single dose of 1 × 105 CFU at P0.Citation18,Citation54 However, in this study, which was conducted as part of a pathological study, a higher dose of BCG (1 × 106 CFU) was repeatedly injected into adult APP/PS1 mice. Thus, the different observations made regarding the physiological and pathological status of the neurons possibly resulted from differences in the dose of the living BCG, which then resulted in different types of T cells being stimulated and different Tregs levels.Citation55 This was further validated by the quantity and quality differences in the induced cytokines and neurotrophic factors, as well as other factors. Despite these differences, the neuro-beneficial effects of BCG were evident both pathologically and physiologically.
It is worth noting that in addition to dendrite complexity and spine morphology, a different methodology has emerged in the study of brain-learning. It has shown efficient backpropagation and Hebbian learning on dendritic trees for the dendritic adaptation, which indicated the deep learning could be realized by a single dendrite or neuron. This experiment of dendritic adaptation is expected to be an alternative of the hypothesis of synaptic plasticity.Citation56 Dendritic adaptation is an important new methodology in the study of brain learning; However, the methodology of dendritic adaptation still need to be refined and further verified.
In summary, the results of this study demonstrates that immunization of APP/PS1 mice with BCG mitigates hippocampal dendritic spine pathology, especially after the third immunization. Additionally, changes in dendritic characteristics and spine morphology, together with increases in the SYN and PSD-95 levels, possibly reflects the enhanced synaptic transmission. Moreover, the neuroprotective effect of BCG administration in APP/PS1 mice may be related to the mechanisms of increased IFN-γ or IL-4/JAK2/STAT3 following the BCG vaccination. This study provides the basic data for future experiments involving repeated BCG vaccination in animal models. However, future animal research supported by human clinical trials is required to determine whether BCG can be used in the prevention of Alzheimer’s disease or recommended as an immunomodulatory agent in combination with another therapy.
Acknowledgment
We would like to thank Editage (www.editage.cn) for the English language editing.
Disclosure statement
No potential conflict of interest was reported by the author(s).
Additional information
Funding
References
- Baecher-Allan C, Kaskow BJ, Weiner HL. Multiple sclerosis: mechanisms and immunotherapy. Neuron. 2018;97(4):1–11. doi:10.1016/j.neuron.2018.01.021.
- Cédile O, Wlodarczyk A, Owens T. CCL2 recruits T cells into the brain in a CCR2-independent manner. APMIS. 2017;125(11):945–56. doi:10.1111/apm.12740.
- Ziv Y, Schwartz M. Immune-based regulation of adult neurogenesis: implications for learning and memory. Brain Behav Immun. 2008;22(2):167–76. doi:10.1016/j.bbi.2007.08.006.
- Yirmiya R, Goshen I. Immune modulation of learning, memory, neural plasticity and neurogenesis. Brain Behav Immun. 2011;25(2):181–213. doi:10.1016/j.bbi.2010.10.015.
- Ota MO, Vekemans J, Schlegel-Haueter SE, Fielding K, Sanneh M, Kidd M, Newport MJ, Aaby P, Whittle H, Lambert P-H, et al. Influence of mycobacterium bovis Bacillus Calmette-Guérin on antibody and cytokine responses to human neonatal vaccination. J Immunol (Baltimore, MD : 1950). 2002;168(2):919–25. doi:10.4049/jimmunol.168.2.919.
- Libraty DH, Zhang L, Woda M, Acosta LP, Obcena A, Brion JD, Capeding RZ. Neonatal BCG vaccination is associated with enhanced T-helper 1 immune responses to heterologous infant vaccines. Trials Vaccinol. 2014;3:1–5. doi:10.1016/j.trivac.2013.11.004.
- Yong J, Lacan G, Dang H, Hsieh T, Middleton B, Wasserfall C, Tian J, Melega WP, Kaufman DL. BCG vaccine-induced neuroprotection in a mouse model of Parkinson’s disease. PloS One. 2011;6(1):e16610. doi:10.1371/journal.pone.0016610.
- Laćan G, Dang H, Middleton B, Horwitz MA, Tian J, Melega WP, Kaufman DL. Bacillus Calmette-Guerin vaccine-mediated neuroprotection is associated with regulatory T-cell induction in the 1-methyl-4-phenyl-1,2,3,6-tetrahydropyridine mouse model of Parkinson’s disease. J Neurosci Res. 2013;91(10):1292–302. doi:10.1002/jnr.23253.
