ABSTRACT
The receptor-binding domain (RBD) of SARS-CoV-2 S protein is proved to be the major target of neutralizing antibodies. However, on the S protein, only a portion of epitopes in RBD can be effectively displayed with dynamic changes in spatial conformations. Using RBD fragment as antigen can better expose the neutralizing epitopes, but the immunogenicity of RBD monomer is suboptimal. Multimeric display of RBD molecules is a feasible strategy to optimize RBD-based vaccines. In this study, RBD single-chain dimer derived from Wuhan-Hu-1 was fused with a trimerization motif, and a cysteine was also introduced at the C-terminus. The resultant recombinant protein 2RBDpLC was expressed in Sf9 cells using a baculovirus expression system. Reducing/non-reducing PAGE, size-exclusion chromatography and in silico structure prediction indicated that 2RBDpLC polymerized and possibly formed RBD dodecamers through trimerization motif and intermolecular disulfide bonds. In mice, 2RBDpLC induced higher levels of RBD-specific and neutralizing antibody responses than RBD dimer, RBD trimer and prefusion-stabilized S protein (S2P). In addition, cross-neutralizing antibodies against Delta and Omicron VOC were also detected in the immune sera. Our results demonstrate that 2RBDpLC is a promising vaccine candidate, and the method of constructing dodecamers may be an effective strategy for designing RBD-based vaccines.
Introduction
The severe acute respiratory syndrome coronavirus 2 (SARS-CoV-2) has caused the global outbreak of COVID-19, which brought a severe threat to human health and imposed great economic losses. As of August 2022, there have been over 587 million confirmed cases of COVID-19, including over 6 million deaths, reported to the WHO. Several vaccines have been urgently approved for use and played an important role in reducing severe disease and mortality. However, during the epidemic period, the virus has undergone multiple mutations, resulting in a decline in the effectiveness of the vaccines initially developed.Citation1,Citation2 It is expected that new waves of transmission may still occur in the future as new variants of SARS-CoV-2 continuously emerge. Therefore, an effective next-generation vaccine and global vaccination will be the key to controlling the epidemic.
SARS-CoV-2 is an enveloped, positive strand RNA virus that uses human angiotensin-converting enzyme 2 (hACE2) as cellular receptor for infection.Citation3,Citation4 The S protein on the envelop of SARS-CoV-2 binds to hACE2 with its receptor-binding domain (RBD),Citation5 which is proved to be the major target of neutralizing antibodies.Citation6–9 The antigenicity of S protein depends on the maintenance of the pre-fusion structure,Citation10 which is very unstable, as Furin, TMPRSS2 and cathepsin L proteases can cleave the protease site between S1 and S2,Citation6–11Citation11–13 resulting in segregation of S1 subunit. Pallesen et al. found that mutation of two prolines between the central helix (CH) and heptapeptide repeat domain 1 (HR1) can stabilize the pre-fusion conformation of S protein.Citation14 Another study reported that the S trimer vaccine NVX-CoV2373, which contains the two-proline mutation and Furin cleave site mutation, showed a more stable and compact structure,Citation15 and induced high titers of neutralizing antibodies and potent protection responses.Citation15–17 Structural analyses showed that the RBD of SARS-CoV-2 is partially masked in the S protein.Citation6,Citation11,Citation18 Compared to the S protein, the structure of RBD-based antigen is less complex, and the shielded epitopes in the S protein can be better exposed in RBD form. Therefore, RBD is considered as a potential vaccine candidate although its immunogenicity is suboptimal. Many forms of RBD-based vaccines have been reported, including RBD monomers,Citation19–24 RBD single-chain dimer,Citation25 RBD-Fc dimers,Citation26,Citation27 RBD trimers,Citation28–30 RBD nano-particles,Citation31,Citation32 etc. A yeast-expressed RBD monomer formulated with alum-3 M–052 adjuvant induced robust neutralizing antibody and cellular immune responses in rhesus macaques and reduced the virus load and lung inflammation upon SARS-CoV-2 challenge.Citation22 The licensed vaccine Corbevax, composed of RBD monomer and CpG1018 adjuvant, was proved to be safe and effective,Citation23,Citation24 and the vaccine-induced neutralizing antibodies persisted for 12 months after the second dose.Citation23 The RBD single-chain dimer vaccine (ZF2001) induced higher level of neutralizing antibody responses than RBD monomerCitation25 and was shown to be safe and effective against symptomatic and severe-to-critical COVID-19 in clinical trials.Citation2 The RBD trimer (RBD fusing with T4 trimerization domain) adjuvanted with alum-3 M–052 induced potent neutralizing antibody responses and protective immunity against SARS-CoV-2 challenge in both mice and macaques.Citation30 Another RBD trimer, hCMP-mRBD, constructed by fusing RBD with trimerization domain derived from cartilage matrix protein, induced higher titers of neutralizing antibodies than RBD monomer and S2P protein.Citation28 RBD nano-particles constructed with SpyCather-SpyTagCitation31 or I53–50Citation31,Citation32 systems also induced higher levels of neutralizing antibody responses than the RBD monomer or prefusion-stabilized S protein. The AS03-adjuvanted RBD-I53-50 elicited robust and durable protective immune responses in rhesus macaques,Citation33,Citation34 and was approved for use in individuals 18 y or older in Korea. These reports indicate that multimeric display of SARS-CoV-2 RBD protein has a significant advantage in enhancing immunogenicity.
In this study, we explored the use of trimerization motifs to construct RBD recombinant proteins with different polymerization levels, and screened vaccine candidates with good stability and immunogenicity. RBD single-chain dimer derived from S protein of Wuhan-Hu-1 strain was respectively fused with different trimerization motifs to construct RBD hexamers. Moreover, a cysteine was introduced at the C-terminus of trimerization motif to induce further polymerization through intermolecular disulfide bonds, in order to form RBD dodecamers. The RBD dodecamer (2RBDpLC) showed good hACE2 binding activity and induced high level of RBD-specific antibodies and neutralizing antibodies against Wuhan-Hu-1 pseudovirus in mice. In addition, neutralizing antibodies against pseudovirus of Delta and Omicron VOC were also detected in 2RBDpLC immune sera, although the antibody levels were lower than that against Wuhan-Hu-1 strain.
