ABSTRACT
Melanoma stem cells (MSCs)-based vaccine strategies have been a potent immunotherapeutic approach for melanoma treatment, which aimed at inducing specific anti-tumor immunity and targeting cancer stem-like cells. As the main cancer-fighting immune cells, CD8+T cells play an important role in vaccine-induced antitumor immunity. Here, we developed a novel MSC vaccine that induces CD8+T cells to target melanoma stem cells specifically. The MSC vaccine was prepared for our study in order to determine the effectiveness of antitumor immunity. The proportion and activity of CD8+T cells were examined in the spleen after immunization, in particular, the expression and cytotoxicity of the immune checkpoint of spleen lymphocytes were detected by flow cytometry and ELISA, moreover, tumor size and the number of lung metastasis nodules were observed and the specific killing effect of the vaccine was evaluated in immunized mice. We found that the MSC vaccine could promote DCs maturation, activate CD8+T cells, suppress the expression of CTLA-4, PD-1, and Tim-3, and increase the expression of IFN-γ and GzmB of CD8+T cells. Melanoma growth and metastasis were inhibited by the vaccine’s specific targeted killing effect. The vaccines based on melanoma stem cells (MSCs) delay the progression of melanoma by inducing anti-tumor immune responses in CD8+T cells.
Introduction
Melanoma is one of the most sensitive tumors to immune modulation.Citation1,Citation2 In the past decade, immunotherapy has greatly changed the treatment prospects of melanoma.Citation1,Citation3 In particular, immune checkpoint inhibitors (ICIs) targeting cytotoxic T-lymphocyte antigen-4 (CTLA-4) and programmed cell death protein-1 (PD-1) have achieved apparent clinical effects.Citation1,Citation4,Citation5 As an important branch in the field of immunotherapy, vaccines have been rarely studied in melanoma. Gp100 peptide vaccines could produce high levels of circulating T cells in vitro, recognize and kill melanoma cells, but the clinical efficacy was still not ideal.Citation6 Therefore, effective tumor vaccines can be an important supplement in the aspect of melanoma immunotherapy.
Since the discovery of melanoma stem cells (MSCs), it has become more clear how normal melanocytes may become malignant and acquire tumorigenic properties.Citation7–9 It has been suggested that targeted MSC therapy could inhibit tumor growth and reduce melanoma recurrence and metastasis risk.Citation10–12 Therefore, tumor vaccine targeting MSCs will improve the efficacy of current immunotherapy. At present, the research on MSC vaccine in melanoma was mostly to induce protective anti-tumor immunity by sensitizing dendritic cells (DCs) with MSCs.Citation12–14 DCs play an essential role in regulating immune responses and are pivotal mediators of innate and adaptive immunity.Citation15 To treat cancer effectively, mature DCs are required to activate CD8+T cells.Citation16 A recent study has found that CD8+T cells are capable of simultaneously secreting two cytokines, IFN-γ and TNF-α, could induce long-term antitumor immunity.Citation17 Furthermore, MSC vaccine may also elicit innate immune response mediated by CD8+T cells activation.
In addition, immune adjuvants could enhance the anti-tumor immune response of MSC vaccine.Citation18,Citation19 For instance, immune adjuvant IL-21 gene-modified MSCs enhanced complement-dependent cytotoxic activity and natural killer (NK) cells’ cytotoxic activity in mice.Citation20 The pleiotropic immune effector IL-33 plays a substantial role in activating anti-tumor immunity, including stimulating the maturation of DCs, as well as enhancing the killing effect of CD8+T cells and NK cells.Citation21,Citation22 An effective immune adjuvant, IL-33 stimulates antigen-specific effector T cells and memory T cells in vivo,Citation18,Citation23,Citation24 and inhibits tumor growth and lung metastasis in mice.Citation25,Citation26
In this study, we assessed the effect of IL-33 modified MSC vaccine on CD8+T cells, mainly in terms of proliferation, killing, immune checkpoints, and memory phenotype. These factors are strongly related to antitumor immune response. Thus, the inhibition of melanoma growth and metastasis was discovered through direct and indirect improvements in CD8+T cells.
Methods
Cell lines
B16F10 murine melanoma cell line was syngeneic with C57BL/6 mice. These cells were ordered from the iCell Bioscience of China in Shanghai and were cultured at 37°C in 5% CO2 atmosphere in complete media consisting of RPMI 1640 and 10% fetal bovine serum.
Mice
C57BL/6 mice of 5–6 weeks of age were ordered from the Liaoning Changsheng animal experimental center in China. All mice were housed under the pathogen-free condition. All the experiments were performed in compliance with the guidelines of the Animal Research Ethics Board of Jilin University.
Isolation of B16F10-CD133+CD44+ cells and preparation of MSC vaccine
The CD44+CD133+ double-positive cells were isolated from B16F10 cells line with immunomagnetic bead technology (eBioscience Company, USA) using the CD44 and CD133 monoclonal antibodies (eBioscience Company, USA) following the manufacturer’s instructions. The isolated CD44+CD133+ double-positive cells were labeled ‘B16F10-CD44+CD133+’ cells. And then, B16F10-CD44+CD133+ cells were infected by IL-33 overexpression gene or enhanced green fluorescent protein (EGFP) overexpression control adenovirus. Followed by mitomycin C (MMC) 50 μg/ml was used to inactivate the cells. Finally, we could get B16F10-CD44+CD133+ cells, which inhibited cell proliferation but still secreted IL-33. As a result of this method, we can prepare melanoma stem cell vaccine (vaccine group) and inactivate other control cells as well.
Co-culture of vaccine stimulated DC and splenic lymphocytes
DCs were generated from the mice bone marrow (BM). The end of the disinfected femurs and tibias were cut and flushed with RIPM 1640. The BM cells were cultured in RIPM 1640 supplemented with 10% FBS, 20 ng/ml granulocyte macrophage colony-stimulating factor (GM-CSF), and 10 ng/ml IL-4 (Peprotech, American) and divided equally in 6-well plates. On days 2 and 4 of the culture, half volume of culture medium was changed for a fresh one. Immature DCs were derived from the day 6 culture.
