ABSTRACT
The success of mRNA vaccines against SARS-CoV-2 implies that this technology can be applied to target any pathogen. However, the scientific production and research trends using the bibliometric method are still unknown. The top 100 most cited articles on mRNA vaccine research were obtained from the Scopus database from 1995 to 2021. Bibliometrix, an R-Package, and VOSviewer 1.6.11 were used for data analysis. There is a rapid growth in scientific outputs with a gradual increase in 2021. The United States produced 45 (45%) of the articles, followed by Germany with 15 (15%) and Israel with 10 (10%). The New England Journal of Medicine published the most papers in this field 13 (13%), followed by Nature 6(6%). Barney S. Graham was the most productive author among the top 100 most cited mRNA vaccine articles. University of Pennsylvania Perelman School of Medicine, US, was the top ranking institution, having 37 (37%). The visualization map clearly and spontaneously displayed the current state and research hot spots of mRNA research from a specific perspective. The most frequent keywords were COVID-19, vaccine, mRNA vaccine, mRNA, SARS-CoV-2, and immunogenicity, among others. A systematic review of the articles provided evidence that out of 100 articles, approximately 25 (25%) were focused on vaccine production and evaluation, followed by 26 (26%) in mRNA vaccine safety and efficacy, 23 (23%) were into mRNA vaccination, 23 (23%) considered risk factors associated with mRNA vaccination, while 8 (8%) of the articles covered the issue of mRNA vaccine delivery. In addition, 42% of the articles focused on COVID-19, 17% on cancer, 8% on influenza virus, 4% on COVID-19 and kidney disease, 3% COVID-19 and myocarditis, and 3% on rabies virus, among others. The findings of this systematic and thematic analysis provided the knowledge basis for further research on mRNA vaccines globally.
Introduction
Vaccines induce immune responses by simulating the infection process inside the human body and enabling it to develop lasting immunity. When the body is re-exposed to the target antigens, it can produce antibodies that neutralize the antigens and therefore prevent infection.Citation1 Research conducted by Louis Pasteur in the 19th century resulted in the discovery of attenuated pathogens that were inoculated into a subject, to prevent them from infection when exposed to the same pathogen.Citation2
The mRNA vaccines represent a potentially disruptive technology for the vaccine industry.Citation3 Messenger RNA is produced by in vitro reactions with recombinant enzymes, ribo-nucleotide triphosphates, and a DNA template; thus, it is rapid and relatively simple to produce compared with the others were vaccine production platforms.Citation4 A co-formulation of the mRNA of the RNA is encapsulated in lipid nanoparticles that protect the RNA strands and help their absorption into the cells. Upon delivery, cells will take up the mRNA and translate it into protein in situ. The individual’s immune system will then mount a robust adaptive immune response against the target protein.Citation5
Most works with mRNA vaccine were done with cancer,Citation4 and infectious diseases caused by Zika virus, the dengue virus, the respiratory syncytial virus, HIV, influenza H7N9 and Flavivirus,Citation6 and interestingly noninfectious diseases such as diabetes.Citation3 The first mRNA vaccine against an infectious disease that was evaluated in humans targeted the rabies virus, and a clinical trial showed that it elicited functional antibodies.Citation7 The success of mRNA vaccines against SARS-CoV-2 implies that this technology can target any pathogen for which a protein can be identified as an antigen that elicits protective immunity.Citation8
Messenger RNA vaccines have several advantages over conventional vaccines since they do not integrate into the host genome.Citation4 A single mRNA vaccine can encode multiple antigens, strengthening the immune response against resilient pathogens Citation9 and enabling the targeting of multiple microbes or viral variants with a single formulation.Citation10 The simplicity of in vitro transcription of mRNA suggests the potential for fast, scalable, and low-cost manufacturing.Citation11
Many researchers in medical and other scientific fields have used the concept of bibliometrics. Thus, researchers have used both the Web of Sciences and Scopus databases, followed by a quantitative analysis based on mathematical statistics to quantitatively reflect the research hotspots and reveal the future trends of new theories.Citation1–12–Citation25 So far, although there are many articles published on mRNA vaccines globally, no study on the top 100 most cited articles on mRNA vaccines has been conducted. Hence, this study aimed to provide a comprehensive analysis using a systematic and thematic analysis of the top 100 most cited articles published in mRNA vaccine research via analyzing the global publishing landscape, author, and country performance of publications related to mRNA vaccine research retrieved from the Scopus database. Based on developmental trend of mRNA vaccine trends, this paper proposed the aspects that need further study in the future, which may provide a reference for researchers in raising awareness of potential research gaps and research collaboration toward the development of mRNA vaccines to control infectious diseases.
Methods
Study design
A descriptive bibliometric study using a systematic and thematic analysis was performed to evaluate the top 100 most cited articles on global mRNA vaccines through a quantitative analysis.
Study participants
No human subjects were directly involved in this study. The metadata included in the study was searched and collected from the Scopus database (https://www.scopus.com/). Online databases provide an extensive, comprehensive, and multidisciplinary core journal citation index database. The comprehensive online bibliographic database reported in the study was accessed via the electronic library of Southeast University, Nanjing, China.
Publications’ timespan
The top 100 most cited articles in the mRNA vaccine were identified matching the search criteria and were published from 1995 to 2021.
Data sources and search strategy
The data used in this study were extracted from the Scopus database. The search was conducted on September 18, 2022, using the boolean operators such as “AND” or “NOT,” to ensures that we gather accurate information related to the top 100 most cited articles on mRNA vaccine indexed in the Scopus database:
The following search was conducted using the query search as (TITLE (mrna AND vaccine*) OR TITLE (mrna AND vaccination*) OR TITLE (mrna AND vaccine*)) AND (EXCLUDE (PUBYEAR, 2022) OR EXCLUDE (PUBYEAR, 1994)) AND (LIMIT-TO (DOCTYPE, “ar”)) AND (LIMIT-TO (LANGUAGE, “English”))
In total, 733 mRNA publications retrieved were within the Timespan: 1995 to December 31, 2021, and then the top 100 cited articles in mRNA were selected based on the citation times. The raw data was exported into BiB text, comma-separated value (csv) excel, or RIS file format for further in-depth statistical analysis. Finally, the top 100 cited articles on mRNA vaccine were comprehensively analyzed based on the bibliographical information (). The retrieved mRNA data included citation information, bibliographical information, annual trend per year, author’s country or region, source publication, the collaboration between author and countries, institution, and keywords (author keyword, keyword Plus, and Abstract) were analyzed using bibliometric tools. The journal Impact factor (JIF) was determined by the Journal Citation Report 2021. The current study does not require ethical approval and, like other bibliometric analyses, used secondary data without any human participants.
Bibliometric analysis
Data were analyzed using the open-source R. Studio software (www.rstudio.com), version 4.0.5, and bibliometrix, an R-Package,Citation26 VOSviewer 1.6.11 software was used to create authorship co-occurrence network by units of authors and countries.Citation27 The thickness shown in the VOSviewer mapping, specifically the connecting lines between nodes represents the degree of connection between authors or countries. The thicker the line, the stronger the connection.Citation28,Citation29 In addition, current approaches and directions for future research and main contributions to mRNA vaccine are summarized.
Results
Basic characteristics of the data
The annual number of the top 100 most cited articles in mRNA vaccine was published between 1995 and 2021 (). The scientific publications of the top 100 most cited mRNA vaccines have increased gradually after the year 2020 (). Overall, the retrieved articles received a total of 309.7 average citations per documents, with an annual growth rate of 16.9%. The articles were published in 48 journal sources, contributed by 3107 authors, 37.3% coauthors per documents, and 40% international co-authorship. The other characteristics of current mRNA vaccine research outputs are summarized in .
Table 1. The top 100 cited articles on the mRNA vaccine.
The top 100 most cited articles
The top 100 cited articles on mRNA were published from 1995 to 2021, with citation range (83 to 5757) times.Citation7–30–Citation54–56–Citation62–65–Citation67–69–Citation72–104–Citation106–108–Citation116–118–Citation128 The article titled “Safety and efficacy of the BNT162b2 mRNA COVID-19 vaccine” by Polack F.P., et al. 2020 published in the New England Journal of Medicine was the top cited article cited 5757 times.Citation30 However, the article “Efficacy and safety of the mRNA-1273 SARS-CoV-2 vaccine” by Baden L.R., et al. 2021 published in the same journal was ranked second, receiving 3768 citations Citation31 as presented in ().
Authorship impact on mRNA vaccine research
Out of the 3107 authors who contributed to the top 100 most cited articles, only authors with ≥5 h_index () and authors with over 6000 citation times of articles are presented in . The analysis of authors shows Barney S Graham from Vaccine Research Center, National Institute of Allergy and Infectious Diseases, National Institutes of Health, Bethesda, USA, was the most productive author in this field with eight publications, followed by Hamilton Bennett from Moderna, Inc., Cambridge, United States, Cambridge, USA, with 7 articles, and Brett Leav from Brigham and Women’s Hospital, Boston, and Moderna, Cambridge, USA, with 7 articles (). Barney S. Graham was the most influential authors among the top 100 most cited mRNA vaccine research based on the number of classical citations with 7967 citations ().