Materials and methods
Construction, expression and purification of RBD oligomers
The RBD dimer, RBDST and S2P were designed as described in previous reportsCitation15,Citation25,Citation29 and illustrated in Figure S1a. RBD hexamers and dodecamers were constructed by fusing RBD dimer with trimerization motifs, respectively, with or without a cysteine at the C-terminus (). TS linkers were inserted between RBD and trimerization motif. The amino acid sequences of the trimerization motifs are listed in Table S1. A Strep tag II was added at the C-terminus or N-terminus of each construct. The genes of RBD hexamers and dodecamers (Gene Bank accession: OP293798-OP293803, respectively) were optimized according to the codon usage bias of Sf9 and cloned into pFastBac1 plasmid (Invitrogen). The recombinant proteins were expressed in Sf9 cells using the Bac-to-Bac baculovirus expression system according to the manufacturer’s instructions (Invitrogen). Expression of recombinant proteins was detected by Western blot with anti-Strep tag II monoclonal antibody (BBI Life Sciences).
Figure 1. Expression and characterization of recombinant proteins. (a) Schematic diagram of RBD recombinant proteins. (b) the recombinant proteins were expressed in Sf9-baculovirus expression system, and the expression of each RBD recombinant protein was detected by Western blot with anti-Strep tag II monoclonal antibody (1:3000). (c) Purified recombinant proteins were examined by reducing and non-reducing SDS-PAGE with Coomassie blue staining. NR, non-reducing; R, reducing. (d) Purified recombinant proteins were examined by analytical gel filtration with Tricorn 10/200 Superdex 200 HR. A representative elution curve is shown.
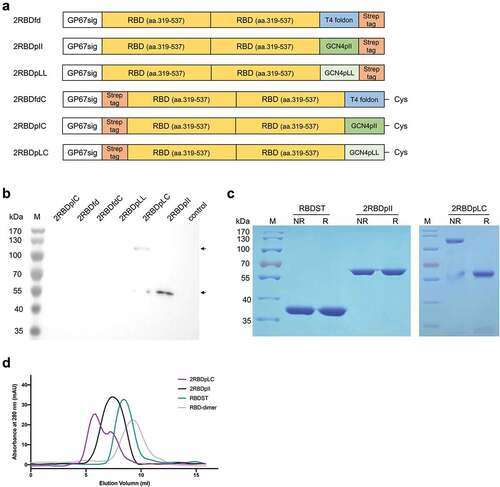
The expressed recombinant proteins were purified from culture medium by Strep-Tactin XT Sepharose affinity chromatography according to the manufacturer’s instructions (GE Healthcare). After purification, proteins were dialyzed against PBS at 4°C for 24 h. Total proteins were measured by Pierce BCA Protein Assay kit (Thermo Scientific), and the yield of each recombinant protein was calculated and shown in Table S2. The purified proteins were analyzed by reducing and non-reducing SDS-PAGE with Coomassie blue staining and were further analyzed by gel-filtration chromatography (SEC) using a Tricorn 10/200 Superdex 200 HR column (GE Healthcare).
Enzyme-linked immunosorbent assay (ELISA)
The binding of RBD oligomers to hACE2 was analyzed by ELISA. Briefly, ELISA plates were respectively coated with serially diluted RBD oligomers and S2P (0.078–2 pmol/well/100 μl) at 4°C overnight, and then blocked with 5% bovine serum albumin (BSA) at room temperature for 2 h. After blocking, hACE2-hIgG-Fc proteins (2 μg/ml, AntibodyChina) were added to the plates and incubated at room temperature for 2 h, followed by three washes with PBST (0.05% Tween 20 in PBS). HRP-conjugated goat anti-human IgG (1:500, Beyotime) was added and incubated at 37°C for 1 h. Enzyme activity was revealed with substrate O-phenylenediamine (Sigma-Aldrich) and the reaction was stopped by 2 M H2SO4. The absorbance was measured at 490 nm. The ELISA data were fitted to a dose–response four-parameter logistic (4PL) model, and the binding EC50 was calculated. The experiment was repeated twice.
The binding of RBD oligomers to SARS-CoV-2 neutralizing monoclonal antibody (MAb) was analyzed by ELISA. Plates were respectively coated with serially diluted RBD oligomers and S2P (0.078–2 pmol/well) at 4°C overnight. After blocking, SARS-CoV-2 RBD-specific neutralizing MAbs (1 μg/ml, human IgG1, Beyotime) were added to the plates and incubated at room temperature for 2 h. HRP-conjugated goat anti-human IgG (1:500, Beyotime) was added and incubated at 37°C for 1 h. Enzyme activity was detected and the binding EC50 was calculated as described above.
The sera IgG/IgG1/IgG2a titers against SARS-CoV-2 RBD were also analyzed by ELISA. Briefly, plates were coated with RBD (200 ng/well) at 4°C overnight. After blocking, plates were incubated with serially diluted immune sera at RT for 2 h, and then incubated with HRP-conjugated goat anti-mouse IgG (1:5000)/IgG1 (1:1000)/IgG2a (1:1000) at 37°C for 1 h. Enzyme activity was detected as described above. The binding titer was determined as the reciprocal of the highest serum dilution with an OD490 greater than 0.2 and twofold higher than control sera at the same dilution.
Animal immunizations
BALB/c mice were purchased from the Institute of Laboratory Animal Science, Chinese Academy of Medical Sciences (CAMS) and kept in the animal facility of the Institute of Basic Medical Sciences (IBMS), CAMS, under pathogen-free conditions. All animal studies were performed in accordance with the guidelines of the Institutional Animal Care and Use Committee (IACUC) of IBMS, CAMS, and all protocols were reviewed and approved by IACUC.
