ABSTRACT
Matrix-M™ adjuvant is a key component of several novel vaccine candidates. The Matrix-M adjuvant consists of two distinct fractions of saponins purified from the Quillaja saponaria Molina tree, combined with cholesterol and phospholipids to form 40-nm open cage-like nanoparticles, achieving potent adjuvanticity with a favorable safety profile. Matrix-M induces early activation of innate immune cells at the injection site and in the draining lymph nodes. This translates into improved magnitude and quality of the antibody response to the antigen, broadened epitope recognition, and the induction of a Th1-dominant immune response. Matrix-M-adjuvanted vaccines have a favorable safety profile and are well tolerated in clinical trials. In this review, we discuss the latest findings on the mechanisms of action, efficacy, and safety of Matrix-M adjuvant and other saponin-based adjuvants, with a focus on the severe acute respiratory syndrome coronavirus 2 (SARS-CoV-2) subunit vaccine candidate NVX-CoV2373 developed to prevent coronavirus disease 2019 (COVID-19).
Introduction
The worldwide pandemic of coronavirus disease 2019 (COVID-19), caused by the severe acute respiratory syndrome coronavirus 2 (SARS-CoV-2), has led to unprecedented urgency of vaccine development and subsequent mass vaccination campaigns.Citation1–3 Novavax has developed an adjuvanted, recombinant, subunit vaccine targeting the SARS-CoV-2 spike protein, NVX-CoV2373 (Nuvaxovid™). This vaccine is well tolerated and has high efficacy for the prevention of COVID-19 in phase 2/3 clinical trials.Citation4–6 The vaccine’s immunogenicity is critically dependent on the adjuvant, Matrix-M™, which has antigen dose-sparing properties and promotes the induction of potent humoral and cellular immune responses. In this review, we will describe why this adjuvant is a critical component for NVX-CoV2373 and other novel vaccines.
Vaccine adjuvants are substances that enhance and modulate the immunogenicity of antigens.Citation7,Citation8 Due to their capacity to activate components of the innate immune system, adjuvants increase the magnitude, broaden the specificity, change the humoral and cellular characteristics of the vaccine-induced immune responses, and improve memory responses. These properties have enabled the development of vaccines for target populations (e.g., infants, older adults, and immunocompromised persons) and infectious diseases (e.g., malaria, herpes zoster, and avian influenza) for which traditional vaccines have been unsuccessful or of limited efficacy. In addition, adjuvants may also help reduce the number of doses needed to immunize an individual or reduce the amount of antigen needed in each dose (dose-sparing effect).
The need for new adjuvants has been strongly driven by the development of vaccines containing highly purified antigens derived from recombinant technologies, which are more precisely characterized and safer, but potentially less immunogenic, than live-attenuated or inactivated whole-cell vaccines. Indeed, most older live-attenuated, inactivated, or toxoid vaccines are “self-adjuvanted” because they contain intrinsic molecules called pathogen-associated molecular patterns that are recognized by cells of the innate immune system such as monocytes, macrophages, and dendritic cells (DCs) via a range of so-called pattern recognition receptors.Citation7 In contrast, purified recombinant protein vaccines often lack these microbial patterns necessary to trigger innate immune responses. Adding adjuvants to the vaccine antigens triggers a rapid innate immune response at the injection site and/or the draining lymph nodes (dLNs) by attracting and activating antigen-presenting cells (APCs). The APCs can then process and present the antigen to CD4+ and CD8+ T cells. Both T-cell types are capable of providing cellular effector functions and CD4+ T cells help to develop a mature adaptive immune response. This acute response sets the stage to create immunological memory that can persist for years and react more rapidly upon subsequent infection. By modifying the initial signal provided to the innate immune system, the adjuvant also influences the type of adaptive immune responses induced against the vaccine antigen. Although adjuvants have been used for more than a century, the mechanisms of action of the ones most widely used in humans – aluminum salts, oil-in-water emulsions, saponin-based adjuvants (SBAs), and Toll-like receptor (TLR) agonists – are still not fully understood, and animal models may not fully reflect the more nuanced picture found in human data. This review presents an overview of the effects of the saponin-based Matrix-M adjuvant in light of the mechanism of action data generated to date for Matrix-M itself and other SBAs.
A brief history of saponin-based adjuvants
Saponins are a large family of glycoconjugates that share a triterpene structure with a variety of glycoside side chains.Citation9 Most saponins used in vaccine adjuvants and food are extracted through a sustainable process from the bark of the South American soapbark tree Quillaja saponaria Molina.Citation9,Citation10 These bark extracts contain a heterogeneous mixture of dozens of closely related saponins with structurally different glycosylation or acylation patterns that also affect their biological activities.Citation10–13 Of the numerous components that can be purified, Q. saponaria Molina QS-21 is the best characterized, both structurally and functionally, and is associated with a potent adjuvant activity.Citation10,Citation14 However, QS-21 is chemically unstable in alkaline conditions, shows hemolytic activity in vitro, and is associated with immediate pain at the injection site.Citation12,Citation15 These limitations of free QS-21 can be attenuated by incorporating it into particles with cholesterol, as done in the liposome-based Adjuvant System 01 (AS01) and the Army Liposome Formulation Q (ALFQ),Citation16–18 or with a combination of cholesterol and phospholipid, as in immune-stimulating complexes (ISCOMs) or Matrix-M nanoparticles.Citation19 In addition to “quenching” saponin hemolytic activity, the formulation into nanoparticles allows targeting the delivery of the adjuvant to phagocytic cells, thus focusing stimulation to appropriate cells.Citation16,Citation18,Citation20 Saponin adjuvants can also synergize with other classes of adjuvants, such as TLR agonists. This characteristic is used in the liposome-based adjuvant AS01, which combines QS-21 and the TLR4 agonist MPL (3-O-desacyl-4’-monophosphoryl lipid A), a nontoxic derivative from Salmonella minnesota lipopolysaccharide.Citation16 The QS-21 and MPL components of this adjuvant enhance antigen-specific antibody responses and promote T-cell responses.Citation16 AS01 is currently used in the licensed vaccines against malaria and herpes zoster, both developed by GSK (Rixensart, Belgium).Citation10,Citation16 The synergistic adjuvant effects of saponins and MPL are also seen when saponins and MPL are incorporated into ISCOM nanoparticles.Citation21
ISCOMs were initially developed by Morein and colleagues to improve the immunogenicity of membrane-derived viral glycoproteins by coformulation of these glycoproteins with adjuvant-active Quillaja saponins present in the bark extract fractions.Citation22 The characteristic structure of ISCOMs relies on the strong affinity between saponins and cholesterol. ISCOMs are stable particles formed by Q. saponaria saponins, cholesterol, and phospholipids, in which multiple copies of antigens are physically incorporated into a matrix of saponins and lipids. ISCOMs are 40-nm-diameter particles with typical rigid cage-like structures.Citation19 ISCOMs induce strong and long-lasting antigen-specific humoral and cellular immune responses, including CD4+ helper T cells and CD8+ cytotoxic T cells. However, a limitation of the ISCOM system is that only hydrophobic membrane proteins were readily incorporated into ISCOMs without modification.Citation22 Manufacturing challenges that arose when incorporating a broader variety of antigens into the ISCOM particles led to the discovery that similar characteristic structures were produced even without antigen incorporation.Citation23 These complexes, later called Matrix, constituted a potent adjuvant that could be simply mixed with a broad range of antigens.Citation23 The Matrix technology was further developed under the trademark ISCOMATRIX™ (CSL Limited, Parkville, Victoria, Australia).Citation24,Citation25 Although antigens are not physically linked to the particles, these formulations retain the ISCOMs’ ability to induce strong humoral and cellular immune responses, and as they are not limited to hydrophobic membrane proteins, they potentially have broader applications.
The ISCOM/ISCOMATRIX-branded technology was further developed by Isconova/Novavax AB (Uppsala, Sweden) and led to the development of the Matrix-M formulation. The Matrix-M adjuvant consists of two different populations of physically stable nanoparticles mixed at a defined ratio (85% Matrix-A + 15% Matrix-C). Matrix-A™ and Matrix-C™ contain different Q. saponaria saponin fractions with complementary properties.Citation19,Citation26,Citation27 Matrix-C particles contain Fraction-C saponins (mainly consisting of QS-21), which have strong adjuvant activity but are reactogenic in mice, as measured by lethargy and lethality. Matrix-A particles contain Fraction-A saponins, which have a weaker adjuvant activity than Fraction-C at the same doses but are better tolerated in mice. A combination of these two types of particles was tested and reduced the reactogenicity observed in animal models while preserving the adjuvant activity. In addition, Matrix-M is stable in aqueous solution at 2–8°C for several years. Matrix-M is the adjuvant used in NVX2373 SARS-CoV-2 recombinant spike protein-based vaccine (Novavax) as well as several vaccines that are currently being, or have been, evaluated in clinical trials (summarized in ).
Table 1. Overview of ongoing and concluded clinical trials with Matrix-M™-adjuvanted vaccines.
How do saponin-based adjuvants work?
Many of the insights into the mechanisms of action of SBAs have been obtained from mouse models, using mainly AS01 and ISCOMATRIX. When assessed using Matrix-M, similar results were obtained, suggesting shared mechanisms. The proposed Matrix-M mechanisms of action are summarized in .