- Kulkarni S, Mukherjee S, Pandey A, Dahake R, Padmanabhan U, Chowdhary AS. Bacillus Calmette-Guérin confers neuroprotection in a murine model of Japanese encephalitis. Neuroimmunomodulation. 2016;23(5–6):278–86. doi:10.1159/000452171.
- Zuo Z, Qi F, Yang J, Wang X, Wu Y, Wen Y, Yuan Q, Zou J, Guo K, Yao ZB. Immunization with Bacillus Calmette-Guérin (BCG) alleviates neuroinflammation and cognitive deficits in APP/PS1 mice via the recruitment of inflammation-resolving monocytes to the brain. Neurobiol Dis. 2017;101:27–39. doi:10.1016/j.nbd.2017.02.001.
- Zuo Z, Qi F, Xing Z, Yuan L, Yang Y, He Z, Zhou L, Yao Z. Bacille Calmette-Guérin attenuates vascular amyloid pathology and maximizes synaptic preservation in APP/PS1 mice following active amyloid-β immunotherapy. Neurobiol Aging. 2021;101:94–108. doi:10.1016/j.neurobiolaging.2021.01.001.
- Leshchyns’Ka I, Liew HT, Shepherd C, Halliday GM, Stevens CH, Ke YD, Ittner LM, Sytnyk V. Aβ-dependent reduction of NCAM2-mediated synaptic adhesion contributes to synapse loss in Alzheimer’s disease. Nat Commun. 2015;6(1):8836. doi:10.1038/ncomms9836.
- Kampa BM, Letzkus JJ, Stuart GJ. Dendritic mechanisms controlling spike-timing-dependent synaptic plasticity. Trends Neurosci. 2007;30(9):456–63. doi:10.1016/j.tins.2007.06.010.
- Kasai H, Matsuzaki M, Noguchi J, Yasumatsu N, Honkura N. Structure-stability-function relationships of dendritic spines. Tanpakushitsu kakusan koso Protein Nucleic Acid Enzyme. 2004;49:276–81.
- Forner S, Baglietto-Vargas D, Martini AC, Trujillo-Estrada L, LaFerla FM. Synaptic Impairment in Alzheimer’s disease: a dysregulated symphony. Trends Neurosci. 2017;40(6):347–57. doi:10.1016/j.tins.2017.04.002.
- Marchetti C, Marie H. Hippocampal synaptic plasticity in Alzheimer’s disease: what have we learned so far from transgenic models? Rev Neurosci. 2011;22(4):373–402. doi:10.1515/rns.2011.035.
- Kiros TG, Power CA, Wei G, Bretscher PA. Immunization of newborn and adult mice with low numbers of BCG leads to Th1 responses, Th1 imprints and enhanced protection upon BCG challenge. Immunotherapy. 2010;2(1):25–35. doi:10.2217/imt.09.80.
- Li Q, Qi F, Yang J, Zhang L, Gu H, Zou J, Yuan Q, Yao Z. Neonatal vaccination with Bacillus Calmette–Guérin and hepatitis B vaccines modulates hippocampal synaptic plasticity in rats. J Neuroimmunol. 2015;288:1–12. doi:10.1016/j.jneuroim.2015.08.019.
- Wartman BC, Holahan MR. The impact of multiple memory formation on dendritic complexity in the hippocampus and anterior cingulate cortex assessed at recent and remote time points. Front Behav Neurosci. 2014;8:128. doi:10.3389/fnbeh.2014.00128.
- Pathania M, Davenport EC, Muir J, Sheehan DF, López-Doménech G, Kittler JT. The autism and schizophrenia associated gene CYFIP1 is critical for the maintenance of dendritic complexity and the stabilization of mature spines. Transl Psychiatry. 2014;4(3):e374. doi:10.1038/tp.2014.16.
- Ortiz JB, Anglin JM, Daas EJ, Paode PR, Nishimura K, Conrad CD. BDNF and TrkB mediate the improvement from chronic stress-induced spatial memory deficits and CA3 dendritic retraction. Neuroscience. 2018;388:330–46. doi:10.1016/j.neuroscience.2018.07.049.
- Duan X, Chang JH, Ge S, Faulkner RL, Kim JY, Kitabatake Y, Liu X-B, Yang C-H, Jordan JD, Ma DK, et al. Disrupted-in-schizophrenia 1 regulates integration of newly generated neurons in the adult brain. Cell. 2007;130(6):1146–58. doi:10.1016/j.cell.2007.07.010.
- Milosević NT, Ristanović D. Fractality of dendritic arborization of spinal cord neurons. Neurosci Lett. 2006;396(3):172–76. doi:10.1016/j.neulet.2005.11.031.