Different vaccine groups were used to stimulate the immature DCs. Briefly, vaccines in the same quantity and volume and DCs were directly cocultured in triplicate with 50,000 cells per well in a 6-well plate at a ratio of 1:1. Subsequently, we used 0.4-mm pore transwell inserts (Corning Incorporated, Corning, NY) to create an indirect, bilayer co-culture system allowing for exchange of soluble factors but not cells. Splenic lymphocytes from normal mice were seeded at a density of 10,000 cells in the insert. Splenic lymphocytes without co-culture were used as a blank control (named Tc). After 6 days of co-culture, the supernatant of each group was obtained. IFN-γ and TNF-α in the supernatant were determined by enzyme-linked immunosorbent assay (ELISA).
Immunization protocol
The 100 mice (female, weight: 18–20 g and age between 6 and 7 weeks) were randomly divided into five groups of equal size (20 per group): the B16F10-IL-33-CD44+CD133+(Vac-IL-33), the B16F10-EGFP-CD44+CD133+ (Vac-EGFP), B16F10-CD44+CD133+(Vac), the B16F10 wild-type cells group, and PBS group. The mice received subcutaneous vaccination in the right groin with 5 × 105 different cell vaccines inactivated with MMC (50 μg/ml) for 4 h, a total of three times immunizations with an interval of 7 days between the immunizations. Fifteen vaccinated mice were challenged subcutaneously with 1 × 105 B16F10 cells 7 days after final vaccination, and five vaccinated mice were challenged intravenously with 1 × 105 B16F10 cells. Tumor generation in each mouse was monitored every 2 days by taking 2-dimensional measurements of individual tumors. The pulmonary metastatic nodules were observed weekly after 3 weeks for the last immunization.
Flow cytometry analysis
The mouse spleen lymphocytes were isolated from different groups using mouse lymphocyte separation medium (DAKEWE, China) following the manufacturer’s instructions after 3 weeks for the last immunization. Immunofluorescence surface staining was performed as previously described.Citation27 The FITC-labeled mAbs against CD8, CD44, CD133, and PE-labeled CTLA-4 were purchased from Biolegend, APC-labeled CD69, PD-1, Tim-3 Ab were purchased from BD Biosciences.
Intracellular staining was performed as previously described.Citation27 PE-conjugated or Pacific Blue-conjugated mAbs against IFN-γ and granzyme B (GzmB) were purchased from Biolegend. After staining, cell samples were analyzed using a Beckman CytoFLEX Flow cytometry (Germany).
Cytotoxicity assay
The mouse spleen lymphocytes were isolated from different groups after 3 weeks for the last immunization. After counting, 1 × 107 splenic lymphocytes were taken from each group as effector cells, B16F10, were labeled with Calcein-AM (Biolegend, USA), and were used as target cell. Effector cells were co-cultured with target cells at different ratios (200:1, 100:1, and 50:1) for 8 h. To examine the specific cytotoxicity of the splenic lymphocytes, the spleen lymphocytes of mice in the vaccine group were used as effector cells, B16F10, B16F10-CD44-CD133- and B16F10-CD44+CD133+ were taken as target cells. Then, the cytotoxicity effect of splenic lymphocytes on B16F10 was determined by Calcein AM release assay.
Preparation of single cell from mouse tumor mass
In the tumor-bearing mice model, tumor cells were collected from different groups after 3 weeks for the last immunization. Briefly, the melanoma tissues were removed from the tumor-bearing mice under aseptic conditions and washed with sterile PBS. Then, the tumor tissue was cut into a paste by scissors and digested into single cells with 0.25% trypsin for 60 min. The single-cell suspension was obtained after filtration with a filter. Finally, the expression of CD44 and CD133 in these was determined by flow cytometry with monoclonal antibodies (eBioscience Company, USA).
ELISA for cytokines
+The IFN-γ and TNF-α concentrations in culture supernatants from direct co-culture of DCs and mouse spleen lymphocytes were detected by ELISA assay using ELISA kit (mlbio, China) following the manufacturer’s instructions. In addition, the serum of mice in different groups was collected after 3 weeks for the last immunization. The IFN-α, IL-2, TNF-α, and IFN-γ in the serum of mice were detected by ELISA assay.
Histopathological and immunohistochemical assays
+Formalin-fixed tumor tissues were embedded in paraffin and sliced into 5 μm sections. The sections were stained with H&E and antibody. The sections were incubated with the mouse anti-Ki-67 and caspase-3 (1:500, Abcam) at room temperature for 1 h. Subsequently, the sections were washed 3 times in PBS and incubated for 30 min at room temperature with goat anti-mouse IgG (1:10000, Abcam). The sections were examined by light-microscopy at × 400 magnification.
Statistical analysis
Numerical data in this study were reported using means ± standard deviation (SD). Data analyses were performed using paired t-tests with the GraphPad Prism 8 software. Statistical differences were assessed at P < .05, P < .01, and P < .001.
Results
MSC vaccine induces antitumor immune responses in mice
Previous studies have demonstrated that CD44 and CD133 are stem cell markers.Citation28 CD44+CD133+ stem cells as MSC were isolated from melanoma using immunomagnetic beads. Flow analysis showed that >95% of cells expressed CD44 and CD133 after sorting (Figure S1A). MSC using a lentiviral approach elicited an overexpression of IL-33. Immunofluorescence staining was used to confirm an increase in IL-33 expression after transfection (Figure S1B). Compared with the other groups, serum IL-33 level of mice immunized with MMC-inactivated MSCs was markedly increased (Figure S1C). All the above results confirmed the successful construction of the IL-33 modified MSCs vaccine.
The mouse immune responses induced by MSC vaccine were evaluated. As the most effective antigen-presenting cells (APCs), DCs play an important role in antitumor immune responses.Citation29 The MSC vaccine promotes DC maturation significantly in vitro. As shown in , the expression of maturation markers of DCs in the MSC vaccine group was the highest of the five vaccinated groups. In particular, the expression of CD80 was statistically significant between the MSC vaccine and other groups. CD86 and MHC II expression was higher in the MSC vaccine group than in B16F10 and PBS.
Figure 1. MSC vaccine improves the expression of marker of mature DCs and levels of IFN-γ and TNF-α. (a) Bone marrow-derived DCs from C57/BL6 mice were treated with different groups for 4d. The expression of CD80, CD86 and MHC II is detected by flow cytometry. (b) Different groups were pretreated with DCs for 4d, and then co-cultured with spleen cells for 6d. The secretion of IFN-γ and TNF-α in supernatant was determined by ELISA. (n = 3; *P < .05,**P < .01,***P < .001).