Table 2. The top most productive authors with ≥5 articles.
Table 3. The top most influence authors with over 6000 citation times.
Authorship pattern analysis
Analysis of the authorship pattern on the top 100 most cited articles on mRNA vaccine based on the frequency of publication by authors was described using Lotka’s law (). From the figures, the proportion of all authors based on the number of documents written, 85.84% publish only one article, 10.43% publish two articles, 2.51% publish three articles, and so on a ccording to Lotka’s law.
Analysis of the top productive Journals
According to quantitative analysis, a total of 48 journal sources were reported publishing the top 100 cited articles in mRNA vaccine. The New England Journal of Medicine published 13 articles and cited 15,072 times. The core journals with high recognition in this field can be determined by the researcher to publish their work or search for the articles relevant to mRNA vaccine ().
Table 4. Source local impact with three or more than in mRNA vaccine.
Corresponding author’s country
Of 18 countries that contributed, only the authors with 2 or more articles were reported (). The United States is the top leading country in the top 100 most cited mRNA vaccine research with 45 articles, followed by Germany with 15 articles, and Israel with 10 articles. In addition, the USA, Germany, and Israel were also the most influential in mRNA research based on the number of classical citations reported globally.
Table 5. Corresponding Author’s Country and its Citations.
Top productive institutions in mRNA vaccine
Studies on mRNA vaccines were contributed by 463 different authorship institutions listed in . The leading productive institutions are the University of Pennsylvania Perelman School of Medicine, with 37 articles, and followed by Washington University School of Medicine with, 15 articles, and Vanderbilt University Medical Center with 15 articles, among others ().
Table 6. The top productive institutions in mRNA vaccine research with six or more articles.
Research frontiers based on the keyword
The keywords used by the authors indicate the content of the articles. Visual representation of word frequency was carried out using R-studio, and Word clouds were used to analyze the underlying patterns in the text. From the mapping, we identified that the top Keywords Plus in mRNA vaccine were “Female” (133), “male” (102), “adult” (99), “article” (92), “human” (89), “vaccination” (85), “middle-aged” (80), “RNA” (79), “aged” (78), “messenger RNA” (78) as presented in . Additionally, the top-frequency Author’s Keywords were “COVID-19” (14), “Vaccine” (9), “mRNA vaccine” (7), “SARS-CoV-2” (7), “Immunogenicity” (5), “vaccination” (5), “lipid nanoparticle” (4), and “dendritic cell,” among others, as presented in .
Figure 5. Visualization text analysis results with Word Clouds summarizing the top 100 most commonly mRNA Keywords plus provided by Journal (a), and Author’s Keywords (b).
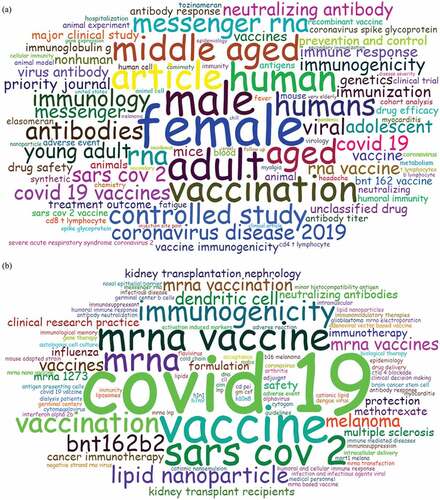
Furthermore, to identify the relationships between topics, the verification was done through Multiple correspondence analysis (MCA) that used dimensionality reduction techniques to draw a conceptual structure map of the 75 Keywords in the mRNA field. This is shown as 5 clusters with the five different colors to show the association between the published topics having similar common concepts ().
Conceptual structure analysis
A variety of themes form the foundation of the mRNA vaccine research field. A comprehensive analysis of the themes of the mRNA was carried out using a thematic map. This strategy combines performance analysis and scientific mapping tools to detect and visualize conceptual subdomains. We used the Author’s Keywords (), keywords plus (), and Title () to generate the thematic map and set three parameters to maintain the richness of information on m RNA vaccine without impairing map readability. The algorithm built the map from the 250 most frequent Author keywords, Keywords Plus, and Title considering only keywords with a minimum frequency of three occurrences per 1,000 documents, with a limit of three keywords for each cluster. The algorithm formed nine clusters in Authors Keywords, three clusters in Keywords Plus, and seven clusters in tiles dispersed in the thematic map.
Figure 7. Thematic Analysis map develop by using Conceptual structure for Author’s Keywords (a), Keyword Plus (b), and Keywords extracted from title (c), clusters produced by Louvain clustering algorithm.
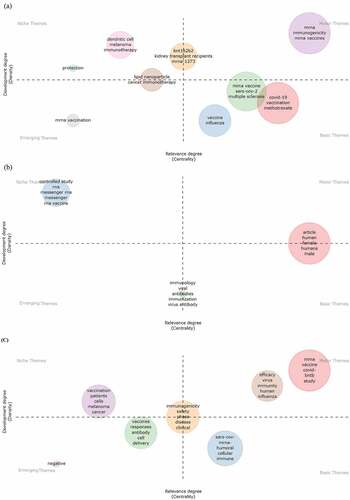
The figure shows the development of the topic into four quadrants comprising the following: first quadrant (top right): motor-themes, both important and well developed; the second quadrant (top left): Niche themes or highly development and isolated themes, which have developed well but the topic reported on the current mRNA vaccines; the third quadrant (bottom left): emerging topic or disappearing themes for mRNA vaccine research; and the fourth quadrant (bottom right): basic themes or basic and transversal Themes, which is important to the field mRNA vaccine research but not well-developed ().
Co-authorship relations among authors
We used the features of the VOSviewer software to create co-occurrence networks, which reported co-authorship by units of authors and countries () of 3107 authors who contributed to the top 100 cited articles, and a minimum of 2 documents from each author were chosen, resulting in 98 meeting the threshold. Only 52 authors were distributed across five clusters highlighted in different colors, with links between authors (L = 193) and total link strength (TLS = 299). Additionally, in , 18 countries were reported and 2 minimum number of documents were chosen, resulting in 19 countries distributed across six clusters, with links between authors (L = 49) and total link strength (TLS = 110).
A systematic review of the top 100 most cited articles identified on mRNA vaccine
The objectives and the main findings of the top 100 cited articles on mRNA vaccine are summarized in . Among the top 100 most cited articles, 25% were related to vaccine production and evaluation,Citation35–37–Citation40–42,Citation43–48–Citation51–54–Citation56–58–Citation61–66–Citation77–91–Citation94–103–Citation108–116–Citation118,Citation119-121–Citation123 followed by 23% to safety and efficacy of the vaccine,Citation7–30–Citation34–41–Citation44,Citation45-49–Citation50-65–Citation67-69–Citation74-83–Citation85-87–Citation89-92–Citation93-113–Citation115-120–Citation124 20% to vaccination,Citation36–46–Citation52,Citation53-57–Citation59-76–Citation78-80–Citation81-86–Citation88-90–Citation96-97–Citation100-104–Citation111-125–Citation128 16% to risk factors associated with vaccination,Citation47,Citation60,Citation62,Citation72,Citation73,Citation75,Citation79,Citation82,Citation95,Citation98,Citation99,Citation101,Citation102,Citation106,Citation109,Citation110,Citation112,Citation114 and 11% of the published articles were focused on the issue of vaccine delivery.Citation55,Citation63,Citation64,Citation68,Citation71,Citation105,Citation107,Citation117
Table 7. Systematic analysis of the top 100 cited articles on mRNA.