Figure 1. Proposed Matrix-M™ adjuvant mechanism of action.
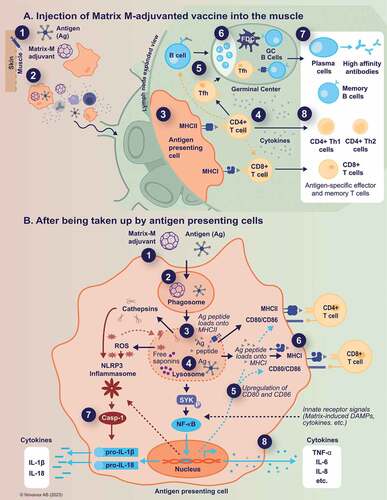
Early events at the site of injection
After intramuscular injection of AS01, saponins can be detected first in the muscle interstitium, but do not linger there. Already at 24 hours post-injection, both saponins and antigens are undetectable at the injection site, thus making a depot effect and slow release of antigen unlikely as an important mechanism of action.Citation28 Early events after immunization with SBAs involve the production of chemokines and cytokines both at the site of injection and in the dLN. The cytokine induction is transient, with cytokine levels declining sharply by 48 hours and returning to baseline by day 7.Citation28 Among the detected cytokines are key mediators of immune activation and immune cell differentiation (interferon-γ [IFN-γ], interleukin [IL]-1β, IL-6, and tumor necrosis factor [TNF]-α). Notably, IFN-γ promotes Th1 cell differentiation and antibody class switching, and upregulates MHC class I and class II expression on DCs, thus promoting the induction of cell-mediated immunity.Citation29 Moreover, chemokines attracting innate immune cells such as monocytes and granulocytes (CXCL1 and CCL2) or T cells (CXCL9 and CXCL10) are produced.Citation28 This cell recruitment occurs rapidly and transiently at the injection site in the muscle, as well as in the dLNs (further discussed below).Citation26,Citation28,Citation30,Citation31 Gene enrichment analysis in specific pathogen-free pigs that had received the research equivalent of the clinical-grade Matrix-M adjuvant (AbISCOⓇ-100, Novavax AB) revealed that genes involved in inflammation, innate immunity, and antigen processing, in particular interferons and interferon-related genes, are upregulated at the injection site and/or in the dLN, thus further supporting the early events observed in mice.Citation32
Enhanced antigen delivery and cellularity of draining lymph nodes
In addition to the recruitment of immune cells to the injection site, cellularity also rapidly increases in the dLN following intramuscular injection with vaccine adjuvanted with SBAs. Immune cells (mainly monocytes, DCs, and neutrophils) are recruited to the dLNs shortly after injection of Matrix-M adjuvant, with or without antigen.Citation26,Citation30,Citation31 In a similar manner, the combination of MPL and QS-21 in AS01 induces strong recruitment of monocytes, neutrophils, and different subtypes of conventional DC populations compared to antigen alone.Citation28,Citation33 A recently described SBA, SMNP (saponin/MPLA nanoparticles), increased the lymph flow in a histamine-dependent manner.Citation21 Increased lymph flow allows for rapid drainage of antigen, adjuvant, and activated immune cells from the injection site to the dLN. Indeed, studies using fluorescently-labeled QS-21 (formulated with MPL into AS01) showed that the adjuvant and the antigen can be readily detected both at the injection site and in the dLN at early time points (0.5 and 3 hours post-immunization), but exclusively in the dLN after 24 hours.Citation28 The rapid delivery of both the antigen and the adjuvant to the dLN through local recruitment of inflammatory cells and/or enhanced lymph flow is consistent with the observation that spatiotemporal colocalization of adjuvant and antigen is necessary for the adjuvant effect of SBAs. When antigen was administered 24–72 hours after AS01, or when antigen and adjuvant were injected in contralateral limbs, the adjuvant effect was suppressed, and the antigen-specific responses were not significantly different from those obtained with unadjuvanted antigen.Citation28 This localization of the effect of SBAs, which has also been shown with Matrix-M, demonstrates that the action of SBAs is dependent on concentrating the antigen and activated immune cells in the same site, rather than inducing a systemic response.Citation16,Citation30 In a direct comparison to non-SBAs including aluminum hydroxide, complete Freund’s adjuvant (mineral oil mixed with killed mycobacteria that is restricted to non-human use because of its toxicity), and AS03 (oil-in-water emulsion containing squalene and α-tocopherol), Matrix-M adjuvant-treated mice had larger dLNs with a higher total cell count, thus indicating that the potent immune activation was focused to the dLNs in these animals.Citation34 Together, these findings suggest that the antigen dose-sparing capacity of Matrix-M adjuvantCitation35,Citation36 may rely on the fact that the antigen is transported to dLNs where the adjuvant has “set the stage” for optimal activation and induction of potent antigen-specific adaptive immune responses. Matrix-M adjuvant-induced leukocyte recruitment to the dLN peaks at 48 hours post-injection.Citation31 Similar kinetics are observed for AS01.Citation28 Increased activity in the dLNs is also observed in pigs, where Matrix-M adjuvant induced dLN enlargement, thus indicating cell recruitment and/or proliferation.Citation37
Inflammasome activation
Whether cellular uptake of SBAs and the antigen occurs at the injection site (followed by cellular transport) or in the dLN (after free-flow through the lymph), or a combination of the two, is not fully understood. However, more is known about what happens to the cells that take up the adjuvant particles. Cellular uptake of SBAs such as QS-21 has been suggested to be mediated via a cholesterol-dependent mechanism followed by trafficking to lysosomes.Citation38 Whereas the association of Quillaja saponins with cholesterol in Matrix-M adjuvant is extremely stable in neutral buffers, this association is seemingly quickly broken down at lower pH in the lysosome. Free saponins released from the Matrix-M particles may then induce lysosomal membrane destabilization, causing leakage of lysosomal enzymes and endocytosed antigens into the cytosol of APCs.Citation10,Citation38,Citation39 This appears to trigger inflammasome activation, as well as Syk- and cathepsin B-dependent cell activation and cytokine production.Citation38 In addition, saponin-induced destabilization of lysosomal membranes generates reactive oxygen species that in turn can contribute to inflammasome activation.Citation39 Inflammasomes are cytoplasmic multiprotein complexes that play an important role in innate immunity.Citation40 In response to infections and cellular damage sensed by a variety of pattern recognition receptors, inflammasomes mediate maturation and secretion of the pro-inflammatory cytokines IL-1β and IL-18 through caspase-1 activation.Citation39,Citation41 These two cytokines can enhance NK cell activation (resulting in IFN-γ production) and Th1 responses. Consequently, they are crucial for eliminating intracellular pathogens such as viruses and thus an important target for vaccines.Citation42,Citation43 Indeed, both ISCOMATRIX and AS01 can potently activate the NLRP3 inflammasome in APCs, resulting in the production of IL-1β and IL-18.Citation13,Citation39 A similar NLRP3-dependent release of IL-1β was observed with the research equivalent of Matrix-M, AbISCO-100, administered in combination with MPL.Citation13 However, NLRP3 seems dispensable in different mouse models, suggesting that additional pathways may be involved in vivo.Citation13,Citation38,Citation39,Citation44
Activation of antigen-presenting cells and cross-presentation
In addition to leukocyte recruitment, enhanced activation of immune cells occurs in the dLNs at early time points. The dLNs from mice injected with Matrix-M adjuvant exhibit an increased frequency of DCs expressing the T-cell costimulatory molecule CD86 and the early activation marker CD69, and an increased frequency of CD69-expressing neutrophils and macrophages.Citation34 These effects were not seen with the non-SBAs tested and did not rely on the presence of antigens. Additionally, an increased number of B cells, DCs, and monocytes expressing CD86 and CD69 were found in the dLNs of mice immunized with Matrix-M-adjuvanted influenza hemagglutinin (HA) vaccine.Citation26 Thus, activated antigen-presenting cells are ready to present antigens to T cells and activate them. Antigens in inactivated or subunit vaccines are typically taken up by APCs and processed like other extracellular material, resulting in presentation on MHC-II and activation of CD4+ T cells.