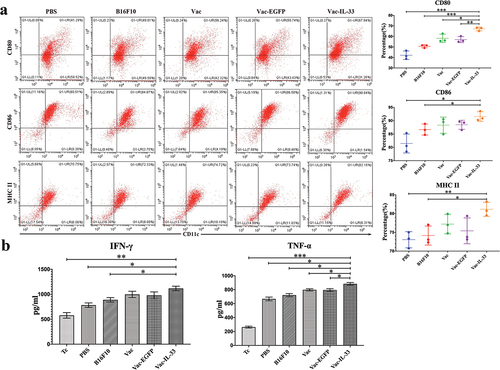
We further investigated whether the level of cytokines IFN-γ and TNF-α in the supernatant could be changed as well. shows that, when compared with the Tc, PBS, and B16F10 group, the IFN-γ level was dramatically increased in the Vac-IL-33 group, and the TNF-α level was significantly increased in the Vac-IL-33 group.
The spleen lymphocytes were isolated from different groups after 3 weeks for the last immunization. We evaluate the proportion and activity of CD8+T cells in splenocytes. The expressions of CD3, CD8, and CD69 of different groups were detected by flow cytometry. As shown in , the proportion of CD3/CD8 double-positive cells in Vac-IL-33 group was significantly higher than that in B16F10 and PBS groups. Similarly, immunohistochemistry revealed that the expression of CD8 in the Vac-IL33 group was distinctly greater than that in others (). In addition, the expression of early activation marker CD69 on CD8+T cells in Vac-IL-33 groups was significantly higher than that of others (). The above results indicated that the MSC vaccine was able to activate and accumulate CD8+T cells in immunized mice.
Figure 2. MSC vaccine increases the number of CD8+T cells and activates them. (a, b) Splenocytes were isolated from different groups. The expression of CD3, CD8 and CD69 in splenic lymphocytes of mice in different groups was determined by flow cytometry. (c) Immunohistochemical analysis of CD8 in tumor tissues. (n = 3; *P < .05, **P < .01).
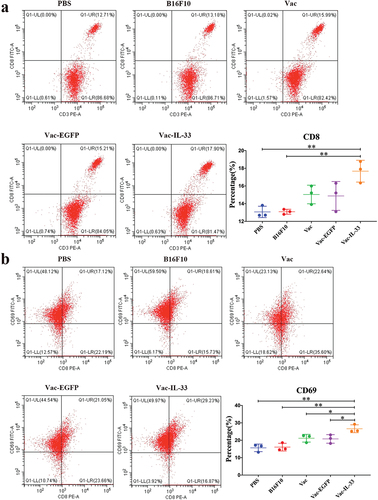
It is generally known that T cell activation is dependent on T cell receptor and co-stimulatory signals that can be inhibited through CTLA-4, PD-1, and Tim-3. These receptors play a critical role in the down regulation of T cell responses.Citation30,Citation31 We considered whether the expression of these immune checkpoints were suppressed by MSC vaccine in CD8+T cells. The expressions of PD-1 and CTLA-4 on CD8+T cells in Vac-IL-33 group were significantly lower than those in other control groups. Similarly, the expression of Tim-3 in Vac-IL-33 group was less than that in B16F10 and PBS groups (). These results showed that the vaccine could inhibit the expression of immune checkpoints on CD8+T cells, especially on PD-1 and CTLA-4.
Figure 3. MSC vaccine decreases the expression of immune checkpoints and enhances central memory CD8+T cells. (a) Splenic lymphocytes were harvested in 3 weeks after the last immunization. Flow cytometry was used to determine the expression of PD-1, CTLA-4, Tim-3. (b) CD8+T cells were obtained 3 weeks after the last immunization. Intracellular IFN-γ and GzmB in CD8+T cells were examined by flow cytometry. (c) Serum was collected from eyeball blood of the mice. Levels of IL-2, IFN-α, TNF-α and IFN-γ were detected in serum from through ELISA. (d) the expression of CD44 and CD62 L on CD8+T cells in different groups was determined by flow cytometry. The central memory T cells (Tcm, CD8+ CD44+ CD62 L+) in vaccine group was significantly higher than those in B16F10 and PBS groups, (n = 3; *P < .05,**P < .01, ***P < .001). (n = 3; *P < .05,**P < .01,***P < .001).
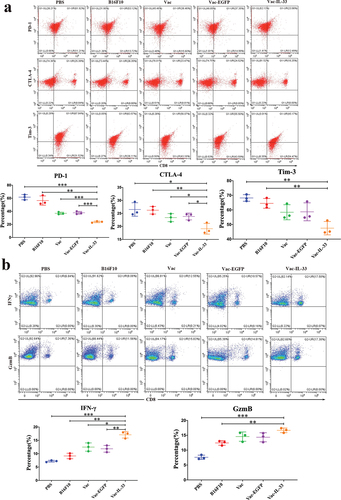
IFN-γ and GzmB are released by CD8+T cells against tumors.Citation32 Therefore, intracellular flow cytometry detected the expressions of IFN-γ and GzmB in CD8+T cells. Their expression in Vac-IL-33 group was higher than that in other control groups (). In addition, the levels of IL-2, IFN-α, TNF-α, and IFN-γ in serum of mice were detected by ELISA. The levels of IL-2, IFN-α and IFN-γ in Vac-IL-33 groups were greater than those in B16F10 and PBS group, and the level of TNF-α in Vac-IL-33 group was higher than those in other control groups (). Central memory T cells (Tcm) were phenotyped as CD8+CD44+CD62 L+. TCM cells located in secondary lymphoid organs have little or no effector function but readily expand and differentiate to effector cells in response to antigenic stimulation. Using flow analysis, we showed that Vac-IL-33 group displayed an augmented CD8+CD44+CD62 L+ positive percent (). The results demonstrated that the vaccine stimulated the killing effects of CD8+T cells by eliciting the expression and secretion anti-tumor cytokines and regulating TCM formation.
