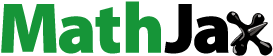
ABSTRACT
Vaccine sedimentation and resuspension are properties that vaccine makers use to characterize a suspension product during research and development as well as throughout the shelf life of the vaccine. Three vaccines with three different aluminum adjuvants and different antigens were selected and monitored over the course of sedimentation using water proton nuclear magnetic resonance (wNMR) relaxometry. This simple method measured fully intact, single-dose vaccine vials and reported sedimentation profiles for each, which readily distinguished freeze-stressed vaccines from unstressed vaccines.
Introduction
Aluminum salt microparticles are one of the most widely used vaccine adjuvants. Since the aluminum microparticles are heavier than water, these vaccines naturally settle under gravity. Characterizing and manufacturing suspension vaccines is non-trivial. The vaccines need to withstand shear forces (e.g. mixing, pumping, filling)Citation1 and long-term storage conditions, which ultimately affect the redispersion behavior of the vaccine.Citation2 Optimizing these vaccines during development and scale-up, and understanding the effects of various manufacturing conditions requires methods that characterize suspensions. Commonly used methods for characterizing aluminum-adjuvanted vaccines include microscopy for particle size and morphology, zeta potential for surface charge, and light transmission/backscattering under centrifugal or gravitational forces for sedimentation kinetics.Citation3,Citation4
Adding to the complexity of characterizing suspension vaccines, aluminum-adjuvanted vaccines are both heat- and freeze-sensitive, with freezing being a greater danger to the vaccine integrity than mild heat exposure, according to the World Health Organization (WHO).Citation5 Once a vaccine vial experiences a freeze/thaw (F/T) event, it loses its potency irreversibly.Citation5,Citation6 The visual appearance of fully dispersed aluminum-adjuvanted vaccines, which are whitish aqueous suspensions, does not change drastically after F/T. At the microscopic scale, however, aluminum microparticles of vaccines undergo various changes upon F/T, such as dehydration and loss of hydroxyls at the particle surface, antigen desorption and particle agglomeration.Citation7–9
Freeze-damaged agglomerated aluminum microparticles settle faster than those that have never been frozen. This forms the basis for the WHO Shake Test, the currently used qualitative technique for detecting freeze-damaged aluminum-adjuvanted vaccines. In the WHO Shake Test, a trained technician visually compares the settling rate of select vaccine vials to determine whether a batch of vaccines has been accidentally frozen.Citation9,Citation10 The Shake Test requires a sufficient sample volume, several milliliters, and peeling off the label in order to visually observe the vaccine suspension settling behavior. A practical difficulty in implementing the Shake Test is that most single-dose aluminum-adjuvanted vaccines are formulated as 0.5-mL or 1.0-mL volumes in vials or prefilled syringes, making in situ (i.e., in the original vial or syringe) visual observation of their sedimentation process not feasible. Typically, multiple single-dose vials are pooled together for the WHO Shake Test. Frequent cold-chain breaches and thermal excursionsCitation11,Citation12 underscore the importance of techniques that can quantitatively assess the quality of aluminum-adjuvanted vaccines in lieu of qualitative visual inspection.
Water proton nuclear magnetic resonance (wNMR) is a noninvasive analytical technique with much potential in the pharmaceutical industry where measurements on process streams or products can inform decision-making in real time. While the 1H populations from both the solvent and solutes are measured at once, for liquid dosage forms of pharmaceutical products, the overwhelming majority of 1H signal comes from the solvent, water. So, it is the water protons that report on the solutes that are dissolved in water, such as antigens or excipients, or the particles suspended in the water, such as aluminum adjuvants. wNMR could serve as a robust way to rapidly assess the quality of a pharmaceutical product. Protein aggregate formation can be detected and quantified,Citation13 and final product content (concentration) deviations in a suspension insulin product can be detected in situ.Citation14
We have previously applied wNMR to detect F/T-damaged aluminum-adjuvanted vaccines. It was shown that a single wNMR parameter, the water proton transverse relaxation rate, R2(1H2O), can readily distinguish F/T-damaged vials from properly stored vials (i.e., unstressed vials).Citation15 We also applied wNMR to monitor the sedimentation kinetics of free aluminum adjuvants dispersed in water in a volume representative of multi-dose vials (4 mL).Citation16 Here we applied wNMR to monitor the sedimentation kinetics of marketed aluminum-adjuvanted vaccine products (i.e. antigen-adsorbed to aluminum microparticles in formulation) presented in single-dose vials. The goal is to overcome two limitations of the WHO Shake Test: reliance on human inspectors and inapplicability to single-dose vials.