The top 100 cited articles indicated that the monoclonal antibodies elicited by the mRNA-1273 vaccines potently neutralize SARS-CoV-2.Citation77,Citation91 Nucleoside-modified mRNA-LNPs elicit rapid and durable protective immunity against the Zika virus.Citation103 The human respiratory syncytial virus is a possible vector for protective antigens in another respiratory tract and might have utility in transient gene therapy of the respiratory tract.Citation35 LNP-formulated, modified mRNA vaccines can induce protective immunogenicity with acceptable tolerability profiles against the influenza virus.Citation118 Self-amplifying RNA is a promising platform for vaccines against viral diseases.Citation74 The treatment effect of autoimmune encephalomyelitis is associated with a reduction of effector T cells and the development of regulatory T cell (Treg cell) populations.Citation43 RNA tumor antigen vaccine strategy has potential applications for human cancer treatment and prevention.Citation47 The feasibility of a non-replicating mRNA rabies vaccine in small and large animals highlights the promise of mRNA vaccines for the prevention of infectious diseases.Citation98 In addition, mRNA/LNP is a versatile platform that enables the development of vaccination strategies that could prevent cytomegalovirus infection and consequent disease in different target populations.Citation102
The safety and efficacy of the mRNA vaccine showed that a two-dose regimen of BNT162b2 conferred 95% protection against COVID-19 in persons 16 years of age or older.Citation54,Citation66 While the mRNA-1273 vaccine showed 94.1% efficacy at preventing COVID-19 illness, including severe disease.Citation58 Women who are vaccinated with COVID-19 vaccine at later times during early pregnancy have less time during which they are at risk for pregnancy loss.Citation121 The antibody-mediated response to the BNT162b2 mRNA covid-19 vaccine in patients with CLL is markedly impaired and affected by disease activity and treatment.Citation31 A prophylactic mRNA-based candidate vaccine can induce functional antibodies against a viral antigen when administered with a needle-free device. The vaccine was generally safe with a reasonable tolerability profile.Citation7 The first mRNA vaccines against H10N8 and H7N9 influenza viruses were well tolerated and elicited robust humoral immune responses.Citation50 The self adjuvant RNActive® vaccine CV9103 was well tolerated and immunogenic.Citation96 The self incidence of myocarditis, although low, increased after the receipt of the BNT162b2 vaccine, particularly after the second dose among young male recipients.Citation86 Vaccination of guinea pigs induced EBOV-specific IgG and neutralizing antibody responses and 100% survival after EBOV infection.Citation129
The authorized mRNA COVID-19 vaccines effectively prevented SARS-CoV-2 infection, regardless of symptom status, among working-age adults in real-world conditions.Citation49,Citation94,Citation119 The establishment of autologous glioblastoma stem cells (GSCs) cultures under reasonable manufacturing procedures (GMP) is feasible, and the vaccination against GSCs is safe, well-tolerated, and may prolong recurrence-free survival.Citation67 A third dose of the BNT162b2 mRNA vaccine effectively protects individuals against severe COVID-19-related outcomes, compared with receiving only two doses at least 5 months ago.Citation85 Therapeutic vaccination with autologous TriMix-DC is feasible, safe, and immunogenic and can be combined with sequential IFN-a-2b.Citation90,Citation124 The COVID-19 vaccine was immunogenic in pregnant women, and the vaccine elicited antibodies that were transported to infant cord blood and breast milk.Citation44 The feasibility of vaccination with DCs loaded with mRNA encoding a defined antigen identified of immunogenic T-cell epitopes. This type of vaccination provides an opportunity for direct and fast discovery of novel T-cell epitopes from any tumor-specific or tumor-associated antigen.Citation110
The risk factors associated with mRNA vaccination were significantly lower and associated with previous infection, gender, age, ethnicity, job role, and Index of Multiple Deprivation score. BNT162b2 vaccine can prevent symptomatic and asymptomatic infection in working-age adults.Citation123 The potential for rare vaccine-related adverse events must be considered in the context of the well-established risk of morbidity, including cardiac injury, following COVID-19 infection.Citation60,Citation75,Citation87 Cladribine treatment does not impair the humoral response to COVID-19 vaccination.Citation93,Citation97 mRNA BNTb262 vaccine was immunogenic in the majority of patients with AIIRD, with an acceptable safety profile. Treatment with glucocorticoids, rituximab, MMF, and abatacept was associated with a significantly reduced BNT162b2-induced immunogenicity.Citation113 Most kidney transplant recipients remain at high risk for COVID-19 despite vaccination.Citation68,Citation72,Citation79,Citation115 The incidence of serious outcomes, including acute myocardial infection, Bell palsy, cerebral venous sinus thrombosis, Guillain-Barre syndrome, myocarditis/pericarditis, pulmonary embolism, stroke, and thrombosis with thrombocytopenia syndrome was not significantly higher at 1–21 days post-vaccination compared with 22–42 days post-vaccination.Citation88 Different strategies may need to be explored in patients with IMID taking methotrexate to increase the chances of immunization efficacy against SARS-CoV-2 as has been demonstrated for augmenting immunogenicity to other viral vaccines.Citation107
Among the potential non-viral vectors, lipid nanoparticles are particularly promising for vaccine delivery in cancer.Citation34 Direct injection of protamine-protected mRNA is feasible and safe.Citation41 Treatment with naked mRNA results in protein expression is feasible and safe. Furthermore, an increase in antitumor humoral immune response was seen in some patients.Citation83 Administration of globin UTR-stabilized mRNA is a versatile vaccination strategy that can be manipulated to fit the requirement of antiviral, antibacterial, or antitumor immunity.Citation46 The observed tumor immunity correlates with splenic antigen-specific CD81 T cells and is achieved only when mRNA is delivered in nanoparticles but not in naked format.Citation114
Discussion
The current study provided an overview of the top 100 cited articles on mRNA vaccine globally from documents indexed in the Scopus database for the first time. Scopus is one of the most appropriate online databases widely used for bibliometric analysis. The study revealed a clear interest in mRNA research productivity in the year 2021. This may be owed to the outbreak of COVID-19 pandemic. The study used R software to provide the researcher with mRNA vaccine author’s productivity by using Lotka’s Law as one of the classical laws of bibliometrics to examine the distribution of frequency of the researcher’s publications as well as reports in other scientific fields.Citation130 Thus, findings would be of interest to the scientific community to demonstrate the general applicability of this relation to authors in mRNA vaccine top cited articles and their author’s productivity in the subject.Citation70,Citation131
The study highlights that the top-rank paper was Safety and efficacy of the BNT162b2 mRNA COVID-19 vaccine, published in 2020 in the New England Journal of Medicine. The article indicated that a two-dose regimen of BNT162b2 conferred 95% protection against COVID-19 in persons 16 years of age or older. Due to the COVID-19 pandemic, this article became the most influential with 5757 citations.Citation54 Graham BS and Bennett H were considered as the most influential authors reported in the top 100 most cited mRNA vaccine. According to Lotka’s law, only 0.3% percent of the authors in a given field produced more than 5 articles.
The New England Journal of Medicine published the most mRNA vaccine papers. This journal is the most pioneer journal in medical research, which publishes high-quality research. mRNA vaccine research was conducted by the developed countries because they own the most potential funding agencies that fund scientific research. As previously reported, funding is one of the potential mechanisms to encourage and increase productivity.Citation18 Keyword analysis indicated that the top-cited articles on mRNA vaccine covered various key aspects, including COVID-19, vaccine, mRNA vaccine, mRNA, SARS-CoV-2, and immunogenicity, among others. Evidence from the network analysis reveals a significant level of collaboration among authors in the field, as shown in the clusters. The collaboration is vital as it enables the sharing of knowledge and findings of scientific research, such as genome sequences and the possible development of the mRNA vaccine.
The mRNA vaccine articles were classified into vaccine production and evaluation, safety and efficacy of the vaccine, vaccination, risk factors associated with vaccination and vaccine delivery system. Meanwhile, the most cited articles were focused on COVID-19, followed by cancer, influenza virus, COVID-19 and kidney diseases, COVID-19 and myocarditis, and rabies virus, among others.
This study has limitations that related to its theme’s diversity. The methodology always emphasizes the most statistically most relevant themes, but we do not represent all the themes of the research field, in addition the study only provides statistics based on the number of articles and highest citation, whereas the contribution of other excellence researchers in the field was not shown in the current analysis. We use only one database (Scopus); thus, there is the possibility of missing out on key top-cited articles that may be available in other databases such as Web of Sciences, PubMed, and Google Scholar. Therefore, the results of the current assessment might not be comprehensive. Furthermore, we only consider full research articles as inclusion criteria for the purpose of the analysis. Thus, with the inclusion of all documents in the research field, the results could be a little different. Future studies could discuss and improve the methodological strategy adopted and provide a comprehensive analysis to show the contribution of at least 100 most impact authors in the field. In addition, the presence of false-positive and false-negative results must be regarded as in any bibliometric study. Other limitations, were that we only included studies published in the English language and underestimated studies published in other languages, which may also have higher citation scores than the ones we extracted.
Above all these limitations, the results consolidate the recent knowledge on mRNA vaccines and will prove helpful for researchers to understand the top 100 most cited articles based on their themes and systematic analysis.
Conclusions
The study provides quantitative and qualitative analyses of the top 100 most cited articles on mRNA vaccine. The annual number of articles has steadily increased between 2020 and 2021. The comprehensive analysis also provides insights into scientific research productivity by authors, countries, and institutions, which will assist in generating evidence-based descriptions, comparisons, and mapping visualizations of research output in mRNA vaccines. In addition, the study provides a road map of the characteristics of the top 100 most cited articles and extensively describes their patterns of performance and the impact of mRNA vaccine research, core journals, and corresponding author’s countries, and other information that can be a useful and helpful tool for future studies.
Application and future research directions
The current analysis provides readers with applied evidence and gap maps on top 100 most cited articles on mRNA vaccine, providing a visual representation of the conceptual structure analysis of research based on the mapping analysis. Moreover, the study also provides a scientific relationship between researchers and countries toward research collaboration to enhance research on mRNA vaccines. Through a comprehensive analysis of co-authorships, our study provides an insight into partners in research endeavors and describes the scientific network. Furthermore, the qualitative analysis of the keywords helps to identify the current focus and future research direction within mRNA vaccine research. Through the thematic analysis, the current investigation was able to use conceptual structure analysis to describe patterns across qualitative data on the reported topic on specific themes (motor-themes, Niche themes, emerging topics or disappearing themes, and basic themes or basic and Transversal Themes) based on the Relevance Degree (Centrality) and Degree (Density) in the vertical and horizontal axis.