- Selenica ML, Alvarez JA, Nash KR, Lee DC, Cao C, Lin X, Reid P, Mouton PR, Morgan D, Gordon MN. Diverse activation of microglia by chemokine (C-C motif) ligand 2 overexpression in brain. J Neuroinflammation. 2013;10(1):86. doi:10.1186/1742-2094-10-86.
- Li Q, Zhang Y, Zou J, Qi F, Yang J, Yuan Q, Yao Z. Neonatal vaccination with Bacille Calmette-Guérin promotes the dendritic development of hippocampal neurons. Human Vaccines Immunother. 2016;12(1):140–49. doi:10.1080/21645515.2015.1056954.
- Sholl DA. Dendritic organization in the neurons of the visual and motor cortices of the cat. J Anat. 1953;87:387–406.
- Geinisman Y. Structural synaptic modifications associated with hippocampal LTP and behavioral learning. Cerebral Cortex (New York, NY: 1991). 2000;10(10):952–62. doi:10.1093/cercor/10.10.952.
- Urbanska M, Swiech L, Jaworski J. Developmental plasticity of the dendritic compartment: focus on the cytoskeleton. Adv Exp Med Biol. 2012;970:265–84.
- Alvarez VA, Sabatini BL. Anatomical and physiological plasticity of dendritic spines. Annu Rev Neurosci. 2007;30(1):79–97. doi:10.1146/annurev.neuro.30.051606.094222.
- Spedding M, Gressens P Neurotrophins and cytokines in neuronal plasticity. Novartis Found Symp. 2008;289:222–33; discussion 233–240.
- Nicolas CS, Peineau S, Amici M, Csaba Z, Fafouri A, Javalet C, Collett V, Hildebrandt L, Seaton G, Choi S-L, et al. The Jak/STAT pathway is involved in synaptic plasticity. Neuron. 2012;73(2):374–90. doi:10.1016/j.neuron.2011.11.024.
- Nicolas CS, Amici M, Bortolotto ZA, Doherty A, Csaba Z, Fafouri A, Dournaud P, Gressens P, Collingridge GL, Peineau S. The role of JAK-STAT signaling within the CNS. JAK-STAT. 2013;2(1):e22925. doi:10.4161/jkst.22925.
- Chiba T, Yamada M, Sasabe J, Terashita K, Shimoda M, Matsuoka M, Aiso S. Amyloid-β causes memory impairment by disturbing the JAK2/STAT3 axis in hippocampal neurons. Mol Psychiatry. 2009;14(2):206–22. doi:10.1038/mp.2008.105.
- Thiel G. Synapsin I, synapsin II, and synaptophysin: marker proteins of synaptic vesicles. Brain Pathol (Zurich, Switzerland). 1993;3(1):87–95. doi:10.1111/j.1750-3639.1993.tb00729.x.
- Evans GJ, Cousin MA. Tyrosine phosphorylation of synaptophysin in synaptic vesicle recycling. Biochem Soc Trans. 2005;33(6):1350–53. doi:10.1042/BST0331350.
- Chen X, Nelson CD, Li X, Winters CA, Azzam R, Sousa AA, Leapman RD, Gainer H, Sheng M, Reese TS. PSD-95 is required to sustain the molecular organization of the postsynaptic density. J Neurosci. 2011;31(17):6329–38. doi:10.1523/JNEUROSCI.5968-10.2011.
- Taft CE, Turrigiano GG. PSD-95 promotes the stabilization of young synaptic contacts. Philos Trans Royal Soc B: Biol Sci. 2014;369(1633):20130134. doi:10.1098/rstb.2013.0134.
- Barthet G, Mulle C. Presynaptic failure in Alzheimer’s disease. Prog Neurobiol. 2020;194:101801. doi:10.1016/j.pneurobio.2020.101801.
- Lei M, Xu H, Li Z, Wang Z, O’Malley TT, Zhang D, Walsh DM, Xu P, Selkoe DJ, Li S. Soluble Aβ oligomers impair hippocampal LTP by disrupting glutamatergic/GABAergic balance. Neurobiol Dis. 2016;85:111–21. doi:10.1016/j.nbd.2015.10.019.
- Selkoe DJ. Alzheimer’s disease is a synaptic failure. Science (New York, NY). 2002;298(5594):789–91. doi:10.1126/science.1074069.
- Guo L, Tian J, Du H. Mitochondrial dysfunction and synaptic transmission failure in Alzheimer’s disease. J Alzheimer’s Dis: JAD. 2017;57(4):1071–86. doi:10.3233/JAD-160702.