Materials and methods
Vaccines and freeze-thaw stress procedure
Three vaccines were used in this study, including Daptacel® (Sanofi) against diphtheria, tetanus and pertussis, Engerix-B® (GSK) against Hepatitis B virus, and VAQTA® (Merck) against Hepatitis A virus. They respectively contain aluminum phosphate (AP, at 0.66 mg/mL of Al(III)), aluminum hydroxide (AH, at 0.5 mg/mL of Al(III)), and amorphous aluminum hydroxyphosphate sulfate (AAHS, at 0.45 mg/mL of Al(III)) as the adjuvant. They are all presented in single-dose glass vials sealed with rubber septa and aluminum caps (). The suspension volume in Daptacel®, Engerix-B® and VAQTA® is respectively 0.5, 1.0 and 1.0 mL.
Figure 1. Noninvasive wNMR measurements of vaccines. (a). Daptacel®, Engerix-B®, and VAQTA® (left to right) vials contained 0.5-, 1.0-, and 1.0- mL aqueous vaccine suspension respectively. (b). The label mostly obscures the view of the suspension inside the vials, but the bottom view of vials laying on their side shows the suspension inside the vials. (c). Measurements were made on sealed, intact vials with labels, that were lowered, via a thread looped around the vial neck, into an inner and outer glass NMR tube situated inside the NMR probe. (d). View of vial inside inner and outer glass NMR tubes. (e). Temperature-controlled benchtop NMR relaxometer (Resonance Systems GmbH) with an arrow indicating the bore location for sample lowering into probe.
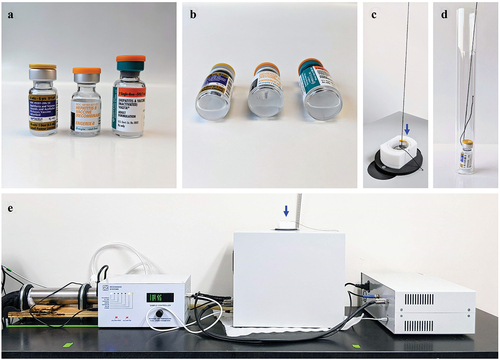
One vial of each vaccine was subjected to three cycles of −18°C for ~18 hr (freezing condition) and then 5°C for 1.5–2.0 hr (thawing condition). Before and after the F/T cycles, the vial was stored at 5°C. Unstressed control vials were stored at 5°C throughout.
NMR instrumentation
A wide-bore, variable temperature benchtop NMR analyzer (Resonance Systems GmbH, Germany) with a 1H resonance frequency of 22.4 MHz (0.52 T, magnet temperature 22°C) was used for all NMR measurements of vaccines. Measurements had a set probe cavity temperature of 4.5°C and the actual sample temperature measured by a built-in, calibrated infrared thermometer ranged from 4–5°C. The room temperature was maintained at ~ 19°C. Intact single-dose vials were inserted into the benchtop NMR bore, one at a time, for data collection (). Vials were centered inside the NMR by placing them into a 20-mm O.D. round bottom glass inner tube, which was inside a 26-mm O.D. flat bottom glass outer tube (). shows the benchtop NMR set-up, including the temperature control unit, with an arrow indicating where the vial is lowered into the NMR probe.