Data access
All data presented in this article can be retrieved from Scopus using keywords listed in the methodology.
Disclosure statement
No potential conflict of interest was reported by the author(s).
Additional information
Funding
References
- Musa IH, Afolabi LO, Zamit I, Musa TH, Musa HH, Tassang A, Akintunde TY, Li W. Artificial intelligence and machine learning in cancer research : a systematic and thematic analysis of the top 100 cited articles indexed in Scopus database. Cancer Control. 2022;29. doi:10.1177/10732748221095946.
- Biggs PM. Gordon memorial lecture. Vaccines and vaccination–past, present and future. Br Poult Sci. 1990;31:1–24. doi:10.1080/00071669008417226.
- Cai X, Li JJ, Liu T, Brian O, Li J. Infectious disease mRNA vaccines and a review on epitope prediction for vaccine design. Brief Funct Genomics. 2021;20:289–303. doi:10.1093/bfgp/elab027.
- Pardi N, Hogan MJ, Porter FW, Weissman D. mRNA vaccines - a new era in vaccinology. Nat Rev Drug Discov. 2018;17:261–79. doi:10.1038/nrd.2017.243.
- Bettini E, Locci M. SARS-CoV-2 mRNA Vaccines: immunological mechanism and beyond. Vaccines. 2021;9:147. doi:10.3390/vaccines9020147.
- Chaudhary N, Weissman D, Whitehead KA. mRNA vaccines for infectious diseases: principles, delivery and clinical translation. Nat Rev Drug Discov. 2021;20:817–38. doi:10.1038/s41573-021-00283-5.
- Alberer M, Gnad-Vogt U, Hong HS, Mehr KT, Backert L, Finak G, Gottardo R, Bica MA, Garofano A, Koch SD, et al. Safety and immunogenicity of a mRNA rabies vaccine in healthy adults: an open-label, non-randomised, prospective, first-in-human phase 1 clinical trial. Lancet (London, England). 2017;390:1511–20. doi:10.1016/S0140-6736(17)31665-3.
- Casadevall A. The mRNA vaccine revolution is the dividend from decades of basic science research. J Clin Invest. 2021:131. doi:10.1172/JCI153721.
- Freyn AW, Ramos da Silva J, Rosado VC, Bliss CM, Pine M, Mui BL, Tam YK, Madden TD, de Souza Ferreira LC, Weissman D, et al. A multi-targeting, nucleoside-modified mRNA influenza virus vaccine provides broad protection in mice. Mol Ther. 2020;28:1569–84. doi:10.1016/j.ymthe.2020.04.018.
- Wu K, Choi A, Koch M, Elbashir S, Ma L, Lee D, Woods A, Henry C, Palandjian C, Hill A, et al. Variant SARS-CoV-2 mRNA vaccines confer broad neutralization as primary or booster series in mice. Vaccine. 51;39: 7394–7400 . 2021.
- Sandbrink JB, Shattock RJ. RNA vaccines: a suitable platform for tackling emerging pandemics? Front Immunol. 2020;11:608460. doi:10.3389/fimmu.2020.608460.
- Akintunde TY, Musa TH, Musa HH, Musa IH, Shaojun C, Ibrahim E, Tassang AE, MSEDM H. Bibliometric analysis of global scientific literature on effects of COVID-19 pandemic on mental health. Asian J Psychiatr. 2021;63:102753. doi:10.1016/j.ajp.2021.102753.
- Musa IH, Musa TH, Zamit I, Okeke M. Artificial intelligence and machine learning in oncology: historical overview of documents indexed in the web of science database. Eurasian J Med Oncol. 2021;5:239–48. doi:10.14744/ejmo.2021.24856.
- Kawuki J, Ghimire U, Papabathini SS, Obore N, Musa TH. A bibliometric analysis of childhood obesity research from China indexed in Web of Science. J Public Heal Emerg. 2021;5:3. doi:10.21037/jphe-20-95.
- Musa TH, Ahmad T, Li W, Kawuki J, Wana MN, Musa HH, Wei P. A bibliometric analysis of global scientific research on Scrub Typhus. Biomed Res Int. 2020;2020:1–9. doi:10.1155/2020/5737893.
- Musa TH, Li W, Kawuki J, Wei P. The 100 top-cited articles on scrub typhus : a bibliometric analysis. Osong Public Health Res Perspect. 2021;12:126–35. doi:10.24171/j.phrp.2021.12.2.10.
- Akintunde TY, Musa TH, Musa HH, Ibrahim E, Muhideen S, Kawuki J. Mapping the global research output on Ebola vaccine from research indexed in web of science and Scopus : a comprehensive bibliometric analysis. Hum Vaccin Immunother. 2021;00:1–13. doi:10.1080/21645515.2021.1948785.
- Musa HH, El-Sharief M, Musa IH, Musa TH, Akintunde TY. Global scientific research output on sickle cell disease : a comprehensive bibliometric analysis of web of science publication. Sci African. 2021;12:e00774. doi:10.1016/j.sciaf.2021.e00774.
- Gatasi G, Musa TH, Odjidja EN. Bibliometric analysis of the top 100 cited articles on HIV/AIDS. Ann Infect. 2021;5:6. doi:10.21037/aoi-20-17.
- Akintunde TY, Chen S, Musa TH, Amoo FO, Adedeji A, Ibrahim E, Tassang AE, Musa IH, Musa HH. Tracking the progress in COVID-19 and vaccine safety research - a comprehensive bibliometric analysis of publications indexed in Scopus database. Hum Vaccin Immunother. 2021;17:3887–97. doi:10.1080/21645515.2021.1969851.
- Kawuki J, Yu X, Musa TH. Bibliometric analysis of Ebola research indexed in web of science and Scopus (2010-2020). Biomed Res Int. 2020;2020:1–12. doi:10.1155/2020/5476567.
- Musa TH, Akintunde TY, Musa HH, Ghimire U, Gatasi G. Malnutrition research output : a bibliometric analysis for articles index in web of science between 1900 and 2020. Electron J Gen Med. 2021;18:em293. doi:10.29333/ejgm/10840.
- Sharun K, Musa TH, Musa HH, Kumar R, Pawde AM, Chandra V, Tuli HS, Dhama K, Sharma GT. Mapping global trends in adipose-derived mesenchymal stem cell research: a bibliometric analysis using Scopus database. Ann Med Surg. 2022;77:103542. https://www.sciencedirect.com/science/article/pii/S2049080122003028.
- Musa TH, Akintunde TY, Musa IH, Mohammed LA, Tassang AE, Musa HH. Rift valley fever: thematic analysis of documents indexed in the web of science core collection database. Ann Infect. 2022;6:1. doi:10.21037/aoi-21-9.
- Kawuki J, Musa TH, Papabathini SS, Ghimire U, Obore N, Yu X. The 100 top-cited studies on Ebola: a bibliometric analysis. Electron J Gen Med. 2021;18:em276. doi:10.29333/ejgm/9694.
- Dervis H. Bibliometric analysis using bibliometrix an R package. J Scientometr Res. 2019;8:156–60. doi:10.5530/jscires.8.3.32.
- Van Eck NJ, Waltman L. Vosviewer Manual version 1.6.10. CWTS Meaningful Metrics . 2019;1–53.
- Chen C, Sanjuan FI, Hou J. The structure and dynamics of co ‐ citation clusters : a multiple ‐ perspective co ‐ citation analysis. J Am Soc Inf Science Technol. 2010;61(7):1386–409. doi:10.1002/asi.21309.
- Moral-Muñoz JA, Herrera-Viedma E, Santisteban-Espejo A, Cobo MJ. Software tools for conducting bibliometric analysis in science: an up-to-date review. El Profesional de la Información. 2020;29. doi:10.3145/epi.2020.ene.03.
- Polack FP, Thomas SJ, Kitchin N, Absalon J, Gurtman A, Lockhart S, Perez JL, Pérez Marc G, Moreira ED, Zerbini C, et al. Safety and efficacy of the BNT162b2 mRNA Covid-19 vaccine. N Engl J Med. 2020;383:2603–15. doi:10.1056/NEJMoa2034577.
- Baden LR, El Sahly HM, Essink B, Kotloff K, Frey S, Novak R, Diemert D, Spector SA, Rouphael N, Creech CB, et al. Efficacy and Safety of the mRNA-1273 SARS-CoV-2 Vaccine. N Engl J Med. 2021;384:403–16. doi:10.1056/NEJMoa2035389.
- Jackson LA, Anderson EJ, Rouphael NG, Roberts PC, Makhene M, Coler RN, McCullough MP, Chappell JD, Denison MR, Stevens LJ, et al. An mRNA Vaccine against SARS-CoV-2 — Preliminary Report. N Engl J Med. 2020;383:1920–31. doi:10.1056/NEJMoa2022483.
- Dagan N, Barda N, Kepten E, Miron O, Perchik S, Katz MA, Hernán MA, Lipsitch M, Reis B, Balicer RD. Bnt162b2 mRNA Covid-19 vaccine in a nationwide mass vaccination setting. N Engl J Med. 2021;384:1412–23. doi:10.1056/NEJMoa2101765.