Female BALB/c mice (n = 5) were vaccinated subcutaneously at d 0, 10 with 3 μg of recombinant proteins formulated with 25 μg Alum and 5 μg CpG 7909, respectively. At d 20, sera were collected and subjected to ELISA analysis and SARS-CoV-2 pseudovirus (PsV)-based neutralization assay.
SARS-CoV-2 PsV-based neutralization assay
SARS-CoV-2 PsV-based neutralization assay was performed as previously described with minor modifications.Citation35 Briefly, 293T-hACE2 cells were seeded in 96-well plate at 1.5 × 104 cells/well and incubated overnight at 37°C. The mixture of serially diluted antisera and PsVs (Wuhan-Hu-1, Delta B.1.617.2 and Omicron B.1.1.529) (Biodragon, Jiangsu, China) were transferred to pre-plated 293T-hACE2 cells and cultured for 48–72 h. The 293T-hACE2 cells were harvested by trypsin digestion and then detected by FACS. The endpoint titer was calculated as the reciprocal of the highest serum dilution with percent infection inhibition higher than 50%.
Data analysis
Statistical significance was determined by unpaired two-tailed nonparametric Mann−Whitney test, or one-way ANOVA followed by post hoc Tukey’s test for multiple comparisons, using GraphPad Prism 8.0. P values < .05 were considered to be statistically significant.
Results
Preparation and characterization of RBD recombinant proteins
As illustrated in , the RBD recombinant proteins were composed of RBD single-chain dimer and trimerization motifs. To facilitate protein secretion, a GP67 signal peptide sequence was added to the N-terminus. A cysteine was introduced to the C-terminus of trimerization domain, in order to form higher-level assembly structures by the formation of intermolecular disulfide bond. The recombinant proteins were expressed in Sf9 using baculovirus expression system. The expression of recombinant proteins was examined by Western blot with anti-Strep tag II antibody. A specific band of about 60 kDa was observed in 2RBDpII and 2RBDpLC samples (), which was consistent with the theoretical molecular weight. A band of about 120 kDa was also detected in the 2RBDpLC sample. Expression of 2RBDfd, 2RBDfdC, 2RBDpLL and 2RBDpIC was not observed. The RBD dimer,Citation25 RBD trimer (RBDST) Citation29 and S2PCitation15 were constructed as previously reported (Figure S1a) and also expressed in Sf9-baculovirus system (Figure S1b). In addition, we determined the mRNA secondary structures of RBD oligomers by Vienna RNAfold Web Server, and found that the RBD oligomers showed similar mRNA secondary structures with similar levels of minimum free energy (Figure S2a). Moreover, mRNA levels were examined by QPCR, and the results showed that no statistical difference was observed among the mRNA expression levels of RBD oligomers (Figure S2b). These data indicated that differences in RBD oligomer expression levels were not associated with mRNA structure and abundance.
To evaluate the formation of intermolecular disulfide bond, 2RBDpII, 2RBDpLC and a previously reported RBD trimer (RBDST)Citation29 were purified and analyzed by reducing and non-reducing SDS-PAGE (). Under non-reducing conditions, 2RBDpLC protein showed a major band at 120 kDa and a minor band at 60 kDa, while under reducing conditions, only one band at 60 kDa was observed, indicating that an intermolecular disulfide bond was formed in 2RBDpLC protein. But for RBDST and 2RBDpII protein, intermolecular disulfide bond was not observed, as only one band was shown under either reducing or non-reducing conditions. The results of analytical SEC showed that 2RBDpLC was eluted first, followed by 2RBDpII, RBDST and RBD dimer (), indicating that 2RBDpLC has a higher degree of polymerization compared to other oligomers. Therefore, we speculate that 2RBDpLC may first form a trimer of RBD dimers through the trimerization domain, and then form disulfide bonds through the C-terminal cysteines for further polymerization, as suggested by the structural prediction results ().
Figure 2. Structure prediction of RBD oligomers. Structures of RBDST, 2RBDpII and 2RBDpLC proteins were predicted by ColabFold (AlphaFold2 using MMseqs2)Citation36,Citation37.
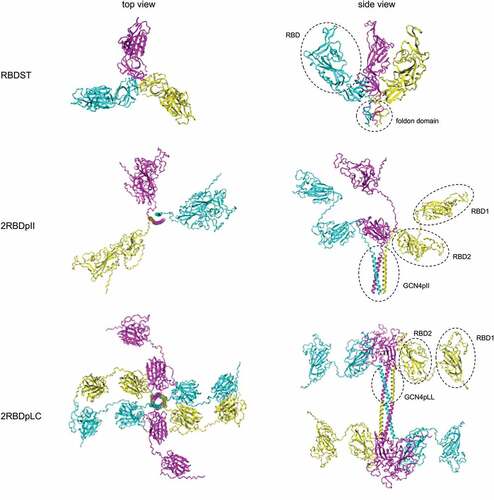
The exposure of neutralizing epitopes in RBD recombinant proteins was assessed by ELISA (), and the binding EC50 was calculated (). The RBD-specific neutralizing MAb bound strongly to 2RBDpLC, RBDST and RBD dimer, suggesting that neutralizing epitopes were well exposed. However, the binding activity of 2RBDpII was much lower than that of 2RBDpLC, indicating that the neutralizing epitopes were not well exposed or properly formed in 2RBDpII. We also evaluated the binding activities of RBD recombinant proteins to hACE2. As illustrated in , the binding activities of 2RBDpLC were the highest, followed by RBDST, 2RBDpII, RBD dimer and S2P. The hACE2-binding EC50 of 2RBDpLC was significantly lower than that of other RBD oligomers and S2P (, p < .001).