Effects of MSC vaccine against melanoma growth and metastasis
To assess the antitumor effect of MSC vaccine in vivo, tumor size was measured at 2, 3, and 4 weeks after tumor bearing. The PBS and B16F10 groups did not affect tumor growth, while a moderate antitumor effect was observed for Vac and Vac-EGFP groups, indicating the tumor inhibition efficacy of the +vaccine. Notably, the best efficacy was obtained for MSC vaccine, which can be attributable to the IL-33 (). We then studied whether MSC vaccine inhibits lung metastases by using a C57BL/6 melanoma model (the tail vein inoculation). The number of lung metastatic nodules was significantly decreased in the Vac-IL-33 group in comparison with the others (). These results clarified that MSC vaccine could inhibit melanoma growth and lung metastasis.
Figure 4. (a) Tumor size is measured at 2 weeks, 3 weeks and 4 weeks after tumor inoculation, including the maximum and minimum diameter of tumor. (b) At 3, 4, and 5 weeks after the tumor cells are injected into the tail vein of mice, the lung tissues are taken out and the number of metastatic nodules is counted. (c) The HE staining of tumor tissue. (d, e) The expression of Ki-67 and caspase-3 in tumors removed from C57/BL6 mice were detected by immunohistochemistry respectively. (n = 3; *P < .05,**P < .01,***P < .001).
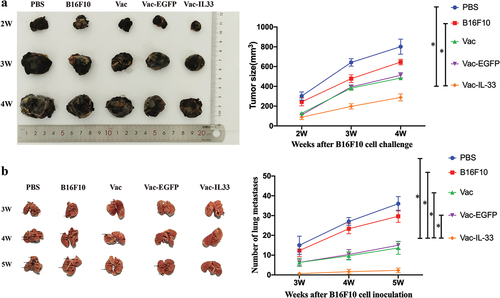
+Moreover, tumors from immunized mice were obtained 3 weeks after the last immunization for histopathology and immunohistochemistry. H&E staining revealed that the tumor tissue cells were uniform in size and the nucleus was intact in the Vac-IL-33 group. Cells showed different degrees of nuclear fragmentation in B16F10 group and nuclei pyknosis in tumor cells and necrosis were observed in the PBS group (). The expression of Ki-67, proliferation marker, detected via immunohistochemistry, obviously decreased in the Vac-IL-33 group compared to other control groups, while they increased in B16F10 and PBS groups (). Conversely, the expression of caspase-3, apoptotic markers, was higher than in other groups (). The above results demonstrated that MSC vaccine could induce apoptosis of tumor cells and protect normal cells from tumor.
Targeted killing effect of MSC vaccines
MSCs possibly lead to melanoma progression via multiple mechanisms. Targeting MSCs therapy for malignant melanoma has been making encouraging progress.Citation33 Therefore, cytotoxicity assay and flow cytometry were used to detect whether MSC vaccine targets MSCs. When the mouse spleen lymphocytes acted as effector cells, B16F10 cells were used as target cells. The cytotoxicity of splenic lymphocytes in Vac-IL-33 group was stronger than that in other control groups (). Likewise, the spleen lymphocytes of mice in Vac-IL-33 group were served as effector cells, and B16F10, B16F10-CD44-CD133-, and B16F10-CD44+CD133+ cells were used as target cells. We found that the cytotoxicity of spleen lymphocytes on B16F10-CD44+CD133+ cells in the Vac-IL-33 group was obviously stronger than that on B16F10 and B16F10-CD44-CD133- cells (). It is proved that the vaccine had a potent capacity to elicit specific cytotoxic T-lymphocyte effects. It had a targeted killing effect on melanoma stem cells.
Figure 5. MSC vaccine enhances the specific cytotoxic T-lymphocyte effects. (a) Splenic lymphocytes of different groups mice were collected as effector cells, and B16F10 cells as target cells. (b) Splenic lymphocytes in Vac-IL-33 group were collected as effector cells, and B16F10 cells, B16F10-CD44-CD133 -and B16F10-CD44+CD133+ as target cells. (c) MSC vaccine kills specifically CD44+CD133+ melanoma cells. The expression of CD44 and CD133 in melanoma tumor from different groups was evaluated by flow cytometry(n = 3; *P < .05).
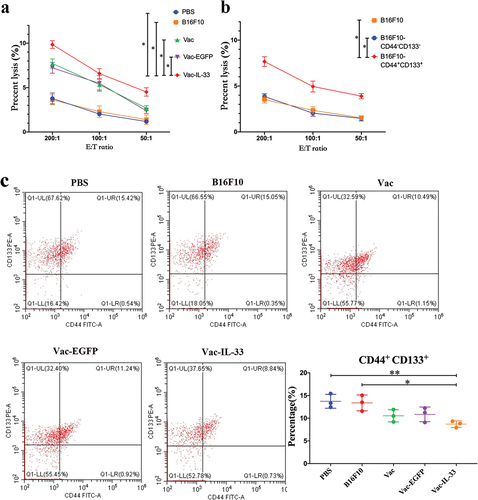
Additionally, the tumor cells in the tumor-bearing mice were collected from different groups after 3 weeks for the last immunization. The expressions of CD44 and CD133 in Vac-IL-33 group were lower than that in B16F10 and PBS groups. These results suggested that the vaccine could kill specifically CD44+CD133+ melanoma cells ().
Discussion
Cancer stem cells (CSCs) have been proven to exist in many solid tumors and expressed specific markers.Citation34–36 In melanoma, CD44 and CD133 can serve as potential markers of MSCs.Citation37,Citation38 In preclinical studies, CSCs-based immunotherapy has been tried in mice and humans, and achieved good results. DCs-CSCs vaccine can induce humoral and cellular immune responses against CSCs, thus producing efficient anti-tumor immunity.Citation8,Citation39,Citation40 Herein, the B16F10-D44+CD133+ cells were separated by immunomagnetic beads, and IL-33 overexpression was used to modify the sorted melanoma stem cells to prepare anti-tumor vaccine (MSC vaccine). Prophylactic immunization of mice showed that the MSC vaccine could activate the anti-tumor immune response by initiating CD8+T cells in multiple facets, and hence distinctly inhibit tumor progression and lung metastasis.