- Anderson EJ, Rouphael NG, Widge AT, Jackson LA, Roberts PC, Makhene M, Chappell JD, Denison MR, Stevens LJ, Pruijssers AJ, et al. Safety and immunogenicity of SARS-CoV-2 mRNA-1273 vaccine in older adults. N Engl J Med. 2020;383:2427–38. doi:10.1056/NEJMoa2028436.
- Wang Z, Schmidt F, Weisblum Y, Muecksch F, Barnes CO, Finkin S, Schaefer-Babajew D, Cipolla M, Gaebler C, Lieberman JA, et al. mRNA vaccine-elicited antibodies to SARS-CoV-2 and circulating variants. Nature. 2021;592:616–22. doi:10.1038/s41586-021-03324-6.
- Haas EJ, Angulo FJ, McLaughlin JM, Anis E, Singer SR, Khan F, Brooks N, Smaja M, Mircus G, Pan K, et al. Impact and effectiveness of mRNA BNT162b2 vaccine against SARS-CoV-2 infections and COVID-19 cases, hospitalisations, and deaths following a nationwide vaccination campaign in Israel: an observational study using national surveillance data. Lancet (London, England). 2021;397:1819–29. doi:10.1016/S0140-6736(21)00947-8.
- Corbett KS, Flynn B, Foulds KE, Francica JR, Boyoglu-Barnum S, Werner AP, Flach B, O’Connell S, Bock KW, Minai M, et al. Evaluation of the mRNA-1273 vaccine against SARS-CoV-2 in nonhuman primates. N Engl J Med. 2020;383:1544–55. doi:10.1056/NEJMoa2024671.
- Corbett KS, Edwards DK, Leist SR, Abiona OM, Boyoglu-Barnum S, Gillespie RA, Himansu S, Schäfer A, Ziwawo CT, DiPiazza AT, et al. SARS-CoV-2 mRNA vaccine design enabled by prototype pathogen preparedness. Nature. 2020;586:567–71. doi:10.1038/s41586-020-2622-0.
- Pardi N, Hogan MJ, Pelc RS, Muramatsu H, Andersen H, DeMaso CR, Dowd KA, Sutherland LL, Scearce RM, Parks R, et al. Zika virus protection by a single low-dose nucleoside-modified mRNA vaccination. Nature. 2017;543:248–51. doi:10.1038/nature21428.
- Richner JM, Himansu S, Dowd KA, Butler SL, Salazar V, Fox JM, Julander JG, Tang WW, Shresta S, Pierson TC, et al. Modified mRNA vaccines protect against Zika virus infection. Cell. 2017;168:1114–25.e10. doi:10.1016/j.cell.2017.02.017.
- Tartof SY, Slezak JM, Fischer H, Hong V, Ackerson BK, Ranasinghe ON, Frankland TB, Ogun OA, Zamparo JM, Gray S, et al. Effectiveness of mRNA BNT162b2 COVID-19 vaccine up to 6 months in a large integrated health system in the USA: a retrospective cohort study. Lancet (London, England). 2021;398:1407–16. doi:10.1016/S0140-6736(21)02183-8.
- Collins PL, Hill MG, Camargo E, Grosfeld H, Chanock RM, Murphy BR. Production of infectious human respiratory syncytial virus from cloned cDNA confirms an essential role for the transcription elongation factor from the 5’ proximal open reading frame of the M2 mRNA in gene expression and provides a capability for vaccine. Proc Natl Acad Sci U S A. 1995;92:11563–67. doi:10.1073/pnas.92.25.11563.
- Collier DA, De Marco A, Ferreira IATM, Meng B, Datir RP, Walls AC, Kemp SA, Bassi J, Pinto D, Silacci-Fregni C, et al. Sensitivity of SARS-CoV-2 B.1.1.7 to mRNA vaccine-elicited antibodies. Nature. 2021;593:136–41. doi:10.1038/s41586-021-03412-7.
- Thomas SJ, Moreira EDJ, Kitchin N, Absalon J, Gurtman A, Lockhart S, Perez JL, Pérez Marc G, Polack FP, Zerbini C, et al. Safety and efficacy of the BNT162b2 mRNA Covid-19 vaccine through 6 months. N Engl J Med. 2021;385:1761–73. doi:10.1056/NEJMoa2110345.
- Shimabukuro TT, Kim SY, Myers TR, Moro PL, Oduyebo T, Panagiotakopoulos L, Marquez PL, Olson CK, Liu R, Chang KT, et al. Preliminary findings of mRNA Covid-19 vaccine safety in pregnant persons. N Engl J Med. 2021;384:2373–82. https://www.scopus.com/inward/record.uri?eid=2-s2.0-85106349611&doi=10.1056%2FNEJMoa2104983&partnerID=40&md5=b06b3188e964e1dc834988c23a9cc7db.
- Thompson MG, Burgess JL, Naleway AL, Tyner HL, Yoon SK, Meece J, Olsho LEW, Caban-Martinez AJ, Fowlkes A, Lutrick K, et al. Interim estimates of vaccine effectiveness of BNT162b2 and mRNA-1273 COVID-19 vaccines in preventing SARS-CoV-2 infection among health care personnel, first responders, and other essential and frontline workers - Eight U.S. Locations, December 2020-March. Morbid Mortality Weekly Rep. 2021;70:495–500. doi:10.15585/mmwr.mm7013e3.
- Hall VJ, Foulkes S, Saei A, Andrews N, Oguti B, Charlett A, Wellington E, Stowe J, Gillson N, Atti A, et al. COVID-19 vaccine coverage in health-care workers in England and effectiveness of BNT162b2 mRNA vaccine against infection (SIREN): a prospective, multicentre, cohort study. Lancet. 2021;397:1725–35. doi:10.1016/S0140-6736(21)00790-X.
- Bahl K, Senn JJ, Yuzhakov O, Bulychev A, Brito LA, Hassett KJ, Laska ME, Smith M, Almarsson Ö, Thompson J, et al. Preclinical and clinical demonstration of immunogenicity by mRNA vaccines against H10N8 and H7N9 influenza viruses. Mol Ther. 2017;25:1316–27. doi:10.1016/j.ymthe.2017.03.035.
- Herishanu Y, Avivi I, Aharon A, Shefer G, Levi S, Bronstein Y, Morales M, Ziv T, Shorer Arbel Y, Scarfò L, et al. Efficacy of the BNT162b2 mRNA COVID-19 vaccine in patients with chronic lymphocytic leukemia. Blood. 2021;137:3165–73. doi:10.1182/blood.2021011568.
- Barda N, Dagan N, Ben-Shlomo Y, Kepten E, Waxman J, Ohana R, Hernán MA, Lipsitch M, Kohane I, Netzer D, et al. Safety of the BNT162b2 mRNA Covid-19 vaccine in a nationwide setting. N Engl J Med. 2021;385:1078–90. doi:10.1056/NEJMoa2110475.
- Petsch B, Schnee M, Vogel AB, Lange E, Hoffmann B, Voss D, Schlake T, Thess A, Kallen K-J, Stitz L, et al. Protective efficacy of in vitro synthesized, specific mRNA vaccines against influenza a virus infection. Nat Biotechnol. 2012;30:1210–16. doi:10.1038/nbt.2436.
- Barda N, Dagan N, Cohen C, Hernán MA, Lipsitch M, Kohane IS, Reis BY, Balicer RD. Effectiveness of a third dose of the BNT162b2 mRNA COVID-19 vaccine for preventing severe outcomes in Israel: an observational study. Lancet (London, England). 2021;398:2093–100. doi:10.1016/S0140-6736(21)02249-2.
- Ebinger JE, Fert-Bober J, Printsev I, Wu M, Sun N, Prostko JC, Frias EC, Stewart JL, Van Eyk JE, Braun JG, et al. Antibody responses to the BNT162b2 mRNA vaccine in individuals previously infected with SARS-CoV-2. Nat Med. 2021;27:981–84. doi:10.1038/s41591-021-01325-6.
- Zhang N-N, X-F L, Deng Y-Q, Zhao H, Huang Y-J, Yang G, Huang W-J, Gao P, Zhou C, Zhang R-R, et al. A Thermostable mRNA Vaccine against COVID-19. Cell. 2020;182:1271–83.e16. doi:10.1016/j.cell.2020.07.024.
- Reichmuth AM, Oberli MA, Jeklenec A, Langer R, Blankschtein D. mRNA vaccine delivery using lipid nanoparticles. Ther Deliv. 2016;7:319–34. doi:10.4155/tde-2016-0006.
- Goel RR, Apostolidis SA, Painter MM, Mathew D, Pattekar A, Kuthuru O, Gouma S, Hicks P, Meng W, Rosenfeld AM, et al. Distinct antibody and memory B cell responses in SARSCoV-2 naïve and recovered individuals following mRNA vaccination. Sci Immunol. 2021;6:1–19. https://www.scopus.com/inward/record.uri?eid=2-s2.0-85104447408&doi=10.1126%2Fsciimmunol.abi6950&partnerID=40&md5=3f8771d9ed789e8df10d7e4d6e56492c.