Figure 3. Binding activities of RBD oligomers with RBD-specific neutralizing MAbs and hACE2. ELISA plates were coated with serially diluted RBD oligomers and S2P (0.078–2 pmol/well/100 μl). (a) Plates were incubated with RBD-specific neutralizing MAbs (1 μg/ml, human IgG1). Binding activity was detected with HRP-conjugated goat-anti-human IgG (1:500). (b) Plates were incubated with hACE2-Fc recombinant protein (2 μg/ml). Binding activity was detected with HRP-conjugated goat-anti-human IgG (1:500). Reactivity was determined by measuring the mean optical density (OD) values at 490 nm. The ELISA data were fitted to a dose-response four-parameter logistic (4PL) model, and the binding EC50 of RBD oligomers to neutralizing MAbs (c) and to hACE2 were calculated respectively. The experiment was repeated twice. The statistically significant differences (using one-way ANOVA) were indicated by **p < .01; ***p < .001.
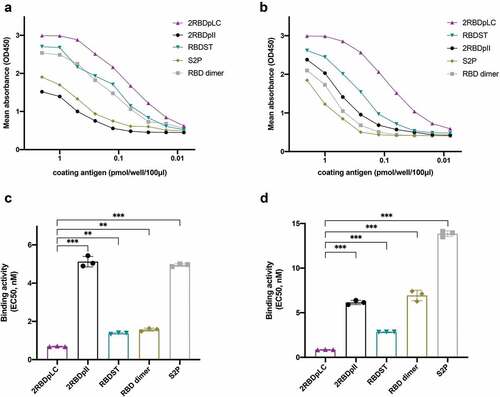
2RBDpLC induced high level of RBD-specific antibody responses
To test the ability of RBD oligomers to induce specific antibody responses, sera from mice that were immunized with Alum-CpG adjuvanted recombinant proteins were analyzed by ELISA (). The results showed that 2RBDpLC induced significantly higher levels of RBD-specific IgG (mean titer, 35,840) than other recombinant proteins (), which was 3.1 times that of RBD dimer (11,680, p < .01), 4.6 times that of RBDST (7,820, p < .01), 10.0 times that of S2P (3,600, p < .01), and 10.2 times that of 2RBDpII (3,520, p < .01). There was no statistical difference between the other groups. Then. we measured the RBD-specific IgG1 and IgG2a () levels in the 2RBDpLC immune sera and observed that the IgG2a titers (mean titer, 1,280) were higher than IgG1 titers (mean titer, 340).
Figure 4. Sera IgG responses to RBD oligomers. Groups of mice (n = 5) were immunized subcutaneously at d 0 and 10 with RBD oligomers (3 μg) or S2P (3 μg) formulated with alum (25 μg) and CpG7909 (5 μg). Sera were collected at d 20 and tested for anti-RBD IgG titers (a). The anti-RBD IgG1/IgG2a titers induced by 2rbdplc were also detected (b). ELISA plates were coated with RBD (200 ng/well), and binding with serially diluted sera was detected with HRP-conjugated goat-anti-mouse IgG, HRP-conjugated rabbit-anti-mouse IgG1 or HRP-conjugated rabbit-anti-mouse IgG2a. Reactivity was determined by measuring the mean optical density (OD) values at 490 nm. The results were presented as mean titer ± SD, and the dotted line indicates the limit of detection for the assay. The statistically significant differences (using one-way ANOVA) were indicated by **p < .01; ***p < .001.
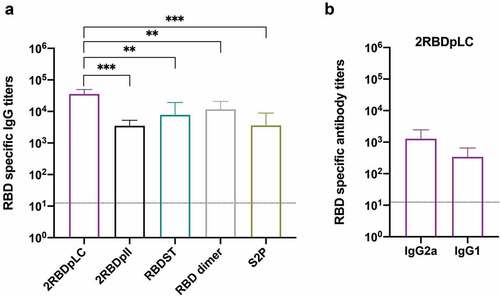
2RBDpLC induced robust neutralizing antibody responses against SARS-CoV-2
To elucidate whether the induced RBD-specific antibodies also have neutralizing activities, the immune sera were further determined by SARS-CoV-2 pseudovirus-based neutralization assay. We first detected the neutralizing antibody titers against Wuhan-Hu-1 strain (). The results showed that 2RBDpLC induced the most potent neutralizing antibody responses with a mean titer of 700, which was 6.3 times that of RBD dimer (mean titer, 112; p < .05), 6.6 times that of RBDST (mean titer, 106; p < .05) and 10.6 times that of S2P (mean titer, 66; p < .05). In contrast, 2RBDpII induced only weak neutralizing antibody responses with a mean titer of 19. This result indicates that the immunogenicity of 2RBDpLC is superior to other oligomer vaccine candidates, which is also consistent with the RBD-specific IgG antibody titers.
Figure 5. Neutralizing antibody titers induced by RBD oligomers. Groups of mice (n = 5) were immunized subcutaneously at d 0 and 10 with RBD oligomers (3 μg) or S2P (3 μg) formulated with Alum (25 μg) and CpG7909 (5 μg). Sera were collected at d 20 and tested for PsV neutralizing antibody titers against Wuhan-Hu-1 (a), Delta B.1.617.2 (b) and Omicron B.1.1.529 (c). Individual titers of 2rbdplc immune sera against Wuhan-Hu-1 and VOC were compared (d). The results were presented as mean titer ± SD, and the dotted line indicates the limit of detection for the assay. The overlaid points were slightly staggered to show each individual. The statistically significant differences were indicated by *p < .05; **p < .01.