wNMR acquisition parameters and experimental procedure
The applied Carr-Purcell-Meiboom-Gill (CPMG) NMR pulse sequence resulted in spin-echo intensity decays over time. Transverse relaxation (spin-spin relaxation) rate constants were extracted from the single-exponential fit of the said decay of spin-echo intensities using the following equation:
where t is time (0 ≤ t ≥ 9 s), I(t) is the recorded spin-echo intensity at time t, and I(0) is the initial spin-echo intensity at time t = 0. The built-in NMR analyzer software, Relax8, operated the instrument and controlled the data collection. Acquisition parameters for all vaccines were automatically set by the instrument software based on an anticipated T2 of 1.5 s. The relaxation delay (RD), also called relaxation period, was 8 s in the first set of sedimentation measurements (July-Oct. 2020) and 12 s in the second set of sedimentation measurements (Sept. 2022). The 90° pulse length (P90°) was 18 μs and 180° pulse length (P180°) was 40 μs throughout, calibrated using a mineral oil standard (4 mL), and the inter-pulse delay of the CPMG pulse sequence (τ) was 0.5 ms. The number of scans (NS) was 4 for all experiments. An automated program script enabled multiple consecutive CPMG experiments to monitor sedimentation in regular periodicity of time. In the first set of sedimentation experiments (July-Oct. 2020), the automated script recorded data approximately every 6 min 20 sec, which included the CPMG measurement and tuning taking 1 min 20 sec followed by a 5-min wait period between adjacent CPMG measurements, and in the second set of sedimentation experiments (Sept. 2022), it recorded data approximately every 3 min 14 sec, which included the CPMG measurement and tuning taking 1 min 44 sec followed by a 1.5-min wait period between adjacent CPMG measurements, for a total of 13 and 24 R2(1H2O) values over the course of the sedimentation process, respectively. In the Relax8 software, for the first set of sedimentation experiments (July-Oct. 2020), a total of ~ 9000 spin-echoes were collected where the average intensity of every 16th spin-echo was recorded such that the total number of recorded echoes was 562, and for the second set of sedimentation experiments (Sept. 2022), a total of ~12,000 spin-echoes were collected where the average intensity of every 21st spin-echo was recorded such that the total number of recorded spin-echoes was 571. Each CPMG decay of spin-echo intensity, I(t), was fit using the Origin 2019b software (OriginLab Corp., Northampton, MA), and the data of the R2(1H2O) changes over the sedimentation time, T, were also plotted using Origin 2019b.
Vaccine vials were measured intact, without opening the septa/cap seal or removing the label. NMR measurements do not require the sample to be optically transparent. Vaccine vials were lowered into the NMR tubes inside the NMR probe and retrieved from the NMR probe via a thread that was looped around the necks of the vaccine vials. All vaccine vials were temperature equilibrated inside the NMR probe for 40 min before data collection. For the first set of sedimentation experiments (July-Oct. 2020), the already settled vaccine vials were not shaken before lowering into the probe for temperature equilibration. After 40 min equilibration, the vial was quickly retrieved from the probe, manually inverted 20 times to fully disperse the vaccine, and swiftly lowered again into the NMR to begin measurement. For the second set of sedimentation experiments (Sept. 2022), the already settled vials were vigorously shaken by hand before lowering into the NMR probe for temperature equilibration. After 40 min equilibration, the vial was quickly retrieved from the probe, manually inverted 10 times to fully disperse the vaccine, and swiftly lowered to begin measurement. Inversions of the vials were done manually by handling only the aluminum cap of the vials to minimize warming.
Results and discussion
The sedimentation process of three suspension vaccines, Daptacel®, Engerix-B® and VAQTA®, inside sealed single-dose vaccine vials was monitored using wNMR. Neither the qualitative WHO Shake Test nor quantitative optical analytical techniques can monitor vaccine sedimentation in single-dose vials because the volume is too small for visual or optical tracking of suspension settling.Citation2,Citation17,Citation18 For the Shake Test and optical analytical techniques, the vial label poses another obstacle.
The wNMR parameter used for the observation of sedimentation was the transverse relaxation rate of water protons, R2(1H2O). At the start of the measurement, the vaccine suspension was inverted multiple times to fully disperse the vaccine. Data collection and sedimentation commenced immediately after the vaccine vial was lowered into the probe cavity of benchtop NMR (). Hence, the first R2(1H2O) data point represents the suspension roughly 1 min 20 sec into the sedimentation process for the first set of sedimentation experiments (July-Oct. 2020), and 1 min 44 seconds into the sedimentation process for the second set of sedimentation experiments (in Sept. 2022) rather than 0 min of sedimentation time. As sedimentation proceeds and a sediment begins to form, the R2(1H2O) decreases due to a dearth of aluminum microparticles in the supernatant that are left to affect the water proton relaxation.Citation16 Eventually, R2(1H2O) reaches a plateau, indicating the sedimentation has completed. For the three unstressed vaccines, it took about 1 hr for R2(1H2O) to reach plateau (). Previously, we have observed that at an Al(III) concentration of 0.5 mg/mL, it took free AP in water about 80 hr for R2(1H2O) to reach plateau and free AH in water about 160 hr for R2(1H2O) to reach plateau.Citation16 Note that Daptacel® and Engerix-B® respectively contain 0.66 mg/mL Al(III) as AP and 0.5 mg/mL Al(III) as AH. Hence, compared with free adjuvant microparticles of similar Al(III) content, the antigen-adjuvant microparticles settle at a much faster rate than free aluminum adjuvants dispersed in water. The much faster settling rate of antigen-adjuvants is probably because the antigen adsorbed on the aluminum microparticle surface and the formulation excipients reduce the electrostatic repulsion between aluminum microparticles, thereby hastening sedimentation.