In previous studies, the vaccine that DCs loaded with lysate of MSCs (ALDEFLUOR+ D5 cells) could greatly inhibit melanoma growth and lung metastasis in immunized mice. The mechanism is that the vaccine activated antibodies and T cells to selectively target MSCs and induce protective anti-tumor immunity.Citation8,Citation14 IL-33 modified MSCs (B16F10-CD44+CD133+) vaccine in this study could also play an anti-tumor role by selectively targeting B16F10-CD44+CD133+ cells. Importantly, the anti-tumor immune mechanism of the MSC vaccine is through activating CD8+T cells in direct and indirect ways.
In the process of tumor immune response, the transformation of DCs from immature to mature is very important to initiate antigen-specific T cells.Citation41 Immature DCs have the inherent ability to internalize and endocytose vaccines through several mechanisms, including constitutive macropinocytosis and receptor-mediated endocytosis and phagocytosis.Citation29,Citation42 When DCs phagocytose vaccines, they undergo maturation and have an increased expression of B7 co-stimulatory molecules (CD80 and CD86), as well as present antigens to helper T cells or effector T cells to trigger specific cytotoxic T lymphocytes (CTLs) responsesCitation42,Citation43 (). Tumor cell lysates that are produced after CTLs kill the tumor can serve as the tumor antigen to further stimulate DCs. In addition, hence, the immune system is activated again to elicit an effective antitumor response (). Indeed, as the most sensitive activator to stimulate T cells, DCs need a very small amount of antigen to initiate T cell proliferation.Citation41,Citation44 Furthermore, IL-33, as an immune effector, can evoke DCs maturation and induce DCs recruitment.Citation45,Citation46 Although IL-33 has no direct effect on naive T cells, it can directly activate DCs to express costimulatory molecules in vivo,Citation46 and inhibit CD8+T cell differentiation depletion by down-regulating the expression of PD-1 and 2B4.Citation18 As we know, mature DCs promoted by IFN-γ and TNF-α appeared to be highly potent APC with increased expression of MHC II and T-cell costimulatory molecules (CD80 and CD86).Citation47,Citation48 CIK cells can greatly provoke tumor vaccines with the help of DCs that are an effective T cell stimulator.Citation49 CD69 is an early upregulated cell surface antigen based on activation of CD8+T cells.Citation50,Citation51 Previous studies showed that CD8+T cells are the ultimate effector cells against tumors.Citation52,Citation53 Our study showed that the IL-33-modified MSCs vaccine inhibited tumor growth by augmenting DC mature and activated CD8+T cells.
PD-1, CTLA-4, and Tim-3 as the main checkpoints contribute to weakening the activation of T cells, leading to the immunosuppression and promoting cancer progression.Citation54–56 Moreover, IL-33 is able to induce memory phenotype of tumor antigen-specific CD8+T cells in vitro.Citation45 Here, the MSC vaccine activated CD8+T cells by stimulating DCs maturation, including inducing the proliferation of effective T cells and initiating the secretion of anti-tumor cytokines.
So far, there have been very few common antigens discovered among cancers, and most of these antigens are not suitable for preparing universal vaccines.Citation57 This is due to the heterogeneity and complexity of cancer antigens.Citation58 Consequently, most cancer patients possess very few common antigens, and tumor tissues/cells are the best sources of antigens for cancer vaccines. Considering MSCs that contain antigens that can induce immune responses against melanoma. Intriguingly, the number of MSCs (CD44+CD133+ melanoma cells) in tumor tissue is significantly less after immunization ().
The main aims of anti-tumor vaccine are to expand high-affinity CD8+T cells, impel the T cells to differentiate into CTLs that can kill specifically cancer cells, and produce long-lived memory CD8+ T cells.Citation59 CTL's response is the most effective reaction to induce potential protective immunity against almost all tumors.Citation60,Citation61 The MSC vaccine in our study increased the expression of IFN-γ and GzmB on CD8+T cells, and enhanced the activation of specific CTLs. In addition, the formation of malignant tumor presents a chronic process, which creates conditions for the formation of memory CD8+T cells,Citation62 the vaccination is able to induce the formation of long-term memory CD8+T cells, which can prevent tumor recurrence.Citation59 In this study, the MSC vaccine can promote the formation of central memory CD8+T cells in spleen. These cells have the potential ability for proliferation and self-renewal,Citation63 and when encountering antigen again, they have a high proliferation potential and can differentiate into effector T cells.Citation64,Citation65
Conclusion
Taken together, this study confirms that the MSC vaccine expressing IL-33 promotes anti-tumor immunity in CD8+T cells and boosts targeted killing of melanoma stem cells, and, as a result, growth and metastasis of melanoma were significantly inhibited. Furthermore, our findings lay a theoretical and experimental foundation for the application of melanoma stem cell vaccine in the immunotherapy of melanoma, and provide ideas for the exploration of new therapeutic schemes for melanoma.
Abbreviations
MSCs | = | Melanoma stem cells |
CTL | = | cytotoxic T lymphocyte |
ICIs | = | immune checkpoint inhibitors |
CTLA-4 | = | cytotoxic T-lymphocyte antigen-4 |
PD-1 | = | programmed cell death protein-1 |
DCs | = | dendritic cells |
NK | = | natural killer cell |
EGFP | = | enhanced green fluorescent protein |
MMC | = | mitomycin C |
GM-CSF | = | granulocyte-macrophage colony-stimulating factor |
MHC | = | major histocompatibility complex |
GzmB | = | granzyme B |
APC | = | antigen presenting cells |
CSCs | = | Cancer stem cells |
Author’s contributions
QY and NX: conception and design, collection and/or assembly of data, data analysis and interpretation, and manuscript writing. HJ and WL: conception and design, supervision of the study, and final approval of the manuscript. NZ, YC: collection and/or assembly of data, data analysis, and manuscript writing. MD, MX, WX: collection and/or assembly of data. All authors read and approved the final manuscript.
Ethics approval and consent to participate
The use of human tissue was approved by the ethics committee of the First Hospital of Jilin University, China (EK Nr. 985/2016, 25 January 2018), and the donor gave written consent.
Supplemental Material
Download PDF (749.6 KB)Disclosure statement
No potential conflict of interest was reported by the author(s).
Data availability statement
The datasets used and/or analyzed during the current study are available from the corresponding author on reasonable request.
Supplemental data
Supplemental data for this article can be accessed on the publisher’s website at https://doi.org/10.1080/21645515.2022.2158670.