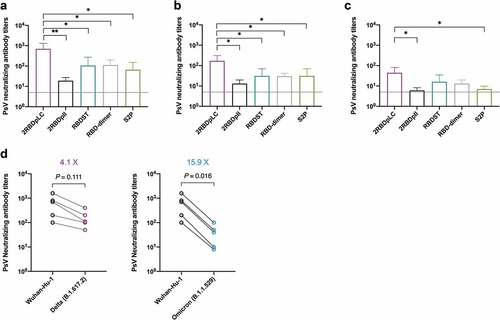
As SARS-CoV-2 mutates rapidly, many variants escape from previous neutralizing antibodies, resulting in a decline in the effectiveness of the initially manufactured vaccines.Citation1,Citation2 The RBD oligomers in this study were constructed according to the amino acid sequence of Wuhan-Hu-1 strain. In order to determine whether the neutralizing antibodies induced by the recombinant proteins, especially 2RBDpLC, can cross-neutralize different variants of concern (VOC), pseudoviruses of Delta (B.1.617.2) and Omicron (B.1.1.529) VOC were used, respectively. The results showed that neutralizing antibodies against Delta strain could be detected in all serum samples of 2RBDpLC group with a mean titer of 170 (), which was significantly higher than that of 2RBDpII, RBDST, RBD dimer and S2P (p < .05). Moreover, neutralizing antibodies against Omicron strain were also detected in 2RBDpLC immune sera (), and the mean titer was higher than that of 2RBDpII and S2P (p < .05). The results suggested that 2RBDpLC has the advantage of eliciting cross-neutralizing antibodies against variants of SARS-CoV-2. Comparing the neutralizing activities of 2RBDpLC immune sera against Wuhan-Hu-1 and VOC, we found that the neutralizing titer decreased 4.1-fold for Delta and decreased 15.9-fold for Omicron ().
Discussion
As the baculovirus-insect cell expression system has the advantages of high yield of target protein and good safety, many approved vaccines have been manufactured with this system, including HPV vaccine Cervarix and quadrivalent influenza vaccine Flublok, etc. It is reported that the SARS-CoV RBD protein (aa.318–510) expressed in Sf9 insect cells elicited significantly higher titers of neutralizing antibodies than RBD expressed in prokaryotic cells.Citation38 In this study, three recombinant proteins, 2RBDfd, 2RBDpII and 2RBDpLL, containing two copies of RBD fused with different trimerization motifs, were expressed in insect cells. Another three proteins, 2RBDfdC, 2RBDpIC and 2RBDpLC, with an additional cysteine at the C-terminus were also constructed. We found that 2RBDpII and 2RBDpLC were expressed efficiently, while other four recombinant proteins were not detected by Western blot ()). To clarify whether the differences in protein expression were associated with mRNA structures and abundance, we predicted the mRNA secondary structures and employed qPCR. To our surprise, the RBD oligomers showed similar secondary structures and expression levels of mRNA (Figure S2). We also observed that 2RBDpII was easy to degrade after freeze–thaw cycles, while 2RBDpLC was relatively stable. Therefore, we speculate that the trimerization motifs and location of purification tags might affect the spatial structure and stability of RBD oligomers and thus affect the protein expression levels.
The 2RBDpLC bound to neutralizing MAb and hACE2 with high affinity (), and the binding EC50 to neutralizing MAb and hACE2 were significantly lower than that of other RBD oligomers (). The results indicate that neutralizing epitopes in 2RBDpLC were properly formed and exposed, and the critical sites for ACE2-binding were probably in the native conformation. Contrarily, 2RBDpII bound poorly with the neutralizing MAb and bound moderately with hACE2, indicating that 2RBDpII may form a suboptimal conformation. To be noted, 2RBDpLC induced relatively high levels of RBD-specific and PsV neutralizing antibodies (), which was consistent with the results of binding activity analysis.
Fusing RBD single-chain dimer with a trimerization motif may form RBD hexamer protein. Previous studies have shown that the addition of cysteine to the N-terminus of the leucine zipper can lead to further oligomerizationCitation39; thus, we speculate that introducing a cysteine at the C-terminus may presumably promote the formation of RBD dodecamer. Non-reducing SDS-PAGE () and analytical SEC () revealed that 2RBDpLC assembled into oligomers of heterogeneous sizes, and the intermolecular disulfide bonds were partially formed between 2RBDpLC molecules. It is possible that large steric hindrance is created when multiple copies of RBD molecules are displayed, making it difficult for the two C-terminal cysteines to approach each other. Further modifications, such as adding a linker between the trimerization motif and C-terminal cysteine, may improve the homogeneity of 2RBDpLC oligomers.
Prophylactic vaccines usually prevent viral infections by inducing neutralizing antibodies. Passive transfer of convalescent sera and anti-spike neutralizing antibodies proved that neutralizing antibodies played an important role in blocking SARS-CoV-2 infection.Citation40–42 Therefore, RBD specific antibody titers and neutralizing antibody titers induced by RBD oligomers were analyzed in this study. The results showed that 2RBDpLC induced significantly higher titers of anti-RBD IgG and neutralizing antibodies than RBD dimer, RBDST and S2P (). Structure prediction showed that RBD fragments present a relatively stretched structure in the 2RBDpLC oligomer (), and furthermore, 2RBDpLC bound to hACE2 with high affinity (). So, we speculate that 2RBDpLC not only sufficiently displayed the neutralizing epitopes on RBD but also further enhanced the immune activity by increasing the copy number of RBD. Potent adjuvants can also improve the immunogenicity of recombinant protein vaccines. S trimer recombinant protein vaccines adjuvanted with either Alum/CpG or AS03 induced robust neutralizing antibody responses.Citation43 When formulated with Alum/CpG adjuvant, 2RBDpLC also induced high level of humoral immunity, suggesting that CpG/Alum is a suitable adjuvant for RBD-based recombinant protein vaccines.
Pseudovirus neutralization assay revealed that 2RBDpLC induced relatively high neutralization titers against Delta VOC, although there was a 4.1-fold decrease compared with the titers against Wuhan-Hu-1 strain. Since B.1.617.2 strain has only two mutations in RBD (L452 R and T478K), it is reasonable that the 2RBDpLC immune sera retained neutralization capacity against this variant. A previous study reported that lineages with the L452 R mutation led to a lower decrease in vaccine-induced neutralization than other variants.Citation44 Compared with Delta VOC, Omicron led to significant reduction of neutralization capacity. As the RBD of Omicron contains 15 mutations, the variant escapes from the majority of existing SARS-CoV-2 neutralizing antibodies.Citation45 It is reported that the neutralizing antibody titers induced by BNT162b2 vaccine or by three doses of inactivate vaccines, respectively, reduced 22-fold and 16.5-fold for Omicron than for the ancestral virus.Citation46,Citation47 In this study, neutralization titers induced by 2RBDpLC reduced 15.9-fold for Omicron, which was consistent with previous reports. Since 2RBDpLC induced higher titers of neutralizing antibodies than other RBD oligomers, we believe that combining with stronger adjuvants or an additional boost may further increase 2RBDpLC-induced VOC neutralizing antibody titers.