- Weide B, Pascolo S, Scheel B, Derhovanessian E, Pflugfelder A, Eigentler TK, Pawelec G, Hoerr I, Rammensee H-G, Garbe C. Direct injection of protamine-protected mRNA: results of a phase 1/2 vaccination trial in metastatic melanoma patients. J Immunother (1991). 2009;32:498–507. doi:10.1097/CJI.0b013e3181a00068.
- Turner JS, O’Halloran JA, Kalaidina E, Kim W, Schmitz AJ, Zhou JQ, Lei T, Thapa M, Chen RE, Case JB, et al. SARS-CoV-2 mRNA vaccines induce persistent human germinal centre responses. Nature. 2021;596:109–13. doi:10.1038/s41586-021-03738-2.
- Stamatatos L, Czartoski J, Wan Y-H, Homad LJ, Rubin V, Glantz H, Neradilek M, Seydoux E, Jennewein MF, MacCamy AJ, et al. mRNA vaccination boosts cross-variant neutralizing antibodies elicited by SARS-CoV-2 infection. Science. 2021;372:1413–18. doi:10.1126/science.abg9175.
- Ryan M, Montgomery J, Engler R, Hoffman D, McClenathan B, Collins L, Loran D, Hrncir D, Herring K, Platzer M, et al. Myocarditis following immunization with mrna covid-19 vaccines in members of the us military. JAMA Cardiol. 2021;6:1202–06. doi:10.1001/jamacardio.2021.2833.
- Pardi N, Hogan MJ, Naradikian MS, Parkhouse K, Cain DW, Jones L, Moody MA, Verkerke HP, Myles A, Willis E, et al. Nucleoside-modified mRNA vaccines induce potent T follicular helper and germinal center B cell responses. J Exp Med. 2018;215:1571–88. doi:10.1084/jem.20171450.
- Furer V, Eviatar T, Zisman D, Peleg H, Paran D, Levartovsky D, Zisapel M, Elalouf O, Kaufman I, Meidan R, et al. Immunogenicity and safety of the BNT162b2 mRNA COVID-19 vaccine in adult patients with autoimmune inflammatory rheumatic diseases and in the general population: a multicentre study. Ann Rheum Dis. 2021;80:1330–38. doi:10.1136/annrheumdis-2021-220647.
- Hassett KJ, Benenato KE, Jacquinet E, Lee A, Woods A, Yuzhakov O, Himansu S, Deterling J, Geilich BM, Ketova T, et al. Optimization of lipid nanoparticles for intramuscular administration of mRNA vaccines. Mol Ther - Nucleic Acids. 2019;15:1–11. doi:10.1016/j.omtn.2019.01.013.
- Miao L, Li L, Huang Y, Delcassian D, Chahal J, Han J, Shi Y, Sadtler K, Gao W, Lin J, et al. Delivery of mRNA vaccines with heterocyclic lipids increases anti-tumor efficacy by STING-mediated immune cell activation. Nat Biotechnol. 2019;37:1174–85. doi:10.1038/s41587-019-0247-3.
- Feldman RA, Fuhr R, Smolenov I, Ribeiro AM, Panther L, Watson M, Senn JJ, Smith M, Almarsson Ӧ, Pujar HS, et al. mRNA vaccines against H10N8 and H7N9 influenza viruses of pandemic potential are immunogenic and well tolerated in healthy adults in phase 1 randomized clinical trials. Vaccine. 2019;37:3326–34. doi:10.1016/j.vaccine.2019.04.074.
- Vogel AB, Lambert L, Kinnear E, Busse D, Erbar S, Reuter KC, Wicke L, Perkovic M, Beissert T, Haas H, et al. Self-amplifying RNA vaccines give equivalent protection against influenza to mRNA vaccines but at much lower doses. Mol Ther. 2018;26:446–55. doi:10.1016/j.ymthe.2017.11.017.
- Geisen UM, Berner DK, Tran F, Sümbül M, Vullriede L, Ciripoi M, Reid HM, Schaffarzyk A, Longardt AC, Franzenburg J, et al. Immunogenicity and safety of anti-SARS-CoV-2 mRNA vaccines in patients with chronic inflammatory conditions and immunosuppressive therapy in a monocentric cohort. Ann Rheum Dis. 2021;80:1306–11. doi:10.1136/annrheumdis-2021-220272.
- Thompson MG, Burgess JL, Naleway AL, Tyner H, Yoon SK, Meece J, Olsho LEW, Caban-Martinez AJ, Fowlkes AL, Lutrick K, et al. Prevention and attenuation of Covid-19 with the BNT162b2 and mRNA-1273 vaccines. N Engl J Med. 2021;385:320–29. doi:10.1056/NEJMoa2107058.
- Mevorach D, Anis E, Cedar N, Bromberg M, Haas EJ, Nadir E, Olsha-Castell S, Arad D, Hasin T, Levi N, et al. Myocarditis after BNT162b2 mRNA vaccine against Covid-19 in Israel. N Engl J Med. 2021;385:2140–49. doi:10.1056/NEJMoa2109730.
- Vik-Mo EO, Nyakas M, Mikkelsen BV, Moe MC, Due-Tønnesen P, Suso EMI, Sæbøe-Larssen S, Sandberg C, Brinchmann JE, Helseth E, et al. Therapeutic vaccination against autologous cancer stem cells with mRNA-transfected dendritic cells in patients with glioblastoma. Cancer Immunol Immunother. 2013;62:1499–509. doi:10.1007/s00262-013-1453-3.
- Weide B, Carralot J-P, Reese A, Scheel B, Eigentler TK, Hoerr I, Rammensee H-G, Garbe C, Pascolo S. Results of the first phase I/II clinical vaccination trial with direct injection of mRNA. J Immunother (1991). 2008;31:180–88. doi:10.1097/CJI.0b013e31815ce501.
- Grupper A, Rabinowich L, Schwartz D, Schwartz IF, Ben-Yehoyada M, Shashar M, Katchman E, Halperin T, Turner D, Goykhman Y, et al. Reduced humoral response to mRNA SARS-CoV-2 BNT162b2 vaccine in kidney transplant recipients without prior exposure to the virus. Am J Transplant. 2021;21:2719–26. doi:10.1111/ajt.16615.
- Klein NP, Lewis N, Goddard K, Fireman B, Zerbo O, Hanson KE, Donahue JG, Kharbanda EO, Naleway A, Nelson JC, et al. Surveillance for adverse events after COVID-19 mRNA vaccination. JAMA. 2021;326:1390–99. doi:10.1001/jama.2021.15072.
- Liu X, Shaw RH, Stuart AS, Greenland M, Aley PK, Andrews NJ, Cameron JC, Charlton S, Clutterbuck EA, Collins AM, et al. Safety and immunogenicity of heterologous versus homologous prime-boost schedules with an adenoviral vectored and mRNA COVID-19 vaccine (Com-COV): a single-blind, randomised, non-inferiority trial. Lancet (London, England). 2021;398:856–69. doi:10.1016/S0140-6736(21)01694-9.
- Achiron A, Mandel M, Dreyer-Alster S, Harari G, Magalashvili D, Sonis P, Dolev M, Menascu S, Flechter S, Falb R, et al. Humoral immune response to COVID-19 mRNA vaccine in patients with multiple sclerosis treated with high-efficacy disease-modifying therapies. Ther Adv Neurol Disord. 2021;14. doi:10.1177/17562864211012835
- Goel RR, Painter MM, Apostolidis SA, Mathew D, Meng W, Rosenfeld AM, Lundgreen KA, Reynaldi A, Khoury DS, Pattekar A, et al. mRNA vaccines induce durable immune memory to SARS-CoV-2 and variants of concern. Science. 2021;374:abm0829. doi:10.1126/science.abm0829.
- Pegu A, O’Connell SE, Schmidt SD, O’Dell S, Talana CA, Lai L, Albert J, Anderson E, Bennett H, Corbett KS, et al. Durability of mRNA-1273 vaccine-induced antibodies against SARS-CoV-2 variants. Science. 2021;373:1372–77. doi:10.1126/science.abj4176.
- Liu L, Wang Y, Miao L, Liu Q, Musetti S, Li J, Huang L. Combination immunotherapy of MUC1 mRNA Nano-vaccine and CTLA-4 blockade effectively inhibits growth of triple negative breast cancer. Mol Ther. 2018;26:45–55. doi:10.1016/j.ymthe.2017.10.020.
- Apostolidis SA, Kakara M, Painter MM, Goel RR, Mathew D, Lenzi K, Rezk A, Patterson KR, Espinoza DA, Kadri JC, et al. Cellular and humoral immune responses following SARS-CoV-2 mRNA vaccination in patients with multiple sclerosis on anti-CD20 therapy. Nat Med. 2021;27:1990–2001. doi:10.1038/s41591-021-01507-2.
- Collier A-R, McMahan K, Yu J, Tostanoski LH, Aguayo R, Ansel J, Chandrashekar A, Patel S, Apraku Bondzie E, Sellers D, et al. Immunogenicity of COVID-19 mRNA vaccines in pregnant and lactating women. J Am Med Assoc. 2021;325:2370–80. doi:10.1001/jama.2021.7563.