CD8+ T cells, which can attack and destroy infected host cells, contribute to protection against viruses and other intracellular pathogens. However, CD8+ T-cell activation requires antigen presentation in the context of MHC-I molecules, with processing and loading of pathogen proteins taking place in the host cell cytosol.Citation45 Achieving this is generally difficult with inactivated or subunit vaccines,Citation46,Citation47 because they tend to be degraded in the lysosomal compartment and consequently presented exclusively in the context of MHC-II molecules. However, the above-described lysosomal membrane destabilization induced by free saponins released from the SBAs may facilitate antigen translocation from the phagolysosome to the cytosol,Citation38 thus enabling antigen cross-presentation on MHC-I and activation of CD8+ T cells. Indeed, experiments in mouse models have demonstrated that SBAs do facilitate cross-presentation of exogenous antigens on MHC-I in DCs by inducing antigen translocation from endosomes into the cytosol, more specifically through the PKR-like endoplasmic reticulum kinase (PERK) pathway.Citation28,Citation44,Citation48,Citation49 Among the different adjuvants tested (both SBAs and non-SBAs), the highest effect on cross-presentation was obtained with Matrix-C, a component of Matrix-M adjuvant.Citation48 The SBAs specifically induced intracellular lipid bodies (phospholipid monolayers surrounding a core of neutral lipids) in the CD11b+ DC subset both in vitro and in vivo. These lipid bodies were crucial for cross-presentation and subsequent effective cross-priming of CD8+ T cells.Citation48,Citation49 Matrix-M adjuvant is expected to share the same ability to facilitate cross-presentation via saponin-mediated permeabilization of the phagolysosome. Indeed, specific CD8+ T-cell responses have been demonstrated in both mice and humans upon vaccination with Matrix-M-adjuvanted NVX-CoV2373.Citation35,Citation50 Of note, antigen-specific CD8+ T-cell responses are detected after the first or second vaccination in smaller proportion of human subjects.Citation50 Nevertheless, these responses correlate with the antigen-specific CD4+ T-cell response. In addition, a recent comparison of CD8+ T-cell responses between NVX-CoV2373, two mRNA vaccines, and an adenovirus vector vaccine (all against SARS-CoV-2), demonstrated a somewhat lower responder frequency for NVX-CoV2373 compared to the other vaccine platforms.Citation51 However, frequencies of antigen-specific memory CD8+ T cells were comparable to those in SARS-CoV-2 recovered individuals at 6 months. Together, this suggests that Matrix-M can cross a boundary to induce a CD8+ T-cell response, which then could be amplified by the abundant CD4+ T-cell response. Of note, human CD8+ T-cell responses have also been observed with ISCOMATRIX-adjuvanted vaccines.Citation52–54
Enhanced humoral immunity and memory response
SBAs induce high titers of antigen-specific antibodies, a desirable and important outcome of vaccination.Citation14,Citation24 Such antibodies are the result of germinal center interactions, which include B cells as well as T follicular helper (Tfh) cells. Our studies show that Matrix-M-adjuvanted vaccines are potent inducers of both germinal center B cells and Tfh cells, and that long-lived plasma cells are formed.Citation27,Citation35,Citation50 Strong germinal center responses are also observed with other SBAs such as AS01, SMNP, and ISCOMATRIX.Citation21,Citation55,Citation56
Studies using ISCOMATRIX provide further understanding of the impact of Matrix-M on humoral immune responses. Analysis of the antibody repertoire developed in previously-naïve humans vaccinated with a baculovirus/Sf9-derived influenza A/H7N9 virus-like particle (VLP) vaccine in the presence or absence of ISCOMATRIX showed that ISCOMATRIX promotes epitope spreading and antibody affinity maturation.Citation57 This correlated with enhanced hemagglutination inhibition (HAI) and neutralization antibody titers, suggesting that ISCOMATRIX reduced the epitope recognition threshold of immune cells and broadened the number of unique epitopes recognized. Similarly, adjuvanting a novel seasonal influenza recombinant HA subunit vaccine with Matrix-M induced cross-neutralizing and protective antibody responses to multiple historical influenza virus strains in ferrets. This suggested induction of potent antibody responses to conserved broadly neutralizing influenza HA epitopes and the potential for cross-protective responses against future drifted strains.Citation58,Citation59 Although not formally demonstrated yet, Matrix-M most likely presents the same capacity to promote epitope spreading and antibody affinity maturation in humans, thus eliciting durable, high-affinity, and broad immune responses to the antigen.Citation60
Enhanced cell-mediated immunity and memory response
CD4+ Th cells are first activated by antigen presentation in association with MHC-II together with costimulatory signals, but rely on the local cytokine landscape to polarize the response. Both Th1 and Th2 CD4+ T cells support clonal expansion of B cells and drive antibody class-switching, albeit to different isotypes. By secreting the pro-inflammatory cytokines IL-2, IFN-γ, and TNF-α in various combinations, Th1 cells can also promote cell-mediated immunity that is important in protecting against intracellular pathogens. On the other hand, Th2 cells are characterized by the secretion of IL-4, IL-5, IL-10, or IL-13, which promote optimal antibody-mediated immune responses against extracellular pathogens. Hence, the ability to induce balanced or Th1-biased CD4+ T-cell responses may be important in vaccine development, especially when the target is a virus or another intracellular pathogen. Moreover, a strongly Th2-biased response may in fact be detrimental. Such responses have been hypothesized to be responsible for the vaccine-induced enhancement of RSV disease in naïve infants (as reviewed by Carroll and colleagues).Citation61
ISCOMs and Matrix-M adjuvant induce strong Th1-biased CD4+ responses as well as clear cytotoxic (CD8+) T-cell responses in animal models.Citation62–64 Indeed, Matrix-M consistently exhibits a strong ability to induce high levels of antigen-specific IL-2 and IFN-γ production in splenocytes (mainly T cells) in mice when included in different vaccines, including subunit vaccines against SARS-CoV-2, influenza, West Nile, and Ebola viruses.Citation27,Citation34,Citation35,Citation65 In addition, multifunctional memory CD4+ and memory CD8+ T cells secreting IFN-γ, IL-2, and/or TNF-α are induced in mice immunized with an Ebola virus glycoprotein or SARS-CoV-2 spike glycoprotein antigen combined with Matrix-M adjuvant.Citation27,Citation35 Splenocytes from mice immunized with influenza antigen in the presence of Matrix-M adjuvant produced both Th1 and Th2 cytokines upon in vitro re-stimulation, thus also demonstrating the generation of a balanced Th1/Th2 memory.Citation34
A few hours after injection of AS01-adjuvanted vaccines, lymph node-resident cells, mainly NK cells, release IFN-γ in the dLN.Citation66 This production is antigen-independent, controlled by macrophages via IL-18 secretion after inflammasome activation, and supported by IL-12. Both MPL and QS-21 contribute to the transcriptional response in the dLN and the production of IFN-γ at the earliest stages of vaccination. This IFN-γ production is critical for promoting multifunctional Th1-biased antigen-specific CD4+ T-cell responses to AS01-adjuvanted antigens.
Matrix-M adjuvant from preclinical to clinical development
Saponin-based adjuvants, and Matrix-M in particular, have the unique capacity to enhance the magnitude and quality of the antibody response and to simultaneously induce cellular immunity consisting of Th1-dominant CD4+ responses, as demonstrated using different vaccine candidates both in animal models and humans. In addition, a smaller but growing body of evidence suggests that CD8+ T-cell responses are also induced. Altogether, these responses translate into high vaccine efficacy. Some examples of this unique capacity of the Matrix-M adjuvant are presented below.
SARS-CoV-2
The ongoing COVID-19 pandemic caused by SARS-CoV-2 is an ongoing major public health problem. Although vaccines based on mRNA and replication-incompetent adenovirus vectors have been authorized worldwide, manufacturing and distribution of COVID-19 vaccines needed to be achieved at an unprecedented scale.Citation3,Citation67 As of October 2022, approximately 68% of the global population – more than 4 billion persons – have received at least one dose of a COVID-19 vaccine so far, but only 22% of persons in low-income countries.Citation3,Citation67 Additional SARS-CoV-2 vaccines are thus urgently needed to protect the population worldwide and decrease the incidence of cases and the emergence of new variants that pose a greater risk for immune escape.
Novavax developed a SARS-CoV-2 spike nanoparticle vaccine candidate based on previous experience gained in mice with Matrix-M-adjuvanted recombinant nanoparticle vaccines against the severe acute respiratory syndrome coronavirus (SARS-CoV), which emerged in 2003, and the Middle East respiratory syndrome coronavirus (MERS-CoV), which emerged in 2012.Citation68,Citation69 BALB/c mice vaccinated with these vaccines developed high titers of neutralizing antibodies targeted to the respective spike proteins of SARS-CoV and MERS-CoV.Citation68 Furthermore, the MERS-CoV nanoparticle vaccine completely blocked MERS-CoV replication in the lungs and protected mice from infection in vivo.Citation69
NVX-CoV2373 is a nanoparticle vaccine candidate consisting of a recombinant SARS – CoV-2 (rSARS-CoV-2) spike glycoprotein (GP) constructed from the full – length, wild-type sequence from the Wuhan strain (GenBank gene sequence MN908947, nucleotides 21563–25384) optimized for the baculovirus/Sf9 insect cell expression system, stabilized in the prefusion conformation, and adjuvanted with Matrix-M.Citation35,Citation36,Citation70 In mice and non-human primate models, NVX-CoV2373 induced high anti-spike antibody titers that blocked binding to the human angiotensin-converting enzyme 2 (hACE2) receptor on host cells.Citation35,Citation70,Citation71 In the mouse spleen, NVX-CoV2373 also elicited multifunctional CD4+ and CD8+ T cells, CD4+ Tfh cells, and antigen-specific germinal center B cells.Citation35 In animals that received rSARS-CoV-2 spike with Matrix-M, increases in frequencies of antigen-specific CD4+ and CD8+ T cells secreting IFN-γ, TNF-α, and IL-2 (i.e., classical Th1 cytokines) were significantly greater than those of IL-4 and IL-5-secreting cells (i.e, Th2 cytokines). This contrasted with the pattern seen in animals immunized with rSARS-CoV-2 spike alone and confirmed that Matrix-M induces a Th1-dominant response.Citation35
In mice, hamsters, and non-human primates, the antibodies induced by NVX-CoV2373 neutralized wild-type virus and protected against a SARS-CoV-2 challenge, with no evidence of vaccine-associated enhanced respiratory disease.Citation35,Citation70 Where examined, CD4+ T-cell responses demonstrated a balanced or Th1-dominant phenotype in each species. Cynomolgus macaques, immunized with NVX-CoV2373 had little or no detectable replicating SARS-CoV-2 in their respiratory tracts and were protected against upper and lower infection and pulmonary disease following intranasal and intratracheal challenge.Citation70 Furthermore, neutralization of wild-type virus exceeded the magnitude of responses measured in human convalescent serum.Citation70
Based on these promising results, NVX-CoV2373 has been evaluated in clinical trials. A randomized, controlled, phase 1–2 trial was conducted to evaluate the safety and immunogenicity of two intramuscular injections of placebo or NVX-CoV2373 in healthy adults.Citation36 Two different dosages of rSARS-CoV-2 spike protein were evaluated (5 or 25 μg), with (both doses) or without (25 µg only) Matrix-M adjuvant. Both Matrix-M adjuvanted doses of rSARS-CoV-2 spike protein induced high immune responses, with neutralizing antibody levels that closely correlated with anti-spike IgG. At 35 days post-vaccination, NVX-CoV2373 elicited neutralizing antibody responses exceeding those observed in COVID-19 convalescent serum from patients hospitalized with COVID-19.Citation36 The Matrix-M adjuvant had a clear impact on the magnitude of the humoral and T-cell responses and the induction of functional antibodies. Matrix-M induced CD4+ T-cell responses that were biased toward a Th1 phenotype. Furthermore, the immune responses in the 5- and 25-μg adjuvanted vaccine regimens were similar, suggesting that Matrix-M had antigen dose-sparing capacities.