Recent studies reported that S protein vaccine derived from Omicron VOC is not as potent as the vaccine derived from the original strain in inducing neutralizing antibodies.Citation48 Clinical studies of the vaccines derived from the ancestral virus have shown that the protection against Omicron was observed although the protection rate was remarkably decreased.Citation2 These findings indicate that improving the immunogenicity of vaccines constructed from the original strain is a possible way to deal with VOC. The 2RBDpLC protein constructed in this study showed an oligomeric strategy that can effectively increase the immunogenicity of RBD. Our results may provide a reference for the development of efficient RBD vaccines against different VOC in future.
Supplemental Material
Download PDF (703.1 KB)Acknowledgments
The authors would like to thank Prof. Ye Liu (Institute of Medical Biology, Chinese Academy of Medical Sciences) for his generously offering 293T-hACE2 cells.
Disclosure statement
No potential conflict of interest was reported by the author(s).
Supplementary data
Supplemental data for this article can be accessed on the publisher’s website at https://doi.org/10.1080/21645515.2023.2174755.
Additional information
Funding
References
- Muik A, Wallisch AK, Sänger B, Swanson KA, Mühl J, Chen W, Cai H, Maurus D, Sarkar R, Türeci Ö, et al. Neutralization of SARS-CoV-2 lineage B.1.1.7 pseudovirus by BNT162b2 vaccine–elicited human sera. Science. 2021;371(6534): 1152–9. doi:10.1126/science.abg6105.
- Dai L, Gao L, Tao L, Hadinegoro SR, Erkin M, Ying Z, He P, Girsang RT, Vergara H, Akram J, et al. Efficacy and safety of the RBD-Dimer–based Covid-19 vaccine ZF2001 in adults. N Engl J Med. 2022;386(22): 2097–111. doi:10.1056/NEJMoa2202261.
- Lu R, Zhao X, Li J, Niu P, Yang B, Wu H, Wang W, Song H, Huang B, Zhu N, et al. Genomic characterisation and epidemiology of 2019 novel coronavirus: implications for virus origins and receptor binding. Lancet. 2020;395(10224): 565–74. doi:10.1016/S0140-6736(20)30251-8.
- Wang Q, Zhang Y, Wu L, Niu S, Song C, Zhang Z, Lu G, Qiao C, Hu Y, Yuen KY, et al. Structural and functional basis of SARS-CoV-2 entry by using human ACE2. Cell. 2020;181(4): 894–904.e9. doi:10.1016/j.cell.2020.03.045.
- Lan J, Ge J, Yu J, Shan S, Zhou H, Fan S, Zhang Q, Shi X, Wang Q, Zhang L, et al. Structure of the SARS-CoV-2 spike receptor-binding domain bound to the ACE2 receptor. Nature. 2020;581(7807): 215–20. doi:10.1038/s41586-020-2180-5.
- Wrapp D, Wang N, Corbett KS, Goldsmith JA, Hsieh CL, Abiona O, Graham BS, McLellan JS. Cryo-EM structure of the 2019-nCov spike in the prefusion conformation. Science. ( 1979) 2020;367(6483):1260–63. doi:10.1126/science.abb2507.
- Cao Y, Su B, Guo X, Sun W, Deng Y, Bao L, Zhu Q, Zhang X, Zheng Y, Geng C, et al. Potent neutralizing antibodies against SARS-CoV-2 identified by high-throughput single-cell sequencing of convalescent patients’ B Cells. Cell. 2020;182(1): 1–12. doi:10.1016/j.cell.2020.05.025.
- Yuan M, Wu NC, Zhu X, Lee CCD, So RTY, Lv H, Mok CKP, Wilson IA. A highly conserved cryptic epitope in the receptor binding domains of SARS-CoV-2 and SARS-CoV. Science. ( 1979) 2020;368(6491):630–33. doi:10.1126/science.abb7269.
- Jiang S, Hillyer C, Du L. Neutralizing antibodies against SARS-CoV-2 and other human coronaviruses. Trends Immunol. 2020;41(5):355–59. doi:10.1016/j.it.2020.03.007.
- Hsieh C-L, Goldsmith JA, Schaub JM, DiVenere AM, Kuo H-C, Javanmardi K, Le KC, Wrapp D, Lee AG, Liu Y, et al. Structure-based design of prefusion-stabilized SARS-CoV-2 spikes. Science. 2020;369(6510): 1501–05. doi:10.1126/science.abd0826.
- Wang MY, Zhao R, Gao LJ, Gao XF, Wang DP, Cao JM. SARS-CoV-2: structure, biology, and structure-based therapeutics development. Front Cell Infect Microbiol. 2020;10. doi:10.3389/fcimb.2020.587269.
- Shang J, Wan Y, Luo C, Ye G, Geng Q, Auerbach A, Li F. Cell entry mechanisms of SARS-CoV-2. Proc Nat Acad Sci. 2020;117(21):11727–34. doi:10.1073/pnas.2003138117.
- Hoffmann M, Kleine-Weber H, Schroeder S, Krüger N, Herrler T, Erichsen S, Schiergens TS, Herrler G, Wu NH, Nitsche A, et al. SARS-CoV-2 cell entry depends on ACE2 and TMPRSS2 and is blocked by a clinically proven protease inhibitor. Cell. 2020;181(2): 271–80.e8. doi:10.1016/j.cell.2020.02.052.
- Pallesen J, Wang N, Corbett KS, Wrapp D, Kirchdoerfer RN, Turner HL, Cottrell CA, Becker MM, Wang L, Shi W, et al. Immunogenicity and structures of a rationally designed prefusion MERS-CoV spike antigen. Proc Natl Acad Sci U S A. 2017;114(35): E7348–57. doi:10.1073/pnas.1707304114.