- Pollard C, Rejman J, De Haes W, Verrier B, Van Gulck E, Naessens T, De Smedt S, Bogaert P, Grooten J, Vanham G, et al. Type I IFN counteracts the induction of antigen-specific immune responses by lipid-based delivery of mRNA vaccines. Mol Ther. 2013;21:251–59. doi:10.1038/mt.2012.202.
- Abu Jabal K, Ben-Amram H, Beiruti K, Batheesh Y, Sussan C, Zarka S, Edelstein M. Impact of age, ethnicity, sex and prior infection status on immunogenicity following a single dose of the BNT162b2 mRNA COVID-19 vaccine: real-world evidence from healthcare workers, Israel, December 2020 to January 2021. Eurosurveillance. 2021;26:2100096. doi:10.2807/1560-7917.ES.2021.26.6.2100096.
- Chemaitelly H, Yassine HM, Benslimane FM, Al Khatib HA, Tang P, Hasan MR, Malek JA, Coyle P, Ayoub HH, Al Kanaani Z, et al. mRNA-1273 COVID-19 vaccine effectiveness against the B.1.1.7 and B.1.351 variants and severe COVID-19 disease in Qatar. Nat Med. 2021;27:1614–21. doi:10.1038/s41591-021-01446-y.
- Kübler H, Scheel B, Gnad-Vogt U, Miller K, Schultze-Seemann W, Vom Dorp F, Parmiani G, Hampel C, Wedel S, Trojan L, et al. Self-adjuvanted mRNA vaccination in advanced prostate cancer patients: a first-in-man phase I/IIa study. J Immunother Cancer. 2015;3:26. doi:10.1186/s40425-015-0068-y.
- El Sahly HM, Baden LR, Essink B, Doblecki-Lewis S, Martin JM, Anderson EJ, Campbell TB, Clark J, Jackson LA, Fichtenbaum CJ, et al. Efficacy of the mRNA-1273 SARS-CoV-2 vaccine at completion of blinded phase. N Engl J Med. 2021;385:1774–85. doi:10.1056/NEJMoa2113017.
- Watad A, De Marco G, Mahajna H, Druyan A, Eltity M, Hijazi N, Haddad A, Elias M, Zisman D, Naffaa ME, et al. Immune-mediated disease flares or new-onset disease in 27 subjects following mRNA/DNA SARS-CoV-2 vaccination. Vaccines. 2021;9:435. doi:10.3390/vaccines9050435.
- Laczkó D, Hogan MJ, Toulmin SA, Hicks P, Lederer K, Gaudette BT, Castaño D, Amanat F, Muramatsu H, Oguin TH, et al. A single immunization with nucleoside-modified mRNA vaccines elicits strong cellular and humoral immune responses against SARS-CoV-2 in mice. Immunity. 2020;53:724–32.e7. doi:10.1016/j.immuni.2020.07.019.
- Schmidt T, Klemis V, Schub D, Mihm J, Hielscher F, Marx S, Abu-Omar A, Ziegler L, Guckelmus C, Urschel R, et al. Immunogenicity and reactogenicity of heterologous ChAdox1 nCov-19/mrna vaccination. Nat Med. 2021;27:1530–35. doi:10.1038/s41591-021-01464-w.
- Liang F, Lindgren G, Lin A, Thompson EA, Ols S, Röhss J, John S, Hassett K, Yuzhakov O, Bahl K, et al. Efficient targeting and activation of antigen-presenting cells in vivo after modified mRNA vaccine administration in Rhesus Macaques. Mol Ther. 2017;25:2635–47. doi:10.1016/j.ymthe.2017.08.006.
- Bonehill A, Van Nuffel AMT, Corthals J, Tuyaerts S, Heirman C, François V, Colau D, van der Bruggen P, Neyns B, Thielemans K. Single-step antigen loading and activation of dendritic cells by mRNA electroporation for the purpose of therapeutic vaccination in melanoma patients. Clin Cancer Res. 2009;15:3366–75. doi:10.1158/1078-0432.CCR-08-2982.
- Lederer K, Castaño D, Gómez Atria D, Oguin TH, Wang S, Manzoni TB, Muramatsu H, Hogan MJ, Amanat F, Cherubin P, et al. SARS-CoV-2 mRNA vaccines foster potent antigen-specific germinal center responses associated with neutralizing antibody generation. Immunity. 2020;53:1281–95.e5. doi:10.1016/j.immuni.2020.11.009.
- Tang P, Hasan MR, Chemaitelly H, Yassine HM, Benslimane FM, Al Khatib HA, AlMukdad S, Coyle P, Ayoub HH, Al Kanaani Z, et al. Bnt162b2 and mRNA-1273 COVID-19 vaccine effectiveness against the SARS-CoV-2 Delta variant in Qatar. Nat Med. 2021;27:2136–43. doi:10.1038/s41591-021-01583-4.
- Choi A, Koch M, Wu K, Chu L, Ma L, Hill A, Nunna N, Huang W, Oestreicher J, Colpitts T, et al. Safety and immunogenicity of SARS-CoV-2 variant mRNA vaccine boosters in healthy adults: an interim analysis. Nat Med. 2021;27:2025–31. doi:10.1038/s41591-021-01527-y.
- Jalkanen P, Kolehmainen P, Häkkinen HK, Huttunen M, Tähtinen PA, Lundberg R, Maljanen S, Reinholm A, Tauriainen S, Pakkanen SH, et al. COVID-19 mRNA vaccine induced antibody responses against three SARS-CoV-2 variants. Nat Commun. 2021;12. doi:10.1038/s41467-021-24285-4.
- Stumpf J, Siepmann T, Lindner T, Karger C, Schwöbel J, Anders L, Faulhaber-Walter R, Schewe J, Martin H, Schirutschke H, et al. Humoral and cellular immunity to SARS-CoV-2 vaccination in renal transplant versus dialysis patients: a prospective, multicenter observational study using mRNA-1273 or BNT162b2 mRNA vaccine. Lancet Reg Heal - Eur. 2021;9:100178. doi:10.1016/j.lanepe.2021.100178.
- Painter MM, Mathew D, Goel RR, Apostolidis SA, Pattekar A, Kuthuru O, Baxter AE, Herati RS, Oldridge DA, Gouma S, et al. Rapid induction of antigen-specific CD4(+) T cells is associated with coordinated humoral and cellular immunity to SARS-CoV-2 mRNA vaccination. Immunity. 2021;54:2133–42.e3. doi:10.1016/j.immuni.2021.08.001.
- Tenforde MW, Self WH, Adams K, Gaglani M, Ginde AA, McNeal T, Ghamande S, Douin DJ, Talbot HK, Casey JD, et al. Association between mRNA vaccination and COVID-19 hospitalization and disease severity. JAMA. 2021;326:2043–54. doi:10.1001/jama.2021.19499.
- Crommelin DJA, Anchordoquy TJ, Volkin DB, Jiskoot W, Mastrobattista E. Addressing the cold reality of mRNA vaccine stability. J Pharm Sci. 2021;110:997–1001. doi:10.1016/j.xphs.2020.12.006.
- Kim HW, Jenista ER, Wendell DC, Azevedo CF, Campbell MJ, Darty SN, Parker MA, Kim RJ. Patients with acute myocarditis following mRNA COVID-19 vaccination. JAMA Cardiol. 2021;6:1196–201. doi:10.1001/jamacardio.2021.2828.
- Wilgenhof S, Van Nuffel AMT, Corthals J, Heirman C, Tuyaerts S, Benteyn D, De Coninck A, Van Riet I, Verfaillie G, Vandeloo J, et al. Therapeutic vaccination with an autologous mRNA electroporated dendritic cell vaccine in patients with advanced melanoma. J Immunother (1991). 2011;34:448–56. doi:10.1097/CJI.0b013e31821dcb31.
- Cucchiari D, Egri N, Bodro M, Herrera S, Del Risco-Zevallos J, Casals-Urquiza J, Cofan F, Moreno A, Rovira J, Banon-Maneus E, et al. Cellular and humoral response after MRNA-1273 SARS-CoV-2 vaccine in kidney transplant recipients. Am J Transplant. 2021;21:2727–39. doi:10.1111/ajt.16701.
- Abu Mouch S, Roguin A, Hellou E, Ishai A, Shoshan U, Mahamid L, Zoabi M, Aisman M, Goldschmid N, Berar Yanay N. Myocarditis following COVID-19 mRNA vaccination. Vaccine. 2021;39:3790–93. doi:10.1016/j.vaccine.2021.05.087.
- Krienke C, Kolb L, Diken E, Streuber M, Kirchhoff S, Bukur T, Akilli-Öztürk Ö, Kranz LM, Berger H, Petschenka J, et al. A noninflammatory mRNA vaccine for treatment of experimental autoimmune encephalomyelitis. Science. 2021;371:145–53. doi:10.1126/science.aay3638.