Additional information
Funding
References
- Onitilo AA, Wittig JA. Principles of immunotherapy in melanoma. Surg Clin North Am. 2020;100(1):161–13. doi:10.1016/j.suc.2019.09.009.
- Leonardi GC, Candido S, Falzone L, Spandidos DA, Libra M. Cutaneous melanoma and the immunotherapy revolution (Review). Int J Oncol. 2020;57(3):609–18. doi:10.3892/ijo.2020.5088.
- Livingstone A, Agarwal A, Stockler MR, Menzies AM, Howard K, Morton RL. Preferences for immunotherapy in melanoma: a systematic review. Ann Surg Oncol. 2020;27(2):571–84. doi:10.1245/s10434-019-07963-y.
- Albittar AA, Alhalabi O, Glitza Oliva IC. Immunotherapy for melanoma. Adv Exp Med Biol. 2020;1244:51–68.
- Seidel JA, Otsuka A, Kabashima K. Anti-PD-1 and anti-CTLA-4 therapies in cancer: mechanisms of action, efficacy, and limitations. Front Oncol. 2018;8:86. doi:10.3389/fonc.2018.00086.
- Yazdani M, Gholizadeh Z, Nikpoor AR, Hatamipour M, Alani B, Nikzad H, Mohamadian Roshan N, Verdi J, Jaafari MR, Noureddini M, et al. Vaccination with dendritic cells pulsed ex vivo with gp100 peptide-decorated liposomes enhances the efficacy of anti PD-1 therapy in a mouse model of melanoma. Vaccine. 2020;38(35):5665–77. doi:10.1016/j.vaccine.2020.06.055.
- Zhao F, Zhang R, Wang J, Wu D, Pan M, Li M, Guo M, Dou J. Effective tumor immunity to melanoma mediated by B16F10 cancer stem cell vaccine. Int Immunopharmacol. 2017;52:238–44. doi:10.1016/j.intimp.2017.09.019.
- Ning N, Pan Q, Zheng F, Teitz-Tennenbaum S, Egenti M, Yet J, Li M, Ginestier C, Wicha MS, Moyer JS, et al. Cancer stem cell vaccination confers significant antitumor immunity. Cancer Res. 2012;72(7):1853–64. doi:10.1158/0008-5472.CAN-11-1400.
- Yaiza JM, Gloria RA, Maria Belen GO, Elena L-R, Gema J, Juan Antonio M, María Ángel GC, Houria B. Melanoma cancer stem-like cells: optimization method for culture, enrichment and maintenance. Tissue Cell. 2019;60:48–59. doi:10.1016/j.tice.2019.07.005.
- Wang W, Bai L, Xu D, Li W, Cui J. Immunotherapy: a potential approach to targeting cancer stem cells. Curr Cancer Drug Targets. 2020;21(2):117–31. doi:10.2174/1568009620666200504111914.
- Badrinath N, Yoo SY. Recent advances in cancer stem cell-targeted immunotherapy. Cancers (Basel). 2019;11(3):310. doi:10.3390/cancers11030310.
- Zhang D, Tang DG, Rycaj K. Cancer stem cells: regulation programs, immunological properties and immunotherapy. Semin Cancer Biol. 2018;52:94–106. doi:10.1016/j.semcancer.2018.05.001.
- Zhou L, Lu L, Wicha MS, Chang AE, Xia J-C, Ren X, Li Q. Promise of cancer stem cell vaccine. Hum Vaccin Immunother. 2015;11(12):2796–99. doi:10.1080/21645515.2015.1083661.
- Dashti A, Ebrahimi M, Hadjati J, Memarnejadian A, Moazzeni SM. Dendritic cell based immunotherapy using tumor stem cells mediates potent antitumor immune responses. Cancer Lett. 2016;374(1):175–85. doi:10.1016/j.canlet.2016.01.021.
- Falcon-Beas C, Tittarelli A, Mora-Bau G, Tempio F, Pérez C, Hevia D, Behrens C, Flores I, Falcón-Beas F, Garrido P, et al. Dexamethasone turns tumor antigen-presenting cells into tolerogenic dendritic cells with T cell inhibitory functions. Immunobiology. 2019;224(5):697–705. doi:10.1016/j.imbio.2019.05.011.
- Kim WS, Han JM, Song HY, Byun EH, Lim ST, Byun EB. Annona muricata L. – derived polysaccharides as a potential adjuvant to a dendritic cell-based vaccine in a thymoma-bearing model. Nutrients. 2020;12(6):1602. doi:10.3390/nu12061602.
- Song HY, Han JM, Byun EH, Kim WS, Seo HS, Byun EB. Bombyx batryticatus protein-rich extract induces maturation of dendritic cells and Th1 polarization: a potential immunological adjuvant for cancer vaccine. Molecules. 2021;26(2):476. doi:10.3390/molecules26020476.
- Liu N, Jiang Y, Chen J, Nan H, Zhao Y, Chu X, Wang A, Wang D, Qin T, Gao S, et al. IL-33 drives the antitumor effects of dendritic cells via the induction of Tc9 cells. Cell Mol Immunol. 2019;16(7):644–51. doi:10.1038/s41423-018-0166-0.
- Jevtovic A, Pantic J, Jovanovic I, Milovanovic M, Stanojevic I, Vojvodic D, Arsenijevic N, Lukic ML, Radosavljevic GD. Interleukin-33 pretreatment promotes metastatic growth of murine melanoma by reducing the cytotoxic capacity of CD8(+) T cells and enhancing regulatory T cells. Cancer Immunol Immunother. 2020;69(8):1461–75. doi:10.1007/s00262-020-02522-x.
- Wang X, Zhao F, Shi F, He X, Pan M, Wu D, Li M, Zhang Y, Dou J. Reinforcing B16F10/GPI-IL-21 vaccine efficacy against melanoma by injecting mice with shZEB1 plasmid or miR200c agomir. Biomed Pharmacother. 2016;80:136–44. doi:10.1016/j.biopha.2016.03.013.
- Xu L, Zheng Y, Wang J, Xu Y, Xie Y, Yang ZP. IL33 activates CD8+T and NK cells through MyD88 pathway to suppress the lung cancer cell growth in mice. Biotechnol Lett. 2020;42(7):1113–21. doi:10.1007/s10529-020-02815-2.