- Tian JH, Patel N, Haupt R, Zhou H, Weston S, Hammond H, Logue J, Portnoff AD, Norton J, Guebre-Xabier M, et al. SARS-CoV-2 spike glycoprotein vaccine candidate NVX-CoV2373 immunogenicity in baboons and protection in mice. Nat Commun. 2021;12(1). doi:10.1038/s41467-020-20653-8.
- Guebre-Xabier M, Patel N, Tian JH, Zhou B, Maciejewski S, Lam K, Portnoff AD, Massare MJ, Frieman MB, Piedra PA, et al. NVX-CoV2373 vaccine protects cynomolgus macaque upper and lower airways against SARS-CoV-2 challenge. Vaccine. 2020;38(50): 7892–96. doi:10.1016/j.vaccine.2020.10.064.
- Keech C, Albert G, Cho I, Robertson A, Reed P, Neal S, Plested JS, Zhu M, Cloney-Clark S, Zhou H, et al. Phase 1–2 Trial of a SARS-CoV-2 recombinant spike protein nanoparticle vaccine. N Engl J Med. 2020;383(24): 2320–32. doi:10.1056/NEJMoa2026920.
- V’kovski P, Kratzel A, Steiner S, Stalder H, Thiel V. Coronavirus biology and replication: implications for SARS-CoV-2. Nat Rev Microbiol. 2021;19(3):155–70. doi:10.1038/s41579-020-00468-6.
- Yang J, Wang W, Chen Z, Lu S, Yang F, Bi Z, Bao L, Mo F, Li X, Huang Y, et al. A vaccine targeting the RBD of the S protein of SARS-CoV-2 induces protective immunity. Nature. 2020;586(7830): 572–77. doi:10.1038/s41586-020-2599-8.
- Malladi SK, Singh R, Pandey S, Gayathri S, Kanjo K, Ahmed S, Khan MS, Kalita P, Girish N, Upadhyaya A, et al. Design of a highly thermotolerant, immunogenic SARS-CoV-2 spike fragment. J Biol Chem. 2021;296:100025. doi:10.1074/jbc.RA120.016284.
- Pollet J, Chen WH, Versteeg L, Keegan B, Zhan B, Wei J, Liu Z, Lee J, Kundu R, Adhikari R, et al. SARS‑CoV-2 RBD219-N1C1: a yeast-expressed SARS-CoV-2 recombinant receptor-binding domain candidate vaccine stimulates virus neutralizing antibodies and T-cell immunity in mice. Hum Vaccin Immunother. 2021;17(8): 2356–66. doi:10.1080/21645515.2021.1901545.
- Pino M, Abid T, Pereira Ribeiro S, Edara VV, Floyd K, Smith JC, Latif MB, Pacheco-Sanchez G, Dutta D, Wang S, et al. A yeast-expressed RBD-based SARS-CoV-2 vaccine formulated with 3M-052-alum adjuvant promotes protective efficacy in non-human primates. Sci Immunol. 2021;6(61). doi:10.1126/sciimmunol.abh3634.
- Thuluva S, Paradkar V, Gunneri SR, Yerroju V, Mogulla R, Turaga K, Kyasani M, Manoharan SK, Medigeshi G, Singh J, et al. Evaluation of safety and immunogenicity of receptor-binding domain-based COVID-19 vaccine (Corbevax) to select the optimum formulation in open-label, multicentre, and randomised phase-1/2 and phase-2 clinical trials. EBioMedicine. 2022;83:104217. doi:10.1016/j.ebiom.2022.104217.
- Thuluva S, Paradkar V, Gunneri S, Yerroju V, Mogulla RR, Suneetha PV, Turaga K, Kyasani M, Manoharan SK, Adabala S, et al. Safety, tolerability and immunogenicity of biological E’s CORBEVAX™ vaccine in children and adolescents: a prospective, randomised, double-blind, placebo controlled, phase-2/3 study. Vaccine. 2022;40(49): 7130–40. doi:10.1016/j.vaccine.2022.10.045.
- Dai L, Zheng T, Xu K, Han Y, Xu L, Huang E, An Y, Cheng Y, Li S, Liu M, et al. A universal design of betacoronavirus vaccines against COVID-19, MERS, and SARS. Cell. 2020;182(3): 722–33.e11. doi:10.1016/j.cell.2020.06.035.
- Sun S, Cai Y, Song TZ, Pu Y, Cheng L, Xu H, Sun J, Meng C, Lin Y, Huang H, et al. Interferon-armed RBD dimer enhances the immunogenicity of RBD for sterilizing immunity against SARS-CoV-2. Cell Res. 2021;31(9): 1011–23. doi:10.1038/s41422-021-00531-8.
- Liu Z, Xu W, Xia S, Gu C, Wang X, Wang Q, Zhou J, Wu Y, Cai X, Qu D, et al. RBD-Fc-based COVID-19 vaccine candidate induces highly potent SARS-CoV-2 neutralizing antibody response. Signal Transduct Target Ther. 2020;5(1): 282. doi:10.1038/s41392-020-00402-5.
- Malladi SK, Patel UR, Rajmani RS, Singh R, Pandey S, Kumar S, Khaleeq S, van Vuren PJ, Riddell S, Goldie S, et al. Immunogenicity and protective efficacy of a highly thermotolerant, trimeric SARS-CoV-2 receptor binding domain derivative. ACS Infect Dis. 2021;7(8): 2546–64. doi:10.1021/acsinfecdis.1c00276.
- Zhang T, Wang Z, Xu X. Impact of glycosylation and length of RBD of SARS-CoV-2 S protein on the immunogenicity of RBD protein vaccines. Basic Clin Med. 2020;40:1645–50.
- Routhu NK, Cheedarla N, Bollimpelli VS, Gangadhara S, Edara VV, Lai L, Sahoo A, Shiferaw A, Styles TM, Floyd K, et al. SARS-CoV-2 RBD trimer protein adjuvanted with Alum-3M-052 protects from SARS-CoV-2 infection and immune pathology in the lung. Nat Commun. 2021;12(1): 3587. doi:10.1038/s41467-021-23942-y.