- Maneikis K, Šablauskas K, Ringelevičiūtė U, Vaitekėnaitė V, Čekauskienė R, Kryžauskaitė L, Naumovas D, Banys V, Pečeliūnas V, Beinortas T, et al. Immunogenicity of the BNT162b2 COVID-19 mRNA vaccine and early clinical outcomes in patients with haematological malignancies in Lithuania: a national prospective cohort study. Lancet Haematol. 2021;8:e583–92. doi:10.1016/S2352-3026(21)00169-1.
- Carralot J-P, Probst J, Hoerr I, Scheel B, Teufel R, Jung G, Rammensee H-G, Pascolo S. Polarization of immunity induced by direct injection of naked sequence-stabilized mRNA vaccines. Cell Mol Life Sci. 2004;61:2418–24. doi:10.1007/s00018-004-4255-0.
- Verbeke R, Lentacker I, De Smedt SC, Dewitte H. The dawn of mRNA vaccines: the COVID-19 case. J Control Release. 2021;333:511–20. doi:10.1016/j.jconrel.2021.03.043.
- Mockey M, Bourseau E, Chandrashekhar V, Chaudhuri A, Lafosse S, Le Cam E, Quesniaux VFJ, Ryffel B, Pichon C, Midoux P. mRNA-based cancer vaccine: prevention of B16 melanoma progression and metastasis by systemic injection of MART1 mRNA histidylated lipopolyplexes. Cancer Gene Ther. 2007;14:802–14. doi:10.1038/sj.cgt.7701072.
- Lutz J, Lazzaro S, Habbeddine M, Schmidt KE, Baumhof P, Mui BL, Tam YK, Madden TD, Hope MJ, Heidenreich R, et al. Unmodified mRNA in LNPs constitutes a competitive technology for prophylactic vaccines. NPJ Vaccines. 2017;2:29. doi:10.1038/s41541-017-0032-6.
- Haberman RH, Herati R, Simon D, Samanovic M, Blank RB, Tuen M, Koralov SB, Atreya R, Tascilar K, Allen JR, et al. Methotrexate hampers immunogenicity to BNT162b2 mRNA COVID-19 vaccine in immune-mediated inflammatory disease. Ann Rheum Dis. 2021;80:1339–44. doi:10.1136/annrheumdis-2021-220597.
- Deepak P, Kim W, Paley MA, Yang M, Carvidi AB, Demissie EG, El-Qunni AA, Haile A, Huang K, Kinnett B, et al. Effect of immunosuppression on the immunogenicity of mRNA vaccines to SARS-CoV-2 : a prospective cohort study. Ann Intern Med. 2021;174:1572–85. doi:10.7326/M21-1757.
- Pascolo S. Vaccination with messenger RNA (mRNA). Handb Exp Pharmacol. 2008;183:221–35.
- Kadali RAK, Janagama R, Peruru S, Malayala SV. Side effects of BNT162b2 mRNA COVID-19 vaccine: a randomized, cross-sectional study with detailed self-reported symptoms from healthcare workers. Int J Infect Dis. 2021;106:376–81. doi:10.1016/j.ijid.2021.04.047.
- Ali K, Berman G, Zhou H, Deng W, Faughnan V, Coronado-Voges M, Ding B, Dooley J, Girard B, Hillebrand W, et al. Evaluation of mRNA-1273 SARS-CoV-2 Vaccine in Adolescents. N Engl J Med. 2021;385:2241–51. doi:10.1056/NEJMoa2109522.
- Rozen-Zvi B, Yahav D, Agur T, Zingerman B, Ben-Zvi H, Atamna A, Tau N, Mashraki T, Nesher E, Rahamimov R. Antibody response to SARS-CoV-2 mRNA vaccine among kidney transplant recipients: a prospective cohort study. Clin Microbiol Infect. 2021;27:e1173.1–34. doi:10.1016/j.cmi.2021.04.028.
- Brazzoli M, Magini D, Bonci A, Buccato S, Giovani C, Kratzer R, Zurli V, Mangiavacchi S, Casini D, Brito LM, et al. Induction of broad-based immunity and protective efficacy by self-amplifying mRNA vaccines encoding influenza virus hemagglutinin. J Virol. 2016;90:332–44. doi:10.1128/JVI.01786-15.
- Hobo W, Novobrantseva TI, Fredrix H, Wong J, Milstein S, Epstein-Barash H, Liu J, Schaap N, van der Voort R, Dolstra H. Improving dendritic cell vaccine immunogenicity by silencing PD-1 ligands using siRNA-lipid nanoparticles combined with antigen mRNA electroporation. Cancer Immunol Immunother. 2013;62:285–97. doi:10.1007/s00262-012-1334-1.
- Li M, Zhao M, Fu Y, Li Y, Gong T, Zhang Z, Sun X. Enhanced intranasal delivery of mRNA vaccine by overcoming the nasal epithelial barrier via intra- a nd paracellular pathways. J Control Release. 2016;228:9–19. doi:10.1016/j.jconrel.2016.02.043.
- Schnee M, Vogel AB, Voss D, Petsch B, Baumhof P, Kramps T, Stitz L. An mRNA vaccine encoding rabies virus glycoprotein induces protection against lethal infection in mice and correlates of protection in adult and newborn pigs. PLoS Negl Trop Dis. 2016;10:e0004746. doi:10.1371/journal.pntd.0004746.
- Oberhardt V, Luxenburger H, Kemming J, Schulien I, Ciminski K, Giese S, Csernalabics B, Lang-Meli J, Janowska I, Staniek J, et al. Rapid and stable mobilization of CD8+ T cells by SARS-CoV-2 mRNA vaccine. Nature. 2021;597:268–73. doi:10.1038/s41586-021-03841-4.
- Chu L, McPhee R, Huang W, Bennett H, Pajon R, Nestorova B, Leav B. A preliminary report of a randomized controlled phase 2 trial of the safety and immunogenicity of mRNA-1273 SARS-CoV-2 vaccine. Vaccine. 2021;39:2791–99. doi:10.1016/j.vaccine.2021.02.007.
- Zhou WZ, Hoon DS, Huang SK, Fujii S, Hashimoto K, Morishita R, Kaneda Y. RNA melanoma vaccine: induction of antitumor immunity by human glycoprotein 100 mRNA immunization. Hum Gene Ther. 1999;10:2719–24. doi:10.1089/10430349950016762.
- John S, Yuzhakov O, Woods A, Deterling J, Hassett K, Shaw CA, Ciaramella G. Multi-antigenic human cytomegalovirus mRNA vaccines that elicit potent humoral and cell-mediated immunity. Vaccine. 2018;36:1689–99. doi:10.1016/j.vaccine.2018.01.029.
- Brito LA, Kommareddy S, Maione D, Uematsu Y, Giovani C, Berlanda Scorza F, Otten GR, Yu D, Mandl CW, Mason PW, et al. Self-Amplifying mRNA Vaccines. Adv Genet. 2015;89:179–233.
- Chung H, He S, Nasreen S, Sundaram ME, Buchan SA, Wilson SE, Chen B, Calzavara A, Fell DB, Austin PC, et al. Effectiveness of BNT162b2 and mRNA-1273 covid-19 vaccines against symptomatic SARS-CoV-2 infection and severe covid-19 outcomes in Ontario, Canada: test negative design study. BMJ. 2021;374:n1943.
- Moor MB, Suter-Riniker F, Horn MP, Aeberli D, Amsler J, Möller B, Njue LM, Medri C, Angelillo-Scherrer A, Borradori L, et al. Humoral and cellular responses to mRNA vaccines against SARS-CoV-2 in patients with a history of CD20 B-cell-depleting therapy (RituxiVac): an investigator-initiated, single-centre, open-label study. Lancet Rheumatol. 2021;3:e789–97. doi:10.1016/S2665-9913(21)00251-4.
- Sormani MP, Inglese M, Schiavetti I, Carmisciano L, Laroni A, Lapucci C, Da Rin G, Serrati C, Gandoglia I, Tassinari T, et al. Effect of SARS-CoV-2 mRNA vaccination in MS patients treated with disease modifying therapies. EBioMedicine. 2021;72:103581. doi:10.1016/j.ebiom.2021.103581.
- Amanat F, Thapa M, Lei T, Ahmed SMS, Adelsberg DC, Carreño JM, Strohmeier S, Schmitz AJ, Zafar S, Zhou JQ, et al. SARS-CoV-2 mRNA vaccination induces functionally diverse antibodies to NTD, RBD, and S2. Cell. 2021;184:3936–48.e10. doi:10.1016/j.cell.2021.06.005.
- Shebl FM, Pinto LA, García-Piñeres A, Lempicki R, Williams M, Harro C, Hildesheim A. Comparison of mRNA and protein measures of cytokines following vaccination with human papillomavirus-16 L1 virus-like particles. Cancer Epidemiol Biomarkers Prevent. 2010;19:978–81. doi:10.1158/1055-9965.EPI-10-0064.
- Friedman A. The power of Lotka’ s Law through the eyes of R Romanian Statistical Review. 2015;63: 69–77.
- Pao ML. Lotka’s law: a testing procedure. Inf Process Manag. 1985;21:305–20. https://www.sciencedirect.com/science/article/pii/030645738590055X.
- Sen BK. Lotka’s Law: a viewpoint. Ann Libr Inf Stud. 2010;57:166–68.