The first results of NVX-CoV2373 clinical efficacy were provided with a phase 2b conducted in South Africa and a phase 3 trial conducted in the UK.Citation5,Citation6 In contrast to the first trials evaluating the efficacy of mRNA vaccines and replication-incompetent adenovirus vectors, the timing of these trials allowed to determine vaccine efficacy not only against the original SARS-CoV-2 Wuhan strain but also against the two emerging variants of concern in post-hoc analyses.Citation5,Citation6,Citation72 These trials demonstrated the very high efficacy of NVX-CoV2373 against PCR-confirmed symptomatic COVID-19 caused by the original SARS-CoV-2 (96.4%; 95% CI, 73.8%–99.5%).Citation5 This high efficacy was maintained against the more transmissible B.1.1.7 variant (alpha) originating from the UK (86.3%; 95% CI, 71.3%–93.5%) and, to a lesser extent, to the highly escaped B.1.351 variant (beta) originating from South Africa (51.0% in all participants; 95% CI, −0.6% to 76.2% and 60.1% in HIV-negative participants 18–84 years of age).Citation5,Citation6 Similar results were found in a large phase 3, randomized, placebo-controlled trial conducted in the United States and Mexico during the first half of 2021 that evaluated the efficacy and safety of NVX-CoV2373 in nearly 30,000 adults who had not had a previous severe SARS-CoV-2 infection.Citation4 Over a period of 3 months, vaccine efficacy was 90.4% (95% CI, 82.9%–94.6%; p < .001). Vaccine efficacy against moderate-to-severe disease was 100% (95% CI, 87.0%–100%) and 92.6% (95% CI, 83.6%–96.7%) against any disease caused by a variant of concern or interest, the alpha variant being the predominant viral genome identified during the study. Therefore, NVX-CoV2373 represents an additional highly effective vaccine in the toolbox for the control of COVID-19 pandemics due to different SARS-CoV-2 strains.
Malaria
The leading malaria vaccine candidate, RTS,S (Mosquirix™, GSK), is composed of AS01-adjuvanted VLPs that contain a recombinant fusion protein consisting of repeated sequences and T-cell epitope domain of the Plasmodium falciparum circumsporozoite protein (CSP) linked to the hepatitis B surface antigen (HbsAg) along with HbsAg. In a large phase 3 trial conducted in children aged 5–17 months, efficacy was 56% over the first year and only 36% (95% CI, 32–41) over a median follow-up of 48 months.Citation73
A second-generation RTS,S-like vaccine candidate, called R21, has been developed to improve protective immunity to malaria.Citation74 In contrast to the RTS,S VLPs, R21 particles contain a single CSP-HBsAg fusion protein leading to a higher CSP antigen density on the VLP surface than in RTS,S. R21 immunogenicity was evaluated with different adjuvants in BALB/c mice.Citation74 R21 was immunogenic at the low dose of 0.5 µg and induced very high levels of CSP-specific antibodies but, in contrast to RTS,S, it induced only minimal antibodies to HBsAg. CSP-specific antibody titers induced by R21 were higher with AbISCO-100, Matrix-M, and squalene-based oil-in-water emulsions than with polyanionic carbomer (Carbopol™) and aluminum hydroxide-based adjuvant (Alhydrogel™), but T-cell induction was significantly higher after administration of R21 with AbISCO-100 or Matrix-M.Citation74 R21 elicited sterile protection against a transgenic sporozoite challenge when administered with AbISCO-100 or Matrix-M, but not with any of the other adjuvants evaluated.
Following these preclinical results, R21 was combined with Matrix-M for clinical development. In a phase 2a trial conducted in the UK using controlled human malaria infection in healthy adults, 3 doses of R21/Matrix-M given 4 weeks apart demonstrated high sterile efficacy (81.8%; n = 11) in the prevention of P. falciparum parasitemia.Citation75 In another phase 2 trial conducted in a malaria endemic area with high transmission in Burkina Faso, R21 (5 µg) adjuvanted with two different doses of Matrix-M (25 or 50 µg) was evaluated in children between 5–17 months of age.Citation76 The R21/Matrix-M vaccine resulted in high titers of malaria-specific antibodies 28 days after the third vaccination, which almost doubled with the highest dose of Matrix-M. This response translated into 74% (95% CI, 63%–82%) efficacy with 25 µg Matrix-M and 77% (95% CI, 67%–84%) with 50 µg Matrix-M after 6 months. This high efficacy was maintained at 12 months, with 77% (95% CI, 67%–84%) efficacy in children who received R21 with the highest dose of Matrix-M. Although anti-CSP antibody titers waned over time, a booster dose administered 12 months after the third dose returned antibody levels to near-peak values.Citation76 A follow-up of this trial showed that high vaccine efficacy was maintained against clinical malaria episodes over 2 years after the primary vaccination series and 1 year after the booster dose.Citation77 A phase 3 clinical trial is currently being conducted across five African countries with different malaria transmission and seasonality.
Seasonal influenza
In contrast to SARS-CoV-2 and malaria vaccines that have been deployed only recently, influenza vaccines have been used since the 1950s. However, to address the gaps limiting the effectiveness of existing influenza vaccines, including the induction of egg-adaptive antigenic changes in vaccine virus hemagglutinins induced by classical egg-based manufacturing processes, highly strain-specific responses, and suboptimal T-cell responses in older adults, various strategies to develop novel vaccines with broader protection and higher effectiveness are under investigation, including adjuvanted recombinant protein and viral vector vaccines.Citation78
Novavax has developed a recombinant Matrix-M-adjuvanted HA nanoparticle influenza vaccine candidate (NIV) produced in the Spodoptera frugiperda Sf9 insect cell/baculovirus system.Citation58,Citation79,Citation80 In ferrets, trivalent NIV (tNIV) administered with Matrix-M elicited HAI and microneutralizing (MN) antibody responses significantly greater than those induced by a licensed trivalent high-dose inactivated influenza vaccine (IIV3-HD; Fluzone™ High-Dose) produced in eggs against homologous A(H3N2), A(H1N1), and B strain viruses. Trivalent NIV with Matrix-M also induced HAI and MN antibodies against a broad range of influenza A(H3N2) subtypes spanning almost 2 decades of influenza seasons (2000–2017), whereas IIV and IIV3-HD-induced responses were narrowly directed against the homologous strains.Citation58 Competition antibody studies have revealed that recombinant hemagglutinin nanoparticles adjuvanted with Matrix-M induce responses to several highly conserved HA head and stem broadly neutralizing HA epitopes and are therefore highly cross-neutralizing against both homologous and antigenically drifted strains.Citation59
In a phase 2 clinical trial, Matrix-M-adjuvanted quadrivalent NIV (qNIV) induced higher HAI antibody responses against homologous and several drifted A(H3N2) strains than either unadjuvanted qNIV or IIV3-HD. Substantially higher induction of multifunctional CD4+ T-cell responses against both vaccine-homologous and drifted A(H3N2) viruses were observed in contrast to IIV3-HD and a licensed quadrivalent recombinant vaccine (RIV4; FluBlok™).Citation79,Citation81 A phase 3 immunogenicity trial conducted in healthy adults≥65 years of age demonstrated immunological non-inferiority of qNIV to a licensed IIV4 in terms of HAI antibody responses against the four homologous strains in assays using egg-derived hemagglutinins.Citation80 However, when HAI antibody responses were evaluated with wild-type hemagglutinins, a more biologically and clinically meaningful assay format, qNIV induced higher HAI antibody responses against 4 homologous A(H3N2) strains (24%–66% improvements) and 6 antigenically distinct A(H3N2) strains (34%–46% improvements) compared to IIV4. Furthermore, qNIV induced markedly higher total and effector (memory) CD4+ T cells specific for A(H3N2) and B/Victoria strain HA epitopes, with 126%–189% increases compared to IIV4 in the 7 days after vaccination. Most of these CD4+ effector T cells produced multiple Th1 phenotype cytokines. Notably, substantial multifunctional CD4+ T-cell responses were induced after vaccination with qNIV even in participants with low baseline CD4+ T-cell responses, in sharp contrast to those in the IIV4 group.Citation80 Altogether, these results suggest that Matrix-M-adjuvanted qNIV may be more effective, in the current setting of rapid HA evolution, than the currently licensed influenza vaccines, especially in the high-risk population of older adults, because it induces both antibodies and multifunctional CD4+ T-cell responses broadly cross-reactive against potential future drifted stains.