- Kang YF, Sun C, Zhuang Z, Yuan RY, Zheng Q, Li JP, Zhou PP, Chen XC, Liu Z, Zhang X, et al. Rapid development of SARS-CoV-2 spike protein receptor-binding domain self-assembled nanoparticle vaccine candidates. ACS Nano. 2021;15(2): 2738–52. doi:10.1021/acsnano.0c08379.
- Walls AC, Fiala B, Schäfer A, Wrenn S, Pham MN, Murphy M, Tse L, Shehata L, O’connor MA, Chen C, et al. Elicitation of potent neutralizing antibody responses by designed protein nanoparticle vaccines for SARS-CoV-2. Cell. 2020;183(5): 1367–82.e17. doi:10.1016/j.cell.2020.10.043.
- Arunachalam PS, Feng Y, Ashraf U, Hu M, Walls AC, Edara VV, Zarnitsyna VI, Aye PP, Golden N, Miranda MC, et al. Durable protection against the SARS-CoV-2 Omicron variant is induced by an adjuvanted subunit vaccine. Sci Transl Med. 2022;14(658): 4130. doi:10.1126/scitranslmed.abq4130.
- Arunachalam PS, Walls AC, Golden N, Atyeo C, Fischinger S, Li C, Aye P, Navarro MJ, Lai L, Edara VV, et al. Adjuvanting a subunit COVID-19 vaccine to induce protective immunity. Nature. 2021;594(7862): 253–58. doi:10.1038/s41586-021-03530-2.
- Nie J, Li Q, Wu J, Zhao C, Hao H, Liu H, Zhang L, Nie L, Qin H, Wang M, et al. Establishment and validation of a pseudovirus neutralization assay for SARS-CoV-2. Emerg Microbes Infect. 2020;9(1): 680–86. doi:10.1080/22221751.2020.1743767.
- Mirdita M, Schütze K, Moriwaki Y, Heo L, Ovchinnikov S, Steinegger M. ColabFold: making protein folding accessible to all. Nat Methods. 2022;19(6):679–82. doi:10.1038/s41592-022-01488-1.
- Jumper J, Evans R, Pritzel A, Green T, Figurnov M, Ronneberger O, Tunyasuvunakool K, Bates R, Žídek A, Potapenko A, et al. Highly accurate protein structure prediction with AlphaFold. Nature. 2021;596(7873): 583–89. doi:10.1038/s41586-021-03819-2.
- Du L, Zhao G, Chan CCS, Sun S, Chen M, Liu Z, Guo H, He Y, Zhou Y, Zheng BJ, et al. Recombinant receptor-binding domain of SARS-CoV spike protein expressed in mammalian, insect and E. coli cells elicits potent neutralizing antibody and protective immunity. Virology. 2009;393(1): 144–50. doi:10.1016/j.virol.2009.07.018.
- Harbury PB, Zhang T, Kim PS, Alber T. A switch between two-, three-, and four-stranded coiled coils in GCN4 leucine zipper mutants. Science. ( 1979) 1993;262(5138):1401–07. doi:10.1126/science.8248779.
- Pooladanda V, Thatikonda S, Godugu C. The current understanding and potential therapeutic options to combat COVID-19. Life Sci. 2020;254:117765. doi:10.1016/j.lfs.2020.117765.
- Shen C, Wang Z, Zhao F, Yang Y, Li J, Yuan J, Wang F, Li D, Yang M, Xing L, et al. Treatment of 5 critically ill patients with COVID-19 with convalescent plasma. JAMA. 2020;323(16): 1582–89. doi:10.1001/jama.2020.4783.
- Zost SJ, Gilchuk P, Case JB, Binshtein E, Chen RE, Nkolola JP, Schäfer A, Reidy JX, Trivette A, Nargi RS, et al. Potently neutralizing and protective human antibodies against SARS-CoV-2. Nature. 2020;584:443–49.
- Liang JG, Su D, Song TZ, Zeng Y, Huang W, Wu J, Xu R, Luo P, Yang X, Zhang X, et al. S-trimer, a COVID-19 subunit vaccine candidate, induces protective immunity in nonhuman primates. Nat Commun. 2021;12(1). doi:10.1038/s41467-021-21634-1.
- Lucas C, Vogels CBF, Yildirim I, Rothman JE, Lu P, Monteiro V, Gehlhausen JR, Campbell M, Silva J, Tabachnikova A, et al. Impact of circulating SARS-CoV-2 variants on mRNA vaccine-induced immunity. Nature. 2021;600(7889): 523–29. doi:10.1038/s41586-021-04085-y.
- Cao Y, Wang J, Jian F, Xiao T, Song W, Yisimayi A, Huang W, Li Q, Wang P, An R, et al. Omicron escapes the majority of existing SARS-CoV-2 neutralizing antibodies. Nature. 2022;602(7898): 657–63. doi:10.1038/s41586-021-04385-3.
- Cele S, Jackson L, Khoury DS, Khan K, Moyo-Gwete T, Tegally H, San JE, Cromer D, Scheepers C, Amoako DG, et al. Omicron extensively but incompletely escapes Pfizer BNT162b2 neutralization. Nature. 2022;602(7898): 654–56. doi:10.1038/s41586-021-04387-1.
- Wang K, Jia Z, Bao L, Wang L, Cao L, Chi H, Hu Y, Li Q, Zhou Y, Jiang Y, et al. Memory B cell repertoire from triple vaccinees against diverse SARS-CoV-2 variants. Nature. 2022;603(7903): 919–25. doi:10.1038/s41586-022-04466-x.
- He C, He X, Yang J, Lei H, Hong W, Song X, Yang L, Li J, Wang W, Shen G, et al. Spike protein of SARS‐CoV‐2 Omicron (B.1.1.529) variant have a reduced ability to induce the immune response. Signal Transduct Target Ther. 2022;7(1). doi:10.1038/s41392-022-00980-6.