- Jan YN, Jan LY. Dendrites. Genes Dev. 2001;15(20):2627–41. doi:10.1101/gad.916501.
- Meilandt WJ, Ngu H, Gogineni A, Lalehzadeh G, Lee S-H, Srinivasan K, Imperio J, Wu T, Weber M, Kruse AJ, et al. Trem2 deletion reduces late-stage amyloid plaque accumulation, elevates the Aβ42:aβ40 ratio, and exacerbates axonal dystrophy and dendritic spine loss in the PS2APP Alzheimer’s Mouse Model. J Neurosci. 2020;40(9):1956–74. doi:10.1523/JNEUROSCI.1871-19.2019.
- Manczak M, Kandimalla R, Yin X, Reddy PH. Hippocampal mutant APP and amyloid beta-induced cognitive decline, dendritic spine loss, defective autophagy, mitophagy and mitochondrial abnormalities in a mouse model of Alzheimer’s disease. Hum Mol Genet. 2018;27(8):1332–42. doi:10.1093/hmg/ddy042.
- Schwartz M, Shechter R. Protective autoimmunity functions by intracranial immunosurveillance to support the mind: the missing link between health and disease. Mol Psychiatry. 2010;15(4):342–54. doi:10.1038/mp.2010.31.
- Kumar M, Behera AK, Matsuse H, Lockey RF, Mohapatra SS. A recombinant BCG vaccine generates a Th1-like response and inhibits IgE synthesis in BALB/c mice. Immunology. 1999;97(3):515–21. doi:10.1046/j.1365-2567.1999.00782.x.
- Teixeira HC, Munk ME, Kaufmann SH. Frequencies of IFNγ- and IL-4-producing cells during Mycobacterium bovis BCG infection in two genetically susceptible mouse strains: role of αβ T cells and NK1.1 cells. Immunol Lett. 1995;46(1–2):15–19. doi:10.1016/0165-2478(95)00009-T.
- Cossu D, Ruberto S, Yokoyama K, Hattori N, Sechi LA. Efficacy of BCG vaccine in animal models of neurological disorders. Vaccine. 2022;40(3):432–36. doi:10.1016/j.vaccine.2021.12.005.
- Banks WA. Blood-brain barrier transport of cytokines: a mechanism for neuropathology. curr Pharm Des. 2005;11(8):973–84. doi:10.2174/1381612053381684.
- Chugh D, Nilsson P, Afjei SA, Bakochi A, Ekdahl CT. Brain inflammation induces post-synaptic changes during early synapse formation in adult-born hippocampal neurons. Exp Neurol. 2013;250:176–88. doi:10.1016/j.expneurol.2013.09.005.
- Jakubs K, Nanobashvili A, Bonde S, Ekdahl CT, Kokaia Z, Kokaia M, Lindvall O. Environment matters: synaptic properties of neurons born in the epileptic adult brain develop to reduce excitability. Neuron. 2006;52(6):1047–59. doi:10.1016/j.neuron.2006.11.004.
- Gardoni F, Boraso M, Zianni E, Corsini E, Galli CL, Cattabeni F, Marinovich M, Di Luca M, Viviani B. Distribution of interleukin-1 receptor complex at the synaptic membrane driven by interleukin-1β and NMDA stimulation. J Neuroinflammation. 2011;8(1):14. doi:10.1186/1742-2094-8-14.
- Husain AA, Kashyap RS, Kalorey DR, Warke SR, Purohit HJ, Taori GM, Daginawal HF. Effect of repeat dose of BCG vaccination on humoral response in mice model. Indian J Exp Biol. 2011;49:7–10.
- Yang J, Qi F, Gu H, Zou J, Yang Y, Yuan Q, Yao Z. Neonatal BCG vaccination of mice improves neurogenesis and behavior in early life. Brain Res Bull. 2016;120:25–33. doi:10.1016/j.brainresbull.2015.10.012.
- Burl S, Adetifa UJ, Cox M, Touray E, Ota MO, Marchant A, Whittle H, McShane H, Rowland-Jones SL, Flanagan KL. Delaying Bacillus Calmette-Guérin vaccination from birth to 4 1/2 months of age reduces postvaccination Th1 and IL-17 responses but leads to comparable mycobacterial responses at 9 months of age. J Immunol (Baltimore, MD: 1950). 2010;185(4):2620–28. doi:10.4049/jimmunol.1000552.
- Hodassman S, Vardi R, Tugendhaft Y, Goldental A, Kanter I. Efficient dendritic learning as an alternative to synaptic plasticity hypothesis. Sci Rep. 2022;12(1):6571. doi:10.1038/s41598-022-10466-8.