Pandemic influenza
In preclinical murine studies, a Matrix-M-adjuvanted A(H5N1) vaccine formulated as VLPs (virosomal vaccine) induced higher levels of multifunctional CD4+ T cells and protection against pathogenic A(H5N1) challenge than the vaccine alone.Citation62,Citation82 A phase I clinical trial conducted in adults showed that Matrix-M significantly enhanced CD4+ Th1 responses (as measured by intracellular staining for IL-2, IFN-γ, and TNF-α) and seroprotection rate compared to A(H5N1) VLPs alone, indicating a dose-sparing effect.Citation83 In addition, Matrix-M enhanced long-term Th1 responses. Although not demonstrated for Matrix-M, a clinical trial evaluating influenza A(H7N9) VLPs also showed that ISCOMATRIX has dose-sparing capacity for the induction of functional HAI and neuraminidase inhibition antibodies.Citation84
Ebola virus
Following the large Ebola outbreaks that occurred in several West African countries between 2013 and 2016, Novavax developed a recombinant Ebola virus (EBOV) GP nanoparticle vaccine formulated with Matrix-M.Citation27,Citation85 In BALB/c mice, EBOV GP co-administered with Matrix-M was significantly more immunogenic than GP co-administered with aluminum phosphate or GP without adjuvant in terms of antigen-specific IgG, virus neutralization titers, and cellular responses.Citation27 Notably, and consistent with results observed in other systems, the EBOV GP/Matrix-M formulation converted the Th2-dominant response to the unadjuvanted or aluminum-adjuvanted GP antigen (as indicated by its minimal IgG2a antibody production), to a balanced Th1/Th2 CD4+ T-cell response (as indicated by similar IgG1 and IgG2a GP-specific antibody levels).Citation27 Immunization with EBOV GP/Matrix-M was 100% protective against a lethal viral challenge, whereas only 10% of mice were protected with the EBOV GP antigen alone and none with the aluminum phosphate.Citation27 Interestingly, two studies evaluating EBOV GP with Matrix-M in cynomolgus macaques suggested a correlation between anti-GP IgG antibodies and survival outcome after vaccination.Citation86
In the first-in-human trial, 230 healthy adults received a placebo or four different doses of EBOV GP antigen, as 1- or 2-dose regimens, with or without Matrix-M.Citation87 Immunization with two doses of EBOV GP/Matrix-M elicited a rapid and robust increase of the GP-specific IgG response, a response that persisted through 1 year, as well as neutralizing antibodies in both wild-type virus and pseudovirion-based assays. These responses were indistinguishable at antigen doses from 6.5 to 50 μg, indicating that Matrix-M has strong antigen dose-sparing capacities.Citation87
Vaccines formulated with Matrix-M adjuvant are well tolerated and have an acceptable safety profile
As of February 2022, vaccines formulated with the Matrix-M adjuvant have been administered to more than 37,000 individuals in a total of 29 clinical trials in the United States, Mexico, Europe, Africa, and Australia (data on file). In these trials, the proportion of subjects with short-term reactogenicity, including local and systemic reactions, was higher among recipients of Matrix-M formulations than recipients of unadjuvanted formulations or placebo. Reactions were, however, of mild-to-moderate intensity and transient (≤2 days) in most cases and represented typical vaccine reactogenicity symptoms that were actively solicited for the first 7 days after vaccination. There were no significant disproportions of unsolicited subject-reported adverse events in any organ system among clinical trial subjects followed for 6 months to 1 year after the administration of these vaccines. The favorable safety profile observed in phase 1–2 trials was confirmed in two phase 3 trials evaluating NVX-CoV2373 that enrolled 15,187 and 29,949 participants, respectively.Citation4,Citation5 Injection-site tenderness and pain, fatigue, headache, and muscle pain were the most frequently reported solicited reactions. Fever in the 7 days after injections was rarely reported (< 5%), and fever > 39°C was rare (< 1%). Reactions were more common after the second dose than after the first one and in participants 18–64 years of age than in those ≥ 65 years of age.Citation5 The frequency of unsolicited adverse events was higher among vaccine recipients than among placebo recipients (25.3% vs. 20.5%), but the majority of this difference consisted of events that were consistent with a reactogenic response. The frequency of serious adverse events was low and similar between groups (0.5% in each).Citation5 No deaths were attributed to NVX-CoV2373. Furthermore, none of the safety signals under observation with other COVID-19 vaccines were reported during the approximately 3-month safety follow-up in the phase 3 trial conducted in the United States and Mexico.Citation4 Indeed, no episodes of anaphylaxis or Guillain – Barré syndrome and no imbalance in myocarditis, pericarditis, and vaccine-induced immune thrombosis with thrombocytopenia were observed in the clinical program. Furthermore, no evidence of vaccine-associated enhanced COVID-19 was found.Citation4,Citation5 As with all novel adjuvants, regulatory authorities continue to monitor the possibility of triggering the onset or worsening of immune-mediated diseases.Citation88 Despite instructions to investigators in clinical trials to report a broad range of potentially immune-mediated diseases, no excess incidence of such illnesses has been observed in recipients of vaccines adjuvanted with Matrix-M in clinical trials to date.
Following emergency and conditional marketing authorization of NVX-CoV2373, reports of anaphylaxis, myocarditis, and pericarditis have been received. These events are considered risks with the NVX-CoV2373 vaccine, but because these events were reported voluntarily, their frequency is currently unknown. Myocarditis and pericarditis occur after other SARS-CoV-2 vaccines without Matrix-M (i.e., mRNA and adenovirus vectors) and thus may be class effects associated with the spike protein target antigen; whereas anaphylaxis occurs with low frequency after many vaccines, with or without adjuvant.Citation89–91 These events are presented here for completeness but their relation to Matrix-M is uncertain.
In summary, although no specific safety concern has been identified so far in clinical trials with vaccines adjuvanted with Matrix-M, the safety profile of NVX-CoV2373 continues to be monitored through post-marketing surveillance.
Conclusions
Over the last 20 years, Matrix-M has proven to be a stable and potent adjuvant that enhances immune responses to a wide variety of subunit antigens both in preclinical models and clinical trials. Matrix-M is notably able to create a favorable immunological environment for antigen presentation in dLNs, as reflected by the rapid recruitment and activation of APCs and leukocytes. The activation of innate immune cells, mediated at least in part by activation of the inflammasome pathway, leads to the production of different cytokines and chemokines, including early production of IFN-γ that is essential for promoting Th1 CD4+ T-cell responses. Consequently, Matrix-M is able to improve the magnitude and quality of the antibody response to the antigen, including the production of functional antibodies, as assessed by the blocking of pathogen-host binding interactions and neutralization in many systems (i.e., seasonal and pandemic influenza viruses, Ebola virus, SARS-CoV, MERS-CoV, and SARS-CoV-2). Matrix-M is also able to induce a strong Th1-dominant immune response, with multifunctional Th1-biased CD4+ T-cells producing IFN-γ, IL-2, or TNF-α as shown for influenza, Ebola, and SARS-CoV-2 vaccines. Matrix-M also has the potential to induce CD8+ T-cell responses through antigen cross-presentation, as supported by a recent study in humans.Citation50 Matrix-M-adjuvanted vaccines promote the recognition of a broader range of conserved epitopes and, thus, have the potential to induce a broad cross-reactive immune response, as observed with influenza or SARS-CoV-2 vaccines. This strong immunogenicity of Matrix-M-adjuvanted vaccines translates into high vaccine efficacy, as illustrated by performance in recognized small animal models for influenza and SARS-CoV-2, non-human primate challenge model for Ebola virus, and, more recently, for the NVX-CoV2373 vaccine candidate in two large-scale phase 3 trials that provided mutually supportive estimates of approximately 90% efficacy in prevention of symptomatic COVID-19.Citation4,Citation5 In addition, Matrix-M has antigen dose-sparing capacities, as shown for SARS-CoV-2, malaria, and Ebola, which help reduce the quantity of antigen per dose or the number of vaccinations required to achieve sufficient protection and, thereby, enhance global vaccine supply notably in case of pandemic situations. Finally, Matrix-M-containing vaccines have a favorable safety profile and are well tolerated. Altogether, these data suggest that Matrix-M meets all the criteria required for novel adjuvants and has therefore the potential to play an important role in public health in the future, as in the context of the ongoing COVID-2019 pandemic.Citation92,Citation93
Acknowledgments
Medical writing support was provided by Drs. Julie Harriague (4Clinics, France) on behalf of Novavax, Inc. The authors thank Emily Adams (Novavax, Inc.) for creation of the figure.