Figure 2. Vaccine sedimentation monitored by wNMR for 2 hr 40 min. R2(1H2O) was measured approximately every 3 min 14 sec over the course of vaccine sedimentation for Daptacel® (a), Engerix-B® (b), and VAQTA® (c) where the unstressed vaccines (black) were compared with F/T-stressed vaccines that were exposed to subzero temperatures (red). Daptacel® and Engerix-B® were observed to have frozen, while VAQTA® was not frozen upon subzero temperature exposure. Sedimentation was monitored at 5°C on thawed (if frozen) vials fully dispersed at the beginning of the measurement.
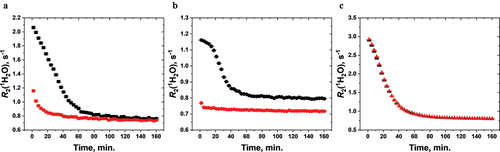
The WHO Shake Test rests on the visual observation that aluminum-adjuvanted vaccines that experience F/T incidences settle at a much faster rate than those vaccines that do not experience F/T incidences.Citation9 This visual observation is quantitatively confirmed here by wNMR. One vial of each of Daptacel®, Engerix-B® and VAQTA®, was exposed thrice to −18°C for 18 hr. Vials of Daptacel® and Engerix-B® solidly froze after each exposure while the vial of VAQTA® never froze. As shown in , the F/T-stressed vials of Daptacel® and Engerix-B® settle much faster than their unstressed controls. This is particularly striking for the F/T-stressed Engerix-B® vial; its R2(1H2O) reached plateau within minutes. Also, the fully dispersed F/T-stressed vials of Daptacel® and Engerix-B® have a much lower initial R2(1H2O) than their unstressed controls (first data point in ), an observation that we have reported previously.Citation15 The VAQTA® vial never froze despite being exposed thrice to freezing temperatures. Its sedimentation time course, as judged by R2(1H2O, T), is nearly identical to that of the unstressed control vial (). Combined, these sedimentation results suggest that wNMR can distinguish between vaccine vials that have actually experienced F/T events () and vaccine vials that were merely exposed to freezing temperatures but never froze ().
For Daptacel®, stressed and unstressed vaccine vials have approximately the same R2(1H2O) value after sedimentation (), whereas for Engerix-B®, the F/T-stressed vaccine vial plateaus at a much lower R2(1H2O) than the unstressed vial (). However, after ca. two weeks of storage, stressed and unstressed vaccine vials for both Daptacel® and Engerix-B® have approximately the same R2(1H2O).Citation19 A two-week storage also leads to a smaller R2(1H2O) than a two-hour storage for both Daptacel® and Engerix-B®, suggesting there is further compaction of the sediment layer during long-term storage.Citation19
The noninvasive nature of wNMR measurements means the same vaccine vial can be measured repeatedly over time to monitor its stability (longitudinal stability monitoring). In this work, the sedimentation of both properly stored (unstressed) and F/T-stressed vials of the three vaccines were measured twice over a period of two years, first in July-October of 2020 and then in September of 2022 (). For every vial measured, the two R2(1H2O, T) sedimentation profiles are nearly identical, indicating that neither the unstressed nor the F/T-stressed vials of these vaccines underwent changes during these two years of storage at 2–8°C. The results suggest that there is no recovery of F/T-damaged Daptacel® and Engerix-B®, which is in concordance with the literature regarding the irreversibility of F/T damage to aluminum-adjuvanted vaccines.Citation5,Citation6
Figure 3. Vaccine sedimentation of the same set of unstressed and stressed vials measured two years apart by wNMR. The R2(1H2O, T) of (a) Daptacel®, (b) Engerix-B®, and (c) VAQTA®. Unstressed vaccines: black (Sept. 2022) and green (July-Oct. 2020); Stressed vaccines: red (Sept. 2022) and blue (July-Oct. 2020). F/T-stressed Daptacel® and Engerix-B® were observed to have solidly frozen, while VAQTA® was not frozen upon subzero temperature exposure. For each vial, its two sedimentation profiles measured two years apart almost superimpose each other.