- Jin Z, Lei L, Lin D, Liu Y, Song Y, Gong H, Zhu Y, Mei Y, Hu B, Wu Y, et al. IL-33 released in the liver inhibits tumor growth via promotion of CD4 (+) and CD8 (+) T cell responses in hepatocellular carcinoma. J Immunol. 2018;201(12):3770–79. doi:10.4049/jimmunol.1800627.
- Qi L, Zhang Q, Miao Y, Kang W, Tian Z, Xu D, Xiao W, Fang F. Interleukin-33 activates and recruits natural killer cells to inhibit pulmonary metastatic cancer development. Int J Cancer. 2020;146(5):1421–34. doi:10.1002/ijc.32779.
- Dominguez D, Ye C, Geng Z, Chen S, Fan J, Qin L, Long A, Wang L, Zhang Z, Zhang Y, et al. Exogenous IL-33 restores dendritic cell activation and maturation in established cancer. J Immunol. 2017;198(3):1365–75. doi:10.4049/jimmunol.1501399.
- Afferni C, Buccione C, Andreone S, Galdiero MR, Varricchi G, Marone G, Mattei F, Schiavoni G. The pleiotropic immunomodulatory functions of IL-33 and its implications in tumor immunity. Front Immunol. 2018;9:2601. doi:10.3389/fimmu.2018.02601.
- Gao X, Wang X, Yang Q, Zhao X, Wen W, Li G, Lu J, Qin W, Qi Y, Xie F, et al. Tumoral expression of IL-33 inhibits tumor growth and modifies the tumor microenvironment through CD8 + T and NK cells. J Immunol. 2015;194(1):438–45. doi:10.4049/jimmunol.1401344.
- Zhao Y, Chu X, Chen J, Wang Y, Gao S, Jiang Y, Zhu X, Tan G, Zhao W, Yi H, et al. Dectin-1-activated dendritic cells trigger potent antitumour immunity through the induction of Th9 cells. Nat Commun. 2016;7:12368. doi:10.1038/ncomms12368.
- Sun B, Fang YT, Jin DJ, Chen Z-Y, Li Z-Y, Gu X-D, Xiang J-B. miR-194 inhibits the proliferation of SW620 colon cancer stem cells through downregulation of SSH2 Expression. Cancer Manage Res. 2019;11:10229–38. doi:10.2147/CMAR.S221150.
- Lee YS, Radford KJ. The role of dendritic cells in cancer. Int Rev Cell Mol Biol. 2019;348:123–78.
- Rajamanickam A, Munisankar S, Dolla C, Nutman TB, Babu S, Herbert DR. Cytotoxic T-lymphocyte-associated antigen 4 (CTLA-4)- and programmed death 1 (PD-1)-mediated regulation of monofunctional and dual functional CD4 (+) and CD8 (+) T-cell responses in a chronic helminth infection. Infect Immun. 2019;87(12). doi:10.1128/IAI.00469-19.
- Kandel S, Adhikary P, Li G, Cheng K. The TIM3/Gal9 signaling pathway: an emerging target for cancer immunotherapy. Cancer Lett. 2021;510:67–78. doi:10.1016/j.canlet.2021.04.011.
- Galassi C, Manic G, Musella M, Sistigu A, Vitale I. Assessment of IFN-gamma and granzyme-B production by in “sitro” technology. Methods Enzymol. 2020;631:391–414.
- Korn P, Kampmann A, Spalthoff S, Jehn P, Tavassol F, Lentge F, Gellrich N-C, Zimmerer R. Suitability of CD133 as a marker for cancer stem cells in melanoma. Asian Pac J Cancer Prev. 2021;22(5):1591–97. doi:10.31557/APJCP.2021.22.5.1591.
- Tirino V, Desiderio V, Paino F, De Rosa A, Papaccio F, Fazioli F, Pirozzi G, Papaccio G. Human primary bone sarcomas contain CD133 + cancer stem cells displaying high tumorigenicity in vivo. FASEB J. 2011;25(6):2022–30. doi:10.1096/fj.10-179036.
- Yu C, Yao Z, Jiang Y, Keller ET. Prostate cancer stem cell biology. Minerva Urol Nefrol. 2012;64:19–33.
- Chu P, Clanton DJ, Snipas TS, Lee J, Mitchell E, Nguyen M-L, Hare E, Peach RJ. Characterization of a subpopulation of colon cancer cells with stem cell-like properties. Int J Cancer. 2009;124(6):1312–21. doi:10.1002/ijc.24061.
- Kloskowski T, Jarzabkowska J, Jundzill A, Balcerczyk D, Buhl M, Szeliski K, Bodnar M, Marszałek A, Drewa G, Drewa T, et al. CD133 antigen as a potential marker of melanoma stem cells: in vitro and in vivo studies. Stem Cells Int. 2020;2020:8810476. doi:10.1155/2020/8810476.
- Zimmerer RM, Matthiesen P, Kreher F, Kampmann A, Spalthoff S, Jehn P, Bittermann G, Gellrich N-C, Tavassol F. Putative CD133+ melanoma cancer stem cells induce initial angiogenesis in vivo. Microvasc Res. 2016;104:46–54. doi:10.1016/j.mvr.2015.12.001.
- Xu Q, Liu G, Yuan X, Xu M, Wang H, Ji J, Konda B, Black KL, Yu JS. Antigen-specific T-cell response from dendritic cell vaccination using cancer stem-like cell-associated antigens. Stem Cells. 2009;27(8):1734–40. doi:10.1002/stem.102.
- Teitz-Tennenbaum S, Wicha MS, Chang AE, Li Q. Targeting cancer stem cells via dendritic-cell vaccination. Oncoimmunology. 2012;1(8):1401–03. doi:10.4161/onci.21026.
- Santos PM, Butterfield LH. Dendritic cell-based cancer vaccines. J Immunol. 2018;200(2):443–49. doi:10.4049/jimmunol.1701024.
- Trombetta ES, Mellman I. Cell biology of antigen processing in vitro and in vivo. Annu Rev Immunol. 2005;23:975–1028. doi:10.1146/annurev.immunol.22.012703.104538.
- Collin M, Bigley V. Human dendritic cell subsets: an update. Immunology. 2018;154(1):3–20. doi:10.1111/imm.12888.