Disclosure statement
KLB, LS, BC, AKP, BZ, SM, CC, and CLA are current employees of Novavax AB. GG, VS, and GS are current employees of Novavax, Inc. LF is a contractor to Novavax, Inc.
Additional information
Funding
References
- Lurie N, Saville M, Hatchett R, Halton J. Developing Covid-19 vaccines at pandemic speed. N Engl J Med. 2020;382(21):1969–13. doi:10.1056/NEJMp2005630.
- Slaoui M, Hepburn M. Developing safe and effective covid vaccines - Operation warp speed’s strategy and approach. N Engl J Med. 2020;383(18):1701–03. doi:10.1056/NEJMp2027405.
- Ritchie H, Mathieu E, Rodés-Guirao L, Appel C, Giattino C, Ortiz-Ospina E, Hasell J, Macdonald B, Diana B, Roser M. Statistics and research: coronavirus (COVID-19) vaccinations. 2020 [accessed 2022 Oct 5]. https://ourworldindata.org/covid-vaccinations.
- Dunkle LM, Kotloff KL, Gay CL, Anez G, Adelglass JM, Barrat Hernandez AQ, Harper WL, Duncanson DM, McArthur MA, Florescu DF, et al. Efficacy and safety of NVX-CoV2373 in adults in the United States and Mexico. N Engl J Med. 2022;386(6):531–43. doi:10.1056/NEJMoa2116185.
- Heath PT, Galiza EP, Baxter DN, Boffito M, Browne D, Burns F, Chadwick DR, Clark R, Cosgrove C, Galloway J, et al. Safety and efficacy of NVX-CoV2373 Covid-19 vaccine. N Engl J Med. 2021;385(13):1172–83. doi:10.1056/NEJMoa2107659.
- Shinde V, Bhikha S, Hoosain Z, Archary M, Bhorat Q, Fairlie L, Lalloo U, Masilela MSL, Moodley D, Hanley S, et al. Efficacy of NVX-CoV2373 Covid-19 vaccine against the B.1.351 variant. N Engl J Med. 2021;384(20):1899–909. doi:10.1056/NEJMoa2103055.
- Di Pasquale A, Preiss S, Tavares Da Silva F, Garcon N. Vaccine adjuvants: from 1920 to 2015 and beyond. Vaccines (Basel). 2015;3(2):320–43. doi:10.3390/vaccines3020320.
- Reed SG, Orr MT, Fox CB. Key roles of adjuvants in modern vaccines. Nat Med. 2013;19(12):1597–608. doi:10.1038/nm.3409.
- Reichert CL, Salminen H, Weiss J. Quillaja saponin characteristics and functional properties. Annu Rev Food Sci Technol. 2019;10(1):43–73. doi:10.1146/annurev-food-032818-122010.
- Lacaille-Dubois MA. Updated insights into the mechanism of action and clinical profile of the immunoadjuvant QS-21: a review. Phytomedicine. 2019;60:152905. doi:10.1016/j.phymed.2019.152905.
- Fleck JD, Betti AH, da Silva FP, Troian EA, Olivaro C, Ferreira F, Verza SG. Saponins from Quillaja saponaria and Quillaja brasiliensis: particular chemical characteristics and biological activities. Molecules. 2019;24(1):171. doi:10.3390/molecules24010171.
- Kensil CR, Patel U, Lennick M, Marciani D. Separation and characterization of saponins with adjuvant activity from Quillaja saponaria Molina cortex. J Immunol. 1991;146(2):431–37. doi:10.4049/jimmunol.146.2.431.
- Marty-Roix R, Vladimer GI, Pouliot K, Weng D, Buglione-Corbett R, West K, MacMicking JD, Chee JD, Wang S, Lu S, et al. Identification of QS-21 as an inflammasome-activating molecular component of saponin adjuvants. J Biol Chem. 2016;291(3):1123–36. doi:10.1074/jbc.M115.683011.
- Marciani DJ. Elucidating the mechanisms of action of saponin-derived adjuvants. Trends Pharmacol Sci. 2018;39(6):573–85. doi:10.1016/j.tips.2018.03.005.
- Waite DC, Jacobson EW, Ennis FA, Edelman R, White B, Kammer R, Anderson C, Kensil CR. Three double-blind, randomized trials evaluating the safety and tolerance of different formulations of the saponin adjuvant QS-21. Vaccine. 2001;19(28–29):3957–67. doi:10.1016/S0264-410X(01)00142-6.
- Didierlaurent AM, Laupeze B, Di Pasquale A, Hergli N, Collignon C, Garcon N. Adjuvant system AS01: helping to overcome the challenges of modern vaccines. Expert Rev Vaccines. 2017;16(1):55–63. doi:10.1080/14760584.2016.1213632.
- Garçon N, Leroux-Roels G, Cheng W-F. Vaccine adjuvants. Perspect Vaccinol. 2011;1(1):89–113. doi:10.1016/j.pervac.2011.05.004.
- Alving CR, Peachman KK, Matyas GR, Rao M, Beck Z. Army Liposome Formulation (ALF) family of vaccine adjuvants. Expert Rev Vaccines. 2020;19(3):279–92. doi:10.1080/14760584.2020.1745636.
- Lovgren Bengtsson K, Morein B, Osterhaus AD. ISCOM technology-based Matrix M adjuvant: success in future vaccines relies on formulation. Expert Rev Vaccines. 2011;10(4):401–03. doi:10.1586/erv.11.25.
- Kishimoto TK, Maldonado RA. Nanoparticles for the induction of antigen-specific immunological tolerance. Front Immunol. 2018;9:230. doi:10.3389/fimmu.2018.00230.
- Silva M, Kato Y, Melo MB, Phung I, Freeman BL, Li Z, Roh K, Van Wijnbergen JW, Watkins H, Enemuo CA, et al. A particulate saponin/TLR agonist vaccine adjuvant alters lymph flow and modulates adaptive immunity. Sci Immunol. 2021;6(66):eabf1152. doi:10.1126/sciimmunol.abf1152.
- Morein B, Sundquist B, Hoglund S, Dalsgaard K, Osterhaus A. Iscom, a novel structure for antigenic presentation of membrane proteins from enveloped viruses. Nature. 1984;308(5958):457–60. doi:10.1038/308457a0.
- Lovgren K, Morein B. The requirement of lipids for the formation of immunostimulating complexes (iscoms). Biotechnol Appl Biochem. 1988;10(2):161–72. doi:10.1111/j.1470-8744.1988.tb00012.x.
- Drane D, Gittleson C, Boyle J, Maraskovsky E. ISCOMATRIX adjuvant for prophylactic and therapeutic vaccines. Expert Rev Vaccines. 2007;6(5):761–72. doi:10.1586/14760584.6.5.761.
- Garcia A, Lema D. An updated review of ISCOMSTM and ISCOMATRIXTM vaccines. Curr Pharm Des. 2016;22(41):6294–99. doi:10.2174/1381612822666160915161302.
- Magnusson SE, Altenburg AF, Bengtsson KL, Bosman F, de Vries RD, Rimmelzwaan GF, Stertman L. Matrix-M adjuvant enhances immunogenicity of both protein- and modified vaccinia virus Ankara-based influenza vaccines in mice. Immunol Res. 2018;66(2):224–33. doi:10.1007/s12026-018-8991-x.
- Bengtsson KL, Song H, Stertman L, Liu Y, Flyer DC, Massare MJ, Xu RH, Zhou B, Lu H, Kwilas SA, et al. Matrix-M adjuvant enhances antibody, cellular and protective immune responses of a Zaire Ebola/Makona virus glycoprotein (GP) nanoparticle vaccine in mice. Vaccine. 2016;34(16):1927–35. doi:10.1016/j.vaccine.2016.02.033.
- Didierlaurent AM, Collignon C, Bourguignon P, Wouters S, Fierens K, Fochesato M, Dendouga N, Langlet C, Malissen B, Lambrecht BN, et al. Enhancement of adaptive immunity by the human vaccine adjuvant AS01 depends on activated dendritic cells. J Immunol. 2014;193(4):1920–30. doi:10.4049/jimmunol.1400948.
- Schroder K, Hertzog PJ, Ravasi T, Hume DA. Interferon-gamma: an overview of signals, mechanisms and functions. J Leukoc Biol. 2004;75(2):163–89. doi:10.1189/jlb.0603252.
- Bengtsson KL, Karlsson KH, Magnusson SE, Reimer JM, Stertman L. Matrix-M adjuvant: enhancing immune responses by ‘setting the stage’ for the antigen. Expert Rev Vaccines. 2013;12(8):821–23. doi:10.1586/14760584.2013.814822.
- Reimer JM, Karlsson KH, Lovgren-Bengtsson K, Magnusson SE, Fuentes A, Stertman L. Matrix-M adjuvant induces local recruitment, activation and maturation of central immune cells in absence of antigen. PLoS One. 2012;7(7):e41451. doi:10.1371/journal.pone.0041451.