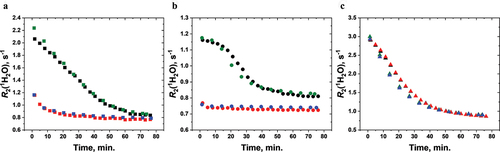
wNMR provides quantitative, instrument-based confirmation of the qualitative, visual observation-based WHO Shake Test. Since wNMR is noninvasive and nondestructive to the product, the only comparable method is human visual inspection. Among the numerous differences between wNMR and the WHO Shake Test, perhaps the most consequential one is that wNMR involves no human inspector and hence is suited for automation while the WHO Shake Test involves trained-human inspectors. Additionally, the WHO Shake Test cannot be applied to single-dose vaccine vials. For the time being, the WHO Shake Test may be more suited than wNMR for locales with minimal resources. However, as this technology advances, it may become better suited for low-resource settings and could gradually replace the WHO Shake Test. The long-term goal is to make wNMR affordable, portable, and easy-to-use with minimal training.
Aluminum-adjuvanted vaccines are formulated as whitish suspensions. The aluminum adjuvant microparticles are heterogenous in size and shape and settle under gravity. As such, they present stiff challenges to analytical techniques. Most, if not all, analytical techniques require dilution to analyze aluminum-adjuvanted vaccines. wNMR, however, can analyze such vaccines as is, without any dilution or sample preparation procedures and, importantly, in the original drug product container. In previous work, wNMR was applied to monitor the sedimentation of free aluminum adjuvants presented in multi-dose vials with a sample volume of 4 mL and sample height of 26–29 mm.Citation16 The present work applies wNMR to marketed vaccines presented in single-dose vials with a sample volume of either 0.5 or 1.0 mL, and sample height of ~7 mm. Small sample volumes and sample heights make sedimentation monitoring difficult, either by optical techniques or visual observations by a human inspector. For example, optical techniques currently used by vaccine makers to monitor aluminum microparticle sedimentation, such as laser scattering (e.g., Turbiscan®), typically require several milliliters of suspension in specialized cuvettes.Citation2 The ability to monitor sedimentation in single-dose vials is a significant improvement over current analytical techniques.
In addition to analyzing finished vaccine products, as shown in this work, wNMR may also be used in the development and production of aluminum-adjuvanted vaccines, as we demonstrated in a recent study in collaboration with vaccine makers.Citation20
Conclusion
wNMR can monitor the sedimentation of aluminum-adjuvanted vaccines in sealed, single-dose vials noninvasively. wNMR demonstrates that F/T-stressed vaccines settle much faster than properly stored vaccines. The noninvasive nature of wNMR allows for the same vaccine vial to be monitored over time and makes it possible to collect data on a vial of a vaccine without compromising the integrity of the vaccine product so that quality vaccines may be used after wNMR testing. wNMR could enhance vaccine quality assurance by quantitatively inspecting vials before release and before injection, using a univariate mode (single R2(1H2O) value), or using a more in-depth multivariate mode (multiple R2(1H2O) values) for product characterization of selected vials as demonstrated here by monitoring sedimentation.
Acknowledgments
We are also grateful to Dr. L. Grunin (Resonance Systems GmbH) for his help with sedimentation kinetics measurement protocol.
Disclosure statement
K.T.B., M.B.T. and Y.B.Y. are coinventors of patents on wNMR technologies owned by the University of Maryland Baltimore.
Additional information
Funding
References
- Kolade OO, Jin W, Tengroth C, Green KD, Bracewell DG. Shear effects on aluminum phosphate adjuvant particle properties in vaccine drug products. J Pharm Sci. 2015;104(2):378–6. doi:10.1002/jps.24127.
- Langford A, Horwitz T, Adu-Gyamfi E, Wiley C, Holding E, Zimmermann D, Ignatius AA, Ohtake S. Impact of formulation and suspension properties on redispersion of aluminum-adjuvanted vaccines. J Pharm Sci. 2020;109(4):1460–6. doi:10.1016/j.xphs.2020.01.006.
- Langford A, Bruchsaler M, Gupta M. 8 - suspension properties and characterization of aluminum-adjuvanted vaccines. In: Kolhe P, Ohtake SBTPA of VD, editors. Practical aspects of vaccine development. Academic Press; 2022. p. 225–66. doi:10.1016/B978-0-12-814357-5.00008-8.
- Mei C, Deshmukh S, Cronin J, Cong S, Chapman D, Lazaris N, Sampaleanu L, Schacht U, Drolet-Vives K, Ore M, et al. Aluminum phosphate vaccine adjuvant: analysis of composition and size using off-line and in-line tools. Comput Struct Biotechnol J. 2019;17:1184–94. doi:10.1016/j.csbj.2019.08.003.