- Bhardwaj N, Young JW, Nisanian AJ, Baggers J, Steinman RM. Small amounts of superantigen, when presented on dendritic cells, are sufficient to initiate T cell responses. J Exp Med. 1993;178(2):633–42. doi:10.1084/jem.178.2.633.
- Dominguez D, Zhang Y, Zhang B. IL-33 in tumor immunity: nothing to sneeze at. Crit Rev Immunol. 2018;38(6):453–70. doi:10.1615/CritRevImmunol.2018026335.
- Besnard AG, Togbe D, Guillou N, Erard F, Quesniaux V, Ryffel B. IL-33-activated dendritic cells are critical for allergic airway inflammation. Eur J Immunol. 2011;41(6):1675–86. doi:10.1002/eji.201041033.
- Fainaru O, Woolf E, Lotem J, Yarmus M, Brenner O, Goldenberg D, Negreanu V, Bernstein Y, Levanon D, Jung S, et al. Runx3 regulates mouse TGF-β-mediated dendritic cell function and its absence results in airway inflammation. EMBO J. 2004;23(4):969–79. doi:10.1038/sj.emboj.7600085.
- Chen YF, Wang YH, Lei CS, Changou CA, Davis ME, Yen Y. Host immune response to anti-cancer camptothecin conjugated cyclodextrin-based polymers. J Biomed Sci. 2019;26(1):85. doi:10.1186/s12929-019-0583-0.
- Xu K, Meng Z, Mu X, Sun B, Chai Y. One single site clinical study: to evaluate the safety and efficacy of immunotherapy with autologous dendritic cells, cytokine-induced killer cells in primary hepatocellular carcinoma patients. Front Oncol. 2020;10:581270. doi:10.3389/fonc.2020.581270.
- Zhao X, Wang W, Zhang K, Yang J, Fuchs H, Fan H, Perricone C. Involvement of CD26 in differentiation and functions of Th1 and Th17 subpopulations of T lymphocytes. J Immunol Res. 2021;2021:6671410. doi:10.1155/2021/6671410.
- Kunert A, Basak EA, Hurkmans DP, Balcioglu HE, Klaver Y, van Brakel M, Oostvogels AAM, Lamers CHJ, Bins S, Koolen SLW, et al. CD45RA+CCR7− CD8 T cells lacking co-stimulatory receptors demonstrate enhanced frequency in peripheral blood of NSCLC patients responding to nivolumab. J Immunother Cancer. 2019;7(1):149. doi:10.1186/s40425-019-0608-y.
- Li S, Luo M, Wang Z, Feng Q, Wilhelm J, Wang X, Li W, Wang J, Cholka A, Fu Y-X, et al. Prolonged activation of innate immune pathways by a polyvalent STING agonist. Nat Biomed Eng. 2021;5(5):455–66. doi:10.1038/s41551-020-00675-9.
- Tan S, Wang K, Sun F, Li Y, Gao Y. CXCL9 promotes prostate cancer progression through inhibition of cytokines from T cells. Mol Med Rep. 2018;18(2):1305–10. doi:10.3892/mmr.2018.9152.
- Wang F, Liu Y, Xu W, Zhang C, Lv J, Ma S. Fulminant myocarditis induced by immune checkpoint inhibitor nivolumab: a case report and review of the literature. J Med Case Rep. 2021;15(1):336. doi:10.1186/s13256-021-02934-y.
- Guo LL, Wang GC, Li PJ, Wang CM, Liu LB. Recombinant adenovirus expressing a dendritic cell-targeted melanoma surface antigen for tumor-specific immunotherapy in melanoma mice model. Exp Ther Med. 2018;15(6):5394–402. doi:10.3892/etm.2018.6085.
- El-Ashmawy NE, Salem ML, Abd El-Fattah EE, Khedr EG. Targeting CD166(+) lung cancer stem cells: molecular study using murine dendritic cell vaccine. Toxicol Appl Pharmacol. 2021;429:115699. doi:10.1016/j.taap.2021.115699.
- Melief CJ, van Hall T, Arens R, Ossendorp F, van der Burg SH. Therapeutic cancer vaccines. J Clin Invest. 2015;125(9):3401–12. doi:10.1172/JCI80009.
- Ott PA, Hu Z, Keskin DB, Shukla SA, Sun J, Bozym DJ, Zhang W, Luoma A, Giobbie-Hurder A, Peter L, et al. An immunogenic personal neoantigen vaccine for patients with melanoma. Nature. 2017;547(7662):217–21. doi:10.1038/nature22991.
- Palucka K, Banchereau J. Dendritic-cell-based therapeutic cancer vaccines. Immunity. 2013;39(1):38–48. doi:10.1016/j.immuni.2013.07.004.
- Celluzzi CM, Mayordomo JI, Storkus WJ, Lotze MT, Falo LD Jr. Peptide-pulsed dendritic cells induce antigen-specific CTL-mediated protective tumor immunity. J Exp Med. 1996;183(1):283–87. doi:10.1084/jem.183.1.283.
- Nair SK, Heiser A, Boczkowski D, Majumdar A, Naoe M, Lebkowski JS, Vieweg J, Gilboa E. Induction of cytotoxic T cell responses and tumor immunity against unrelated tumors using telomerase reverse transcriptase RNA transfected dendritic cells. Nat Med. 2000;6(9):1011–17. doi:10.1038/79519.
- Shrikant PA, Rao R, Li Q, Kesterson J, Eppolito C, Mischo A, Singhal P. Regulating functional cell fates in CD8 T cells. Immunol Res. 2010;46(1–3):12–22. doi:10.1007/s12026-009-8130-9.
- Reiser J, Banerjee AE. Memory, and dysfunctional CD8(+) T cell fates in the antitumor immune response. J Immunol Res. 2016;2016:8941260. doi:10.1155/2016/8941260.
- Wherry EJ, Kurachi M. Molecular and cellular insights into T cell exhaustion. Nat Rev Immunol. 2015;15(8):486–99. doi:10.1038/nri3862.
- Klebanoff CA, Gattinoni L, Restifo NP. CD8+ T-cell memory in tumor immunology and immunotherapy. Immunol Rev. 2006;211:214–24. doi:10.1111/j.0105-2896.2006.00391.x.