- Ahlberg V, Lovgren Bengtsson K, Wallgren P, Fossum C. Global transcriptional response to ISCOM-Matrix adjuvant at the site of administration and in the draining lymph node early after intramuscular injection in pigs. Dev Comp Immunol. 2012;38(1):17–26. doi:10.1016/j.dci.2012.03.005.
- Bosteels C, Fierens K, De Prijck S, Van Moorleghem J, Vanheerswynghels M, De Wolf C, Chalon A, Collignon C, Hammad H, Didierlaurent AM, et al. CCR2- and Flt3-dependent inflammatory conventional Type 2 dendritic cells are necessary for the induction of adaptive immunity by the human vaccine adjuvant system AS01. Front Immunol. 2020;11:606805. doi:10.3389/fimmu.2020.606805.
- Magnusson SE, Reimer JM, Karlsson KH, Lilja L, Bengtsson KL, Stertman L. Immune enhancing properties of the novel Matrix-M adjuvant leads to potentiated immune responses to an influenza vaccine in mice. Vaccine. 2013;31(13):1725–33. doi:10.1016/j.vaccine.2013.01.039.
- Tian JH, Patel N, Haupt R, Zhou H, Weston S, Hammond H, Logue J, Portnoff AD, Norton J, Guebre-Xabier M, et al. SARS-CoV-2 spike glycoprotein vaccine candidate NVX-CoV2373 immunogenicity in baboons and protection in mice. Nat Commun. 2021;12(1):372. doi:10.1038/s41467-020-20653-8.
- Keech C, Albert G, Cho I, Robertson A, Reed P, Neal S, Plested JS, Zhu M, Cloney-Clark S, Zhou H, et al. Phase 1–2 trial of a SARS-CoV-2 recombinant spike protein nanoparticle vaccine. N Engl J Med. 2020;383(24):2320–32. doi:10.1056/NEJMoa2026920.
- Ahlberg V, Hjertner B, Wallgren P, Hellman S, Lovgren Bengtsson K, Fossum C. Innate immune responses induced by the saponin adjuvant Matrix-M in specific pathogen free pigs. Vet Res. 2017;48(1):30. doi:10.1186/s13567-017-0437-2.
- Welsby I, Detienne S, N’kuli F, Thomas S, Wouters S, Bechtold V, De Wit D, Gineste R, Reinheckel T, Elouahabi A, et al. Lysosome-dependent activation of human dendritic cells by the vaccine adjuvant QS-21. Front Immunol. 2016;7:663. doi:10.3389/fimmu.2016.00663.
- Wilson NS, Duewell P, Yang B, Li Y, Marsters S, Koernig S, Latz E, Maraskovsky E, Morelli AB, Schnurr M, et al. Inflammasome-dependent and -independent IL-18 production mediates immunity to the ISCOMATRIX adjuvant. J Immunol. 2014;192(7):3259–68.
- Zheng D, Liwinski T, Elinav E. Inflammasome activation and regulation: toward a better understanding of complex mechanisms. Cell Discovery. 2020;6:36.
- Swanson KV, Deng M, Ting JP. The NLRP3 inflammasome: molecular activation and regulation to therapeutics. Nat Rev Immunol. 2019;19:477–89.
- Takeda K, Tsutsui H, Yoshimoto T, Adachi O, Yoshida N, Kishimoto T, Okamura H, Nakanishi K, Akira S. Defective NK cell activity and Th1 response in IL-18-deficient mice. Immunity. 1998;8:383–90.
- Martynova E, Rizvanov A, Urbanowicz RA, Khaiboullina S. Inflammasome contribution to the activation of Th1, Th2, and Th17 immune responses. Front Microbiol. 2022;13:851835.
- Duewell P, Kisser U, Heckelsmiller K, Hoves S, Stoitzner P, Koernig S, Morelli AB, Clausen BE, Dauer M, Eigler A, et al. ISCOMATRIX adjuvant combines immune activation with antigen delivery to dendritic cells in vivo leading to effective cross-priming of CD8+ T cells. J Immunol. 2011;187(1):55–63.
- Embgenbroich M, Burgdorf S. Current concepts of antigen cross-presentation. Front Immunol. 2018;9:1643.
- Cunningham AL, Heineman TC, Lal H, Godeaux O, Chlibek R, Hwang SJ, McElhaney JE, Vesikari T, Andrews C, Choi WS, et al. Immune responses to a recombinant Glycoprotein E Herpes Zoster vaccine in adults aged 50 years or older. J Infect Dis. 2018;217(11):1750–60.
- Beura LK, Jameson SC, Masopust D. Is a human CD8 T-Cell vaccine possible, and if so, what would it take? CD8 T-Cell vaccines: to B or not to B? Cold Spring Harb Perspect Biol. 2018;10(9):a028910.
- den Brok MH, Bull C, Wassink M, de Graaf AM, Wagenaars JA, Minderman M, Thakur M, Amigorena S, Rijke EO, Schrier CC, et al. Saponin-based adjuvants induce cross-presentation in dendritic cells by intracellular lipid body formation. Nat Commun. 2016;7:13324.
- Huis In ’t Veld LGM, Ho NI, Wassink M, den Brok MH, Adema GJ. Saponin-based adjuvant-induced dendritic cell cross-presentation is dependent on PERK activation. Cell Mol Life Sci. 2022;79(5):231.
- Rydyznski Moderbacher C, Kim C, Mateus J, Plested J, Zhu M, Cloney-Clark S, Weiskopf D, Sette A, Fries L, Glenn G, et al. NVX-Cov2373 vaccination induces functional SARS-CoV-2-specific CD4+ and CD8+ T cell responses. J Clin Invest. 2022;132(19):e160898.
- Zhang Z, Mateus J, Coelho CH, Dan JM, Moderbacher CR, Galvez RI, Cortes FH, Grifoni A, Tarke A, Chang J, et al. Humoral and cellular immune memory to four COVID-19 vaccines. Cell. 2022;185(14):2434–51 e17.
- Davis ID, Chen W, Jackson H, Parente P, Shackleton M, Hopkins W, Chen Q, Dimopoulos N, Luke T, Murphy R, et al. Recombinant NY-ESO-1 protein with ISCOMATRIX adjuvant induces broad integrated antibody and CD4(+) and CD8(+) T cell responses in humans. Proc Natl Acad Sci U S A. 2004;101(29):10697–702.
- Drane D, Maraskovsky E, Gibson R, Mitchell S, Barnden M, Moskwa A, Shaw D, Gervase B, Coates S, Houghton M, et al. Priming of CD4+ and CD8+ T cell responses using a HCV core ISCOMATRIX vaccine: a phase I study in healthy volunteers. Hum Vaccin. 2009;5(3):151–57.
- Cebon JS, Gore M, Thompson JF, Davis ID, McArthur GA, Walpole E, Smithers M, Cerundolo V, Dunbar PR, MacGregor D, et al. Results of a randomized, double-blind phase II clinical trial of NY-ESO-1 vaccine with ISCOMATRIX adjuvant versus ISCOMATRIX alone in participants with high-risk resected melanoma. J ImmunoTher Cancer. 2020;8(1):e000410.
- Pallikkuth S, Chaudhury S, Lu P, Pan L, Jongert E, Wille-Reece U, Pahwa S. A delayed fractionated dose RTS,S AS01 vaccine regimen mediates protection via improved T follicular helper and B cell responses. Elife. 2020;9:e51889.
- Scales HE, Meehan GR, Hayes AJ, Benson RA, Watson E, Walters A, Tomura M, Maraskovsky E, Garside P, Baz Morelli A, et al. A novel cellular pathway of antigen presentation and CD4 T cell activation in vivo. Front Immunol. 2018;9:2684.
- Chung KY, Coyle EM, Jani D, King LR, Bhardwaj R, Fries L, Smith G, Glenn G, Golding H, Khurana S. ISCOMATRIX adjuvant promotes epitope spreading and antibody affinity maturation of influenza a H7N9 virus like particle vaccine that correlate with virus neutralization in humans. Vaccine. 2015;33:3953–62.
- Smith G, Liu Y, Flyer D, Massare MJ, Zhou B, Patel N, Ellingsworth L, Lewis M, Cummings JF, Glenn G. Novel hemagglutinin nanoparticle influenza vaccine with Matrix-M adjuvant induces hemagglutination inhibition, neutralizing, and protective responses in ferrets against homologous and drifted A(H3N2) subtypes. Vaccine. 2017;35:5366–72.
- Portnoff AD, Patel N, Massare MJ, Zhou H, Tian JH, Zhou B, Shinde V, Glenn GM, Smith G. Influenza hemagglutinin nanoparticle vaccine elicits broadly neutralizing antibodies against structurally distinct domains of H3N2 HA. Vaccines (Basel). 2020;8(1):99.
- Alves K, Plested JS, Galbiati S, Chau G, Cloney-Clark S, Zhu M, Kalkeri R, Patel N, Smith K, Marcheschi A, et al. Immunogenicity of a fourth homologous dose of NVX-CoV2373. N Engl J Med. 2023;388(9):857–859.