- World Health Organization. Temperature sensitivity of vaccines. WHO/IVB/0610. Published online 2006: 1–58.
- Prevention C for DC and. Vaccine storage and handling toolkit; 2020. https://www.cdc.gov/vaccines/hcp/admin/storage/toolkit/storage-handling-toolkit-2020.pdf.
- Xu AY, Rinee KC, Stemple C, Castellanos MM, Bakshi K, Krueger S, Curtis JE. Counting the water: characterize the hydration level of aluminum adjuvants using contrast matching small-angle neutron scattering. Colloids Surf A Physicochem Eng Asp. 2022;648:129285. doi:10.1016/j.colsurfa.2022.129285.
- Kurzątkowski W, Kartoğlu Ü, Górska P, Główka M, Woźnica K, Zasada AA, Szczepańska G, Trykowski G, Gniadek M, Donten M. Physical and chemical changes in Alhydrogel™ damaged by freezing. Vaccine. 2018;36(46):6902–10. doi:10.1016/j.vaccine.2018.10.023.
- Kartoğlu Ü, Özgüler NK, Wolfson LJ, Kurzątkowski W. Validation of the shake test for detecting freeze damage to adsorbed vaccines. Bull World Health Organ. 2010;88(7):624–31. doi:10.2471/BLT.08.056879.
- Kurzątkowski W, Kartoğlu Ü, Staniszewska M, Górska P, Krause A, Wysocki MJ. Structural damages in adsorbed vaccines affected by freezing. Biologicals. 2013;41(2):71–6. doi:10.1016/j.biologicals.2011.10.011.
- Levinson DR. Vaccines for Children Program: Vulnerabilities in Vaccine Management (OEI-04-10-00430) Office of Inspector General, Department of Health and Human Services; 2012. https://oig.hhs.gov/oei/reports/oei-04-10-00430.pdf.
- Wilson E, Zhu C, Galea S, Marko A, Victoria Urdaneta V, Straus W. Turning up the heat: effect of new vaccine for children’s (VFC) program recommendations for use of temperature monitors upon incorrect product storage adverse event reporting. Vaccine. 2018;36(11):1516–20. doi:10.1016/j.vaccine.2017.10.059.
- Taraban MB, DePaz RA, Lobo B, Yu YB. Use of water proton NMR to characterize protein aggregates: gauging the response and sensitivity. Anal Chem. 2019;91(6):4107–15. doi:10.1021/acs.analchem.8b05733.
- Briggs KT, Taraban MB, Wang W, Yu YB. Nondestructive quantitative inspection of drug products using benchtop NMR relaxometry-the case of NovoMix® 30. Aaps Pharm Sci Tech. 2019;20(5):214. doi:10.1208/s12249-019-1428-6.
- Briggs KT, Taraban MB, Yu YB. Quality assurance at the point-of-care: noninvasively detecting vaccine freezing variability using water proton NMR. Vaccine. 2020;38(31):4853–60. doi:10.1016/j.vaccine.2020.05.049.
- Taraban MB, Yu YB. Monitoring of the sedimentation kinetics of vaccine adjuvants using water proton NMR relaxation. Magn Reson Chem. 2021;59(2):147–61. doi:10.1002/mrc.5096.
- Fox CB. Characterization of TLR4 agonist effects on alhydrogel®; sedimentation: a novel application of laser scattering optical profiling. J Pharm Sci. 2012;101(11):4357–64. doi:10.1002/jps.23307.
- Muthurania K, Ignatius AA, Jin Z, Williams J, Ohtake S. Investigation of the sedimentation behavior of aluminum phosphate: influence of pH, ionic strength, and model antigens. J Pharm Sci. 2015;104(11):3770–81. doi:10.1002/jps.24584.
- Briggs KT, Taraban MB, Yu YB. Using water proton NMR to characterize aluminum-adjuvanted vaccines. In: Mantle M, and Hughes L, editors. Magnetic resonance and its applications in drug formulation and delivery: new developments in NMR. Cambridge: The Royal Society of Chemistry; 2023.
- Taraban MB, Briggs KT, Yu YB, Jones MT, Rosner L, Bhambhani A, Williams DM, Farrell C, Reibarkh M, Su Y. Assessing antigen-adjuvant complex stability against physical stresses by wNMR. Pharm Res. Published online 2022. doi:10.1007/s11095-022-03437-1.