- Gartlan C, Tipton T, Salguero FJ, Sattentau Q, Gorringe A, Carroll MW. Vaccine-associated enhanced disease and pathogenic human coronaviruses. Front Immunol. 2022;13:882972.
- Madhun AS, Haaheim LR, Nilsen MV, Cox RJ. Intramuscular Matrix-M-adjuvanted virosomal H5N1 vaccine induces high frequencies of multifunctional Th1 CD4+ cells and strong antibody responses in mice. Vaccine. 2009;27:7367–76.
- Morein F, Bengtsson KL. Functional aspects of iscoms. Immunol Cell Biol. 1998;76:295–99.
- Pedersen GK, Sjursen H, Nostbakken JK, Jul-Larsen A, Hoschler K, Cox RJ. Matrix M(TM) adjuvanted virosomal H5N1 vaccine induces balanced Th1/Th2 CD4(+) T cell responses in man. Hum Vaccin Immunother. 2014;10:2408–16.
- Magnusson SE, Karlsson KH, Reimer JM, Corbach-Sohle S, Patel S, Richner JM, Nowotny N, Barzon L, Bengtsson KL, Ulbert S, et al. Matrix-M adjuvanted envelope protein vaccine protects against lethal lineage 1 and 2 West Nile virus infection in mice. Vaccine. 2014;32(7):800–08.
- Coccia M, Collignon C, Herve C, Chalon A, Welsby I, Detienne S, van Helden MJ, Dutta S, Genito CJ, Waters NC, et al. Cellular and molecular synergy in AS01-adjuvanted vaccines results in an early IFNgamma response promoting vaccine immunogenicity. NPJ Vaccines. 2017;2:25.
- Hunter DJ, Abdool Karim SS, Baden LR, Farrar JJ, Hamel MB, Longo DL, Morrissey S, Rubin EJ. Addressing vaccine inequity - Covid-19 vaccines as a global public good. N Engl J Med. 2022;386(12): 1176–1179.
- Coleman CM, Liu YV, Mu H, Taylor JK, Massare M, Flyer DC, Smith GE, Frieman MB. Purified coronavirus spike protein nanoparticles induce coronavirus neutralizing antibodies in mice. Vaccine. 2014;32:3169–74.
- Coleman CM, Venkataraman T, Liu YV, Glenn GM, Smith GE, Flyer DC, Frieman MB. MERS-Cov spike nanoparticles protect mice from MERS-Cov infection. Vaccine. 2017;35:1586–89.
- Guebre-Xabier M, Patel N, Tian JH, Zhou B, Maciejewski S, Lam K, Portnoff AD, Massare MJ, Frieman MB, Piedra PA, et al. NVX-CoV2373 vaccine protects cynomolgus macaque upper and lower airways against SARS-CoV-2 challenge. Vaccine. 2020;38(50):7892–96.
- Tai W, He L, Zhang X, Pu J, Voronin D, Jiang S, Zhou Y, Du L. Characterization of the receptor-binding domain (RBD) of 2019 novel coronavirus: implication for development of RBD protein as a viral attachment inhibitor and vaccine. Cell Mol Immunol. 2020;17:613–20.
- Cascella M, Rajnik M, Aleem A, Dulebohn SC, Di Napoli R. Features, evaluation, and treatment of coronavirus (COVID-19). Treasure Island (FL): StatPearls; 2022.
- The RTS;S Clinical Trials Partnership. Efficacy and safety of RTS,S/AS01 malaria vaccine with or without a booster dose in infants and children in Africa: final results of a phase 3, individually randomised, controlled trial. Lancet. 2015;386(9988):31–45.
- Collins KA, Snaith R, Cottingham MG, Gilbert SC, Hill AVS. Enhancing protective immunity to malaria with a highly immunogenic virus-like particle vaccine. Sci Rep. 2017;7:46621.
- Venkatraman N, Bowyer G, Edwards N, Griffiths O, Powlson J, Silman D, Morter R, Folegatti P, Minassian AM, Poulton I, et al. High level efficacy in humans of a next-generation plasmodium falciparum anti-sporozoite vaccine: r21 in Matrix-MTM adjuvant. Am J Trop Med Hyg. 2017;97:594.
- Datoo MS, Natama MH, Some A, Traore O, Rouamba T, Bellamy D, Yameogo P, Valia D, Tegneri M, Ouedraogo F, et al. Efficacy of a low-dose candidate malaria vaccine, R21 in adjuvant Matrix-M, with seasonal administration to children in Burkina Faso: a randomised controlled trial. Lancet. 2021;397(10287):1809–18.
- Datoo MS, Natama HM, Some A, Bellamy D, Traore O, Rouamba T, Tahita MC, Ido NFA, Yameogo P, Valia D, et al. Efficacy and immunogenicity of R21/Matrix-M vaccine against clinical malaria after 2 years’ follow-up in children in Burkina Faso: a phase 1/2b randomised controlled trial. Lancet Infect Dis. 2022;22(12):1728–1736.
- Paules CI, Sullivan SG, Subbarao K, Fauci AS. Chasing seasonal influenza - the need for a universal influenza vaccine. N Engl J Med. 2018;378:7–9.
- Shinde V, Cai R, Plested J, Cho I, Fiske J, Pham X, Zhu M, Cloney-Clark S, Wang N, Zhou H, et al. Induction of cross-reactive hemagglutination inhibiting antibody and polyfunctional CD4+ T-cell responses by a recombinant Matrix-M-Adjuvanted Hemagglutinin nanoparticle influenza vaccine. Clin Infect Dis. 2020;73(11):e4278–e4287.
- Shinde V, Cho I, Plested JS, Agrawal S, Fiske J, Cai R, Zhou H, Pham X, Zhu M, Cloney-Clark S, et al. Comparison of the safety and immunogenicity of a novel Matrix-M-adjuvanted nanoparticle influenza vaccine with a quadrivalent seasonal influenza vaccine in older adults: a phase 3 randomised controlled trial. Lancet Infect Dis. 2022;22(1):73–84.
- Shinde V, Fries L, Wu Y, Agrawal S, Cho I, Thomas DN, Spindler M, Lindner E, Hahn T, Plested J, et al. Improved titers against influenza drift variants with a nanoparticle vaccine. N Engl J Med. 2018;378(24):2346–48.
- Cox RJ, Pedersen G, Madhun AS, Svindland S, Saevik M, Breakwell L, Hoschler K, Willemsen M, Campitelli L, Nostbakken JK, et al. Evaluation of a virosomal H5N1 vaccine formulated with Matrix M adjuvant in a phase I clinical trial. Vaccine. 2011;29(45):8049–59.
- Pedersen GK, Madhun AS, Breakwell L, Hoschler K, Sjursen H, Pathirana RD, Goudsmit J, Cox RJ. T-helper 1 cells elicited by H5N1 vaccination predict seroprotection. J Infect Dis. 2012;206:158–66.
- Fries LF, Smith GE, Glenn GM. A recombinant virus like particle influenza a (H7N9) vaccine. N Engl J Med. 2013;369:2564–66.
- Sharma AR, Lee YH, Nath S, Lee SS. Recent developments and strategies of Ebola virus vaccines. Curr Opin Pharmacol. 2021;60:46–53.
- Triplett CA, Niemuth NA, Cirimotich C, Meister G, Guebre-Xabier M, Patel N, Massare M, Glenn G, Smith G, Alfson KJ, et al. Immune correlates of protection from filovirus efficacy studies in non-human primates. Vaccines (Basel). 2022;10(8):1338.
- Fries L, Cho I, Krahling V, Fehling SK, Strecker T, Becker S, Hooper JW, Kwilas SA, Agrawal S, Wen J, et al. Randomized, blinded, dose-ranging trial of an Ebola virus glycoprotein nanoparticle vaccine with Matrix-M adjuvant in healthy adults. J Infect Dis. 2020;222(4):572–82.
- Tavares Da Silva F, De Keyser F, Lambert PH, Robinson WH, Westhovens R, Sindic C. Optimal approaches to data collection and analysis of potential immune mediated disorders in clinical trials of new vaccines. Vaccine. 2013;31:1870–76.
- Al-Ali D, Elshafeey A, Mushannen M, Kawas H, Shafiq A, Mhaimeed N, Mhaimeed O, Mhaimeed N, Zeghlache R, Salameh M, et al. Cardiovascular and haematological events post COVID-19 vaccination: a systematic review. J Cell Mol Med. 2022;26(3):636–53.
- McNeil MM, DeStefano F. Vaccine-associated hypersensitivity. J Allergy Clin Immunol. 2018;141:463–72.
- Paul P, Janjua E, AlSubaie M, Ramadorai V, Mushannen B, Vattoth AL, Khan W, Bshesh K, Nauman A, Mohammed I, et al. Anaphylaxis and related events following COVID-19 vaccination: a systematic review. J Clin Pharmacol. 2022;62(11):1335–49.
- Nanishi E, Dowling DJ, Levy O. Toward precision adjuvants: optimizing science and safety. Curr Opin Pediatr. 2020;32:125–38.
- He Q, Mao Q, Zhang J, Bian L, Gao F, Wang J, Xu M, Liang Z. COVID-19 vaccines: current understanding on immunogenicity, safety, and further considerations. Front Immunol. 2021;12:669339.